INTRODUCTION
The White Sands dune field is the largest gypsum dune system in the world, derived from deflation of paleo-Lake Otero (McKee, Reference McKee1966; Langford, Reference Langford2003; Kocurek et al., Reference Kocurek, Carr, Ewing, Havholm, Nagar and Singhvi2007; Allen et al., Reference Allen, Love and Myers2009; Ewing, Reference Ewing, Lancaster and Hesp2020). Understanding the timing of initial dune construction, and therefore lake deflation, is critical for understanding regional landscape evolution, including the history of lake desiccation. The onset of dune construction is currently estimated at ~8000 to 6500 cal yr BP (e.g., Langford, Reference Langford2003; Kocurek et al., Reference Kocurek, Carr, Ewing, Havholm, Nagar and Singhvi2007; Langford et al., Reference Langford, Rose and White2009), but numerical age control is limited. This paper provides new age estimates based on archaeological evidence suggesting two older phases of gypsum dune construction.
The gypsum dune field covers ~500 km2 of the northern Tularosa Basin in south-central New Mexico (Ewing Reference Ewing, Lancaster and Hesp2020). This structural and topographic basin flanks the Rio Grande valley along the east margin of the Rio Grande Rift (Hawley, Reference Hawley, Lucas, Morgan and Zeigler2005; Allen et al., Reference Allen, Love and Myers2009). Like other topographic basins in the southwestern and western United States, the Tularosa Basin contained a perennial lake in the late Pleistocene (Allen et al., Reference Allen, Love and Myers2009; Rachal et al., Reference Rachal, Zeigler, Dello-Russo and Solfisburg2021), termed “Lake Otero” by Herrick (Reference Herrick1904). Clastic and evaporitic sediments accumulated in the paleolake ~30 to ~13 cal ka BP (Lucas and Hawley, Reference Lucas, Hawley, Lueth, Giles, Lucas, Kues, Myers and Ulmer-Scholle2002; Allen et al., Reference Allen, Love and Myers2009; Bustos et al., Reference Bustos, Jakeway, Urban, Holliday, Fenerty, Raichlen and Budka2018; Rachal et al., Reference Rachal, Zeigler, Dello-Russo and Solfisburg2021). Deflation created a broad basin (Alkali Flat) where the lake once resided and built the dunes to the east. The dune sediments were clearly derived from the lacustrine evaporites (Szynkiewicz et al., Reference Szynkiewicz, Ewing, Moore, Glamoclija, Bustos and Pratt2010). The dune field consists of a main body with crescentic and barchan dunes roughly oriented perpendicular to the dominant southwesterly winds, flanked to the north, east, and south by fields of parabolic dunes (Ewing et al., Reference Ewing, Kocurek and Lake2006; Kocurek et al., Reference Kocurek, Carr, Ewing, Havholm, Nagar and Singhvi2007).
Onset of deflation of the paleolake beds and construction of the dunes is not well dated. Langford (Reference Langford2003) provides a minimum age estimate of ~7000 14C yr BP. That estimate is not a specific age determination on eolian sediments. It was derived from Buck (Reference Buck1996; also Buck and Monger, Reference Buck and Monger1999), who indicates a significant shift in plant communities at about that time (~7800 cal yr BP) due to drought, dated by radiocarbon dating of pedogenic carbonate. Subsequently, Kocurek et al. (Reference Kocurek, Carr, Ewing, Havholm, Nagar and Singhvi2007) reported OSL dates of ~7.3 ka from lake beds immediately below the center of the main body of the dune field and ~5.2 ka from within the dune body. They supported Langford's age estimate of ~7000 14C yr BP for the onset of dune construction. Langford et al. (Reference Langford, Rose and White2009) subsequently estimated the onset of dune construction at “6500 BP” based on unpublished optically stimulated luminescence (OSL) dating mentioned by Fryberger (Reference Fryberger2001). That date was from a parabolic dune at the northeast end (i.e., far downwind) of the more active parabolic dunes south of the main dune field. Our data now provide both numerical dating of basal eolian sediments from the major dune body and a minimum age estimate for older flanking parabolic dunes.
Use of archaeological evidence to document the evolution of the White Sands is not a new approach. Langford (Reference Langford2003) used archaeological finds to estimate the age of a shoreline. Worman et al. (Reference Worman, Kurota and Hogan2019) provide a remarkable archaeological record that establishes a chronology of dune movement ~4500 to ~1000 cal yr BP based on dating of ancient cooking hearths built in the dunes. The new archaeological data reported here are associated with the Paleoindian and Archaic technocomplexes, the two oldest subdivisions of the archaeological record in the Southwest and across North America (Cordell and McBrinn, Reference Cordell and McBrinn2012). “Paleoindian” is the term applied to the earliest well-established and widely recognized archaeological assemblages left by mobile hunters and gatherers. The artifact assemblages are typified by distinctive time-diagnostic projectile points and associated tools usually made of high-quality raw materials (e.g., fine-grained cherts). They were followed by later so-called “Archaic” foragers who appear to have been less wide ranging and more focused on gathering and using plants. There is relatively limited age control on Paleoindian and Early Archaic archaeology in the Southwest. For the most part, dating is based on the ages of similar artifact assemblages in neighboring regions such as the Great Plains (Ballenger et al., Reference Ballenger, Holliday, Sanchez, Mills and Fowles2017; Holliday et al., Reference Holliday, Harvey, Cuba and Weber2019). By coincidence, the oldest firmly dated archaeological site in the Americas is in the study area and is dated to ~23.0 cal ka BP (Bennett et al., Reference Bennett, Bustos, Pigati, Springer, Urban, Holliday and Reynolds2021). Otherwise, the oldest Paleoindian sites in the region date to ~13.0 cal ka BP and younger and include Clovis and Folsom artifact styles (Ballenger et al., Reference Ballenger, Holliday, Sanchez, Mills and Fowles2017). Some Paleoindian artifacts from the area are likely of earliest Holocene age and are well documented on the Great Plains. The Early Archaic is generally considered to be Early Holocene, but the Paleoindian to Archaic transition is very poorly documented in the Southwest in the field or via numerical age control (McBrinn and Vierra, Reference McBrinn, Vierra, Mills and Fowles2017).
FIELD SITES AND CHRONOLOGY
Dating of the onset of dune construction is clarified by data from two archaeological sites, LA52362 and Gypsum Overlook (LA199959). Site LA52362 is ~7.5 km south of the current southern margin of the active dune belt, including the southmost active parabolic dunes and downwind of the narrower, shallower southern arm of paleo-Lake Otero (Figs. 1 and 2). The archaeological site consists of a scatter of >100 stone artifacts in an area of ~60 × ~40 m draped over the arm of an inactive gypsum parabolic dune (Lee, Reference Lee2015; New Mexico Cultural Resource Information System, https://nmcris.nmhistoricpreservation.org, last accessed June 1, 2022) oriented southwest to northeast (Fig. 3). The dune is heavily weathered compared with the active parabolic dunes in the area, with an eroded low cross-sectional profile (~60 m wide × ~50 cm high) and an extremely dense, hard upper ~20 cm of gypsum crust. These characteristics suggest considerable age, borne out by the archaeological assemblage on top. The artifacts include Folsom- and Plainview-type Paleoindian projectile points (Lee, Reference Lee2015; New Mexico Cultural Resource Information System, https://nmcris.nmhistoricpreservation.org, last accessed June 1, 2022). These time-diagnostic artifacts often co-occur in the region (Holliday et al., Reference Holliday, Johnson and Knudson2017). Dating indicates that they overlapped between ~12.5 and ~12.2 cal ka BP (Holliday et al., Reference Holliday, Johnson and Knudson2017; Buchanan et al., Reference Buchanan, Kilby, Hamilton, LaBelle, Meyer, Holland-Lulewicz and Andrews2021). A Paleoindian affiliation for the site is reinforced by recovery of 14 end scrapers (small unifacial tools, 2–3 cm long and 1–2 cm wide) made of high-quality chert and typical on many Paleoindian sites in the area (Wessell et al., Reference Wessel, Eidenbach, Meyer, Comer and Knight1997; Holliday et al., Reference Holliday, Harvey, Cuba and Weber2019). The presence of Paleoindian artifacts across the dune demonstrates that eolian deposition predated the human occupation and was in place sometime in the post–last glacial maximum (LGM) late Pleistocene (>12.2 cal ka BP).

Figure 1. The northern Tularosa Basin showing the Alkali Flat deflation basin (eroded paleo-Lake Otero) and other selected deflation basins, the White Sands dune field, approximate extent of paleo-Lake Otero high stand (~1204 m; dashed yellow line), local eolian erosion surfaces around the dune field (from figs. 1 and 2 in Allen at al., Reference Allen, Love and Myers2009; fig. 10A in Szynkiewicz et al., Reference Szynkiewicz, Ewing, Moore, Glamoclija, Bustos and Pratt2010), and selected cultural features. Archaeological site LA52362 ("L" on map) is on an ancient, stabilized gypsum parabolic dune (Fig. 2) south of the main dune belt. The Gypsum Overlook archaeological site ("G" on the map) is immediately west (upwind) of the main dune system, buried within a truncated remnant of the main dune belt. The inset shows the location of the White Sands area (WS) within the state of New Mexico. Map by Paul Neville, University of New Mexico.

Figure 2. Google Earth image of the White Sands Missile Range in the area of older, weathered gypsum parabolic dunes. The dashed blue line is the approximate limit of the southern arms of the 1204 m high stand of paleo-Lake Otero (from fig. 1 in Allen et al., Reference Allen, Love and Myers2009). The location of the lake margin is obscured to the northwest by the younger parabolic dunes. The fresh gypsum parabolic dunes and sand sheet at the top are the southern margin of the active main belt of dunes. The older parabolic dunes dominate the area east (downwind) of the paleolake margin between the younger fresh dunes and U.S. 70. LA52362 is in the area northwest of the U.S. 70 sign (see also Fig. 1; precise location cannot be shared publicly; Fig. 3). The “L” at upper right is a roadcut through a gypsum lunette. A similar roadcut is located along U.S. 70 4.9 km southwest of the bottom of the image.

Figure 3. Google Earth imagery of the ancient, stabilized parabolic dunes (orientations identified with red dashed lines; from Gary Kocurek) south of the main White Sands dune system. Archaeological site LA52362 is indicated, draped over the nose of a dune.
The Gypsum Overlook site is located upwind of the main White Sands dune field within an extensive area of eroded gypsum dunes resting on post-LGM lake beds along the eastern margin of the modern deflation basin (Fig. 1), immediately east of the prominent erosional escarpment identified as a possible lake shoreline (“L1” of Langford, Reference Langford2003; Fig. 4). This setting is described by Szynkiewicz et al. (Reference Szynkiewicz, Ewing, Moore, Glamoclija, Bustos and Pratt2010, pp. 79–80) as “an 18 km long and 1.2 to 2.2 km wide area of remnant dune cross-strata … to the west of the upwind margin of the active dune field … This area … is overall deflationary and absent of much modern dune activity. The cross-strata found in this area are similar in morphology and size to cross-strata found in the interdunes of the active dune, suggesting they were deposited by dunes of similar size and shape as in the modern field.” The remnant dune sediments could be part of a lunette, given their setting immediately adjacent to the prominent scarp on the downwind margin of Alkali Flat. The scarp may have started as a shoreline feature, but it is now clearly undergoing wind erosion that truncates LGM and younger lacustrine and alluvial sediments to the south (Bennett et al., Reference Bennett, Bustos, Pigati, Springer, Urban, Holliday and Reynolds2021). Further, gypsum-rich lunettes cut by U.S. 70 just southeast and south of the dune system (Fig. 2) are only 100–200 m wide and expose no cross bedding. As Szynkiewicz et al. (Reference Szynkiewicz, Ewing, Moore, Glamoclija, Bustos and Pratt2010) indicate, the cross-bedded gypsum deposits in and around the area of the archaeological site are identical to those comprising the main active dune body and are considered to be a remnant of it.

Figure 4. LIDAR-based topographic map of the area of the Gypsum Overlook site showing the locations of the archaeological features. Features 4 and 8 are housepits. The others are hearths or other activity areas. Features 4, 5, and 6 provided the radiocarbon dates. The escarpment at lower left (southwest) is the prominent erosional escarpment that defines the eastern margin of Alkali Flat. The long ridge immediately northeast of the site is a recent gypsum dune. The small hummocks that dot the area in and around the site are cross-bedded remnants of the Early Holocene gypsum dunes (shown in Fig. 5A). Map prepared by Joel Butler, Westwood Professional Services, Inc.
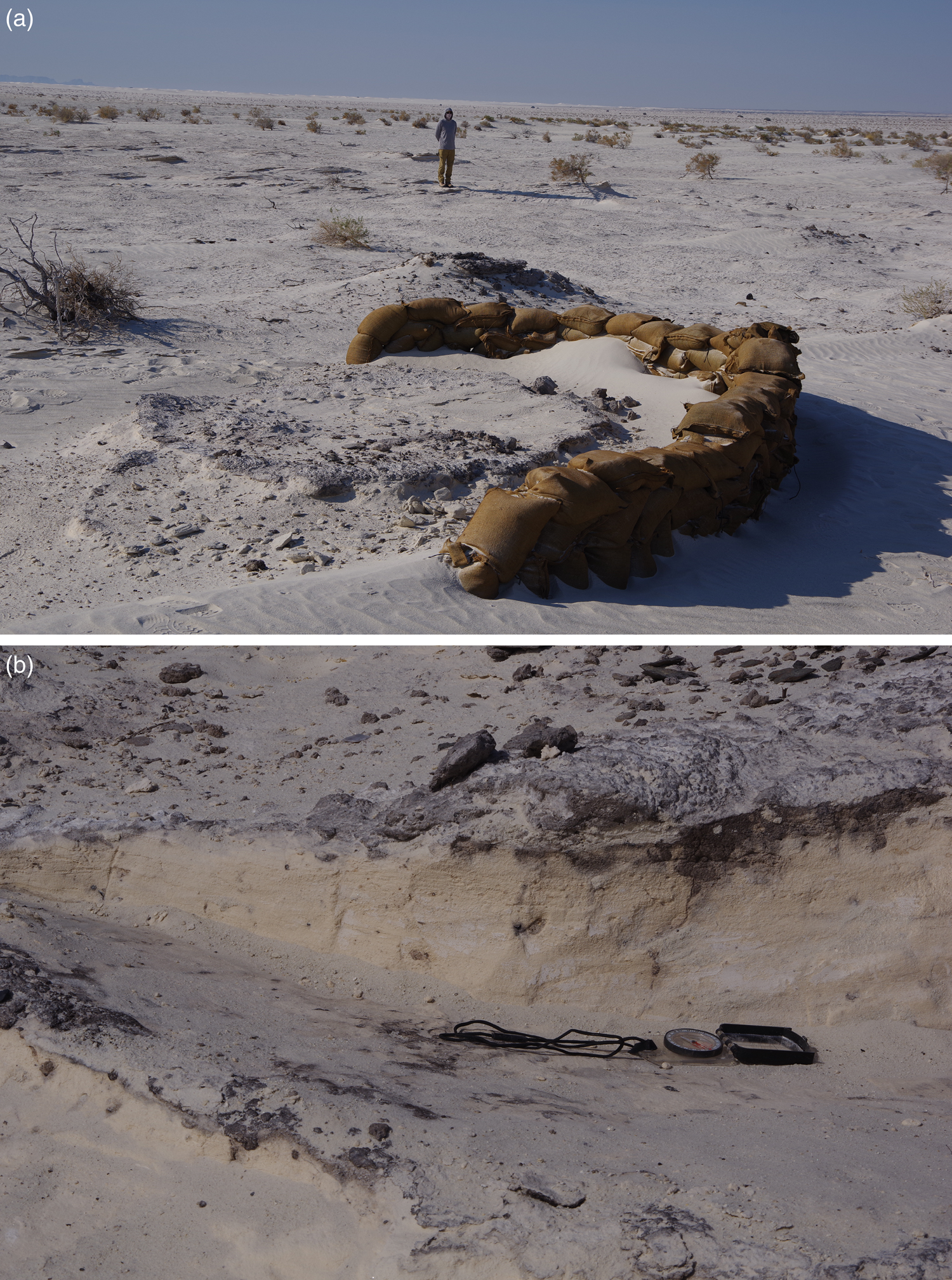
Figure 5. The Gypsum Overlook site exposed in Early Holocene gypsum dunes. (A) The exposed housepit (Feature 4) with Feature 6 hearth (Fig. 6) immediately beyond the sandbags (used as a protective windbreak). Truncated remnants of cross-bedded gypsum dunes (the small hummocks in Fig. 4) are visible where the figure is standing. The open, flat surface between remnants of eroded, cross-bedded sand is immediately underlain by post–last glacial maximum lakebeds. The resistant cap across the surface of the housepit is Feature 4a. Gypsum sand is visible below both Features 4a (B) and 6 (Fig. 6). The resistant layer below and to the left of the sand and 4a is Feature 4b, the lower component of the housepit in B. The Alkali Flat deflation basin can be seen in the distance with the White Sands dune field on the skyline. (B) Gypsum sand below the upper occupation layer (Feature 4a, indurated by burning and dated by radiocarbon samples Beta-61297 and Beta-611249; Table 1) rests on the lower floor (Feature 4b, likewise indurated, dated by radiocarbon samples Beta-612928 and Beta-611250; Table 1). The sand exhibits faint cross bedding, best expressed at left. Thin sections from this sand exhibit evidence for mixing, likely due to human trampling.
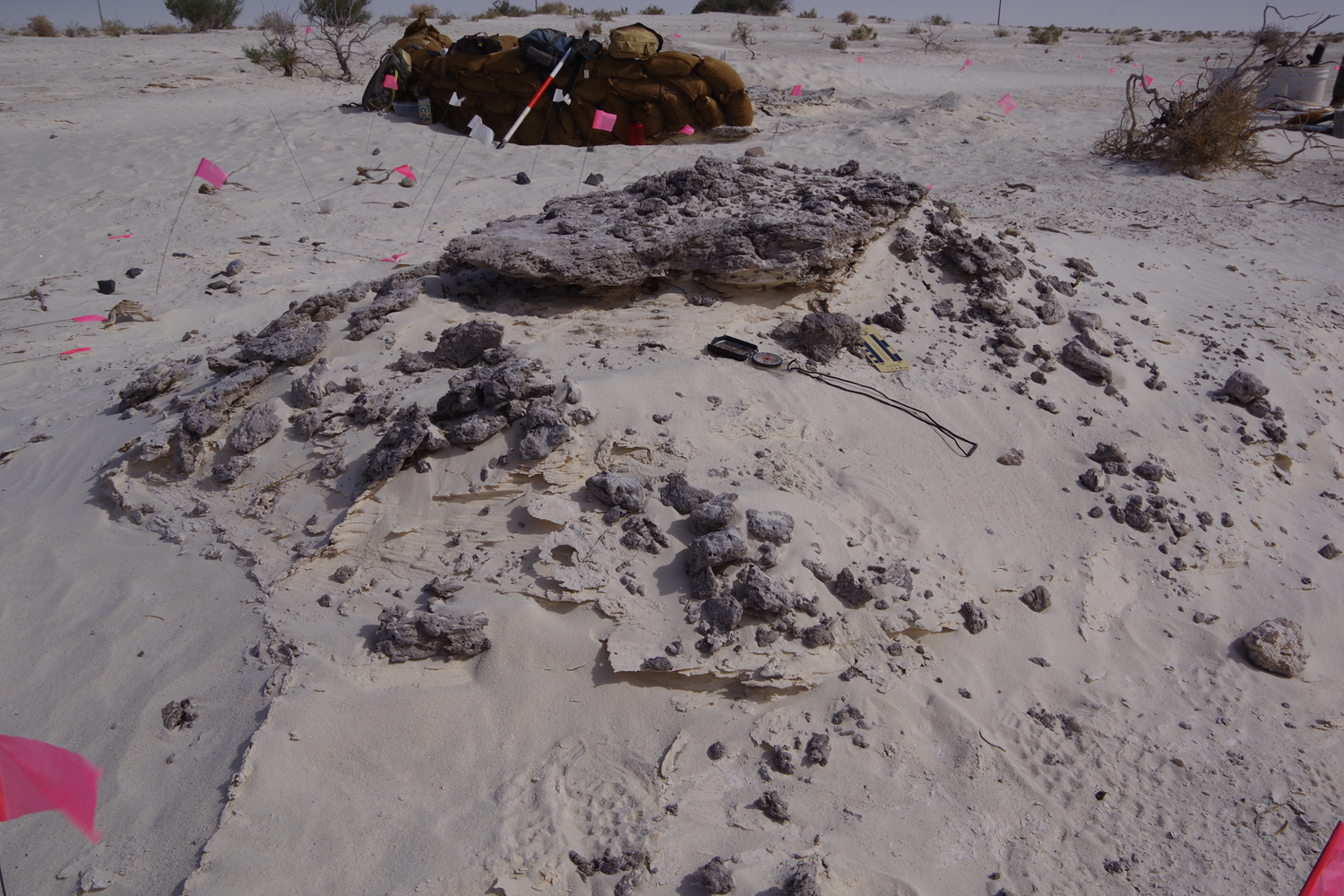
Figure 6. Feature 6 hearth (dated by radiocarbon sample Beta-612926; Table 1) underlain by cross-bedded sand. The indurated character of the baked gypsum (turned to dense anhydrite by heat from cooking) is apparent. Feature 4 and sandbags are visible in the background.
Table 1. Accelerator mass spectrometry (AMS) 14C analysis (Beta Analytic, Inc.) of charred plant material from archaeological features at the Gypsum Overlook site, White Sands Missile Range, NM.

a Measured separately by isotope-ratio mass spectrometry (IRMS).
b Conventional (uncalibrated) AMS 14C ages, corrected for isotopic fractionation, reported in 14C years before present (yr BP; 0 yr BP = AD 1950) at the 1σ (68%) confidence level.
c Rounded to the nearest 10 yr per the conventions of the 1977 International Radiocarbon Conference.
d Calibrated using the Northern Hemisphere atmospheric calibration curve (IntCal20; Reimer et al., Reference Reimer, Austin, Bard, Bayliss, Blackwell, Ramsey and Butzin2020) implemented in OxCal v. 4.4.4 (Bronk Ramsey, Reference Bronk Ramsey2009, Reference Bronk Ramsey2021) and reported in calibrated (calendar) years before present (cal yr BP; 0 yr BP = AD 1950).
The archaeological site consists of eroded remnants of the occupation floors and adjacent hearths in two areas (Fig. 4). The larger and more complete floor (Feature 4) appears to be from a housepit (Fig. 5A). It contains abundant plant material and stone artifacts. Scattered around the housepit are remnants of five cooking hearths. The floor and hearths were cemented in place by burning the underlying gypsum sediments, which created anhydrite, a process described by Worman et al. (Reference Worman, Kurota and Hogan2019) and Harvey (Reference Harvey2013) for Archaic and experimental hearths elsewhere in the dune system. Within and below the Feature 4 house floor and adjacent hearths, erosion exposed cross-bedded gypsum sand identical to cross-bedded gypsum in the surrounding area (Figs. 5A and B, and 6). All these archaeological features are exposed by wind erosion that left them as positive relief owing to their more resistant character (Figs. 4 and 5A).
Six radiocarbon dates were determined on charred plant material from the Gypsum Overlook site. Two samples are from an upper occupation level (4a) in the Feature 4 housepit, two are from a lower occupation level (4b), and two are from remnant hearths (Features 5 and 6) (Figs. 5B and 6, Table 1). The dates are statistically identical and average ~8770 cal yr BP. The presence of cross-bedded sand beneath the hearths, the housepit, and separating occupation levels within the housepit indicate that dune construction was underway during and immediately before that date.
DISCUSSION AND CONCLUSIONS
The archaeological data from gypsum dunes in the White Sands area document two unrecognized phases of dune construction older than the previous age estimate of ~8000 to 6500 cal yr BP for the main sand body. The oldest phase was construction of parabolic dunes south of the currently active White Sands dune system. An archaeological site draped over the dune contains Paleoindian artifacts that overlap in age from ~12.5 to ~12.0 cal ka BP. The dune must therefore date to >12.2 cal ka BP. The next dated phase of dune construction was in the main sand body. A deflated remnant of that sand body exposed an Early Archaic archaeological site with cooking hearths and house floors. The site was on and buried by the now-deflated gypsum dunes. Six radiocarbon dates on charcoal from the house floor and hearths yielded statistically identical dates averaging ~8770 cal yr BP.
Eolian deflation and dune construction is linked to lake level history. Baitis et al. (Reference Baitis, Kocurek, Smith, Mohrig, Ewing and Peyret2014) propose a model for evolution of the main dune body at White Sands, progressing toward the basin (i.e., prograding east to west) following the fall of the paleolake. Older eolian deposits reflecting that proposed sequence may exist beneath the modern dune field, but the scattered data points presented here and by Worman et al. (Reference Worman, Kurota and Hogan2019) show that the surface expression of dunes and eolian deposition is younger to the east. Rachal et al. (Reference Rachal, Zeigler, Dello-Russo and Solfisburg2021; based largely on dating in Allen et al. [Reference Allen, Love and Myers2009]; Bustos et al. [Reference Bustos, Jakeway, Urban, Holliday, Fenerty, Raichlen and Budka2018]; Holliday et al. [Reference Holliday, Harvey, Cuba and Weber2019]; Rachal et al. [Reference Rachal, Dello-Russo and Kurota2020]) propose that the ancient lake began to recede, and lake-bed deflation began ~18–11.5 cal ka BP. The LA52362 parabolic dune fits that interval.
The older set of parabolic dunes is south of the active White Sands dune field and downwind of a narrow, shallower arm of paleo-Lake Otero that may not have been directly linked hydrologically to the main lake system, however. The parabolic dunes are nevertheless indicative of deflation of gypsiferous lake sediments. Rachal et al. (Reference Rachal, Zeigler, Dello-Russo and Solfisburg2021) further propose a shallow perennial lake ~11.5–8.3 cal ka BP followed by regional aridity and deflation. The dating at Gypsum Overlook (also fig. S1 in Bustos et al., Reference Bustos, Jakeway, Urban, Holliday, Fenerty, Raichlen and Budka2018) suggests that regional eolian deposition and construction of the main dune body, and therefore regional deflation, began earlier than ~8.3 cal ka BP. The OSL date of ~7.3 ka on lake beds beneath the main body (Kocurek et al., Reference Kocurek, Carr, Ewing, Havholm, Nagar and Singhvi2007) could be estimating the age of burial of the lacustrine sediments and therefore expansion of the dune field east of that location by the Middle Holocene.
Stratigraphic and paleoenvironmental data from across the greater Southwest beyond the northern Tularosa Basin provide conflicting information on the regional significance and drivers of the two newly recognized phases of deflation and dune construction. Siliciclastic sand-sheet sediments in the southern Tularosa Basin yielded OSL ages that overlap with both newly established phases of gypsum dune construction (Hall et al., Reference Hall, Miller and Goble2010), but that dating is the subject of some debate (Hall et al., Reference Hall, Miller and Goble2012; Monger et al., Reference Monger, Buck, Hawley and Rachal2012). Similarly, the Mescalero sand sheet, east of the Pecos River in southeastern New Mexico, includes a phase of deposition OSL dated ~18 ka to 5 ka (Hall and Goble, Reference Hall and Goble2023). In the Strauss sand sheet in southern New Mexico and Chihuahua, west of the Rio Grande, Hall and Goble (Reference Hall and Goble2015) document eolian deposition OSL dated >16.0 ka and <11.0 ka. The onset of the later phase of deposition is constrained by a single OSL date with low precision (9.91 ± 0.42 ka).
The Estancia Basin, 200 km NNE of the Tularosa Basin and similarly situated on the east flank of the Rio Grande Rift, has the only comparable record of gypsiferous paleolake deposits and deflation creating gypsum dunes (Allen and Anderson, Reference Allen and Anderson2000; Anderson et al., Reference Anderson, Allen and Menking2002). An age model suggests desiccation beginning ~13.9 to ~13.4 cal ka BP (12,000 and 11,500 14C yr BP) and ending by ~11.0 cal ka BP (9650 14C yr BP). This age range encompasses the age estimate for the parabolic dune at LA52362. A subsequent phase of dune construction began by ~7.8 cal ka BP in the Estancia Basin. This coincides with the age of the main dune body at White Sands, but the new data from Gypsum Overlook clearly show that the main body of the dunes was present at least 1000 yr earlier. West of paleo-Lake Estancia, in the Albuquerque Basin, siliciclastic eolian deposits produced OSL ages ranging from ~15.5 to ~11.7 ka (Hall et al., Reference Hall, Goble and Raymond2008), time correlates of the older parabolic dune at White Sands. Approximately 1600 km southeast of the White Sands, in the Basin and Range region but west of the Rio Grande, is the Cuatro Cienegas Basin. It is well known for extensive outcrops of gypsum, including dunes. The eolian deposits are not dated but are considered to be of Holocene age (Czaja et al., Reference Czaja, Estrada-Rodríguez, Olvera, Khan, Böer, Öztürk, Abdessalaam, Clüsener-Godt and Gul2014).
Paleoenvironmental data from packrat middens, pollen, and isotopes provide clues to climate conditions that may have driven deflation of paleo-Lake Otero. Stable isotopes from soils in stratified alluvium 170 km north of White Sands suggest that ca. <11.0 cal ka BP “the climate gradually shifted to less cool and less wet conditions … reaching modern levels by about 9000 cal yr BP” (Hall and Penner, Reference Hall and Penner2013, p. 278). Mean annual temperatures rose and mean annual precipitation declined. Drying beginning ~15.0 cal ka BP and continuing into the Younger Dryas chronozone (YDC) is documented in the mountains and basins of southwest New Mexico and southeast Arizona (Mehringer and Haynes, Reference Mehringer and Haynes1965; Holmgren et al., Reference Holmgren, Peñalba, Rylander and Betancourt2003, Reference Holmgren, Betancourt and Rylander2006). Similar trends in drying during the final millennia of the late Pleistocene (before and through the YDC) are seen in a variety of paleo-vegetation records across northern Sonora and Chihuahua (Devender, Reference Van Devender, Betancourt, Devender and Martin1990a, Reference Van Devender, Betancourt, Devender and Martin1990b).
Secular variation in speleothem δ18O from caves in the New Mexico portion of the Guadalupe Mountains documents changes in the isotopic composition of precipitation in southeastern New Mexico over the last 12,000 yr (Polyak et al., Reference Polyak, Rasmussen and Asmerom2004; Asmerom et al., Reference Asmerom, Polyak, Burns and Rassmussen2007; Fig. 7). Fluctuating trends in δ18O mark periods of both enhanced moisture delivery (either from the North American Monsoon or above-average winter precipitation) and drying coinciding with the YDC (12.9 to 11.5 ka) and the earliest Holocene (11.5 to ~10 ka). High δ18O values and limited speleothem growth during the Early to Middle Holocene (~10–7 ka) suggest prolonged regional aridity.

Figure 7. Median calibrated accelerator mass spectrometry (AMS) 14C ages (filled circles; 0 yr BP = AD 1950) and 2σ age range (yellow-brown area) of archaeological features at the Gypsum Overlook site and variation in precipitation amount inferred from the stable oxygen isotope (δ18O) time series (black line; 0 yr BP = AD 2003) of Pink Panther Cave stalagmite PP1 (after Asmerom et al., Reference Asmerom, Polyak, Burns and Rassmussen2007). Intervals of decreased (increased) moisture inferred from positive (negative) excursions in δ18O. The age of archaeological features (~8770 cal yr BP) and associated eolian deposits coincide with the driest interval of the Holocene (shaded area; ~10–7 cal ka BP) (Asmerom et al., Reference Asmerom, Polyak, Burns and Rassmussen2007).
The regional paleoenvironmental records document a phase of widespread post-LGM aridity and dune construction in the greater Southwest during the latest Pleistocene, but the dating is not necessarily synchronous. The parabolic dune at LA52362 has a minimum limiting age of >12.2 cal ka BP based on the artifact assemblage on top of it. The regional significance of deflation of the narrow southern arm of paleo-Lake Otero is unclear, however. It is shallower than the main basin and may have dried and deflated during the initial phases of aridity before the main waterbody disappeared. No stratigraphic or geomorphic record of concomitant dune construction is apparent downwind of the broader basin, but it could have been destroyed by subsequent deflation or retreat of the eastern escarpment or is buried beneath the main dune body. The absence of Holocene dunes in the area with the older parabolic dunes is also enigmatic. The supply of gypsum in the narrow, shallow southern arm of the ancient lake may have been minimal and quickly exhausted.
Well-dated evidence for Early Holocene eolian deposition in the Southwest is rare. The six consistent radiocarbon ages on charcoal from the Gypsum Overlook site buried within gypsum dune deposits clearly establish a phase of dune construction ~8.8 cal ka BP. The absence of a clear record of correlative eolian deposition at this time may suggest a relatively minor phase of aridity and deflation. Unlike the stabilized siliciclastic sand bodies in the region, broad gypsum flats are relatively sparsely vegetated. They may be more sensitive to aridification.
The precise drivers resulting in deflation are not clear, however. The paleolake must have declined, if not disappeared, in the final millennia of the Pleistocene. Vegetation may have been sparse as well, exacerbating erodibility. Today, a moist surface inhibits wind erosion, but dry conditions promote it. A declining water table and dry conditions would contribute to erosion. Gypsiferous sediments also create limitations in plant micronutrients (e.g., N, P, K) and can form dense crusts that inhibit development of seedlings (Ochoterena et al., Reference Ochoterena, Flores-Olvera, Gómez-Hinostrosa, Moore, Mandujano, Pisanty and Eguiarte2020). Further, once deflation began, fresh gypsum would be continuously exposed. Today, large areas of the Alkali Flat have little vegetational cover and wind erosion persists.
Variable local and regional environmental conditions may have created contrasting dune morphologies as well. Parabolic dunes are created by persistent winds with limited sediment supply on a vegetated surface (McKee, 1979, pp. 94–95; Kocurek and Ewing, Reference Kocurek, Ewing, Budd, Hajek and Purkis2016). Upwind of the southern region of the parabolic dunes, the narrow southern arm of paleo-Lake Otero would limit sediment supply today and in the past. Langford et al. (Reference Langford, Rose and White2009), following a proposal by Fryberger (Reference Fryberger2003), also show that parabolic dunes may form where fresh water is just below the surface. Water with lower salinity allows vegetation to grow and stabilize the sand, except for the active noses of the parabolic dunes. Before the onset of warmer and drier Holocene conditions, fresh water may have persisted below the surface. Environmental changes in the Early Holocene drove deflation and may have resulted in shallower saline ground water, resulting in a persistent unvegetated surface and construction of the modern dune system dominated by crescentic ridges and barchan dunes. Regional aridity initiated at least a limited terminal Pleistocene phase of deflation and dune construction, while extensive exposure of gypsum along with high-salinity ground water contributed to the Early Holocene deflation and initiation of the main dune field.
Acknowledgments
Fieldwork was supported by the Argonaut Archaeological Research Fund (V.T.H., director, University of Arizona Foundation) and White Sands Missile Range. Jim Bowman, cultural resource manager, White Sands Missile Range, facilitated field logistics and radiocarbon dating. Additional logistical support was provided by White Sands National Park. David Meltzer and his crew from Southern Methodist University shared mapping data from Gypsum Overlook (supported by the Quest Archaeological Research Fund; D. Meltzer, director). D. Shane Miller, Andy Richard, and Allison Harvey helped with coring and mapping at LA52362. The cartographic skills for preparation of Figures 1 and 4 were kindly supplied by Paul Neville and Joel Butler. Jim Abbott prepared Figures 2 and 3. Nick Lancaster, and Xiaoping Yang with Quaternary Research, Ryan Ewing, and an anonymous reviewer provided useful commentary that improved the paper. Gary Kocurek provided valuable advice, insights, and the mapping in Figure 3.