Growth assessment in the first 1000 d of life is a shared interest of all health professionals involved in the care of pregnant women, infants and children. Primary care providers, nurses, midwives, obstetricians, paediatricians and dietitians/nutrition professionals all share a common goal to support the foetus/infant to achieve its genetic potential for growth and development(Reference Lees, Romero and Stampalija1,Reference Gardosi, Francis and Turner2) .
In the context of growth assessment, growth refers to changes (increases/decreases) in measurable physical properties (e.g. weight, lengths and circumferences) over time(Reference Lampl, Mummert and Schoen3). The multi-dimensional, non-linear nature of growth necessitates sequential measurements – usually of more than one physical property – to accurately assess changes in body size, proportion and composition(Reference Lees, Romero and Stampalija1,Reference Lampl, Mummert and Schoen3,Reference Fenton, Dai and Lalari4) . Once-off anthropometric assessment has only limited value, as any given size may be the result of consistent growth, growth faltering or excessive growth, each of which implies different health and nutritional conditions and requires different clinical management(Reference Fenton, Dai and Lalari4).
Within a life-course approach, anthropometric assessment serves a purpose beyond the evaluation of current health and nutritional status; it provides summative information about past growth and predicts likely future health outcomes(Reference Russ, Larson and Tullis5,Reference Ben-Shlomo and Kuh6) . The first 1000 d of life (from conception to the second birthday) are particularly important as a critical window of opportunity for health promotion and disease prevention, and nutritional insults during this time can have serious short-term and life-long consequences(Reference Black, Victora and Walker7,Reference Langley-Evans8) . For example, foetal growth restriction (FGR) is an important cause of potentially avoidable stillbirth(Reference Hugh, Williams and Turner9,Reference Hirst, Villar and Victora10) , size at birth may predict neonatal mortality(Reference Kang, Wu and Shaikh11–Reference Halliday13) and future growth(Reference Campbell14) and growth in infancy (as a sensitive marker for nutritional status and overall health) may predict mortality, neurodevelopment, lifetime educational achievement and future non-communicable disease risk(Reference Black, Victora and Walker7,Reference Victora, Adair and Hallal15–Reference Wells, Sawaya and Wibaek17) . Low- and middle-income countries (LMIC) remain burdened by early childhood malnutrition, and growth monitoring and promotion are a cornerstone of primary health care for children(Reference Cash, Keusch and Lamstein18–Reference De Onis, Onyango and Borghi20). Additionally, many LMIC are experiencing a nutrition transition, with widespread chronic undernutrition complicated by increasing obesity prevalence, the so-called double burden of malnutrition. This emphasises the need for appropriate growth monitoring and timely intervention where needed(Reference Black, Victora and Walker7,Reference Wells, Sawaya and Wibaek17) .
Birth is a key event in the first 1000 d of life. In physiological terms, birth represents an interruption in the growth continuum, with a temporary cessation of growth (including weight loss) as the neonate adjusts to extrauterine life. For the health care team, the perinatal period is the point of overlap between the prenatal (obstetrics, midwifery and primary antenatal care) and postnatal (paediatric, child health and primary health care) health care providers (Fig. 1). Clear communication between all these professions is essential for maintaining continuity of care and ensuring optimal outcomes for mothers, infants and young children. This necessitates that we ‘speak the same language’ across disciplines, while also staying abreast of the latest developments in the field of growth assessment. The same is true in research so that findings may be compared across studies and over time. As studies in life-course nutrition show that the consequences of poor growth may only be evident in the distant future; it is important, therefore, that at least the basic measurements lend themselves to such longitudinal analyses.
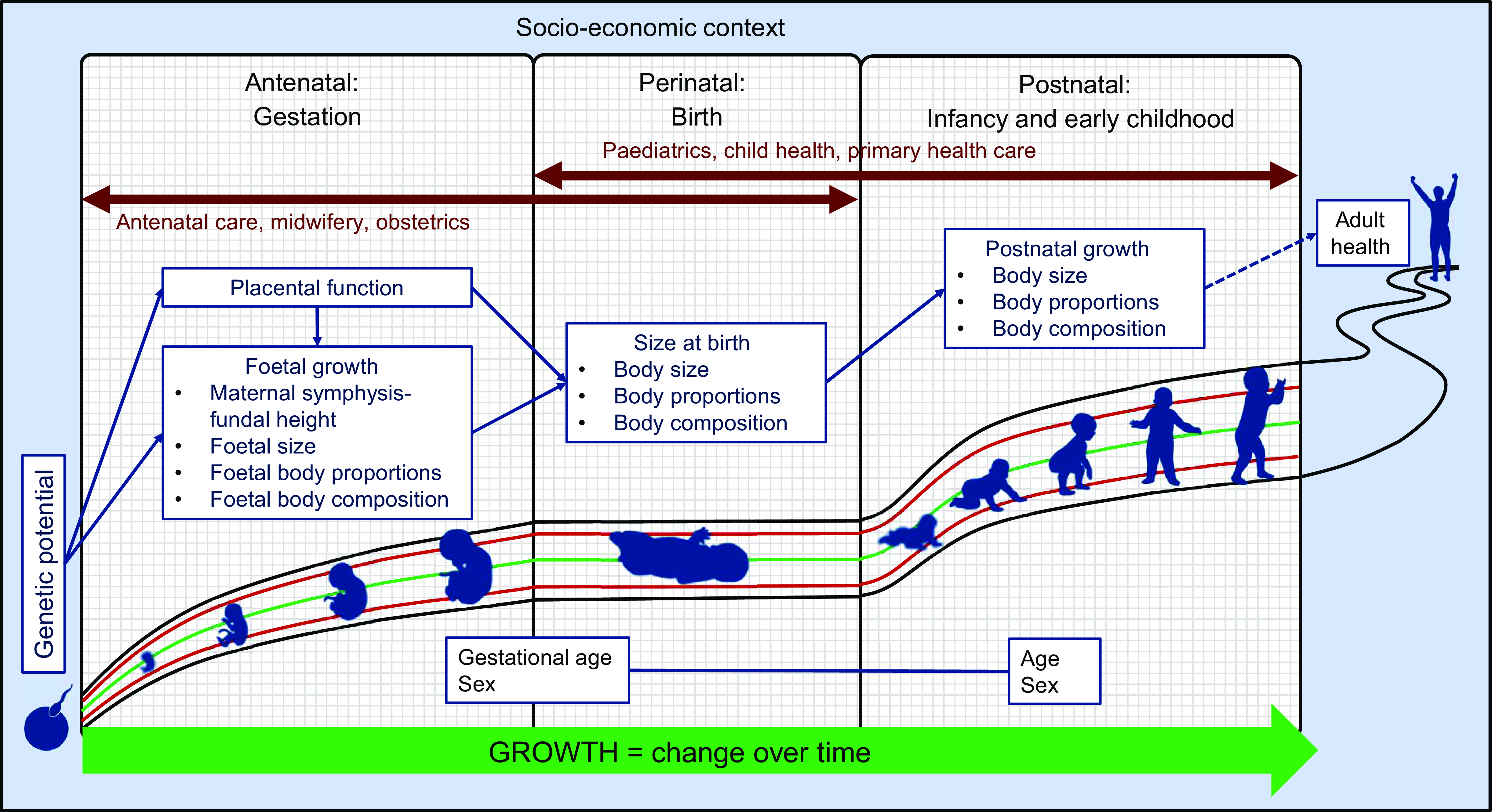
Fig. 1 Growth assessment in the first 1000 d of life: continuity over time and across disciplines
Various factors complicate the interdisciplinary harmonisation of growth assessment in the first 1000 d. Primary among these are the fundamental differences in the established measurements, indices and indicators used by different clinical disciplines. For instance, foetal growth assessment relies on indirect measurements – maternal symphysis-fundal height, ultrasound biometric measurements and calculated estimated foetal weight – as opposed to direct measurement after birth. Discipline-specific measurements may further exacerbate this disconnect: for example, abdominal circumference is a useful measurement in the foetus but is of limited value postnatally; proportionality between weight and head circumference (HC) is of interest at birth but rarely thereafter; and nutritional assessment in infancy and childhood relies heavily on weight-for-length (WFL) or BMI which is never assessed prenatally and only rarely at birth(Reference Victora, Villar and Barros21). This complicates communication between different disciplines. A final challenge is that policy frameworks, while encouraging interdisciplinary cooperation in principle, rarely provide any practical framework for communication across disciplines serving mother–infant dyads during different life stages(22). Rather, they often perpetuate the division of prenatal, perinatal, maternal and infant healthcare by separating them at both the policy and the practical level(23–25).
Addressing the communication gap between healthcare providers will require, firstly, an awareness of the overlap and discrepancies between clinical disciplines as well as an understanding of the ways in which growth is assessed by these disciplines. Clear, open communication in a common language is needed for the health care team to holistically understand the growth of the individual in their care, both in the past and in the future. Finally, the policy environment and healthcare systems should encourage and facilitate interdisciplinary communication and teamwork.
Aim
This paper aims to contribute to evidence-based, integrated implementation of growth assessment and to contribute to the continuity of care in the first 1000 d, by the development of a unified conceptual framework that integrates measurements, indices and indicators of foetal/infant growth assessment from the time of conception until 2 years of age, with an emphasis on application in LMIC. The intention is to promote continuity of transdisciplinary health care provision, based on current scientific understanding and practice.
Key definitions
For the sake of clarity, a distinction was made between measurements, indices and indicators (Table 1). Measurements refer to the quantification of a physical parameter. Indices combine a measurement with other measurement(s) and/or characteristic(s) such as age, gestational age (GA) and sex, in biologically meaningful ways. Indicators place indices in relation to what is expected in healthy individuals, allowing clinically useful conclusions to be drawn(Reference Waterlow26).
Table 1 Differentiation between measurement, index and indicator in growth assessment (based on and adapted from Waterlow, 1992:213)(Reference Waterlow26)

This paper is intended to contribute to implementation science, which may be defined as ‘an interdisciplinary body of theory, knowledge, frameworks, tools and approaches whose purpose is to strengthen implementation quality and impact’(Reference Tumilowicz, Ruel and Pelto27). The topics under discussion are relevant to all professionals who assess foetal and infant growth, whether in clinical practice or for research purposes, as well as to policies governing maternal, neonatal, infant and young child healthcare provision.
Methods
Using a multidisciplinary interactive think-tank approach over many months of regular meetings, a workgroup of researchers and clinicians with specialisation in obstetrics (n 4), paediatrics (n 2) and dietetics/nutrition (n 4) in consultation with a statistician, shared and debated their practice and research experience related to foetal/infant growth assessment. Weekly virtual meetings of academic staff, researchers and postgraduate students affiliated with the Research Centre were initiated in February 2021, in order to maintain contact among geographically remote affiliates, support postgraduate students and foster interdisciplinary thinking and collaboration among researchers from diverse academic backgrounds. This was considered essential in light of the transdisciplinary nature of the Research Centre’s activities. It soon became apparent that different clinical specialties within the team were approaching matters relating to foetal and infant growth from widely differing perspectives, which complicated communication among the group. Establishing a common understanding and language for matters related to growth monitoring was included as a fixed agenda item, and from these discussions, the framework presented here emerged and was formalised and refined. The discussions focussed particularly on identifying parameters that contributed meaningfully to continuous growth assessment by different clinical disciplines over the first thousand days of life. The information was grouped according to antenatal, perinatal and postnatal periods, the domains of the three clinical disciplines represented in the think-tank. The measurements, indices and indicators within each period were identified by the subject specialists in the team, based on clinical practice and evidence-based guidelines, and reviewed by all members of the team. Disagreements were resolved by discussion and referral to published research and clinical guidelines.
The information was further organised using the terminology of measurements, indices and indicators introduced by Waterlow, one of the fathers of child growth monitoring (Table 1)(Reference Waterlow26). As the process advanced, recurring sub-themes emerged within the three assessment periods, namely body size and proportions, body composition and placenta-related matters (in the antenatal and perinatal periods). These were incorporated into the basic framework. The personal practice and research knowledge of the participants was supplemented and verified by published information, and evaluated for clinical applicability, particularly in resource-limited settings.
Results
Figure 1 shows the basic conceptual framework, incorporating the somatic aspects of growth assessment within each of the three distinct assessment periods in the first 1000 d.
The genetic growth potential of the foetus/infant represents the starting point of the framework: conceptually, healthy growth is growth that achieves the genetic potential without overshooting it. However, ‘genetic potential’ is operationally difficult, as it is impossible to quantify. Recently, researchers have begun to incorporate proxies for genetic factors (e.g. ethnicity and maternal height) to develop individualised foetal growth curves and birthweight targets(Reference Gardosi, Francis and Turner2). However, in practice, foetal and infant growth assessment relies on comparing the size/growth of the individual foetus/infant to the expected growth pattern described by reference growth charts(Reference Lees, Romero and Stampalija1,Reference Fenton, Dai and Lalari4) .
Although this framework is concerned mainly with the objective anthropometric assessment of growth, the crucial role of socio-economic factors cannot be ignored. Thus, the socio-economic context is represented as a background that encloses and underlies the framework.
Placental growth and function are included in the antenatal and perinatal phases, firstly because the placenta is derived from foetal tissue and grows in tandem with the foetus, and secondly, because of its critical role in foetal nutrition and growth. Throughout the antenatal and postnatal periods, the emphasis is on change over time, dynamically depicted by an arrow. Conversely, birth is shown as an interruption of the growth continuum, with a temporary flattening of growth curves. Anthropometric assessment at birth is commonly used as a proxy for intrauterine growth, but it provides only limited information, as an understanding of foetal growth is needed to appreciate the value and limitations of birth anthropometry.
The following sections detail the available measurements, indices and indicators in each period, followed by an integrated framework illustrating the relationship between selected growth parameters throughout the first 1000 d.
Antenatal period: foetal growth assessment
During the antenatal period, ultrasound can be used for biometric measurements of the foetus and placenta. The measurements and functional tests, along with their associated indices and indicators, are presented in Table 2.
Table 2 Measurements, indices and indicators for assessment of intra-uterine growth

*Delphi Consensus Statement for Foetal Growth Restriction(Reference Gordijn, Beune and Ganzevoort43):
Early FGR (GA < 32 weeks):
- Any one of: AC < 3rd centile, EFW < 3rd centile or absent end-diastolic flow in the umbilical artery, OR
- AC or EFW < 10th centile PLUS pulsatility index > 95th centile in either the umbilical or uterine artery.
Late FGR (GA ≥ 32 weeks):
- Any one of: AC < 3rd centile or EFW < 3rd centile OR
- Any two of: EFW or AC < 10th centile, AC or EFW crossing centiles by more than two quartiles on standardised growth charts, or cerebroplacental ratio < 5th centile.
Abbreviations: RI, resistance index; PI, pulsatility index; CPR, cerebroplacental ratio; INTERGROWTH-21st, International Fetal and Newborn Growth Consortium for the 21st Century; GA, gestational age; FGR, foetal growth restriction; FL, femur length; BPD, biparietal diameter; HC, head circumference; AC, abdominal circumference; EFW, estimated foetal weight; NICHD, National Institute of Child Health and Human Development; ISUOG, International Society of Ultrasound in Obstetrics and Gynecology.
Foetal measurements are assessed independently of foetal sex, but according to GA. The first important requirement to assess foetal growth, then, is an accurate GA estimate. In the absence of a certain date of conception (or last menstrual period), GA is estimated based on ultrasonographic measurements. However, this approach is less reliable after 14 weeks GA, since foetal growth becomes more variable with advancing GA(Reference Salomon, Alfirevic and Da Silva Costa28). Where ultrasound is unavailable, symphysis-fundal height measurement may be used for GA estimation, although the accuracy is inferior to ultrasound and may be challenging in women with obesity(Reference Wanyonyi and Mutiso29–Reference Geerts, Poggenpoel and Theron31).
Foetal abdominal circumference (AC), HC, femur length and biparietal diameter are commonly measured. Each of these can be assessed according to GA-specific reference charts (Table 2)(32–Reference Kiserud, Benachi and Hecher36), with values between the 10th and 90th percentile considered appropriate for gestational age(Reference Salomon, Alfirevic and Da Silva Costa28). The same holds true for estimated foetal weight, which is calculated using the aforementioned biometric measurements. Various estimated foetal weight equations (i.e. indices) are available, including the widely used Hadlock equations(Reference Hadlock, Harrist and Sharman37) and the newer equation from the International Fetal and Newborn Growth Consortium for the 21st Century (INTERGROWTH-21st)(Reference Stirnemann, Villar and Salomon34). However, 10–15 % errors in estimated foetal weight v. actual weight are not uncommon and may be attributed to high inter- and intra-observer variability in biometric measurements, the choice of equation and the amplification of errors in single parameters when included in a calculation(Reference Salomon, Alfirevic and Da Silva Costa28,Reference Mayer and Joseph38–Reference Plonka, Bociaga and Radon-Pokracka42) .
The aim of foetal growth assessment is the detection of FGR or excessive foetal growth. Numerous diagnostic criteria (i.e. indicators) for FGR have been proposed, but the Delphi Consensus Statement for Foetal Growth Restriction (Table 2)(Reference Gordijn, Beune and Ganzevoort43) is the most widely accepted. Other potentially useful indicators include biometric measurements and/or estimated foetal weight < 10th or >90th percentile for GA (indicating small-for-GA and large-for-GA, respectively)(Reference Salomon, Alfirevic and Da Silva Costa28), as well as foetal proportions and ratios (most commonly the HC/abdominal circumference ratio) as indicators of asymmetric FGR(Reference Hiersch and Melamed44).
Comprehensive growth assessment implies multiple measurements over time(Reference Lees, Romero and Stampalija1,Reference Lampl, Mummert and Schoen3) , but the appropriate interpretation of serial foetal biometry remains unclear(Reference Hiersch and Melamed44). Various approaches have been proposed, including calculation of growth velocity, conditional percentiles, projection of expected birth weight and individualised growth charts(Reference Gardosi, Francis and Turner2,Reference Hiersch and Melamed44) ; however, none of these have been sufficiently validated for widespread incorporation into clinical practice. A minimum interval of three weeks between ultrasound assessments is recommended to minimise over-detection of foetal growth problems(Reference Salomon, Alfirevic and Da Silva Costa28).
In LMIC, ultrasound assessment is mostly limited to high-risk pregnancies. In these settings, maternal symphysis-fundal height measurement is commonly used to monitor foetal growth(Reference Wanyonyi and Mutiso29). However, the sensitivity of a single symphysis-fundal height measurement for detecting small-for-GA/large-for-GA is poor except at the extremes of foetal size(45,Reference Robert Peter, Ho and Valliapan46) . Nonetheless, repeated symphysis-fundal height measurements (at least 2 weeks apart), plotted on an appropriate growth chart (e.g. the INTERGROWTH-21st standard(Reference Papageorghiou, Ohuma and Gravett47)) may be used as a first-level screening tool to identify women who require referral for ultrasound(45,Reference Papageorghiou, Ohuma and Gravett47) .
Measures of placental function by Doppler screening may be useful to detect foetuses at risk of FGR. Doppler devices measure blood flow velocity in maternal or feto-placental blood vessels, including the umbilical, uterine and foetal mid-cerebral arteries. Various indices can be calculated (including the resistance index, pulsatility index, systolic/diastolic ratio and cerebro-placental ratio) and compared to GA-specific reference data (see Table 2), with increasing indices indicating placental dysfunction. Crucially, the low-cost, portable, easy-to-use Umbiflow™ Doppler device makes Doppler screening feasible in resource-limited settings and has value as a once-off assessment of placental function(Reference Vannevel, Vogel and Pattinson48). Optimal indicators and cut-offs for Doppler-derived indices (particularly in the absence of biometric measurements) require further investigation, although some Doppler-derived indicators are included in the Delphi Consensus Statement for FGR(Reference Gordijn, Beune and Ganzevoort43).
Foetal body composition is not routinely assessed. Numerous publications describe visceral and subcutaneous fat thickness in various locations and its association with maternal diabetes mellitus, but no standards are available(Reference Aydin and Fatihoglu49–Reference Lam, Mein and Benzie51). Likewise, measurements of foetal organ size may be used for disease detection, but do not form part of routine foetal growth monitoring(Reference Mayer and Joseph38).
Perinatal period: newborn size and body composition
For the majority of infants, birth marks the first growth assessment, as direct measurements of body size become possible. The infant and placental measurements that can be taken at birth, as well as their associated indices and indicators, are presented in Table 3.
Table 3 Measurements, indices and indicators that can be assessed at birth

Abbreviations: BW, birth weight; PW, placental weight; GA, gestational age; HC, head circumference; INTERGROWTH-21st, International Fetal and Newborn Growth Consortium for the 21st Century; SGA, small for gestational age; AGA, appropriate for gestational age; LGA, large for gestational age; W, weight; L, length; HCZ, head circumference-for-GA z-score; BWZ, birth weight-for-GA z-score; PI, ponderal index FM, fat mass; FFM, fat-free mass; FMI, fat mass index; FFMI, fat-free mass index.
Meaningful interpretation of newborn size relies on sex- and GA-specific reference data (e.g the INTERGROWTH-21st Newborn Size Standards(Reference Villar, Cheikh Ismail and Victora52) and the Fenton 2013 growth chart(Reference Fenton and Kim53)). The 10th and 90th percentiles of birth weight-for-GA are commonly used to distinguish small, appropriate and large for GA infants(Reference Lees, Romero and Stampalija1). However, birth anthropometry only gives a summative snapshot of foetal growth, without any indication of the preceding foetal growth trajectory. Thus, true FGR or excessive foetal growth may be missed. For example, a neonate with birth weight < 10th percentile may simply be constitutionally small, yet growing consistently (i.e. achieving its genetic growth potential), whereas a foetus with faltering growth may remain above the 10th percentile at birth(Reference Lees, Romero and Stampalija1,Reference Damhuis, Ganzevoort and Gordijn54) . This highlights the crucial importance of communication between pre- and postnatal healthcare providers: measurements taken during pregnancy (e.g. serial ultrasound biometry or umbilical artery Doppler) can help to identify truly growth-restricted neonates who are at risk of adverse outcomes and guide paediatric healthcare providers’ expectation for appropriate postnatal growth.
In the absence of antenatal measurements, the proportionality (or symmetry) of the neonate can provide clues about foetal growth. An infant is considered proportional/symmetrical if the GA-related z-scores for weight, length and HC are similar; conversely, if the z-score for weight is markedly lower than that of length and/or HC, the neonate is considered asymmetrically growth restricted. Asymmetrical growth restriction is believed to result from cranial redistribution of foetal circulation due to placental insufficiency, maintaining brain growth at the cost of somatic growth(Reference Halliday13,Reference Sharma, Shastri and Sharma55) . Various attempts have been made to mathematically quantify the relationship between weight and length (including weight-length ratio(Reference Landmann, Reiss and Misselwitz56), BMI-for-GA(Reference Olsen, Lawson and Ferguson57) and ponderal index(Reference Halliday13,Reference Landmann, Reiss and Misselwitz56) ) or weight and HC (including BW/HC ratio(Reference Gonçalves, Lira and Eickmann58) and the difference between HC and BW z-scores(Reference Bocca-Tjeertes, Bos and Kerstjens59)), but none of the related cut-off values have been sufficiently validated for adoption into routine clinical practice. Likewise, it is not yet clear which, if any, of these indices and indicators have superior predictive value for short- and long-term adverse outcomes.
The placenta is routinely weighed at birth, but standardised procedures and consensus on indicators of abnormality are lacking. The birth weight to placental weight ratio (BW:PW; also called the foeto-placental ratio) has been shown to correlate with foetal abdominal circumference growth velocity and Doppler indices of placental function; as such, a low BW:PW ratio may suggest a history of placental insufficiency and FGR(Reference Salavati, Gordijn and Sovio60). The BW:PW ratio increases with advancing gestation, doubling from 24 to 38–40 weeks to reach a ratio of 5–7:1 at term, but a definite cut-off for abnormality remains elusive(Reference Hayward, Lean and Sibley61).
Body composition at birth can be assessed using air-displacement plethysmography (ADP, e.g. using the PEAPOD™ device), which measures body volume and calculates body density, fat mass (FM) and fat-free mass (FFM). Further indices can be calculated relative to total body weight (%FM and %FFM) or length (fat mass index and fat-free mass index – that is, FM or FFM (in kg) divided by length (in m) squared). The interpretation of these indices is linked to infant sex and GA. Various reference charts are available (see Table 3), but as yet no cut-offs have been established for body composition indicators.
Postnatal period: growth in infancy and early childhood
Routine growth monitoring has long been one of the cornerstones of primary health care provision for infants and children(Reference Cash, Keusch and Lamstein18). The various measurements, indices and indicators for assessing growth in the first 2 years after birth are shown in Table 4.
Table 4 Measurements, indices and indicators for assessment of growth in infancy and early childhood

Abbreviations: GA = gestational age; INTERGROWTH-21st, International Fetal and Newborn Growth Consortium for the 21st Century; WHO, World Health Organization; MGRS, Multicentre Growth Reference Study; WFA, weight-for-age; LFA, length-for-age; WFL, weight-for-length; MUAC, mid-upper arm circumference; UNICEF, United Nations Children’s Fund; MAM, moderate acute malnutrition; SAM, severe acute malnutrition; HC, head circumference; TSF, triceps skinfold; SSF, subscapular skinfold; FM, fat mass; FFM, fat-free mass; FMI, fat mass index; FFMI, fat-free mass index; DEXA, dual-energy X-ray absorptiometry.
Anthropometric and body composition parameters are interpreted according to the infant/child’s sex and age (with age correction for preterm infants born at < 37 weeks GA). Weight, length, HC and mid-upper arm circumference (MUAC) are commonly measured, while triceps skinfold and subscapular skinfold are technically much more challenging and much less commonly used. Any of these measurements can be interpreted as a sex-specific index-for-age (i.e. weight-for-age, length-for-age, HC-for-age, MUAC-for-age, triceps skinfold-for-age and subscapular skinfold-for-age), while weight can also be interpreted in relation to length, e.g. WFL or BMI (weight (in kg) divided by length (in m) squared), interpreted as BMI-for-age. This is particularly important in LMIC, where the high prevalence of stunting complicates the interpretation of simple weight-for-age.
Growth charts remain the cornerstone of interpreting growth indices in childhood(62). WHO Multicentre Growth Reference Study (MGRS) Growth Standards are intended as a single, global growth standard by which children of all nationalities and ethnicities can be assessed(Reference De Onis, Garza and Victora63,Reference De Onis, Garza and Onyango64) , although some countries continue to use population-specific growth charts(Reference De Onis, Onyango and Borghi20). Growth standards can be used to determine percentile positions and/or calculate z-scores or to identify the median of a given growth index. Z-score values between –2 and +2 are considered normal, although a small proportion (< 5 %) of a normally distributed population could be expected to fall outside these limits.
Different indicators and classification systems exist for the diagnosis of malnutrition, based on weight-for-age, WFL and length-for-age, and using either z-scores or the measured value as a percentage of the median (see Table 4). Additionally, MUAC is used as such to identify moderate and severe acute malnutrition in children aged 6 months to < 5 years(65). MUAC-for-age charts are available(66), but the appropriate interpretation of z-scores is not clear. The interpretation of BMI-for-age to identify overweight in young children is controversial, but the rise of obesity among children even in LMIC cannot be ignored(Reference Black, Victora and Walker7,Reference Wells, Sawaya and Wibaek17) . Skinfold measurements may be used as a rough indicator of adiposity, and reference data for triceps skinfold and subscapular skinfold are available (WHO-MGRS Growth Standards(67), but the appropriate interpretation remains unclear. Additionally, skinfolds are technically challenging to measure, and intra-observer measurement reliability tends to be poor(Reference Demerath and Fields68).
Anthropometric status (WFL z-score, MUAC) at a single time point may be used to classify moderate and severe acute malnutrition in children aged 6–59 months. Additionally, the presence of bilateral nutritional oedema and/or severe wasting is diagnostic of severe acute malnutrition regardless of anthropometric status(65). Indicators of malnutrition based on the percentage of the median of older reference data have become obsolete in light of the WHO MGRS growth standard, and their routine use is no longer recommended. These include the Waterlow classification(Reference Waterlow69) (used to distinguish between wasting (low WFL) and stunting (low length-for-age)) and the Wellcome classification(Reference Waterlow26) (used to distinguish between underweight, wasting, kwashiorkor and marasmic kwashiorkor, on the basis of weight-for-age and the presence of oedema).
Growth, as stated earlier, refers to change over time; thus, serial interpretation of anthropometric indices is imperative(62). Healthy growth is characterised by growth indices maintaining approximately constant z-scores over time, with some intra-individual variation(62). It is unclear what degree of deviation from an individual’s expected z-score trajectory should be considered problematic(62,Reference Roberfroid, Kolsteren and Hoeree70) . Nonetheless, accurately plotting of consecutive measurements on a suitable growth chart, with consideration of multiple indices, remains the best available method to identify growth faltering or excessive growth(Reference Fenton, Dai and Lalari4,62,Reference Roberfroid, Kolsteren and Hoeree70) . The WHO-MGRS Growth Standards include growth velocity standards for weight, length and HC(71) but these indices are challenging to use and interpret.
Growth assessment in preterm-born infants is less straightforward. There are several controversies, including the type of growth charts to use (charts based on longitudinally collected data, such as the INTERGROWTH-21st Postnatal Growth Standards for Preterm Infants, v. charts based on cross-sectional birth data, such as the Fenton 2013 Growth Charts), the most desirable growth trajectory (regaining the birth weight z-score, maintaining the z-score achieved after the initial weight loss, or various methods for calculating growth velocity), and the desirability of catch-up growth and speed thereof(Reference Fenton, Dai and Lalari4,Reference Cormack, Embleton and van Goudoever72–Reference Martin, Connelly and Bland77) . Similarly, growth assessment in infants and children with certain chronic conditions can be challenging. Length measurement may be impossible in infants/children with neurological impairment, necessitating the use of proxy measurements such as limb segments and circumferences(Reference Romano, van Wynckel and Hulst78). Furthermore, standard growth charts may not be applicable in conditions where ‘normal’ growth cannot reasonably be expected. Specialised growth charts are available for several clinical conditions, including (but not limited to) cerebral palsy(Reference Day, Strauss and Vachon79), Down Syndrome(Reference Zemel, Pipan and Stallings80), Turner Syndrome(Reference Lyon, Preece and Grant81), Prader–Willi syndrome(Reference Butler and Meaney82) and Noonan Syndrome(Reference Witt, Keena and Hall83), but their use is not universally accepted, particularly in infancy and early childhood(Reference Romano, van Wynckel and Hulst78). Body composition assessment may be of value in these populations, although the appropriate indicators of malnutrition remain unclear(Reference Romano, van Wynckel and Hulst78).
Body composition can be assessed by various methods in the first two postnatal years. Although uncommon in clinical practice, research settings may use ADP, stable isotope dilution and dual-energy X-ray absorptiometry(Reference Demerath and Fields68). Body volume is measured by ADP and combined with weight to calculate body density, from which FM and FFM can be calculated(Reference Demerath and Fields68,Reference Mazahery, von Hurst and McKinlay84) . The PEAPOD™ ADP device is only suitable for infants up to 10 kg(Reference Mazahery, von Hurst and McKinlay84,Reference de Fluiter, van Beijsterveldt and Goedegebuure85) , which some infants may reach already at 6–8 months, although smaller infants may remain under 10 kg up to 2 years of age or beyond(66). The BODPOD™ with paediatric attachment can be used for infants from 12 kg, but is only validated from 2 years of age(Reference Fields and Allison86). Stable isotope dilution may be used to assess body composition in infants and children of any age but may be impractical in neonates(Reference Demerath and Fields68,87) . The method involves administering a known dose of a stable isotope (e.g. deuterium-containing water), allowing it to equilibrate in the body and measuring its concentration in saliva or urine. This allows for the calculation of total body water mass and, by extension, FFM and FM(87). The final method, dual-energy X-ray absorptiometry, estimates body composition from the measured attenuation of X-rays through the body(Reference Demerath and Fields68). Unlike the previous two methods, dual-energy X-ray absorptiometry can further distinguish between bone and soft tissue (water and protein). The high cost limits the availability of dual-energy X-ray absorptiometry, and repeated X-ray exposure may not be desirable(Reference Demerath and Fields68). Reference data have been published for each of these methods (Table 4). Bioelectrical impedance analysis has been proposed as potentially useful in infants, but at this time none of the available predictive equations for the calculation of body composition parameters have been sufficiently validated to be recommended for clinical or research use, as found in a recent (2021) systematic review(Reference Lyons-Reid, Derraik and Ward88). It should be emphasised that body composition data obtained using different methods are not interchangeable(Reference Demerath and Fields68,Reference Wrottesley, Pisa and Micklesfield89) , so it is important to use reference data compiled using the same methodology. No cut-off values for indicators of body composition abnormality have been established.
Integrated framework
The preceding discussion identifies numerous measurements, indices and indicators for assessing growth in the first 1000 d of life. Integrating these disparate parameters into a single, unified framework implies identifying those parameters that can meaningfully be compared across the antenatal, perinatal and postnatal periods. The relationship between selected measurements and indices is illustrated in Fig. 2, with solid arrows indicating directly comparable parameters and dashed arrows indicating parameters that are related but not identical. The transition between prenatal and postnatal growth assessment is necessarily somewhat disjointed due to the indirect nature of foetal biometry.

Fig. 2 Integration of growth parameters throughout the first 1000 d of life
Discussion and recommendations
Growth assessment in the first 1000 d of life involves numerous clinical disciplines, measurements, indices and indicators. Growth assessment is inextricably linked to a life-course approach: current growth status results from earlier growth patterns and guides future clinical management. Unfortunately, limited contact between pre- and postnatal healthcare providers undermines interdisciplinary communication and continuity of care. This paper proposes an integrated, interdisciplinary framework for growth assessment in the first 1000 d of life, with the aim of promoting a common understanding among all clinical disciplines involved in this critical period.
Developing a common language among professions as diverse as obstetrics, midwifery, paediatrics, nursing and dietetics/nutrition is no small feat. A shared understanding of measurements and indices and the relationship between them (as shown in Fig. 2) is an important first step. Of course, not all possible measurements, indices and indicators can (or need to) be included in a unified framework, but those parameters that may meaningfully predict future outcomes should be understood by and communicated to all. To this end, differences in terminology, measurement methods, reference data, reporting systems and indices and indicators of interest need to be identified and, where possible, harmonised. For example, in paediatrics, the use of z-scores has become ubiquitous due to their mathematical and statistical utility, whilst percentiles are still preferred in many obstetric settings. Indeed, until recently, no z-score reference data were available for foetal growth indices, but presently the INTERGROWTH-21st Fetal Growth Standards(Reference Papageorghiou, Ohuma and Altman33,Reference Stirnemann, Villar and Salomon34) and Fetal Medicine Foundation’s Fetal Growth calculator(32) both include z-scores. Furthermore, the INTERGROWTH-21st Growth Standards and the Fenton 2013 Growth Chart all demonstrate good continuity with the WHO MGRS Growth Standards, further facilitating the integration of pre- and postnatal growth assessment(Reference Fenton and Kim53,Reference Villar, Giuliani and Bhutta90–Reference Villar, Papageorghiou and Pang92) . Thus, the tools for integration of growth assessment from conception through childhood are there; it is up to us – the clinical and research communities – to embrace these opportunities and optimise patient care and research reporting. Selecting the best set of tools to facilitate such integration will require careful consideration of the scientific merits and drawbacks of each reference. The debate surrounding the optimal growth chart for preterm infants is a good example: despite the conceptual coherence and strict individual-level inclusion criteria of the INTERGROWTH-21st Postnatal Growth Standards for Preterm Infants, the limited sample size at postmenstrual ages < 36 weeks is a serious concern(Reference Cormack, Embleton and van Goudoever72). For this reason, the American Academy of Paediatrics recommend the use of intrauterine (i.e. birthweight-derived) charts for monitoring the postnatal growth of preterm infants(93). Evidence of the superiority of one growth chart over the other, particularly in ethnically diverse LMIC populations, is still lacking.
Three additional practical considerations underlie the successful integration of growth assessment across the first 1000 d. Firstly, measurements must be taken accurately, which requires functional equipment and adequately skilled and motivated measurers. This also implies standardised measurement techniques, e.g. of length measurements at birth and during infancy. Secondly, accurate assessment and documentation of GA is crucial, owing to the non-linearity of growth in the first 1000 d. And finally, measured values must be documented and made available to all members of the health care team. In industrialised countries, universally accessible electronic health records can facilitate this. Where such infrastructure may not be available, a patient-held document (such as the child’s vaccination record) may fulfil the same role.
The measurements, indices and indicators outlined in this paper represent an ideal. However, some of the mentioned equipment and skills may not be available in LMIC and other resource-limited settings. Nonetheless, there are feasible, accessible practices that can be incorporated to improve growth assessment and contribute meaningfully to patient care outcomes. In the prenatal period, routine screening with a low-cost Doppler device (e.g. the Umbiflow™ device) has proven valuable for detecting foetuses at risk of FGR and stillbirth(Reference Vannevel, Vogel and Pattinson48,Reference Nkosi, Makin and Hlongwane94) . At birth, accurate assessment of length and HC, and standardised weighing of the placenta (with calculation of BW:PW ratio), may provide important information about foetal growth. During infancy and childhood, routine assessment of length-for-age, WFL and MUAC can provide valuable information about health and nutrition status that will be missed if only weight-for-age is assessed.
Figure 3 places this theoretical discussion into a practical context, showing how clear communication and information sharing between the antenatal and postnatal care teams can help identify neonates with FGR, who may be at increased risk of growth anomalies, and guide appropriate growth monitoring and promotion in infancy and childhood. Crucially, this information allows postnatal care providers to set appropriate growth targets for postnatal growth and tailor nutrition interventions accordingly. Nutrition, acute/chronic illness and socio-economic factors are highlighted as important determinants of growth; these represent potential areas for intervention to optimise growth and, by extension, long-term health. It also underscores the fact that some factors contributing to poor/excessive growth are systemic and not under the control of the individual. The purpose of clinical labelling is to achieve a concise common language among those involved in health promotion, malnutrition prevention and clinical care. Stigmatisation and blaming of the child and caregiver should be avoided at all cost. Using non-judgemental ‘people-first’ language – for example, using the term ‘child with obesity’ or ‘child with a BMI in the obese category’ rather than ‘obese child’ – can further help to mitigate possible negative effects of clinical labelling.

Fig. 3 Birth as link between antenatal and postnatal health care teams, in the context of identifying and managing the neonate with foetal growth restriction (FGR)
Policy and programmatic implications
Any change in the status quo requires a supportive policy environment. In a public healthcare system that uses standardised clinical documents and practice guidelines, the following is recommended:
-
Incorporating Doppler screening as a test for placental function in basic antenatal care services and recording these results in the child’s health record and vaccination card at birth;
-
Standardising measurement techniques of birth anthropometry, particularly length measurement;
-
Standardising methods for weighing the placenta, and including calculation of BW:PW ratio;
-
Incorporating newborn growth charts for birth weight, HC and length in maternity and child health care records;
-
Including the measurement of length and the assessment of WFL and/or BMI-for-age in routine growth monitoring; and
-
Ensuring that all policies and practices relating to health care worker education, clinical practice and clinical record keeping foster and support the integration of health care across disciplines and over time.
Each of these recommendations requires investment in equipment and training, but the benefit to the lives of infants and young children is likely to be substantial. The political will of policy makers, integrated record-keeping systems, the willingness of health care practitioners to put child well-being above disciplinary advancement, fostering teamwork in professional education and ongoing validation of growth assessment are at the foundation of achieving each child’s genetic potential in the first 1000 d.
Recommendations for research and practice
This framework is not presented as a conclusive standard, but rather as a proposal to prompt discussion, collaboration and research. The long-term usefulness of some indices and indicators (e.g. the various proposed indicators of asymmetry at birth) in predicting short- and long-term adverse outcomes presents an important research opportunity. For this reason, we also recommend that basic measurements (e.g. accurate birth weight, length and HC) always be carefully done and recorded, as the emergence of new indices/indicators can then potentially allow for re-analysis of existing datasets. Research into novel measurements and indices is ongoing, yet even well-established indices still lack agreement in terms of indicators and cut-offs. Research in these fields should focus on identifying indicators that can usefully predict important outcomes, including mortality, morbidity, growth and neurodevelopment. The validity, predictive value and optimal cut-offs of proxy indicators to replace ultrasonography (such as Doppler screening and measurement of the placenta at birth) particularly require investigation. Finally, interdisciplinary approaches should be integrated into pre-service education and continuing professional development initiatives.
Conclusion
Growth occurs on a continuum from conception throughout infancy and childhood, with events in the first 1000 d of life known to have life-long effects. It is crucial, therefore, that clinicians providing care to mothers, infants and young children in different life stages should be able to clearly communicate about common goals and concerns. This paper presents a framework to act as a starting point for such an integrated approach and also highlights areas where further research and policy initiatives are required. Clear communication, a collaborative approach and strong policy-level support will be needed to ensure continuity of care throughout the first 1000 d of life and ultimately promote optimal health and developmental outcomes.
Acknowledgements
Acknowledgements: The authors wish to acknowledge the contributions of all the members of the multidisciplinary think tank: Dr Tsakane Hlongwane, Dr Felicia Molokoane, Mothusi Nyofane, Tanita Botha, Dr Marinel Hoffman, Bontle Mamabolo. Authorship: Conceptualisation: RP, UF, FW; Writing – original draft: SN, FW, VV; Writing – review and editing: SN, FW, VV, UF, HM; Visualisation: SN; Supervision: FW, UF, RP. Ethics of human subject participation: This research did not involve any human participants or animal subjects.
Financial support:
This research received no specific grant from any funding agency, commercial or for-profit sectors.
Conflict of interest:
None of the authors have any conflict of interest to declare.