Malnutrition and growth faltering remain common among children in developing countries. In 2005, intra-uterine growth retardation and severe wasting and stunting were responsible for 2·2 million deaths and for 21 % of disability-adjusted life years in children under 5 years of age globally(Reference Black, Allen and Bhutta1). In rural Indonesia in 2007, 22 % of under 5-year-old children were moderately or severely wasted, whereas the prevalence of moderate or severe stunting was 66 %(2). The process of malnutrition begins early and has adverse short- and long-term effects on child morbidity and mortality(Reference Pelletier and Frongillo3), including poor subsequent growth(Reference Drewett, Corbett and Wright4) and cognitive and behavioural development(Reference Mendez and Adair5), as well as increased risk of adult chronic diseases including diabetes mellitus, CHD and stroke(Reference Barker6). A number of prenatal factors (e.g. low maternal height(Reference Blair, Drewett and Emmett7); inadequate intake of nutrients, especially micronutrients, during pregnancy(Reference Castillo-Duran and Weisstaub8); infection(Reference King9); high caffeine consumption(Reference Fenster, Eskenazi and Windham10, 11); and smoking(Reference Nordentoft, Lou and Hansen12)) have been shown to be related to stunting in children. The immediate factors related to wasting are insufficient supply of protein, energy and micronutrients or severe or frequent infections, especially diarrhoea. The underlying factors are household food insecurity, insufficient child and maternal care, ill health and an unhealthy environment(Reference Müller and Kravinkel13).
Consequently, actions to prevent malnutrition and growth faltering in children should begin as early as possible, perhaps even during pregnancy. Prenatal micronutrient supplementation, especially with Zn, vitamin A and Fe, has attracted attention, as these micronutrients have possible benefits for both the mother and infant. Pregnant women in developing countries show a high prevalence of micronutrient deficiencies(Reference Hambidge, Abebe and Gibson14). However, studies on prenatal micronutrient supplementation and child growth have yielded conflicting results. Supplementing pregnant women with a multiple micronutrient preparation led to significantly increased fetal growth in one study, whereas another study showed similar effects from Zn supplementation alone and a third study showed no effect on birth size(Reference Castillo-Duran and Weisstaub8, Reference Merialdi, Caulfield and Zavaleta15, Reference Hafeez, Mehmood and Mazhar16).
Micronutrient deficiencies are still prevalent among pregnant women in Indonesia. In Purworejo district, Central Java, which was the setting of the present study, 5 % of pregnant women were vitamin A deficient (serum retinol <0·7 μmol/l), with 83 % of these women having a dietary intake of vitamin A lower than the Indonesian RDA (700 retinol equivalents (RE))(Reference Persson, Hartini and Greiner17). Zn deficiency measured as S-Zn <10·7 μmol/l affected 62 % of pregnant women(Reference Persson, Hartini and Greiner17). It should be noted that Zn requirements are higher during pregnancy, when approximately half of the absorbed Zn is deposited in the growing fetus and approximately a quarter is deposited in the uterus. Furthermore, S-Zn levels are physiologically lower as a result of haemodilution, lower levels of Zn-binding protein and hormonal changes. The lower Zn concentrations seen among pregnant women in Purworejo district may reflect these physiological changes, although increased requirements in combination with low bioavailability of Zn in the diet also cause Zn deficiency.
Fe deficiency is the third common micronutrient deficiency, with approximately 50 % of pregnant women in Purworejo being Fe deficient and 19 % having Fe-deficiency anaemia, although the use of antenatal Fe supplementation is widespread(Reference Persson, Hartini and Greiner17). Among pre-pregnant women, 17 % had chronic energy deficiency (BMI <18·4 kg/m2), 10 % were obese and 79 % did not meet the international recommendations for weight gain during pregnancy, contributing to adverse health outcomes for both mothers and newborns(Reference Winkvist, Stenlund and Hakimi18).
On the basis of the high prevalence of growth faltering, with its serious impact on children's health, growth and development in the future, we sought to assess the effects of Zn, vitamin A or vitamin A + Zn supplementation in pregnant women on child growth and the possible prevention of growth faltering up to 2 years of age in a rural area in Indonesia.
Participants and methods
Study design and participants
We conducted the present study in Purworejo district, Central Java, Indonesia, which in 1994 (at the beginning of the study) had a total population of 729 825. The study was coordinated by the Community Health and Nutrition Research Laboratories (CHN-RL) at the Faculty of Medicine, Gadjah Mada University, Yogyakarta, Indonesia, in collaboration with the Indonesian Ministry of Health.
Through a surveillance system we had access to 25 067 women of child-bearing age (Fig. 1). Out of 5736 pregnant women, 2735 women at a gestational age of ≥17 weeks were not eligible for a supplementation study. Of the remaining 3001 pregnant women, 828 were excluded for various reasons. Therefore, 2173 women at a gestational age of <17 weeks were included in the community-based, individually randomized, placebo-controlled and double-blinded study aiming to evaluate the impact of supplementation (vitamin A, Zn, vitamin A + Zn) on maternal morbidity and pregnancy. All participants gave their informed consent. The study was called the Zibuvita Study and was conducted from September 1995 to December 1999.

Fig. 1 The selection process showing selection of pregnant women recruited to the Zibuvita, Zinak and Pronak studies and their offspring included in the data analysis (SB, stillbirth; M, miscarriage; Zt, Zinak therapy: Fe and Zn groups; Zinak P, Zinak placebo group)
Infants born within the study period were, after approval of caregivers, included in one of two follow-up studies on children, the Zinak and Pronak studies. The Zinak Study was a double-blinded, randomized and controlled study aiming to evaluate the effect of supplementation of infants with Zn and/or Fe on their growth and morbidity from 6 to 12 months of age(Reference Lind, Lönnerdal and Stenlund19, Reference Lind, Lönnerdal and Stenlund20). The Pronak Study was an observational study monitoring children from birth onwards with respect to growth and development, feeding practices and morbidity.
The data generation process is illustrated in Fig. 1. Out of 1956 live births in the Zibuvita Study, 680 and 380 infants, whose mothers or caregivers gave consent and who could be followed up after the civil disruption experienced in Indonesia following the Asian economic crisis, were recruited to the Zinak Study and the Pronak Study, respectively. The disruption decreased staff size and data collection activities. Out of 1956 infants in the Zibuvita Study, 896 (46 %) could not be followed up because of the effects of the economic crisis affecting Indonesia from 1997 onwards. Out of the 380 infants in the Pronak Study, 108 were excluded because of missing data. Consequently, in the follow-up we included 272 children from the Pronak Study and all children allocated to the placebo group in the Zinak Study (n 71). To avoid the unwanted effects of postnatal supplementation on outcomes, we excluded children in the Zinak Study who received supplementation (n 609). Thus, we finally analysed 343 children.
The smallest difference between the supplementation and placebo groups with regard to weight-for-age Z-score (WAZ) and height-for-age Z-score (HAZ) that could be detected (α = 0·05, 1−β = 0·80) with the present sample size was 0·4.
Prenatal supplementation in the Zibuvita Study
The micronutrient capsules given to the pregnant mothers from the date of inclusion in the study until delivery contained either 2400 RE of vitamin A as retinyl palmitate or 20 mg of ZnSO4, or the same dose of both vitamin A and ZnSO4, or placebo. All capsules also contained 2 mg of dl-α-tocopherol as an antioxidant and 350 mg of soyabean oil, 20 mg of beeswax and 8 mg of lecithin as capsule filler. Mothers were randomly allocated in a 1:1:1:1 ratio in blocks of twelve on the basis of a list of treatment numbers derived from a pseudo-random number generated using the SAS statistical software package version 5·0 (SAS Institute Inc., Cary, NC, USA). The treatment allocation sequence was prepared and held at the University of Newcastle, New South Wales, Australia. All investigators, field and laboratory staff and participants were blinded to the treatment code until all field data had been collected and preliminary data analysis by coded groups had been completed. Fieldworkers distributed capsules and monitored compliance at the home of the women, resulting in consumption of 70 % of supplements(Reference Persson, Hartini and Greiner17).
Definitions
Growth faltering was defined as a downward change of either weight-for-age or height-for-age percentile crossing two or more major percentile lines (the 5th, 10th, 25th, 50th, 75th, 90th and 95th percentile lines) or WAZ < −2 sd (underweight), HAZ < −2 sd (stunting) or WHZ <−2 sd (wasting) using the WHO 2006 growth curve as reference(Reference Olsen21, 22). The growth chart of each participating child was reviewed individually and the point and time of downward crossing of two or more major percentile lines were recorded.
Prematurity, low birth weight (LBW) and small for gestational age (SGA) were defined as gestational age <37 weeks at birth, birth weight <2500 g and birth weight <10th percentile, respectively(Reference Lubchenco, Hansman and Boyd23). The definition of ‘1 d of diarrhoea’ indicated three or more liquid or semi-liquid stools, whereas ‘1 d of respiratory illness’ indicated the diagnosis of a child suffering from a runny nose or cough, with or without fever. When estimating the number of days with illness, the fieldworker took into account the 14 d before the visit. Duration of diarrhoea and respiratory illness included number of illness days during the 3-month period preceding growth faltering; for those who did not falter, a randomly selected 3-month period was used.
Household characteristics with respect to sources of drinking water and sanitation facilities were defined using the WHO criteria(24). ‘Improved sources of drinking water’ included household connections, public standpipes, boreholes, protected dug wells, protected springs and rain water collections, whereas ‘unimproved sources’ included unprotected wells, unprotected springs, vendor-provided water, bottled water (unless water for other uses was available from an improved source) and tanker truck-provided water. ‘Improved sanitation facilities’ included connection to public sewers or septic systems, pour-flush latrines and simple or ventilated improved pit latrines, whereas ‘unimproved facilities’ included service or bucket latrines from which excreta were manually removed, as well as public latrines and open latrines.
Data collection
At the start of the Zibuvita Study, trained fieldworkers collected identity and socio-economic data from the participating women through home interviews. In addition, anthropometric, dietary and morbidity data on the participating children were collected during home visits. Weight and length data were collected monthly from birth to 12 months of age, and then again at 18 and 24 months of age. Fieldworkers trained in standard anthropometric techniques for children measured weight using a portable electronic scale (Seca 835; CMS Weighing Equipment, London, UK), and length using a locally produced wooden measuring board. Weight was measured with the child completely naked and length was measured with the child in supine position. Measurements were taken in triplicate and mean values were recorded.
Data on breast-feeding practices were collected using the 24 h recall method: weekly during the first 2 months, biweekly during the third and fourth months, monthly from 5 to 18 months of age and finally at 24 months of age. The number of days with symptoms of diarrhoea and respiratory infections was recorded monthly using questionnaires in which the parents were asked for occurrence of symptoms of diarrhoea or respiratory infection on the day of visit by the fieldworker, followed by the same questions for the day before, 2 d before and so forth until 14 d before the visit.
Data entry and statistical analysis
Analyses were conducted using the Statistical Package for the Social Sciences statistical software package version 15·0 (SPSS Inc., Chicago, IL, USA). Anthropometric data were transformed into Z-scores using EpiInfo version 3·3 (Centers for Disease Control and Prevention, Atlanta, GA, USA) and then imported to SPSS for statistical analysis. Imputations for missing values of length (16 %) were carried out by assuming a linear growth pattern; for example, we imputed missing length data at 9 months of age as
![\[--><$$> {\rm{[(length}} \ {\rm{at}} \ {\rm{8}} \ {\rm{months}} + {\rm{length}} \ {\rm{at}} \ {\rm{10}} \ {\rm{months)/2]}}{\rm{.}} \eqno\rm<$$><!--\]](https://static.cambridge.org/binary/version/id/urn:cambridge.org:id:binary:20151030101137160-0190:S1368980011001078_eqnU1.gif?pub-status=live)
Differences between supplementation groups for dichotomous data or proportions were tested using the χ 2 test. ANOVA was performed to test comparability of continuous data. Two-way ANOVA was used to test the main effects and interaction of vitamin A or Zn supplementation on WAZ, HAZ and WHZ at 0, 3, 6, 9, 12, 18 and 24 months, as well as the absolute and relative weight and length growth rates from birth up to 24 months of age. ANCOVA was subsequently used to adjust for possible confounders and effect modifiers of prenatal supplementation on postnatal child growth. Statistical significance was set at P < 0·05 and two-sided hypothesis tests were used unless specified. To identify the determinants of time to first growth faltering, Cox's proportional hazard function was used and hazard ratio (HR) and 95 % CI were calculated.
Ethical considerations
Ethical approval was given by the Ethical Committee of the Medical School at Gadjah Mada University in Yogyakarta, Indonesia. Informed consent was obtained from the pregnant women after detailed information was given to the participants.
Results
Basic characteristics
There were no differences between the included (n 343) and not included (n 1613) women–child pairs with respect to key characteristics such as maternal age (P = 0·20), height (P = 0·09) and pre-pregnancy weight (P = 0·92), nor were there differences with respect to children's sex (P = 0·07), birth weight (P = 0·60) and length (P = 0·25), prevalence of LBW (P = 0·57), water source (P = 0·79), sanitation facilities (P = 0·90) or distribution of prenatal supplementation (P = 0·75).
Mean maternal age, height, pre-pregnancy weight and pregnancy weight increases were 29 years, 150 cm, 46 kg and 9 kg, respectively. The children's mean birth weight and length were 3·0 kg and 49 cm, respectively. The prevalence of LBW and small for gestational age was 7·0 % and 5·2 %, respectively. There were no statistically significant differences among supplementation groups with regard to baseline characteristics of mothers, children and households (Table 1).
Table 1 Basic characteristics of the mothers (pregnancy and education), children (perinatal) and household facilities
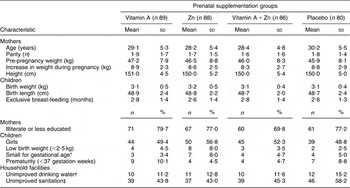
Growth patterns and impact of prenatal supplementation on growth
The growth pattern was similar in boys and girls. The WAZ and HAZ increased slightly from birth to 3 months of age, and thereafter faltered up to 18 months, followed by a slight increase up to 24 months of age. The WHZ decreased continuously from birth to 2 years of age.
Two-factor ANOVA was performed to test the main effects and interaction of prenatal supplementation with vitamin A or Zn. There was a significant main effect of vitamin A on WAZ at 6 months (P = 0·04) but there were no interaction effects of vitamin A and Zn, except at 6 months of age (P = 0·048; Table 2). However, this effect disappeared after adjusting for birth weight and length and for duration of breast-feeding, diarrhoea and respiratory illness (P = 0·67).
Table 2 Impact of prenatal vitamin A and zinc supplementation on child WAZ, HAZ and WHZ at 0, 3, 6, 9, 12, 18 and 24 months of age

WAZ, weight-for-age Z-score; HAZ, height-for-age Z-score; WHZ, weight-for-height Z-score.
*Covariates: birth weight, birth length, exclusive breast-feeding duration, duration of diarrhoea and duration of respiratory illness.
†Vitamin A v. Zn and placebo.
‡Zn v. vitamin A and placebo.
§Significant difference between the vitamin A and placebo groups (ANOVA P < 0·05).
∥Significant difference between the vitamin A and vitamin A + Zn groups (ANOVA P < 0·05).
¶Significant difference between the vitamin A and vitamin A + Zn groups (ANOVA P < 0·05).
**Significant difference between the vitamin A and Zn groups (ANOVA P < 0·05).
††Significant difference between the vitamin A and vitamin A + Zn groups (ANCOVA P < 0·005).
‡‡Significant difference between the vitamin A and vitamin A + Zn groups (ANCOVA P < 0·05).
§§Significant difference between the vitamin A and Zn groups (ANCOVA P < 0·05).
For HAZ, two-factor ANOVA showed a significant main effect of Zn at 6 months (P = 0·002) and significant negative interaction between vitamin A and Zn at 9, 12 and 18 months of age (P = 0·03, P = 0·04 and P = 0·02, respectively; Table 2); however, after controlling for covariates, the difference in HAZ at 3 months became significant (P = 0·03) and the differences at 9 (P = 0·04) and 18 (P = 0·04) months of age remained statistically significant. The absolute differences between the vitamin-A only and vitamin A + Zn groups at 3 and 9 months were 0·34 sd and 0·37 sd, respectively, and the absolute difference between the vitamin A-only and Zn-only groups at 18 months was 0·31 sd.
Finally, for WHZ, two-factor ANOVA did show a significant main effect of vitamin A (P = 0·04) at 6 months but no significant interaction effects of prenatal vitamin A and Zn supplementation. There was no effect of supplementation on growth rate at any age period with regard to weight, height or weight for height (data not shown).
Effects on prevalence of growth faltering
Growth faltering was more prevalent when defined as downward crossing of two or more major percentile lines compared with growth <−2 sd. Using either method, the prevalence of growth faltering increased with age (Figs 2(a) and(b)). The majority of children had crossed two or more major percentile lines by 9 months of age. Prenatal supplementation did not affect the risk of growth faltering. Birth length was the only covariate to be significantly associated with this risk in the present study. An increase in birth length of 1 cm reduced the risk of growth faltering by 9 % (HR = 0·910; 95 % CI 0·831, 0·997; Table 3).

Fig. 2 Prevalence of growth faltering up to 2 years of age, defined as either crossing two or more percentile lines () or growth <−2 sd below the reference population (
) for weight-for-age (a) and height-for-age (b).
Table 3 Risk for growth faltering in relation to some potential risk factors in children from prenatally supplemented mothers in rural Java, Indonesia, analysed using Cox proportional hazard regression

HR, hazard ratio.
*Duration of illness before growth faltering was initially identified.
Discussion
In the present study we found no effect on postnatal child growth from prenatal supplementation with either vitamin A, a combination of vitamin A and Zn, or Zn alone, compared with placebo. However, there was a positive effect of vitamin A supplementation alone on subsequent length of children in comparison with a combination of vitamin A and Zn or with supplementation with Zn only. The effects were small, ranging from 0·31 to 0·37 sd, and inconsistent, as they appeared only at certain ages, that is, at 3, 9 and 18 months, but not at the other time points measured, including birth. In addition, the effects were seen only at the cross-sectional monthly measurements of HAZ, with no effects over time on growth rate or on any of the other anthropometric measurements. Furthermore, the prenatal supplements did not prevent the development of growth faltering.
The minor effects of prenatal vitamin A and/or Zn supplementation on growth indicate that intra-uterine growth is influenced by multiple factors and complex mechanisms. The prevalence of vitamin A, Zn and Fe deficiency among the pregnant women at the present study site indicates multiple micronutrient shortages, possibly reducing the effects of any single supplement(Reference Persson, Hartini and Greiner17). On the other hand, the vitamin A deficiency prevalence of 5 %, indicating only a mild public health impact, may have been insufficient to respond to supplementation. Postnatal vitamin A supplementation has improved growth where endemic deficiency exists(Reference West, Djunaedi and Pandji25). The low prevalence of SGA and LBW compared with that in many other low-income settings may indicate that the fetal nutritional status in the present population was sufficiently good to not respond to prenatal supplementation.
In progeny, maternal vitamin A deficiency has been related to LBW, indicating intra-uterine growth retardation, increased morbidity and poor growth or stunting until 6 months of age(Reference Tielsch, Rahmathullah and Katz26). From animal studies it has been hypothesized that vitamin A stimulates growth by a direct role in cell replication(Reference Zile, Bunge and DeLuca27). In rodents, moderate vitamin A deficiency during pregnancy has been reported to reduce lung weight and muscle development in the offspring(Reference Downie, Antipatis and Delday28). Zn is essential for the activity of over 300 enzymes involved in processes such as mitosis, DNA synthesis and gene activation and expression. Approximately 82 % of pregnant women worldwide are likely to have inadequate Zn intake(Reference Osendarp, West and Black29). Animal studies have shown that mean values of birth weight, birth length and tail length were lower in litters from Fe- or Zn-deficient dams(Reference Shahbazi, Naghdi and Tahmaseli30). In addition, providing postnatal Zn supplementation to Zn-deficient children has been shown to improve growth(Reference Olsen21).
Studies on the effects of prenatal supplementation with vitamin A, Zn or multiple micronutrient combinations containing either vitamin A or Zn on infant growth have shown inconsistent results. Several studies from Nepal have investigated the effects of micronutrient supplementation during pregnancy on outcomes in children. Osrin et al. (Reference Osrin, Vaidya and Shrestha31) supplemented pregnant women with fifteen micronutrients and minerals, including vitamin A and Zn, and reported increased birth weight compared with supplementation with vitamin A alone, whereas Katz et al. (Reference Katz, Christian and Dominici32) reported not only higher birth weight but also a change in birth weight distribution as a result of certain combinations of antenatal supplementation. A follow-up of the latter study population showed a lingering positive impact of maternal Fe, folic acid and Zn supplementation on height and subcutaneous fat at 6–8 years of age(Reference Stewart, Christian and LeClerq33). A follow-up study by Vaidya et al. (Reference Vaidya, Saville and Shrestha34) on 2·5-year-olds showed that weight, head, chest and mid-arm circumference and triceps skin folds remained higher in the multiple micronutrient group, but with no effect on height, compared with Fe + folic acid supplementation during pregnancy. The benefits of prenatal multiple micronutrient supplementation on birth size, neonatal morbidity and mortality and later growth have also been shown in studies conducted in other developing countries(Reference Gupta, Ray and Dua35–Reference Bhutta, Rizvi and Raza39). In one meta-analysis, reduction of LBW was found among infants of mothers prenatally supplemented with multiple micronutrients compared with placebo or Fe and folic acid(Reference Shah and Ohlsson40), whereas other meta-analyses or reviews concluded no added benefits of multiple micronutrient or Zn supplementation on birth weight(Reference Haider and Bhutta41, Reference Hess and King42).
The interpretation of these results, in relation to ours, is that composition and dosage of micronutrients as well as the time during pregnancy when supplements are introduced are crucial for infant growth and may explain the differences seen with different micronutrient and supplementation regimes.
Interactions between vitamin A and Zn may have decreased the effects of prenatal supplementation on growth in the present study. Children of mothers supplemented with the combined vitamin A and Zn supplement were shorter than those who were supplemented with vitamin A only at certain ages. We have no measure of either vitamin A or Zn status of mothers after supplementation; hence, we cannot say whether this interaction was evident in mothers as well. Interactions between vitamin A and Zn during pregnancy have been shown in animal studies. One study showed an increase in hepatic vitamin A concentration in fetuses when dams were fed decreasing amounts of Zn, reflecting impaired mobilization of vitamin A. Another study on pregnant rhesus monkeys showed that, above a certain threshold of plasma Zn, vitamin A transport was not dependent on plasma Zn concentration but that, below the threshold, vitamin A release and transport from the liver was strongly influenced by plasma Zn concentrations. In humans, very few studies have shown interactions between vitamin A and Zn. One study indicated a weak and non-significant correlation between liver Zn and vitamin A content in fetuses and stillborn infants and in infants who died within the first 4 months of life(Reference Christian and West43). These studies shed little light on our findings as, although the women were supplemented with both vitamin A and Zn, their progeny grew less well. Therefore, the conflicting results of the present study should be interpreted with caution, requiring biological explanations unavailable at this time; they may also indicate chance findings.
In the present study, using downward crossing of two or more major percentile lines as the marker for growth faltering, a substantial proportion of children had signs of faltering in weight and height before 6 months of age, and the majority of children were faltered by 9 months of age. Stunting and wasting among children under 5 years of age are associated with significant morbidity and mortality throughout life(Reference Checkley, Buckley and Gilman44, Reference Shrimpton, Victora and De Onis45). In our study, the prevalence of growth faltering was lower if WAZ or HAZ <−2 sd was used. Therefore, crossing major percentile lines may be an earlier sign of growth faltering compared with reaching Z-scores <−2 sd and may offer opportunities for earlier intervention to reverse the faltering growth trend.
The small but positive effects of prenatal vitamin A supplementation on child growth in our study add to a body of conflicting results with regard to maternal supplementation studies, suggesting that more research is required. The children in the present study were born to mothers recruited to an individually randomized, placebo-controlled, double-masked, community-based trial conducted in a rural area with high incidence of micronutrient deficiencies among pregnant women. The adherence to the supplementation scheme (70 %) was high. All outcomes, data on breast-feeding practices and symptoms of infectious diseases, which were potential confounders or effect modifiers, were collected prospectively until 24 months of age. Previous studies have indicated that birth weight and length(Reference Arifeen, Black and Caulfield46, Reference Schmidt, Muslimatun and West47), breast-feeding(Reference Villalpando and López-Alarcón48) and child morbidity, especially diarrhoea(Reference Checkley, Buckley and Gilman44, Reference Moore, Lima and Conaway49) and respiratory illness(Reference Adair and Guilkey50, Reference Umeta, West and Verhoef51), are factors that may affect postnatal growth. After controlling for these covariates, the associations between prenatal vitamin A on child growth remained significant.
A limiting factor in our study was the large loss to follow-up from the initial study to the present. Out of the 1956 live births from the women participating in the Zibuvita Study, only 343 could be included in the final analysis. However, we found no statistically significant differences with respect to key characteristics between these children and those not included. Moreover, the original sample size itself may have been too small to detect growth differences of public health importance. The effects of prenatal supplementation on symptoms of infectious diseases, which can modify the effects on growth, may also have been underestimated, since methods using recall may under-report symptoms of disease, as shown in an Indian study(Reference Ramakrishnan, Venkatarao and Koya52). Nevertheless, all supplementation groups were followed up in a similar manner, which should have adjusted for at least large intergroup variations. Future studies on the impact of prenatal supplementation should clarify the mechanisms and effects of actions and interactions of micronutrients, alone or in combination, on postnatal child growth, bringing deeper understanding to the public health benefits of prenatal supplementation, as well as better tools to prevent childhood growth faltering from the earliest possible date.
In conclusion, the present study shows no effect of prenatal supplementation with either vitamin A or a combination of vitamin A and Zn, or with Zn alone, compared with placebo, on postnatal child growth. However, there was a positive effect of vitamin A supplementation alone on subsequent length of children, compared with a combination of vitamin A and Zn or supplementation with Zn only, but without an effect on other anthropometric measures, growth rate or prevalence of growth faltering. Future studies should duplicate these findings before recommendations on prenatal vitamin A supplementation can be made. Using downward crossing of two or more major percentile lines as the definition of growth faltering, the findings indicate that a substantial proportion of children in the study showed signs of poor growth and at an earlier age than seen using a definition of Z-scores <−2 sd.
Acknowledgements
The present study was supported by grants from MotherCare, John Snow Inc., Washington, DC, USA, and from UNICEF, Jakarta, Indonesia, to the Gadjah Mada University and to the University of Newcastle, Newcastle, Australia. Infrastructure support was provided by the Community Health and Nutrition Research Laboratories (CHN-RL), Faculty of Medicine, Gadjah Mada University and by the Ministry of Health, Republic of Indonesia, through the Third Community Health and Nutrition Development Project Loan from the World Bank (IBRD loan no. 3550-IND). The present study was partly undertaken within the Centre for Global Health at Umeå University, with support from Forskningsrådet för arbetsliv och socialvetenskap (FAS), the Swedish Council for Working Life and Social Research (2006-1512). The authors have no conflict of interest to declare. E.P.P. analysed the data and wrote the manuscript; T.L., L.N., A.I. and H.S. supported the data analysis and contributed to the development of the manuscript. The authors are grateful to Professor Mohammad Hakimi, Department of Obstetrics and Gynaecology and CHN-RL, Medical School, Gadjah Mada University, and to Michael J. Dibley, Senior Lecturer in Epidemiology, Faculty of Medicine and Health Sciences, Centre for Clinical Epidemiology and Biostatistics, School of Population Health, University of Newcastle, Callaghan, NSW, Australia, for providing the Zibuvita data. They also thank Dr Detty S. Nurdiati, Department of Obstetrics and Gynaecology, Professor Djauhar Ismail, Division of Growth and Development, Department of Child Health, and Ekawaty L. Haksari, Division of Perinatology, Department of Child Health, all at Medical School, Gadjah Mada University, Yogyakarta, Indonesia, for their comments on the manuscript, and Muhammad Arifin, CHN-RL, for data preparation.