Inflammatory bowel diseases
Inflammatory bowel diseases (IBD) are chronic and relapsing conditions affecting the gastrointestinal tract. Based on the location of inflammation, IBD are classified as Crohn's disease (CD) or ulcerative colitis (UC). While CD is associated with deep inflammation in any part of the gastrointestinal tract, UC is linked to severe tissue damage of the colon and rectum(Reference Calder, Albers and Antoine1). In the 21st century, IBD has become a major burden in Western countries, with over 1⋅5 and 2 million people affected in North America and Europe, respectively(Reference Ng, Shi and Hamidi2). Although the exact pathogenesis is unclear, there is evidence that IBD develop in genetically susceptible individuals who have been exposed to environmental insults that lead to an aberrant immune response to commensal gut microbiota and chronic inflammation(Reference Abegunde, Muhammad and Bhatti3). Despite both CD and UC having a genetic basis, UC is more strongly affected by environmental factors than genetic factors, compared to CD(Reference Farrell and Peppercorn4). Relevant environmental factors include lifestyle (e.g. smoking, diet and stress), host microbiota (dysbiosis), pharmacologic agents (e.g. antibiotics), ecological factors (e.g. pollution) and surgery (e.g. appendectomy), with smoking having the greatest level of evidence for association with IBD than other factors according to the 2009 Oxford Centre for Evidence-based Medicine levels of Evidence(Reference Abegunde, Muhammad and Bhatti3). Genetic and environmental factors drive alterations to the gut immune response, leading to reduced ability to clear pathogenic bacteria(Reference Shanahan5), lower levels of goblet cells, Paneth cell dysfunction and increased secretion of inflammatory mediators, such as interleukins (IL-1β, IL-6, IL-17, IL-18, IL-22), TNF-α and interferon-γ(Reference Maloy and Powrie6). These processes can cause damage to the intestinal epithelium, which becomes permeable and susceptible to the commensal flora and their metabolites. Whereas in healthy conditions, the gut microbes live in a mutualistic relationship with the host (homeostasis), in IBD this balance is altered (dysbiosis) and the host responds to the commensal bacteria with an aberrant immune response. In recent years, prebiotic oligosaccharide fibres have been studied for their role in maintaining gut health, supporting the growth of health-promoting bacteria and positively modulating gut immunity(Reference Carlson, Erickson and Lloyd7). There is an increasing interest in using prebiotics as a preventive or supportive therapy in IBD, as this is a condition for which there is currently no cure but only maintenance treatments to mitigate the symptoms.
Non-digestible oligosaccharides
Non-digestible oligosaccharides (NDO) are fermentable dietary fibres with a role as prebiotics. According to the International Scientific Association for Probiotics and Prebiotics consensus statement(Reference Gibson, Hutkins and Sanders8), prebiotics are substrates that are selectively utilised by host microorganisms conferring a health benefit. Fructooligosaccharides (FOS), inulin and galactooligosaccharides (GOS) are the most researched prebiotics. Additionally, lactulose-derived oligosaccharides, human milk oligosaccharides (HMO), arabinooligosaccharides, mannanoligosaccharides, xylooligosaccharides, pectic oligosaccharides and glucose-derived oligosaccharides are emerging prebiotics, as the level of evidence of their health benefits is lower than for FOS, inulin and GOS(Reference Gibson, Hutkins and Sanders8–Reference Davani-Davari, Negahdaripour and Karimzadeh10). NDO are carbohydrates made up by three to ten monosaccharide units, usually glucose, galactose, fructose and xylose. The number of monomeric units constituting NDO is also referred to as the degree of polymerisation. The carbons of the monosaccharide units are linked by covalent β-glycosidic bonds rather than by α-glycosidic bonds found in digestible oligosaccharides. This β configuration of the bonds makes NDO indigestible by human salivary and digestive enzymes(Reference Mussatto and Mancilha11). FOS and inulin are made by fructosyl monomers linked by β-(2,1) bonds attached to a terminal glucosyl residue by an α-(1,2) bond. They differ in terms of the length of sugar chains, which are shorter for FOS (2–8 units) than for inulin (up to 60 units)(Reference Kelly12). GOS are made up by galactopyranosyl units linked by β-(1,4) or β-(1,6) bonds and terminating with a glucosyl residue linked by β-(1,4) bonds (Fig. 1)(Reference Meyer, Miguel, Fernández, Ayman and Amer13). Dietary sources of NDO are plants and include chicory, garlic, Jerusalem artichoke, leeks, onions, bananas, barley and wheat(Reference Lomax and Calder14). FOS, inulin and GOS are also produced industrially either by trans-glycosylation of monosaccharides or by enzymatic hydrolysis of polysaccharides(Reference Mussatto and Mancilha11).

Fig. 1. Structure of fructooligosaccharides (FOS) and galactooligosaccharides (GOS). (a) FOS are made by fructosyl monomers linked by β-(2,1) bonds attached to a terminal glucosyl residue by α-(1,2) bond. (b) GOS are made by galactopyranosyl units linked by β-(1,4) or β-(1,6) bonds and terminating with a glucosyl residue linked by β-(1,4) bonds. Fru, fructose; Gal, galactose; Glu, glucose.
Prebiotic mechanism of action
Due to their chemical structure, prebiotic NDO resist low gastric pH and hydrolytic enzymes and reach the colon virtually intact, where they are degraded by specific gut bacteria collectively referred to as the gut microbiota(Reference Huttenhower, Gevers and Knight15).
Lactobacilli and bifidobacteria break down NDO via saccharolytic reactions and use them as energy sources to support their growth. In doing so, SCFA are generated as volatile end-products of the fermentation process. These SCFA, which include acetate, propionate and butyrate(Reference Esgalhado, Kemp and Damasceno16), exert beneficial health effects for the host such as inhibition of pathogens, maintenance of gut barrier integrity, regulation of glucose and lipid metabolism and modulation of immunity(Reference Rios-Covian, Ruas-Madiedo and Margolles17–Reference Valentini, Piermattei and Di Sante21).
Traditionally, immunomodulatory effects of prebiotics were thought to result exclusively from the actions of SCFA and other metabolites (e.g. bactericidal molecules) produced by the microbiota. For example, SCFA are known to modulate cytokine production and immune cell functions (dendritic cells (DC), T cells) as well as to inhibit several pro-inflammatory pathways, as extensively reviewed elsewhere(Reference Carlson, Erickson and Lloyd7, Reference Vieira, Teixeira and Martins22–Reference Schley and Field24). There is growing interest in understanding whether NDO can also modulate immunity in a non-prebiotic manner, especially in those individuals with increased gut permeability, by directly interacting with systemic and gut immune cells. Addressing the aim of this review, microbiota-independent effects of NDO on immunity will be discussed in more detail.
Microbiota-independent effects of non-digestible oligosaccharides on immunity
Evidence for intestinal transportation of non-digestible oligosaccharides
Prebiotics may pass through the gut barrier and enter in direct contact with gut and systemic immune cells when the gut is immature, such as in infants(Reference Weaver, Laker and Nelson25, Reference Commare and Tappenden26), or in other situations characterised by increased gut permeability, such as IBD(Reference Michielan and D'Incà27), obesity(Reference Fasano28, Reference Vajro, Paolella and Fasano29), type 1 diabetes(Reference Vaarala30) and non-alcoholic fatty liver disease(Reference Nicoletti, Ponziani and Biolato31). It is conceivable that NDO may be transported across the intestinal barrier also in individuals exposed to lifestyle-associated stressors that have been linked to alterations in gut permeability, such as high-fat Western diet, alcohol consumption and use of medications(Reference Camilleri, Lyle and Madsen32).
Studies in vitro using cell lines demonstrated that neutral and acidic HMO are transported across the human epithelial colorectal adenocarcinoma cell monolayer via transcellular and/or paracellular pathways(Reference Gnoth, Rudloff and Kunz33) and that short-chain GOS/long-chain FOS are transferred with the rates of 4–14 % depending on their molecular size and structure(Reference Eiwegger, Stahl and Haidl34). In human supplementation studies, HMO, FOS and GOS with a degree of polymerisation between 3 and 9 were found in plasma, urine and stool of infants fed with supplemented human milk or formula containing FOS or GOS(Reference Prieto35, Reference De Leoz, Wu and Strum36), confirming the transport of intact oligosaccharides across the intestinal epithelium, as summarised in Tables 1 and 2. Although it is plausible that prebiotics are transported across the intestinal epithelium not only in infants but also in adults with increased gut permeability, there is an important gap in the research field that needs addressing to support this hypothesis.
Table 1. In vitro studies providing evidence for intestinal transportation of prebiotics

HMO, human milk oligosaccharides; Caco-2 cells, human epithelial colorectal adenocarcinoma cells; HPLC-MS, high-performance liquid chromatography MS; scGOS/lcFOS, short-chain galactooligosaccharides/long-chain fructooligosaccharides; HPAEC-PAD, high-pH anion-exchange chromatography with pulsed amperometric detection.
Table 2. Human studies supporting evidence for intestinal transportation of prebiotics

FOS, fructooligosaccharides; DP, degree of polymerisation; HMO, human milk oligosaccharides; GOS, galactooligosaccharides; B. animalis, Bifidobacterium animalis; LC-TOFMS, liquid chromatography time-of-flight MS.
Evidence for direct effects of non-digestible oligosaccharides on immunity
A systematic literature search was conducted in Ovid MEDLINE(R) from 1946 through December 2019 and EMBASE from 1947 through December 2019. The search terms used included ‘Prebiotic* or Fibre* or Fiber* or Oligo?saccharide* or Oligo?saccharide* fraction* or Human?milk oligosaccharide* or HMO* or Human milk-derived oligo?saccharide* or non?digestible oligosaccharide* or NDO’ and ‘Monocyte* or Lymphocyte* or Dendritic cell* or monocyte* derived dendritic cell* or Intestinal epithel*’ and ‘Gastro?intestinal epithelial transfer or Gastro?intestinal adj2 epithelial adj2 transfer or epithelial transport* or in?vitro transfer or CaCo-2 cell monolayer* or intestinal epithelial cell* or IEC*’. After deduplication, relevant papers were selected and bibliographies of the retrieved articles were searched to identify additional articles of interest.
Twelve in vitro studies involving human or animal cell lines and/or primary cultures and one in vivo study using germ-free mice were reviewed to assess whether NDO could directly affect immunity (Tables 3–5). Three out of thirteen studies focused on HMO(Reference Bode, Kunz and Muhly-Reinholz37–Reference Newburg, Ko and Leone39), whereas the other studies focused on other NDO including FOS, inulin, GOS and/or a combination of these(Reference Zenhom, Hyder and de Vrese40–Reference Lin, Chen and Chen46). Of those studies that looked at the effects of NDO on human cell lines, HMO acidic fractions (12⋅5–125 μg/ml) showed anti-inflammatory effects by dose-dependently inhibiting leucocyte rolling and adhesion, which are two inflammatory processes involved in tissue damage, to human umbilical vein endothelial cells(Reference Bode, Kunz and Muhly-Reinholz37). Treatment with HMO and GOS (both 5 g/l) attenuated TNF-α-, IL-1β- and pathogen-induced inflammatory cytokines (IL-8, monocyte chemoattractant protein-1 (MCP-1) and macrophage inflammatory protein-3 α) in H4 cells, a cell model for human immature intestine(Reference Newburg, Ko and Leone39). When the same experiment was conducted on T84 and NCM-460 cell lines to mimic mature intestinal cells, TNF-α-induced macrophage inflammatory protein-3α was reduced by HMO and GOS treatment. However, TNF-α-induced IL-8 was inhibited in NCM-460, but not T84, cells only by HMO(Reference Newburg, Ko and Leone39). Inflammatory NF-κB signalling was attenuated in H4 and NCM-460 cell lines(Reference Newburg, Ko and Leone39). A reduction in the expression of pro-inflammatory IL-8, as well as IL-12 and TNF-α, was seen after treatment of human epithelial colorectal adenocarcinoma cells with α3-sialyllactose HMO (50 mg/l) and FOS (50 g/l), whose anti-inflammatory effects are likely linked to the induction of PPARγ(Reference Zenhom, Hyder and de Vrese40). When the same cell line was incubated with lower concentrations (5 g/l) of FOS, inulin, GOS and goat milk oligosaccharides (GMO), reduced production of MCP-1, but not IL-8, was observed. However, in the same study, stimulation with FOS, inulin and GMO but not GOS resulted in a higher IL-8 production by human colon cancer cell lines (HT-29), in contrast to the studies on H4, NCM-460 and human epithelial colorectal adenocarcinoma cells(Reference Ortega-Gonzalez, Ocon and Romero-Calvo43). Overall, in vitro work conducted on cell lines consistently supports the hypothesis that NDO are able to modulate cytokine production (e.g. IL-8, MCP-1) in a microbiota-independent manner. However, their anti-inflammatory effects appear to be cell-line-specific and the various NDO structures, doses and stimulation times used in the studies may contribute to the different outcomes observed.
Table 3. In vitro studies with human cell cultures showing direct effects of oligosaccharides on immunity

LPS, lipopolysaccharide; HMO, human milk oligosaccharides; PB, peripheral blood; HUVEC, human umbilical vein endothelial cells; QC, quantitative chromogenic; LAL, Limulus amoebocyte lysate; CBMC, cord blood mononuclear cells; IFN, interferon; Caco-2 cells, human epithelial colorectal adenocarcinoma cells; PGlyRP3, peptidoglycan recognition protein 3; DP, degree of polymerisation; PBMC, peripheral blood mononuclear cells; TLR, toll-like receptor; SC, short chain; LC, long chain; FOS, fructooligosaccharides; GOS, galactooligosaccharides; GMO, goat milk oligosaccharides; HT29 cells, human colorectal adenocarcinoma cells; MCP-1, monocyte chemoattractant protein-1; scGOS/lcFOS, short-chain galactooligosaccharides/long-chain fructooligosaccharides; MoDC, monocyte-derived dendritic cells; LAB, lactic acid bacteria; MIP-3α, macrophage inflammatory protein-3 α; H4 cells, human normal fetal intestinal epithelial cells; T84 cells, human metastatic colonic epithelial cells; NCM-460 cells, human normal colon mucosal epithelial cells; qPCR, quantitative PCR.
Table 4. In vitro studies with animal cell cultures showing direct effects of oligosaccharides on immunity
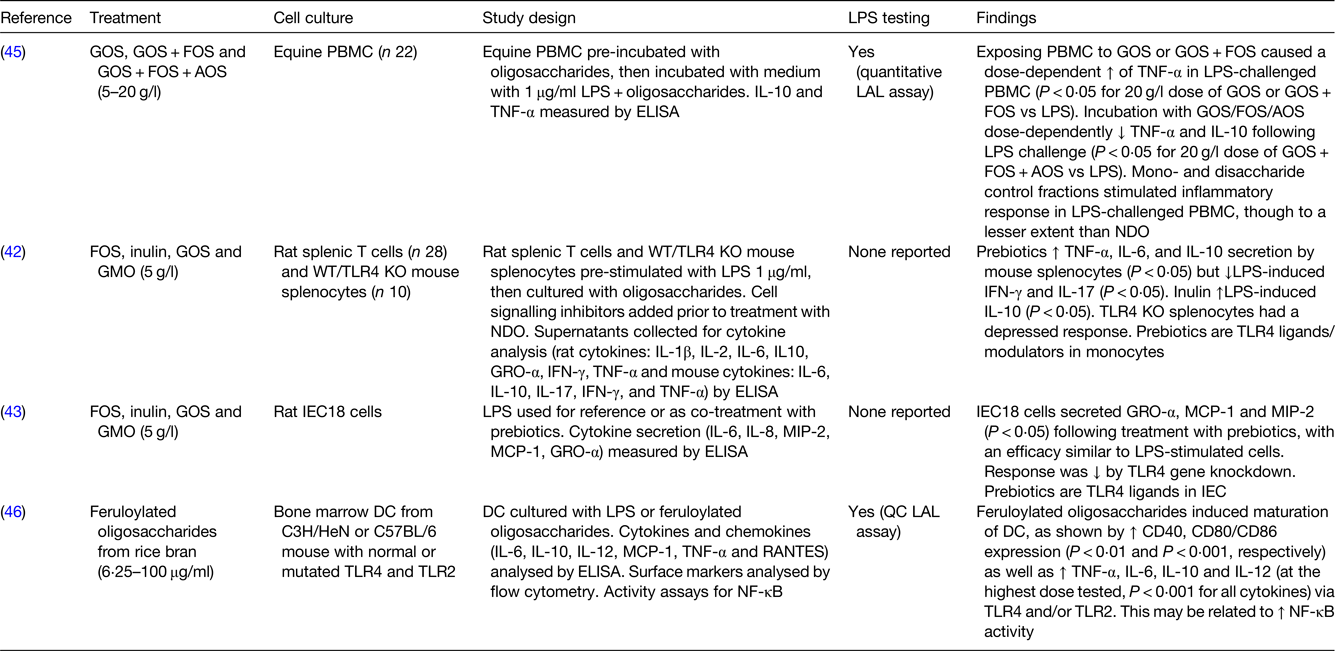
LPS, lipopolysaccharide; GOS, galactooligosaccharides; FOS, fructooligosaccharides; AOS, acidic oligosaccharides; PBMC, peripheral blood mononuclear cells; LAL, Limulus amoebocyte lysate; GMO, goat milk oligosaccharides; WT/TLR4 KO mice, wild-type/toll-like receptor 4 knockout mice; NDO, non-digestible oligosaccharides; GRO-α, growth-related oncogene-α; IFN, interferon; TLR, toll-like receptor; IEC18 cells, non-transformed rat small intestinal epithelial cells; MCP-1, monocyte chemoattractant protein-1; MIP-2, macrophage inflammatory protein-2; IEC, intestinal epithelial cells; DC, dendritic cells; RANTES, regulated on activation, normal T cell expressed and secreted; QC, quantitative chromogenic.
Table 5. In vivo study on germ-free mice showing direct effects of oligosaccharides on immunity

SC, short chain; LC, long chain; GF, germ free; MLN, mesenteric lymph nodes; PP, Peyer's patches; rRNA, ribosomal RNA; Th1, T helper 1; DC, dendritic cells.
Among the in vitro studies that used animal cell cultures, when GOS and GOS/FOS were tested at higher concentrations (5–20 g/l) on lipopolysaccharide (LPS)-challenged equine peripheral blood mononuclear cells, an increase in TNF-α was found. However, a mixture of GOS/FOS/arabinooligosaccharide showed a biphasic effect when used at different doses, with a reduction in TNF-α and IL-10 at higher concentrations and an increase in the same mediators at lower concentrations(Reference Vendrig, Coffeng and Fink-Gremmels45). Stimulation of rat splenic macrophages and T cells with FOS, inulin and GOS (all 5 g/l) increased the levels of TNF-α, IL-6 and IL-10 and reduced the levels of LPS-induced interferon-γ and IL-17, with effects that may be related to the modulation of toll-like receptor (TLR) 4 (Reference Capitan-Canadas, Ortega-Gonzalez and Guadix42). Research conducted on rat small intestinal cell lines (IEC18 cells) confirmed the hypothesis that FOS, GOS and GMO may act as TLR4 ligands. Indeed, there was a reduction in cytokine secretion (growth-regulated oncogene α, MCP-1 and macrophage inflammatory protein 2) seen after treatment with FOS, GOS and GMO (5 g/l) when the TLR4 gene was knocked down in IEC18 cells(Reference Ortega-Gonzalez, Ocon and Romero-Calvo43). Interaction between NDO and TLR4 was demonstrated not only in rat intestinal cells but also in mouse-derived bone marrow DC, whose maturation and cytokine secretion (TNF-α, IL-6, IL-10 and IL-12) was enhanced by treatment with feruloylated oligosaccharides from rice bran tested at different concentrations (6⋅25–100 μg/ml)(Reference Lin, Chen and Chen46).
One in vivo study on germ-free mice receiving short-chain or long-chain β2→1 fructans for 5 d showed that the immunomodulatory effects seen were partially mediated by the microbiota and partially microbiota-independent. Both short-chain and long-chain fructans increased the numbers of T helper cells in the Peyer's patches. Short-chain fructans increased regulatory T cells and CD11b− CD103− DC in the mesenteric lymph node and reduced their CD80 expression in the Peyer's patches, whereas long-chain fructans modulated the B-cell responses. These effects were independent of the gut microbiota and/or their SCFA production(Reference Fransen, Sahasrabudhe and Elderman47).
Research performed on human primary cells revealed that treatment of cord blood mononuclear cells with acidic HMO (1 μg/ml) led to an increase in interferon-γ- and IL-13-producing T helper and/or cytotoxic T cells and stimulated T helper cell maturation, as shown by higher expression of the activation marker CD25(Reference Eiwegger, Stahl and Schmitt38). Culture with FOS and inulin (5 g/l) increased IL-10 and TNF-α, but not IL-1β and IL-8, production by adult peripheral blood monocytes compared to control, and up-regulation in the response to LPS was seen when those cells were co-stimulated with NDO and LPS(Reference Capitan-Canadas, Ortega-Gonzalez and Guadix42). Higher levels of IL-10 were found after stimulation of human immature monocyte-derived DC from peripheral blood with 5 g/l of short-chain GOS/long-chain FOS, through a mechanism that involved NDO binding to TLR4(Reference Lehmann, Hiller and van Bergenhenegouwen44).
Overall, there is evidence to conclude that NDO can exert direct, microbiota-independent effects on immune cells. HMO, FOS, inulin and GOS were consistently shown to directly modulate cytokine production (IL-6, IL-8, IL-10, IL-12, MCP-1, macrophage inflammatory protein-3α and TNF-α) and immune cell maturation (lymphocytes, DC) in in vitro models, with mechanisms that seem to involve TLR ligation. One in vivo study on germ-free mice reinforced the in vitro evidence for microbiota-independent effects of NDO on immunity. However, not all NDO appear to have the same effect (anti-inflammatory v. pro-inflammatory), and within a class of NDO, inconsistent effects have been reported. Whereas HMO displayed clear anti-inflammatory properties in vitro, which might at least partially explain their protective effects against allergy and infection in vivo, FOS, inulin and GOS showed various outcomes on immunity, including anti-inflammatory as well as pro-inflammatory effects. The use of different doses and types of prebiotics as well as various cell culture models may explain the differences in cytokine production observed in vitro. Additionally, the chain length of oligosaccharides appears to have an important role in inducing either anti-inflammatory or pro-inflammatory responses. Vogt et al.(Reference Vogt, Ramasamy and Meyer41) demonstrated that short-chain FOS (2–5 units) elicited the production of anti-inflammatory cytokines to a greater extent than long-chain FOS (>8 units). Additionally, only half the studies assessed the LPS content of oligosaccharide fractions used in culture(Reference Eiwegger, Stahl and Haidl34, Reference Bode, Kunz and Muhly-Reinholz37, Reference Eiwegger, Stahl and Schmitt38, Reference Lehmann, Hiller and van Bergenhenegouwen44–Reference Lin, Chen and Chen46). Because the binding of LPS to TLR4 on monocytes, macrophages and B cells leads to strong, pro-inflammatory responses including the secretion of TNF-α, IL-1β, IL-6, IL-8, IL-10, IL-12, IL-15 and transforming growth factor(Reference Tucureanu, Rebleanu and Constantinescu48), it is important to determine whether the direct effects of oligosaccharides on cytokines are due to the action of the oligosaccharide fractions or to the presence of LPS within prepared fractions. Although dietary oligosaccharides bind and signal via TLR4 in monocytes, macrophages and intestinal epithelial cells(Reference Vogt, Ramasamy and Meyer41, Reference Ortega-Gonzalez, Ocon and Romero-Calvo43, Reference Lehmann, Hiller and van Bergenhenegouwen44, Reference Lin, Chen and Chen46) often eliciting a pro-inflammatory cytokine production in vitro, they are not necessarily pro-inflammatory in vivo. This is most likely due to differences between in vitro and in vivo conditions. Therefore, more research needs to be carried out to understand the mechanisms through which oligosaccharides affect immunity.
Effects of prebiotics on inflammatory bowel diseases: animal models and human clinical trials
IBD patients have lower numbers of bifidobacteria and lactobacilli in the gut, lower concentrations of faecal SCFA and high mucosal inflammation primarily induced by pro-inflammatory cytokines(Reference Scaldaferri, Gerardi and Lopetuso49). Prebiotics are promising in the management of IBD for their role in restoring gut microbiota homeostasis and affecting cytokine production and immune cell maturation. In recent years, animal and human studies on the role of prebiotics in IBD have been extensively reviewed(Reference Hedin, Whelan and Lindsay50–Reference Akram, Garud and Joshi53).
Murine experimental models of colitis associated either with epithelial barrier disruption or with immune cell defects have been developed to mimic IBD in human subjects(Reference Valatas, Bamias and Kolios54). Rodent experimental colitis models showed that both short-term (<1 week) and long-term (>1 month) treatment with prebiotics including lactulose, inulin and GMO reduced colonic damage and inflammation, whereas no convincing reduction of inflammation was seen in animal studies that used GOS or FOS(Reference Looijer-van Langen and Dieleman52). When GOS and FOS were used in association with other soluble and insoluble polysaccharides, a decrease in inflammatory cytokines as well as an increase in IL-10 and regulatory T cells in the mesenteric lymph nodes were observed(Reference Hartog, Belle and Bastiaans55). Together, these results indicate that various prebiotics may have different potential in attenuating inflammation, and that more studies using the experimental colitis model are needed(Reference Looijer-van Langen and Dieleman52).
Human clinical trials on prebiotic use in IBD are available for FOS and inulin (Table 6), but not for GOS and other NDO. In a double-blind placebo-controlled trial on individuals with chronic pouchitis (inflammation in the lining of an artificial rectum created after UC surgery), treatment with 24 g/d inulin for 3 weeks resulted in lower endoscopic and histological inflammation, higher faecal butyrate concentration and a tendency for lower concentrations of secondary bile acids in faeces(Reference Welters, Heineman and Thunnissen56). Similarly, patients with active UC receiving 12 g/d oligofructose-enriched inulin for 2 weeks displayed a reduction in disease activity and in faecal calprotectin (a marker of intestinal inflammation) but no changes in circulating inflammatory mediators (e.g. IL-8), compared to control(Reference Casellas, Borruel and Torrejon57). In another double-blind randomised control trial, supplementation with 10 g/d inulin for 4 weeks increased the numbers of bifidobacteria, led to higher concentrations of faecal acetaldehyde and butyrate and decreased disease activity in CD patients, although there was a higher dropout rate for those undergoing supplementation compared to placebo(Reference De Preter, Joossens and Ballet58). In an open-label trial, treatment with 15 g/d FOS for 3 weeks reduced disease activity in individuals with active ileo-colonic CD and increased the numbers of bifidobacteria as well as the numbers of DC from rectal biopsies expressing IL-10, TLR2 and TLR4(Reference Lindsay, Whelan and Stagg59). These results were only partially confirmed by a larger double-blind randomised control trial, where patients with active CD supplemented 15 g/d FOS for 4 weeks showed higher numbers of IL-10+ DC from rectal biopsies but no differences in disease activity, numbers of bifidobacteria and levels of faecal calprotectin(Reference Benjamin, Hedin and Koutsoumpas60). Several studies conducted on other non-prebiotic dietary fibres, such as ispaghula husk(Reference Hallert, Kaldma and Petersson61) and germinated barley foodstuff(Reference Kanauchi, Mitsuyama and Homma62–Reference Mitsuyama, Saiki and Kanauchi64), showed that also these two polysaccharides may have the potential in attenuating UC and/or CD clinical symptoms. Overall, while inulin appears promising in reducing IBD symptoms and inflammation, there are currently few studies of FOS and no studies of GOS in IBD. More research using standardised methods needs to be conducted to explore the potential preventive and/or therapeutic use of prebiotics in the management of IBD.
Table 6. Human studies of prebiotic use for the management of inflammatory bowel diseases

FOS, fructooligosaccharides; CD, Crohn's disease; TLR, toll-like receptor; DC, dendritic cells; UC, ulcerative colitis; PG-E2, prostaglandin E2.
Conclusions
Prebiotics have been extensively studied for their beneficial role in maintaining gut health and in supporting the growth of health-promoting bacteria. Additionally, prebiotics may positively modulate gut and systemic immunity via microbiota-dependent and microbiota-independent mechanisms, as reviewed in the present paper. Whereas the immunomodulatory effects of prebiotics via the production of SCFA have been extensively seen in the literature, fewer studies are available on direct prebiotic–immune cell interactions. In vitro, FOS, inulin and GOS were shown to exert microbiota-independent effects on immunity, including the binding to TLR on monocytes, macrophages and intestinal epithelial cells and consequent modulation of cytokines and immune cell maturation. In infants, there is good evidence to conclude that prebiotics can pass through the intestinal epithelium and directly modulate gut and systemic immune cells. Although it is logical to speculate that the same might happen in other conditions characterised by increased gut permeability, such as IBD, this hypothesis needs to be confirmed by further research. There is convincing preliminary data to suggest that inulin and lactulose can reduce IBD symptoms and inflammation in animal models and/or in human supplementation studies. However, the mechanisms of action of prebiotics in IBD are not fully understood, and there is a particular need for further research on a wider range of prebiotics, including FOS and GOS. The overall beneficial effects of prebiotics may be as a result of transfer across the permeable gut of IBD patients resulting in direct interaction with gut and systemic immune cells, or prebiotic actions may derive from microbiota-dependent mechanisms, or perhaps they might act through a combination of both these effects. An important first step will be to establish whether prebiotic oligosaccharides do in fact bypass the intestinal epithelium in IBD patients as has been established in infants and in vitro experiments.
Acknowledgements
S. D. F. wishes to thank the organising committee for the invitation to speak at The 13th European Nutrition Conference within the Postgraduate Symposium in October 2019, Dublin.
Financial Support
This review received no specific grant from any funding agency, commercial or not-for-profit sectors. S. D. F. PhD studentship is supported by Clasado Biosciences (grant number 17726/02).
Conflict of Interest
None.
Authorship
S. D. F. performed a review of the literature and drafted the manuscript. P. C. C. and C. E. C. critically revised and approved the final version of the manuscript.