Involuntary weight loss (WL) is a hallmark feature of cancer-associated malnutrition, the prevalence of which has frequently been shown to be one of the highest of all hospital patient groups(Reference Raja, Lim and Lim1–Reference Kruizenga, van Keeken and Weijs3). Several large scale studies over the past 40 years have reported that involuntary WL affects 50–80 % of patients with cancer with the degree of WL dependent on tumour site, type and stage of disease(Reference Bozzetti and Group4–Reference Ní Bhuachalla, Daly and Power7).
Malnutrition and involuntary WL at the time of diagnosis and deterioration of nutritional status during treatment, are associated with poor outcomes. A recent large international cohort of 8160 patients with cancer suggesting that WL of as little as 2⋅4 % predicts survival independent of disease, site, stage or performance score(Reference Martin, Senesse and Gioulbasanis6). In addition to the adverse impact on survival, WL has historically been associated with severe chemotherapy-related toxicity(Reference Andreyev, Norman and Oates8–Reference Cushen, Power and Teo12); and leads to a significant deterioration in a patients' performance status, psychological well-being and overall quality of life (QoL)(Reference Wheelwright, Darlington and Hopkinson13,Reference Daly, Dolan and Power14) .
Aetiology of malnutrition in cancer
The aetiology of malnutrition in cancer is multifactorial and includes the effect of nutrition impact symptoms (NIS) on oral intake, as well as complex metabolic alterations inherent in the disease process(Reference Argiles, Busquets and Stemmler15). The pathophysiology includes derangement of metabolic and hormonal processes due to inflammatory mediators produced by the tumour microenvironment, which can impair appetite and promote an inflammatory state associated with increased energy requirements and anabolic resistance(Reference Baracos, Martin and Korc16,Reference Baracos17) . Therefore, reduced dietary intake, increased requirements, altered substrate utilisation and anabolic resistance, combined with the reduced anabolic stimulus in the form of exercise, all contribute to malnutrition in cancer.
Nutrition impact symptoms
As well as abnormal metabolism of nutrients, patients with cancer often experience a reduction in oral intake and absorption due to nutrition impact symptoms such as anorexia, dysgeusia, nausea, constipation diarrhoea, dysphagia, malabsorption and early satiety. NIS are caused by both the disease itself and cancer treatments. Underlying causes range from the mass-effect of tumours (in the case of pain and dysphagia), as well as more complex, centrally mediated mechanisms such as attenuated orexigen production (caused by systemic inflammation), to iatrogenic conditions such as radiation enteritis(Reference Schiessel and Baracos18). NIS are strongly associated with malnutrition, specifically anorexia and WL(Reference Del Fabbro, Hui and Dalal19). Most NIS reported are of gastrointestinal origin, for example; nausea, vomiting, constipation, taste and smell changes, dumping syndrome and dysphagia. However, pain, fatigue, reduced functional capacity, financial concerns(Reference Nicolini, Ferrari and Masoni20–Reference Omlin, Blum and Wierecky22) and depression are also noted by many patients. These varying symptoms all have a profound impact on QoL(Reference Tong, Isenring and Yates23) and performance status(Reference Attar, Malka and Sabate24). The impact of NIS on performance status is of particular concern as reduced activity levels feed the cycle of cachexia. in that, reduced stimulus to the muscles can lead to muscle atrophy alongside the muscle wasting associated with a lack of substrate and anabolic resistance(Reference Antoun and Raynard25).
Metabolic derangements and increased energy expenditure
While reduced oral intake is a significant contributor to WL in cancer, a recent review showed that in studies where nutritional intake is controlled, WL persists in many patients(Reference Martin and Kubrak26), suggesting that factors such as hypermetabolism and anabolic resistance contribute to cancer-related WL(Reference Vazeille, Jouinot and Durand27). The presence of cancer in the body causes a variety of metabolic and endocrine changes (such as inflammation, anabolic resistance, proteolysis, lipolysis and futile cycling) induced by the tumour and activated immune cells. Complex interactions between inflammation (pro-inflammatory cytokines), neuro-hormonal changes and potential proteolytic and lipolytic factors produced by the host and the tumour, fuel WL and loss of lean mass(Reference Argiles, Busquets and Stemmler15). Hypermetabolism is also thought to be a significant contributor to energy deficits, with resultant WL. Depending on the tumour burden, and the level of anaerobic metabolism, an additional 418–5858 kJ (100–1400 kcals) can be required daily(Reference Friesen, Baracos and Tuszynski28). In addition, significantly increased production of acute-phase proteins and cytokines is an energy-intensive process(Reference Argiles, Busquets and Stemmler15) and receptors for many cytokines are expressed in the feeding centres of the hypothalamus, therefore inflammation-mediated changes in the hypothalamic-pituitary axis result in illness behaviour(Reference Baracos, Martin and Korc16), including aberrations in appetite signalling and inhibition of orexigens resulting in poor oral intake(Reference Grossberg, Scarlett and Marks29). Additional factors such as the browning of adipose tissue(Reference Chu, Bos and Gill30), changes in carbohydrate metabolism (Cori cycle upregulation), changes in fat metabolism (fatty acid cycling), increased insulin resistance(Reference Dev, Bruera and Dalal31) and the demand for amino acids to drive the inflammatory response, results in increased muscle proteolysis and reductions in lean mass, which affects both skeletal muscle and muscular organs, such as the heart(Reference Argiles, Busquets and Stemmler15). Furthermore, upregulation of the ubiquitin-proteasome pathway leads to increased muscle degradation(Reference Muscaritoli, Bossola, Doglietto, Mantovani, Anker, Inui, Morley, Fanelli, Scevola, Schuster and Yeh32).
Increase in sedentary behaviour
A doubly-labelled water study quantifying the physical activity level (PAL) of healthy adults found that the PAL of a sedentary adult is 1⋅4–1⋅5(Reference Black, Coward and Cole33). Compared to this, patients with cancer have been shown in a number of studies to be significantly more inactive that this, with Moses et al. reporting on twenty-four pancreatic cancer patients with cachexia, who had a mean PAL of 1⋅24(Reference Moses, Slater and Preston34) and Gibney et al. who found that lung cancer patients had a PAL of 1⋅36(Reference Gibney, Elia and Jebb35). These values correspond better with the severely disabled than any healthy, sedentary population. Community living spinal cord injury patients have been demonstrated to have a PAL of 1⋅32(Reference Mollinger, Spurr and el Ghatit36) and young patients with cerebral palsy a PAL of 1⋅23(Reference Stallings, Zemel and Davies37). These findings attest to the marked impact of advanced cancer and cachexia on the physical function and QoL of such patients. Levels of physical activity this low may exacerbate muscle wasting and it is well understood in any individual that a lack of physical activity will cause deconditioning and deterioration in muscle mass.
Weight loss and changes in body composition following a cancer diagnosis
The end results of the factors discussed earlier is involuntary WL which is a hallmark feature cancer-associated malnutrition. Often referred to as cancer cachexia, it is now accepted to be a multifactorial syndrome characterised predominantly by the ongoing loss of skeletal muscle mass (with or without loss of fat mass) that cannot be fully reversed by conventional nutrition support(Reference Fearon, Strasser and Anker38). Moderate-to-severe WL is present in 30–70 % of cancer patients(Reference Tangvik, Tell and Guttormsen2,Reference Bozzetti and Group4–Reference Ní Bhuachalla, Daly and Power7,Reference Ryan, Power and Daly39) . In the largest study to-date of 8160 patients with locally advanced or metastatic disease, 73 % experienced involuntary WL(Reference Martin, Senesse and Gioulbasanis6). Table 1 summarises the prevalence of >5 % WL (a key component of the diagnostic criteria of cancer cachexia(Reference Fearon, Strasser and Anker38)) according to tumour site in the scientific literature. WL has consistently been shown to be most frequent in patients with cancers in the upper gut and lung(Reference Bachmann, Heiligensetzer and Krakowski-Roosen40–Reference Nemer, Krishna and Shah44).
Table 1. Prevalence of patients with >5% weight loss according to primary tumour location in the scientific literature
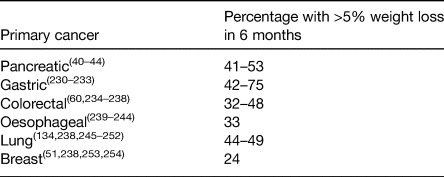
While involuntary WL is reported by the majority of patients with cancer, a significant proportion remain overweight or obese by international standards, thus appearing well-nourished(Reference Bozzetti45). Recent studies have reported that between 40 and 60 % of cancer patients are overweight or obese (BMI >25 kg/m2) even in the setting of metastatic disease(Reference Martin, Senesse and Gioulbasanis6,Reference Ní Bhuachalla, Daly and Power7,Reference Gioulbasanis, Martin and Baracos46–Reference Ramos Chaves, Boleo-Tome and Monteiro-Grillo48) . In a recent pooled analysis of twenty-two randomised trials that included 11 724 patients with cancer, 67 % were shown to be overweight or obese at the time of their cancer diagnosis(Reference Greenlee, Unger and LeBlanc49). As a result, many patients with cancer-related malnutrition are diagnosed with malnutrition late in the course of their disease as nutritional screening instruments such as the Malnutrition Universal Screening Tool, and others, are primarily based on BMI and do not identify these patients as malnourished until they have lost significant weight. Neither BMI nor percentage WL can capture changes in body composition and specifically changes in muscle mass(Reference Bozzetti45). Muscle loss is the most clinically relevant phenotypic feature of cancer cachexia and identifying those with muscle loss can become a huge challenge in overweight and obese patients(Reference Ryan, Power and Daly39). It is also important to note that although muscle loss is commonly associated with cancer, cancer is a disease associated with ageing, and therefore the aetiology of muscle loss in patients with cancer can be 2-fold, first resulting from the age-related decline in muscle mass and second due to cytokine-mediated degradation of muscle and adipose depots, hypermetabolism and anorexia associated with cancer cachexia(Reference Argiles, Busquets and Stemmler15). Distinguishing the exact cause of muscle loss can be difficult.
Lean mass
Computed tomography is now considered a gold standard method of body composition assessment and is of particular convenience in oncology research as these scans are readily available because they are used as part of routine medical care. Axial computed tomography images at the level of L3 are analysed to determine muscle mass, muscle radiodensity and adipose tissue mass (total, subcutaneous and visceral) and excellent inter-observer reliability has been shown(Reference Aubrey, Esfandiari and Baracos50). Regression formulae are available to estimate whole-body compartments using these data. Computed tomography allows the precise quantification of both muscle and adipose tissue and has led to a large volume of research which has increased our understanding of the importance of abnormal body composition phenotypes, such as low muscle mass (sarcopenia), and more recently low muscle attenuation (MA) as important prognostic indicators of unfavourable outcomes in patients with cancer(Reference Martin, Senesse and Gioulbasanis6,Reference Daly, Power and O'Reilly11,Reference Cespedes Feliciano, Kroenke and Bradshaw51,Reference van Vugt, Braam and van Oudheusden52) .
Sarcopenia
Sarcopenia is defined by The European Working Group on Sarcopenia in Older People as ‘a syndrome of progressive and generalised loss of skeletal muscle mass and strength with a risk of adverse outcomes such as physical disability, poor quality of life and death’(Reference Cruz-Jentoft, Baeyens and Bauer53,Reference Cruz-Jentoft, Bahat and Bauer54) . While muscle loss is a normal part of ageing, this syndrome can also occur in association with disease, such as cancer. A generally accepted cut-point is skeletal muscle mass 2 standard deviations below that of a healthy, young population(Reference Baumgartner, Koehler and Gallagher55).
Sarcopenia is now known to relate to asthenia, fatigue, impaired physical function, increased chemotherapy toxicity, impaired QoL and reduced survival(Reference Martin, Senesse and Gioulbasanis6,Reference Wheelwright, Darlington and Hopkinson13,Reference Bozzetti45,Reference Prado, Cushen and Orsso56) . Recent studies have shown that cancer, and its treatment, exacerbate muscle loss and that patients continually lose muscle mass while on treatment(Reference Daly, Power and O'Reilly11,Reference Daly, Ní Bhuachalla and Power57,Reference Rutten, van Dijk and Kruitwagen58) . While healthy adults over the age of 40 years have been shown to lose muscle at a rate of 1–1⋅4 %/year(Reference Frontera, Zayas and Rodriguez59), cancer patients have been shown to have a 24-fold higher rate of muscle loss than that observed in healthy ageing adults(Reference Daly, Ní Bhuachalla and Power57,Reference Blauwhoff-Buskermolen, Versteeg and de van der Schueren60) . In studies examining the rate of muscle loss in cancer patients, rates of 3⋅9 %/100 days have been reported in foregut cancer(Reference Daly, Ní Bhuachalla and Power57), 3⋅1 %/100 days in pancreatic cancer(Reference Tan, Birdsell and Martin61) 3⋅3 %/100 days in metastatic melanoma(Reference Daly, Power and O'Reilly11) and 5⋅2 %/100 days in ovarian cancer(Reference Rutten, van Dijk and Kruitwagen58).
Prevalence of cancer cachexia and sarcopenia in oncology
The prevalence of cancer cachexia and sarcopenia can vary widely depending on the method of assessment and diagnostic criteria used(Reference Daly, Prado and Ryan62). From the literature, it can be estimated that the prevalence of cancer cachexia (based on WL >5 % as per the recent consensus definition(Reference Fearon, Strasser and Anker38)) can vary between 13 and 61 % depending on the tumour site (Table 1), and between 38 and 70 % of patients are considered to have sarcopenia (based on three of the most commonly used diagnostic criteria) (see Fig. 1). The prevalence of sarcopenia is highest in the lung (median 70 %, range 47–79 %)(Reference Baracos, Reiman and Mourtzakis63–Reference Kim, Kim and Park66) and pancreatic cancer (median 56 %, range 44–89 %)(Reference Wesseltoft-Rao, Hjermstad and Ikdahl41,Reference Tan, Birdsell and Martin61,Reference Sandini, Patino and Ferrone67–Reference Carrara, Pecorelli and De Cobelli73) however, it is noteworthy that the majority of studies report a prevalence of above 40 % at most other sites in the body.

Fig. 1. Prevalence of sarcopenia in patients with cancer according to the primary tumour location in the literature (all stages).(Reference Baracos, Reiman and Mourtzakis63–Reference El Amrani, Vermersch and Fulbert68,Reference Fattouh, Chang and Ow136,Reference Wesseltoft-Rao, Hjermstad and Ikdahl41,Reference Tan, Birdsell and Martin61,Reference Di Sebastiano, Yang and Zbuk69–Reference Carrara, Pecorelli and De Cobelli73,Reference Mir, Coriat and Blanchet111,Reference Elliott, Doyle and Murphy180,Reference Harimoto, Shirabe and Yamashita256–Reference Black, Mackay and Ramsay260,Reference Tan, Brammer and Randhawa90,Reference Yip, Goh and Davies94,Reference Anandavadivelan, Brismar and Nilsson261–Reference Awad, Tan and Cui264,Reference Cushen, Power and Teo12,Reference Antoun, Lanoy and Albiges-Sauvin113,Reference Paireder, Asari and Kristo265–Reference Psutka, Boorjian and Moynagh270,Reference Auclin, Bourillon and De Maio95,Reference Huillard, Mir and Peyromaure271–Reference Mason, Boorjian and Bhindi275,Reference van Vugt, Braam and van Oudheusden52,Reference Blauwhoff-Buskermolen, Versteeg and de van der Schueren60,Reference Camus, Lanic and Kraut149,Reference Lanic, Kraut-Tauzia and Modzelewski150,Reference Xiao, Luo and O'Brian276,Reference Thoresen, Frykholm and Lydersen277,Reference Lieffers, Bathe and Fassbender185,Reference Black, Mackay and Ramsay260,Reference van Roekel, Bours and Te Molder278–Reference Chemama, Bayar and Lanoy280,Reference Kurk, Peeters and Dorresteijn86–Reference van der Kroft, Bours and Janssen-Heijnen88,Reference McSorley, Black and Horgan281,Reference Reisinger, van Vugt and Tegels282,Reference Broughman, Williams and Deal283–Reference Barret, Antoun and Dalban287,Reference Palmela, Velho and Agostinho91,Reference Jarvinen, Ilonen and Kauppi288–Reference Psutka, Carrasco and Schmit293,Reference Daly, Power and O'Reilly11,Reference Martin, Birdsell and Macdonald47,Reference Daly, Ní Bhuachalla and Power57,Reference Rutten, van Dijk and Kruitwagen58,Reference Prado, Lieffers and McCargar92,Reference Ataseven, Luengo and du Bois162,Reference Hayashi, Ando and Gyawali164,Reference Nishigori, Okabe and Tanaka268,Reference Kumar, Moynagh and Multinu294,Reference Valentine, François and Nora295,Reference Ní Bhuachalla, Daly and Power7,Reference Shachar, Deal and Weinberg93,Reference Veasey Rodrigues, Baracos and Wheler96,Reference Prado, Baracos and McCargar100,Reference Rier, Jager and Sleijfer165,Reference Del Fabbro, Parsons and Warneke296–Reference Deluche, Leobon and Desport299) .
Prevalence of sarcopenia defined using three of the most common definitions for defining low muscle mass is displayed in Table 1. These definitions are as follows; Prado et al.(Reference Prado, Lieffers and McCargar92): Skeletal muscle index (SMI)<52⋅4 cm2/m2 in men and <38⋅5 cm2/m2 in women; Martin et al.(Reference Martin, Birdsell and Macdonald47): SMI <43⋅0 cm2/m2 in men with a BMI <25 kg/m2 and <53⋅0 cm2/m2 in men with a BMI >25 kg/m2 and SMI <41⋅0 cm2/m2 in women; Baumgartner et al.(Reference Baumgartner, Koehler and Gallagher55) converted dual-energy X-ray absorptiometry cut points by Mourtzakis et al.(Reference Mourtzakis, Prado and Lieffers300) as SMI <55 4 cm2/m2 in men and <38⋅9 cm2/m2 in women.
Our group recently estimated the incidence and prevalence of cachexia and sarcopenia in the UK and Ireland(Reference Sullivan, Daly and Power74). We estimated that across the Republic of Ireland and UK at least 128 892 cancer patients are affected by WL >5 % annually (34 %) and that there are 716 124 cancer survivors who have suffered >5 % WL at some point in their disease trajectory. Furthermore, we estimated that there are at least 133 707 annual cases of cancer patients with sarcopenia (35 %) and 771 589 cancer survivors alive who have been affected by sarcopenia during their disease trajectory.
The rates of muscle wasting seen in cancer populations are of huge public health importance, given that cancer cachexia and sarcopenia have been reported to be unequivocally associated with negative clinical outcomes in patients with cancer including poorer tolerance to anti-cancer treatment, poorer overall QoL, increased risk of post-operative complications and poorer overall survival(Reference Martin, Senesse and Gioulbasanis6,Reference Wheelwright, Darlington and Hopkinson13,Reference Prado, Cushen and Orsso56,Reference Daly, Prado and Ryan62) .
Skeletal degradation in cancer treatment
As well as the ongoing loss of muscle mass, several anti-cancer therapies (both hormonal and non-hormonal) promote bone loss through direct dysregulation of bone turnover and indirectly through hypogonadism and nephrotoxicity. The rate of bone loss from cancer therapy can be ten times higher than in the general population(Reference Sturgeon, Mathis and Rogers75–Reference Maillefert, Sibilia and Michel79) but is highest in breast and prostate cancer(Reference Sturgeon, Mathis and Rogers75) due to commonly administered therapies such as endocrine therapy (breast cancer) and androgen deprivation therapy (prostate cancer). Chemotherapy drugs (cisplatin, doxorubicin, cyclophosphamide, ifosfamide, FOLFIRI, carboplatin, methotrexate and targeted therapies) cause reduced bone volume and radiation therapy, orchiectomy and oophorectomy also result in bone loss. The onset of bone loss from premature menopause is sudden (within 6 months of treatment) and significant (21 % decreased density v. age-matched menstruating women(Reference Cann, Martin and Genant80)). For men with prostate cancer on androgen deprivation therapy loss of bone starts within 6–9 months with annual declines of between 2 and 8 %(Reference Berruti, Dogliotti and Terrone81–Reference Diamond, Higano and Smith83). Reduction in bone quality is also further exacerbated by inactivity. Muscle weakness and exercise intolerance can persist from months to years after remission(Reference Winters-Stone, Bennett and Nail84,Reference Nicolson and Conklin85) . Excess bone resorption can lead to fractures and spinal cord compression(Reference Sturgeon, Mathis and Rogers75).
Why malnutrition matters: impact on tolerance to systemic chemotherapy
Chemotherapy can often be associated with severe toxicity that can result in dose delays, dose reductions and treatment termination, referred to as dose limiting toxicities. Moderate to severe toxicities can lead to interruption, deferral or even cessation of treatment. Severe toxic events can result in hospitalisations and can even be life-threatening. Recent evidence suggest that variability in body composition of cancer patients may be a source of disparities in the metabolism of cytotoxic agents resulting in increased toxicity(Reference Kurk, Peeters and Dorresteijn86–Reference van der Kroft, Bours and Janssen-Heijnen88).
To date, in excess of forty studies have examined the relationship between low lean mass (sarcopenia) and the prevalence of dose limiting toxicity in patients with cancer (we have previously reviewed these(Reference Ryan, Prado and Sullivan89)). The relationship between sarcopenia and increased chemotherapy toxicity has been reported in both early and late-stage disease, at almost all cancer sites and with many modalities of cytotoxic agents (cytotoxic single agents, regimens, targeted agents and immunotherapies)(Reference Tan, Brammer and Randhawa90–Reference Shachar, Deal and Weinberg93). Although the relationship between low lean mass and poorer tolerance to treatment has been observed in the majority of studies, a few smaller studies have reported no association(Reference Blauwhoff-Buskermolen, Versteeg and de van der Schueren60,Reference Rollins, Tewari and Ackner71,Reference Yip, Goh and Davies94–Reference Versteeg, Blauwhoff-Buskermolen and Buffart99) .
Low lean mass can lead to increased toxic side effects to chemotherapy through alterations in the distribution, metabolism and clearance of chemotherapy drugs(Reference Prado, Baracos and McCargar100). The widespread use of body surface area (BSA), relying on height and weight alone(Reference Du Bois and Du Bois101), in dosing chemotherapy drugs presents a problem as there are large discrepancies in muscle mass between people of the same BSA, resulting in potential under or over-dosing when calculations are based on a simple BSA formula(Reference Stobäus, Küpferling and Lorenz102–Reference Prado, Antoun and Sawyer104). A 4–10-fold variation in drug clearance is possible in individuals with a similar BSA and there is concern that this approach to dosing is invalid(Reference Takimoto105,Reference Baker, Verweij and Rowinsky106) . Bodyweight comprises two major components (lean and fat mass) then these are the two major sites of distribution of hydrophilic and lipophilic drugs(Reference Prado, Baracos and McCargar107,Reference Prado, Baracos and Xiao108) . Therefore, variability in individual lean mass or fat mass may lead to changes in the volume of distribution of drugs and therefore adversely affect the tolerance of cytotoxic drugs(Reference Daly, Prado and Ryan62). In sarcopenic obesity, tolerance is further compromised in individuals where the combination of excessive fat mass and low lean mass may significantly impact the tolerance of hydrophilic drugs by resulting in a disproportionally small volume of drug distribution in relation to their body weight or BSA(Reference Prado, Baracos and McCargar100,Reference Prado, Baracos and McCargar107) . Variations in lean and fat mass can therefore lead to considerable variation in the milligram of chemotherapy drug per kilogram lean mass with higher doses per kilogram lean mass shown to be associated with more frequent and severe toxic side effects(Reference Prado, Baracos and McCargar107,Reference Sjøblom, Benth and Grønberg109,Reference Sjøblom, Grønberg and Benth110) . This hypothesis is supported by pharmacokinetic data, with sarcopenic patients experiencing higher plasma concentrations of antineoplastic drugs and experiencing more toxicity(Reference Mir, Coriat and Blanchet111,Reference Massicotte, Borget and Broutin112) . For lipophilic drugs such as doxorubicin or trabectedin, individuals with a low-fat mass may also present with toxicity due to a reduced volume of distribution(Reference Prado, Baracos and Xiao108).
It is also important to note that sarcopenic patients are excessively fragile and highly susceptible to acute medical events that exacerbate chemotherapy-related toxicity(Reference Antoun, Lanoy and Albiges-Sauvin113). In addition, for those patients with systemic inflammation, this has been shown to decrease liver cytochrome activities and drug clearance and may modify drug exposure. Low concentrations of circulation plasma proteins (e.g. albumin), which is commonly seen in those with malnutrition or systemic inflammation (or both) may also affect the distribution of highly protein-bound drugs such as vandetanib, sorafenib and epirubicin(Reference Prado, Baracos and Xiao108,Reference Mir, Coriat and Blanchet111,Reference Massicotte, Borget and Broutin112) . As imaging techniques in body composition become more widely used, this may represent an opportunity for a more personalised approach to chemotherapy dosing.
Why malnutrition matters: impact on performance status and quality of life
The adverse impact of WL on QoL has long been recognised in patients with cancer and WL has been associated with deterioration in patients' performance status and psychosocial well-being(Reference Bachmann, Heiligensetzer and Krakowski-Roosen40,Reference Blum, Omlin and Baracos114,Reference Ravasco, Monteiro-Grillo and Vidal115) . In a recent systematic review examining the impact of WL and QoL, a negative relationship between %WL and QoL was reported in twenty-three of twenty-seven studies included in the analysis(Reference Wheelwright, Darlington and Hopkinson13). However, the mode by which WL exerts its influence on QoL is not fully understood but may relate to muscle atrophy associated with cachexia and WL leading to fatigue or reduced functional capacity(Reference Fearon, Voss and Hustead116). The negative impact on QoL is unsurprising, considering cancer-related malnutrition is a major cause of fatigue(Reference Evans and Lambert117,Reference Ryan, Carroll and Ryan118) , reduced functional ability(Reference Fearon, Voss and Hustead116) and a source of emotional distress(Reference Evans and Lambert117,Reference Hopkinson, Brown and Okamoto119) . Our group recently reported on a cohort of 1027 patients with advanced cancer and showed that WL >10 % was associated with poorer QoL in almost all functional and symptom domains(Reference Daly, Dolan and Power14). In particular, WL in excess of 10 % in the preceding 3 months was independently associated with poorer physical function, fatigue and appetite loss and overall poorer QoL summary scores.
While there is no doubt that WL impacts negatively on QoL, inconsistent reports on the relationship between muscle parameters and QoL have been published in the literature(Reference Parsons, Tsimberidou and Pontikos97,Reference Neefjes, van den Hurk and Blauwhoff-Buskermolen120–Reference Bye, Sjøblom and Wentzel-Larsen122) . Parsons and colleagues reported no significant associations between low Skeletal muscle index and symptom burden or functional life domains assessed by the MD Anderson Symptom Inventory, in a cohort of 104 patients with advanced cancer(Reference Parsons, Tsimberidou and Pontikos97). However, in a study of 734 advanced lung cancer patients, low Skeletal muscle index was non-linearly associated with lower global QoL, physical function and role function, and associated with more symptoms (fatigue and pain), while low MA was associated with poor physical function and more dyspnoea(Reference Bye, Sjøblom and Wentzel-Larsen122). Sarcopenia has also been associated with greater depression symptoms and more fatigue in patients with advanced cancer(Reference Neefjes, van den Hurk and Blauwhoff-Buskermolen120,Reference Nipp, Fuchs and El-Jawahri121) . It may be that low Skeletal muscle index, at a single time point, is not reflective of a dynamic measure of loss and may be influenced by a patient's intrinsic level of muscularity. Perhaps the loss of muscle over time may better reflect poor QoL and further research is needed in this area.
The mode by which WL exerts its influence on QoL is not fully understood but may relate to muscle atrophy associated with cachexia and WL leading to fatigue or reduced functional capacity. Recent work has suggested that the complex interplay between metabolic disruption and pro-inflammatory cytokines (i.e. IL-6, IL-8 and TNF-α) in cancer cachexia often leads to physical, biochemical and nutritional deterioration which subsequently leads to poor QoL(Reference Aapro, Arends and Bozzetti123). It is thought that the systemic inflammatory response has a direct role in the development of cancer-associated symptom clusters, including pain, fatigue, mood, anorexia and physical function(Reference McSorley, Dolan and Roxburgh124). Systemic inflammation and loss of lean mass are also thought to drive cancer-related fatigue, which is thought to affect up to 80 % of cancer patients(Reference Baguley, Skinner and Wright125) both during and after treatment cessation(Reference Baguley, Skinner and Wright125–Reference Wang, Zhao and Fisch128). Severe and persistent fatigue, along with muscle mass wasting has been shown to inhibit QoL by considerably reducing functional capacity to fully participate in daily living tasks(Reference Baguley, Skinner and Wright125). Individual proinflammatory cytokines have been associated with clinical symptoms, e.g., IL-6 and C-reactive protein with anorexia(Reference Paulsen, Laird and Aass129), IL-1ra with fatigue(Reference Paulsen, Laird and Aass129) and IL-6 with major depression(Reference Du, Zhang and Li130,Reference Breitbart, Rosenfeld and Tobias131) . Our group recently reported that systemic inflammation has a negative impact on QoL that is independent of Eastern Cooperative Oncology Group performance status(Reference Daly, Dolan and Power14) which is consistent with previous reports indicating that the systemic inflammatory response is associated with poorer QoL, even in those with a good performance score(Reference Laird, Fallon and Hjermstad132).
Importantly, interventions aimed at targeting nutritional status and attenuating weight have proven successful in improving aspects QoL in patients with cancer(Reference Baldwin, Spiro and Ahern133). In addition, novel cachexia treatments, such as Anamorelin, an oral ghrelin-receptor agonist with appetite enhancing and anabolic activity have shown a favourable clinical response in alleviating anorexia-cachexia symptoms(Reference Temel, Abernethy and Currow134). Research is warranted to determine if attenuating the systemic inflammatory response is capable of producing clinically relevant improvements in symptoms that may represent a new therapeutic approach to symptom management in patients with advanced cancer.
Impact on survival
The impact of sarcopenia on survival in cancer has been extensively studied over the past decade. Most studies report a significant decrease in overall survival in patients with sarcopenia compared with those without sarcopenia, irrespective of the primary cancer site and stage (see Fig. 2). Figure 1 displays a forest plot depicting the summary results of meta-analyses examining the role of sarcopenia in survival in cancer. To-date sarcopenia (diagnosed by computed tomography) has been shown to be independently associated with poorer survival in all those sites included in the meta-analysis in Fig. 2 as well as in head and neck(Reference Cho, Kim and Keum135–Reference Stone, Olson and Mowery140), prostate cancer(Reference Ohtaka, Aoki and Nagata141), cholangiocarcinoma(Reference Coelen, Wiggers and Nio142–Reference Okumura, Kaido and Hamaguchi146), lymphoma(Reference Go, Park and Kang147–Reference Nakamura, Hara and Shibata151) and leukaemia(Reference Nakamura, Ninomiya and Matsumoto152). In a recent systematic review and meta-analysis of thirty-eight studies that included 7843 patients with solid tumours, low muscle cross-sectional area was observed in 27⋅7 % of patients with cancer and associated with poorer overall survival (hazard ratio (HR) 1⋅44, 95 % CI 1⋅32, 1⋅56), cancer-specific survival (HR 1⋅93, 95 % CI 1⋅38, 2⋅70), as well as disease-free survival (HR 1⋅16, 95 % CI 1⋅00, 1⋅30) but not with progression-free survival (HR 1⋅54, 95 % CI 0⋅90, 2⋅64)(Reference Shachar, Williams and Muss153). This meta-analysis demonstrated that the adverse effects of low lean mass on overall survival were similar in both metastatic (HR 1⋅37, 95 % CI 1⋅21, 1⋅56) and non-metastatic disease (HR 1⋅54, 95 % CI 1⋅31, 1⋅79), and this relationship was observed across different primary tumour sites. Recently, in two of the largest observational cohort studies to date, Caan and colleagues(Reference Caan, Meyerhardt and Kroenke154,Reference Caan, Cespedes Feliciano and Prado155) demonstrated the prognostic value of low muscle mass in non-metastatic breast (n 3241) and colorectal cancer (n 3262). Low lean mass was present in 34 and 42 % of patients, respectively, and was independently associated with a 27–41 % higher risk of overall mortality (colon: HR 1⋅24, (95 % CI 1⋅09, 1⋅48); Breast: HR 1⋅41 (95 % CI 1⋅18, 1⋅69)](Reference Caan, Meyerhardt and Kroenke154,Reference Caan, Cespedes Feliciano and Prado155) .
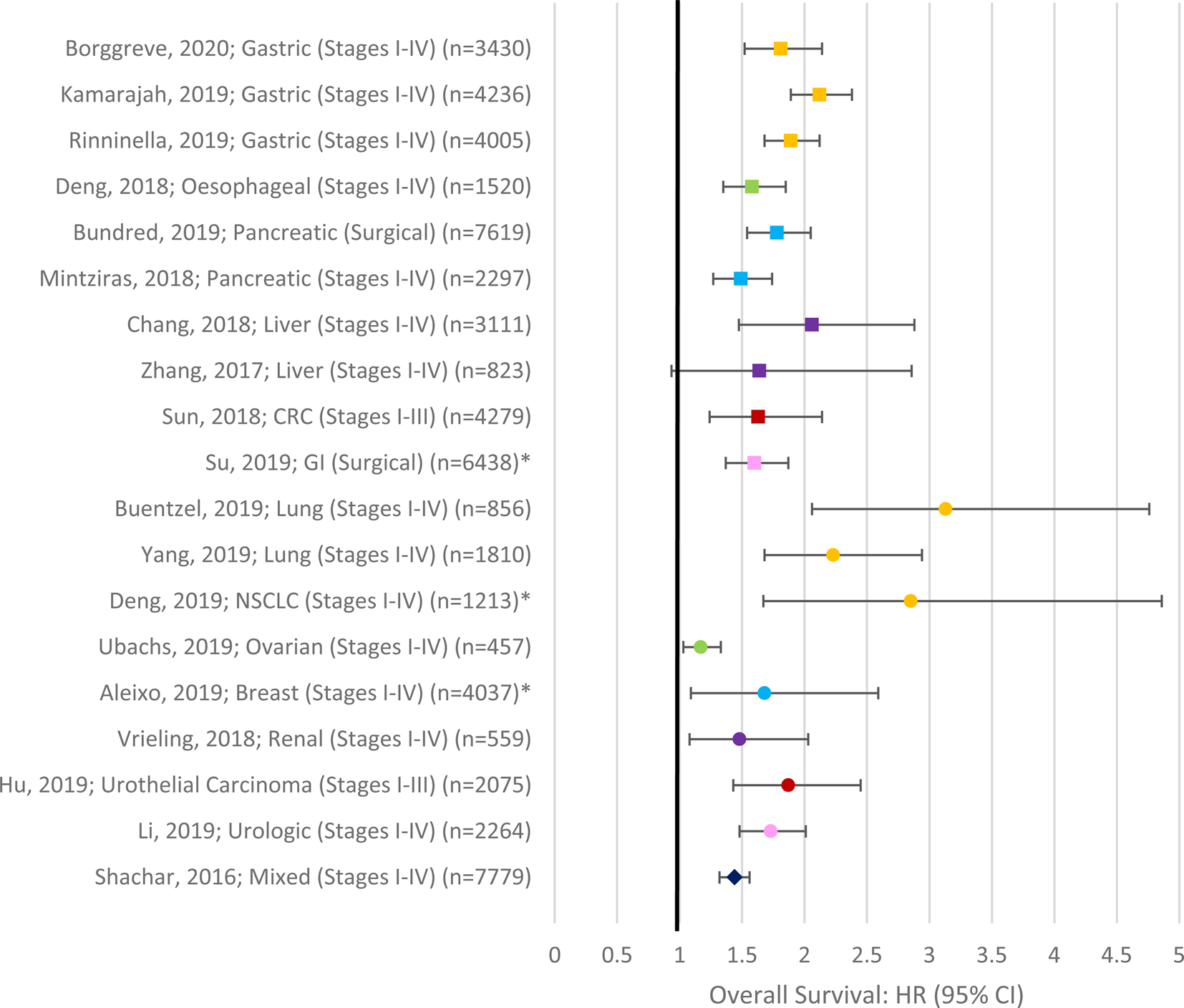
Fig. 2. (Colour online) Forest Plot depicting summary results of meta-analyses examining the role of sarcopenia in survival in cancer. Asterisks denote studies which did not confirm the inclusion of multivariate data in the meta-analysis(Reference Shachar, Williams and Muss153,Reference Hu, Dou and Shao301–Reference Zhang, Meng and Li304,Reference Yang, Shen and Tan305–Reference Aleixo, Williams and Nyrop310,Reference Mintziras, Miligkos and Wachter170,Reference Bundred, Kamarajah and Roberts311–Reference Borggreve, den Boer and van Boxel315) .
In addition to low muscle area (sarcopenia), low MA (density; indicative of fatty infiltration of muscle tissue) is also associated with poorer survival in a variety of tumours including non-small cell lung cancer(Reference Sjøblom, Grønberg and Wentzel-Larsen156), colorectal(Reference Kroenke, Prado and Meyerhardt157–Reference van Baar, Beijer and Bours159), endometrial(Reference de Paula, Rodrigues and Chaves160), renal(Reference Antoun, Lanoy and Iacovelli161), ovarian cancer(Reference Ataseven, Luengo and du Bois162) and melanoma(Reference Sabel, Lee and Cai163). Importantly, in some cases, low MA appears to superior in predicting mortality compared with low lean mass alone(Reference Sjøblom, Grønberg and Wentzel-Larsen156,Reference Hayashi, Ando and Gyawali164–Reference Van Rijssen, van Huijgevoort and Coelen167) . However, it has also been demonstrated that the risk of mortality associated with low lean mass and low MA can be independent of each other(Reference Okumura, Kaido and Hamaguchi146,Reference Charette, Vandeputte and Ameye168,Reference Sueda, Takahasi and Nishimura169) .
Lastly, the combination of sarcopenia and obesity has been shown to have particularly poor clinical outcomes. This may be related to the combined negative effects of both conditions or may be related to poor detection of sarcopenia in a cohort whose muscle loss is masked by excess adiposity. Sarcopenic obesity specifically has been associated with poorer survival in a number of cohorts(Reference Prado, Lieffers and McCargar92,Reference Mintziras, Miligkos and Wachter170) .
Why malnutrition matters: impact of muscle loss during treatment and survival
Notwithstanding the impact of sarcopenia on survival, several studies have emphasised that patients continually lose muscle mass while on treatment and that this is associated with an increased risk of mortality in a number of cancers. Patients with advanced pancreatic cancer (n 97) who experienced the early loss of skeletal muscle (>10 % within 3 months of diagnosis) were at increased risk of poorer overall survival and progression-free survival compared to patients who did not experience muscle loss to the same degree (HR 2⋅16 (95 % CI 1⋅23, 3⋅78), P = 0⋅007 and HR 2⋅31 (95 % CI 1⋅30, 4⋅09), P = 0⋅004)(Reference Basile, Parnofiello and Vitale171). In patients with surgically resected stage I-III colorectal cancer (n 1924), those who experienced the largest decrease in muscle mass (≥2 standard deviations or the equivalent to ≥11⋅4 % loss) and the largest decline in mean MA (≥2 sd; ≥20⋅2 % loss) from baseline were at a significantly increased risk of mortality (HR 2⋅15, 95 % CI 1⋅59, 2⋅92), P < 0⋅001 and HR 1⋅61 95 % CI 1⋅20, 2⋅15, P = 0⋅002, respectively), and these findings were independent of changes in body mass or other body composition parameters(Reference Brown, Caan and Meyerhardt158). To date, losses in muscle have been shown to be prognostic of reduced survival in pancreatic(Reference Dalal, Hui and Bidaut72,Reference Basile, Parnofiello and Vitale171) , oesophageal(Reference Yoon, Oh and Ahn172), gastric(Reference Park, Kim and Beom173), lung(Reference Nattenmuller, Wochner and Muley174), colorectal(Reference Blauwhoff-Buskermolen, Versteeg and de van der Schueren60,Reference Takeda, Akiyoshi and Matsueda175,Reference Miyamoto, Baba and Sakamoto176) , ovarian(Reference Rutten, van Dijk and Kruitwagen58), melanoma(Reference Daly, Power and O'Reilly11) and foregut cancers(Reference Daly, Ní Bhuachalla and Power57).
Why malnutrition matters: impact on surgical outcomes
In cancer patients undergoing surgery, length of stay and post-operative complications are important indicators of surgical morbidity. Sarcopenia has been independently associated with adverse post-operative outcomes including infections, complications, the longer length of hospital stay and risk of readmission following gastrectomy(Reference Lou, Chi and Chen177,Reference Zhang, Wang and Wang178) , pancreatectomy(Reference Cao, Xiong and Zhong179), oesophagectomy(Reference Elliott, Doyle and Murphy180,Reference Ida, Watanabe and Yoshida181) , cystectomy(Reference Mao, Ma and Wang182)pneumonectomy(Reference Madariaga, Troschel Cand Med and Best Cand Med183,Reference Kawaguchi, Hanaoka and Ohshio184) and colectomy(Reference Lieffers, Bathe and Fassbender185–Reference Zhang, Tan and Jiang187). Even in those without complications, length of stay has been shown to be significantly longer in patients with muscle abnormalities(Reference Martin, Hopkins and Malietzis188–Reference Gani, Buettner and Margonis190).
Potential therapies for malnutrition in cancer
Despite much evidence that impaired nutritional status is associated with poor outcomes, the evidence-base regarding the optimal management of malnutrition in cancer and the ability to improve nutritional status to improve clinical outcomes is lacking(Reference Arends, Bachmann and Baracos191). While the treatment of malignancy is the primary method of reversing the metabolic environment which perpetuates cachexia, supportive care is required while this process ensues. In the early stages of cancer cachexia, malnutrition may be reversible; however, in later stages of the disease, it has been difficult to attain significant improvements in nutritional status, although it has been suggested that with the right combination of therapies, even patients with advanced disease may exhibit anabolic potential.
Dietetic management
Nutritional interventions have been a mainstay of cachexia management to date. Nutritional counselling, consisting of dietary advice and ongoing education is the first line for treatment of malnutrition(Reference Arends, Bachmann and Baracos191). While a food-first approach to a high-protein, high-energy diet is recommended, nutrition support, starting with the use of oral nutritional supplements is frequently required to augment volitional intake where appetite is limited. Furthermore, artificial nutrition in the form of enteral or parenteral nutrition may be required due to dysphagia, obstruction of the gastrointestinal tract or severe malabsorption(Reference Arends192). The European Society for Clinical Nutrition and Metabolism (ESPEN) released consensus guidelines in 2017 for the nutritional management of cancer patients(Reference Arends, Bachmann and Baracos191). Despite limited evidence that nutritional interventions improve clinical outcomes, ESPEN strongly recommends, with moderate evidence, that nutritional interventions be employed in those at risk of malnutrition, aiming to increase oral intake, by providing dietary advice, management of metabolic derangements and nutrition impact symptoms, as well as the provision of oral nutritional supplementation where needed. ESPEN recommend that patients' total energy expenditure be assumed as 105–126 kJ/kg (25–30 kcal/kg) body weight daily, unless the direct measurement is available. Given that cancer patients can be hypo-, normo- or hypermetabolic, and displaying varying levels of anabolic resistance, it seems reasonable, in the absence of direct measurements, to take a pragmatic approach, and adjust requirements according to clinical response to the initial estimation.
Apart from energy requirements, meeting protein needs is also a priority in order to maintain lean mass and support recovery throughout the cancer journey. ESPEN recommend 1–1⋅5 g protein/kg body weight in cancer patients but suggest that research is necessary to determine whether a higher level such as 2 g/kg may be beneficial(Reference Arends, Bachmann and Baracos191). PRIMe (ClinicalTrials.gov ID: NCT 02788955) is a feasibility study (n 40) comparing isoenergetic diets in colorectal cancer with either 1 g protein/kg body weight or 2 g protein/kg body weight and assessing the impact of the varying protein intakes on muscle mass and physical functioning.
A number of individual studies have demonstrated positive impacts of nutritional interventions on relevant outcomes. A number of studies have demonstrated that dietitian-led clinics and intensive dietary counselling can reduce nutrition-related admissions(Reference Kiss, Krishnasamy and Loeliger193,Reference Hall, Englehart and Blaseg194) and reduce the length of stay(Reference De Waele, Mattens and Honore195). Improved energy and protein intake(Reference Uster, Ruefenacht and Ruehlin196,Reference Poulsen, Pedersen and Osterlind197) and weight(Reference Isenring, Capra and Bauer198,Reference Isenring, Capra and Bauer199) were noted in some studies and these increases led to improved QoL, functioning and nutritional status(Reference Ravasco, Monteiro-Grillo and Vidal200).
A recent national survey led by our research group in Ireland examined the attitudes and experiences of patients with cancer (n 1085) to nutrition(Reference Sullivan, Rice and Kingston201). Overall 45 % reported problems with diet and eating, 44 % had experienced involuntary WL (mean loss reported 10⋅4 kg, range 1–44 kg) and 52 % reported they had noticed muscle loss. The majority (67 %) wanted more information on diet with 51 % reported they were concerned about their nutritional status and confused by what to eat. Worryingly one in three with involuntary WL had not been able to access a registered dietitian (RD) for individual advice. Despite ESPEN recommendation that all patients receive routine nutritional screening and intervention early in the course of malnutrition(Reference Arends, Bachmann and Baracos191), access to RD for cancer patients is poor. For example, evidence-based guidelines from Australia recommend that all patients receiving radiotherapy to the gastrointestinal tract or head and neck area are routinely referred to dietitians(Reference Isenring, Zabel and Bannister202), however, a service provision audit in the UK found that only 69 % of head and neck cancer patients see a dietitian, with those having oral tumours the most likely to be referred(Reference Bradley, Zutshi and Nutting203). Generally speaking, there is a lack of dietitians providing care to those affected by cancer. In Ireland, as of 2016, there were thirty-six RD working in cancer care, of which only five are practising outside the capital city of Dublin, which provides only one dietitian to every 1389 active cancer patients(204). This represents all dietitians who cover oncology as part of their role and does not constitute the number of dedicated oncology dietitians. In the USA, there are approximately 1⋅7 full-time equivalent dietitians per outpatient cancer centre, corresponding to 1 RD to 1202 patients(Reference Trujillo, Claghorn and Dixon205). In Ontario, Canada, there are few dietitians practising in oncology and palliative care, with 1⋅1–1⋅6 full-time equivalent full-time equivalent RD per 100 inpatient beds and 0⋅2–1⋅4 full-time equivalent/100 patients in the outpatient setting(206). There is also a lack of specialist dietitians in oncology, with only 370 board-certified oncology specialist dietitians in the USA(Reference O'Sullivan Maillet, Brody and Skipper207).
When malnourished patients are seen by dietitians the mainstay dietary treatment, particularly for those with poor appetite is a ‘little and often, high protein high energy diet’. This diet constitutes a food-first approach which involves counselling patients and their carers on foods that are naturally high in protein and energy and providing meal and snack options to achieve this. Our group has developed several cookbooks to bring this advice to life over the past number of years and these are available as free downloadable e-books at www.breakthroughcancerresearch.ie/books. These resources are written in lay language and provide simple high protein high energy meal options. For some patients with continued problems with poor appetite and early satiety, oral nutritional supplements and/or enteral feeding will be necessary to support their nutritional status during cancer treatment.
Systemic inflammation
As observed by Sir David Cuthbertson in 1942(Reference Cuthbertson208) in reference to the post-shock metabolic response ‘it is doubtful whether, during the early catabolic phase, any dietary measure can effectively suppress the catabolic destruction of protein’. One could argue the same is true for cancer where systemic inflammation is present. Systemic inflammation is present in 30–50 % of advanced cancer populations(Reference Shinko, Diakos and Clarke209) and is an independent factor reducing survival(Reference McMillan210). Over the years several pharmaceutical and dietary factors have been examined to reduce inflammation including corticosteroids, non-steroidal inflammatory drugs, statins and n-3 fatty acids. Corticosteriods appear to increase appetite and QoL for a limited period of time but the optimal dose, duration or timing of intervention is not clear. There is good evidence that non-steroidal anti-inflammatory drugs can lead to increases in body weight in cachexia and a plausible mechanism is the attenuation of cachexia-related inflammation and subsequent modulation of the anabolic resistance associated with cancer cachexia. However, non-steroidal anti-inflammatory drugs do have some risks, such as gastrointestinal bleeding, and so further evidence is needed to prove efficacy and safety in cancer cachexia management(Reference Solheim, Fearon and Blum211). n-3 Fatty acids have been associated with weight stabilisation(Reference Ryan, Reynolds and Healy212,Reference Persson, Glimelius and Ronnelid213) , improved QoL(Reference Lewis, Xun and Fly214,Reference Werner, Kullenberg de Gaudry and Taylor215) and increased chemo-sensitivity(Reference Camargo, Mocellin and Brunetta216) in some studies.
Physical activity
Physical activity and more specifically, structured exercise, has been suggested as part of the management of cancer cachexia(Reference Maddocks, Murton and Wilcock217). Exercise has been shown to be safe in individuals living with and beyond cancer(Reference Turner, Steed and Quirk218) and can promote QoL in patients on active treatment(Reference Mishra, Scherer and Snyder219) and during the survivorship period(Reference Mishra, Scherer and Geigle220). Moreover, the specific QoL domains impacted by exercise are those which are commonly impaired in cancer cachexia (physical functioning, role functioning, fatigue and body image/self-esteem)(Reference Mishra, Scherer and Snyder219,Reference Mishra, Scherer and Geigle220) . Of note, it has been shown that the most QoL benefit is gained from supervised exercise programmes(Reference Sweegers, Altenburg and Chinapaw221) and that those patients with the lowest baselines experience the most improvement in fatigue, QoL, aerobic fitness and physical function, indicating that the patients who are most inactive could benefit from any increase in exercise(Reference Buffart, Sweegers and May222).
Based on the current evidence, the American College of Sports Medicine position is that exercise is safe and beneficial for cancer patients and their guidelines provide evidence-based recommendations in terms of safety measures, and exercise prescription specifics across many tumour types, with a focus on the prescription of physical activity using the frequency, intensity, time, type framework. The recommended target to achieve the documented benefits of exercise programmes in cancer is ≥30 min moderate-intensity aerobic exercise ≥3 times per week for at least 8–12 weeks and resistance training ≥2 times per week (≥2 sets of eight–fifteen repetitions ≥60 % one-repetition maximum)(Reference Campbell, Winters-Stone and Wiskemann223,Reference Schmitz, Courneya and Matthews224) . Likewise, the Clinical Oncology Society of Australia has a position statement, as of 2018, which states that all members of the multidisciplinary team should promote physical activity amongst cancer patients and that patients should be encouraged to return to normal levels of activity as soon as possible after diagnosis and that they should aim for ≥150 min moderate-intensity or 75 min vigorous-intensity aerobic exercise per week and 2–3 sessions of resistance exercise per week (moderate to vigorous-intensity exercises targeting the major muscle groups)(Reference Cormie, Atkinson and Bucci225).
Multimodal approaches
When the multifactorial nature of malnutrition in cancer is considered, it seems reasonable that a multimodal approach to treatment may fare better than a unimodal approach. By targeting multiple aetiologies, there is some evidence that multimodal therapies may have a better chance of attenuating the progression of cachexia(Reference Solheim, Vagnildhaug and Laird226). Multimodal management of cancer cachexia should incorporate general interventions such as nutrition counselling, symptom management and exercise as well as focused interventions that address specific aetiological components of the cancer cachexia syndrome, such as fish oil or non-steroidal anti-inflammatory drug to address increased inflammation or corticosteroids to improve appetite(Reference Del Fabbro227).
Early intervention is of the utmost importance as refractory cachexia remains a challenge, still considered irreversible and associated with a terminal prognosis(Reference Fearon, Strasser and Anker38). The European School of Oncology Task Force's official position is that research should focus on the identification and management of cachexia early in the disease course when it is amenable to treatment(Reference Aapro, Arends and Bozzetti123). Furthermore, it has been suggested that a ‘parallel pathway’ approach should be adopted to ensure that cachexia is managed alongside cancer itself, recognising their inherent connection and avoiding the sentiment that cachexia is an inevitable endpoint associated with advanced disease, but rather focusing on optimising clinical outcomes by preventing the development of malnutrition(Reference Muscaritoli, Molfino and Gioia228).
A systematic review by Hall et al. showed that the current literature base for combined nutrition and exercise programmes in advanced cancer is lacking in strong evidence. While studies to date have shown variable improvements in QoL, overall function, fatigue, endurance/strength, depression and nutritional status, the results are inconsistent across studies and they are often underpowered(Reference Hall, Cook and Maddocks229). The strongest evidence in favour of these trials is only of moderate quality and it suggests that physical endurance and strength, as well as mood, can be improved by these interventions. Further well-designed studies are needed in order to verify the utility of multimodal approaches.
MENAC, a large multi-centre phase III trial (A randomised, open-label trial of a multimodal intervention (exercise, nutrition and anti-inflammatory medication) plus standard care v. standard care alone to prevent/attenuate cachexia in advanced cancer patients undergoing chemotherapy) is currently underway (ClinicalTrials.gov ID: NCT02330926)(Reference Solheim, Laird and Balstad230). Identification of a successful cachexia treatment would mark significant progress in the field of oncology nutrition, and given the impact of nutrition of survival and tolerance to treatment, the oncology field as a whole.
Conclusions
WL and abnormalities of body composition are common across all cancer sites and stages and the aetiology of malnutrition in cancer is multifactorial and complex. It is associated with poorer QoL as well as increased morbidity and mortality and is often considered an inevitable part of the cancer trajectory. Irrespective of baseline BMI, muscle and fat wasting are associated with poorer outcomes; however, simple screening tools using weight and BMI alone miss a large proportion of patients with altered body composition who are at risk nutritionally and therefore, techniques to adequately identify patients at risk of malnutrition must be developed and widely implemented in order to facilitate early-intervention and a parallel pathway.
Despite the widespread fatalism with respect to cancer cachexia, patients do retain anabolic potential and although nutritional interventions, to date, have not been shown to increase survival, it may be that these interventions have not been successful in addressing the malnutrition as a primary outcome and thus, the benefit of survival has not been borne out. With more successful therapies, including multimodal and interdisciplinary approaches, it may be that nutritional interventions can improve not only QoL but also the length of life. Management of cancer-related malnutrition must focus on early-intervention with multimodal approaches in order to tackle the multifactorial nature of cachexia pathophysiology.
Further research into the pathogenesis and consequences of cancer-related malnutrition may lead to a better understanding of potential targets for treatment. However, while a number of promising therapies for cachexia are under investigation, the field lacks currently licensed treatments and so interventional research must be prioritised in order to provide an evidence base for the treatment of the condition which is now well documented as causing poor outcomes.
In conclusion, prompt identification of patients with cancer-related malnutrition must be optimised and development of an effective, evidence-based treatment strategy is of the utmost importance as it stands to improve longevity and QoL for cancer survivors.
Acknowledgements
The authors would like to acknowledge the Health Research Board Clinical Research Facility at Mercy University Hospital Cork where a lot of the research mentioned in the article was conducted and their statistician Dr Darren Dahly for his ongoing help and support of our research.
Financial Support
The free cookbooks for cancer patients mentioned in this article received financial support from Breakthrough Cancer Research (Irish Registered Charity). The national survey mentioned in this article on attitudes of Irish cancer patients to nutrition was in part supported by the Irish Society of Clinical Nutrition & Metabolism (IrSPEN)
Conflict of Interest
None
Authorship
A. R. delivered the Cuthbertson Medal Lecture. A. R. and E. S. co-wrote the paper.