Introduction
Insects are hosts of numerous species of nematodes that can have negative impacts on their life cycle. The Heterorhabditidae and Steinernematidae are the two best known families of entomopathogenic nematodes (Liu et al., Reference Liu, Poinar and Berry2000). Their members are lethal obligatory parasites that carry endosymbiotic pathogenic bacteria. Once released into the haemocoel, the bacteria will kill the host insect within a few days through septicemia and/or toxaemia (Kaya and Gaugler, Reference Kaya and Gaugler1993). These nematodes thus have a life cycle similar to parasitoids, as they always complete their development within a single host and they end up killing it (Eggleton and Gaston, Reference Eggleton and Gaston1990; Brodeur and Boivin, Reference Brodeur and Boivin2004). In recent years, entomopathogenic nematodes have generated much interest for biological control applications because they can be easily mass-produced, they are environmentally safe and they can be applied using conventional spraying equipment (Liu et al., Reference Liu, Poinar and Berry2000).
There are other groups of nematodes that infect insects without killing them. Their life cycle is thus more similar to true parasites; they enter the host, use its resources and do not necessarily kill it. Their presence can reduce or increase the longevity of the infected insect, reduce or suppress its reproduction, reduce its gamete size, or reduce its vigour and its competitive ability (Thong and Webster, Reference Thong and Webster1975; Bollache et al., Reference Bollache, Gambade and Cézilly2001). Several obligate parasitic nematode species are known to castrate their host (Hurd, Reference Hurd2001). This destruction or alteration of the gonadal tissues induces a modification of reproductive behaviour and a hormonal imbalance, which in return results in a reduction of the host's reproductive efforts (Baudoin, Reference Baudoin1975). Castration generally benefits the parasite by increasing resource availability and increasing host viability and longevity, a consequence of reduced host reproductive efforts. In theory, both sexes can be affected, but it is predicted that castration should affect the sex that invests most in reproduction, i.e. most often the female (Baudoin, Reference Baudoin1975). Curtailing host reproduction might be the only way for a parasite to utilize host resources without decreasing host survival (Hurd, Reference Hurd2001). It was proposed that some parasites could modulate insect reproductive output by directly interfering with gene expression of the host (Hurd, Reference Hurd1998). This chemical castration would thus be triggered by a molecular signal secreted by the parasite as a result of an adaptive strategy.
Several nematodes in the family Allantonematidae are obligate parasites of different insect orders. The typical life cycle of such nematodes starts when an inseminated female infects a host insect by penetrating through its cuticle or through an opening. The female then grows, produces eggs and releases larvae into the haemocoel of the host. This progeny then exits through the anus or the ovipositor when the host female performs pseudo-oviposition (Jaenike, Reference Jaenike1992). Moreover, Allantonematidae can infect many insect orders, suggesting lots of potential applications for biological control. A species of Allantonematidae, Bradynema listronoti (Nematoda: Allantonematidae), was described from field-collected carrot weevils Listronotus oregonensis oregonensis (LeConte) (Coleoptera: Curculionidae) (hereafter L. oregonensis) (Zeng et al., Reference Zeng, Giblin-Davis, Ye, Bélair, Boivin and Thomas2007). This is the first species of Bradynema to be described from North America and the first isolated from a Curculionidae host. This species exhibits a parasitic lifestyle, infecting a large proportion of the adult population (Zeng et al., Reference Zeng, Giblin-Davis, Ye, Bélair, Boivin and Thomas2007). Its host, the carrot weevil, L. oregonensis, is endemic to eastern North America where it feeds on several umbelliferous plants, including crops such as carrot, celery and parsley (Boivin, Reference Boivin1999).
In this paper, we describe the interaction between the carrot weevil L. oregonensis and the nematode B. listronoti, at both physiological and molecular levels. The prevalence of the parasitic nematode, its emergence from host weevils and its host range are presented, as well as susceptibility of different weevil stages, adult reproductive success and mortality rate. Finally, we monitored the modulation of gene expression in weevils infected by the nematode.
Materials and methods
Field interactions
Infection prevalence
Carrot weevils, L. oregonensis, were collected from carrot plots on an experimental farm located in Sainte-Clotilde-de-Châteauguay, QC, Canada (45°09′N, 73°41′W) from 2002 to 2017 using bait traps developed by Boivin (Reference Boivin1985). Because baited-traps are more effective when there is no competition with mature carrot crops, most of the weevils were captured early in the season (April, May and June), representing the overwintering generation of L. oregonensis. In order to catch weevils from the second generation, specimens were hand-collected during the months of August and September when possible. All sampled adult weevils were dissected and the number of B. listronoti and their developmental stages were monitored under a light microscope. Following dissection, oocyte development was also evaluated in females.
Emergence rate of B. listronoti
The emergence rate of B. listronoti from adult carrot weevils was monitored over a period of 45 days after field collection at the experimental farm. Individual weevils were placed on a sieve over distilled water inside a Solo® cup (P100, 29.6 mL, Solo Cup Company) in a growth chamber (Conviron, Model E15) at 24 °C with a 16:8 day:night cycle and 50% relative humidity. Emerging nematodes were collected every 4 days in distilled water and transferred into a counting chamber for microscopic enumeration. Emergence rates for a total of 80 parasitized carrot weevils, 40 of each sex, were characterized.
Host range and distribution
Host range of B. listronoti was evaluated by sampling (hand-collected) other Curculionidae species found nearby the experimental farm on different host plant (Supplementary Table S1). Each of the collected specimen was dissected to check for the presence of nematodes under the microscope.
Distribution of B. listronoti was assessed by collecting carrot weevil individuals from different fields (n = 7) using bait traps, around the experimental farm (<20 km). Weevils collected were dissected the same day to verify the presence of nematodes under the microscope.
In vitro interactions
Carrot weevil colonies
A colony of L. oregonensis was maintained on carrots (Daucus carota L. var. sativa DC.) using the method of Martel et al. (Reference Martel, Svec and Harris1975). This rearing was set up 15 years ago from weevil individuals collected at the experimental farm in Sainte-Clotilde-de-Châteauguay. A separate rearing was established in 2007 and inoculated with field weevils infected with B. listronoti. Both colonies were incubated in a growth chamber (Conviron, Model E15) at 24 °C with a 16:8 day:night cycle and a relative humidity of 50%. Uninfected carrot weevil rearing is referred hereafter as the ‘healthy colony’, and the infected carrot weevil rearing as the ‘infected colony’.
Life stage susceptibility
The susceptibility of different L. oregonensis life stages was evaluated by placing each weevil stage (larvae, pupae or adult) in soil inoculated with infective larvae of B. listronoti. Weevil larvae used in the experiment were between the third and the fourth larval stage, pupae were 2–3 days old, and adults (sex ratio 1:1) taken from the rearing were <3 months old. Experimental units were composed of ten specimens of carrot weevils in a plastic cup containing 20 cc of soil inoculated with ca. 500 juveniles of B. listronoti. After 1 week of exposure, the insects were removed from the soil and incubated alone for up to 3 months. Eight replicates were made for each treatment, for a total of 80 individuals of each carrot weevil life stages. The percentage of infected weevils was determined through dissection when weevil mortality was observed or at the end of the experiment.
Fecundity and sexual transmission
The number of eggs laid by uninfected and infected carrot weevils was evaluated over a period of 2 months. Each newly emerged female was reared separately inside a Solo® cup with a piece of carrot and maintained in a growth chamber (Conviron, Model E15) as above. Carrot weevil fecundity was expressed as the total number of eggs laid (recorded each 3–4 days) during 2 months. After this time, females were dissected to evaluate oocyte development in the reproductive tract. The experiment was replicated three times using 25 uninfected and infected females for each trial. In order to evaluate the transmission of B. listronoti during weevil mating, 40 infected males from the infected colony were coupled with 1-week-old uninfected virgin females from the healthy colony. After copulation, females were isolated in a Solo® cup as above. After 30–60 min, 20 females were dissected to evaluate the number of nematodes and the presence of sperm in the spermatheca in order to confirm copulation. The remaining 20 females were dissected after 2 months.
Mortality rate
Finally, the mortality rate of 30 females and 30 males from both the healthy and the infected colonies were monitored individually over a period of 3 months under the same rearing conditions (see above). All weevils were dissected after their death or at the end of the experiment to confirm the presence/absence of B. listronoti. This experiment was repeated three times.
Molecular interactions
A comparison of RNA transcripts from uninfected and infected carrot weevils was realized using next-generation sequencing (RNA-Seq) to evaluate the impact of the parasite on gene expression of its host. Samples consisted of individual adult females of the carrot weevil infected with B. listronoti or uninfected females. All weevils were reared in vitro in a controlled environment from two distinct colonies (healthy and infected, see above) and each treatment was repeated three times.
Whole-insect samples were homogenized in 700 µL of RTL plus buffer (Qiagen, Mississauga, Canada), with one 6 mm zirconium bead and 200 µL of 1 mm zirconium beads, using the PowerLyzer 24 Homogenizer (3 × 45 s, at 2500 rpm; MO BIO, Carlsbad, CA, USA), and stored at −80 °C until RNA purification. Total RNA was extracted using the RNeasy Plus mini kit (Qiagen) according to manufacturer's instructions and stored at −80 °C prior to library preparation. RNA samples were quantified with the NanoDrop 2000 (ThermoFisher Scientific, Mississauga, Canada) and their integrity was assessed with the Bioanalyzer 2100 (Agilent Technologies) using the RNA 6000 Nano kit. Library preparation and sequencing were performed at the Institute for Research in Immunology and Cancer (IRIC; Université de Montréal, Montréal, QC, Canada) using the TruSeq RNA sample prep kit v2 (Illumina, San Diego, CA, USA) and the HiSeq 2000 sequencer (Illumina). All samples were multiplexed in a single sequencing lane. Reads were trimmed using the Trimmomatic software (Bolger et al., Reference Bolger, Lohse and Usadel2014). The Trinity software package was used to create a de novo transcriptome assembly and to produce a raw count matrix (Grabherr et al., Reference Grabherr, Haas, Yassour, Levin, Thompson, Amit, Adiconis, Fan, Raychowdhury and Zeng2011; Haas et al., Reference Haas, Papanicolaou, Yassour, Grabherr, Blood, Bowden, Couger, Eccles, Li and Lieber2013). A post-assembly decontamination method using a hierarchical clustering algorithm (Lafond-Lapalme et al., Reference Lafond-Lapalme, Duceppe, Wang, Moffett and Mimee2017) was used to separate the transcripts from B. listronoti and putative contaminants from those of L. oregonensis. Differential expression was measured using the DESeq2 Bioconductor package (Love et al., Reference Love, Huber and Anders2014) in R statistical software (R Core Team, 2014). Transcripts gene ontology (GO) annotation was done using Blast2GO (Conesa et al., Reference Conesa, Götz, García-Gómez, Terol, Talón and Robles2005).
Results
Field interactions
Bradynema listronoti prevalence
A total of 4158 carrot weevils were collected between 2002 and 2017 across 113 sampling dates. Bradynema listronoti prevalence in carrot weevil populations was not statistically different across sampling years at the Sainte-Clotilde site and ranged from 20 to 63% with an average of 42% [F (1,111) = 2.986, P = 0.0868; Fig. 1]. The prevalence of infection increased during the growing season with a mean level of 38% in early-May (day of year 120) and 57% in mid-September (day of year 260) [F (1,111) = 8.347, P = 0.0046; Fig. 2]. Collected weevils were distributed in two clusters (early-season and late-season individuals), representing the first and the second generation, respectively. All developmental stages of the nematode were found in the insect haemocoel and the number of adult nematodes per weevil was one in 30% (n = 539), 2–9 in 55% (n = 987) and >10 in the remaining 15% (n = 269) of infected carrot weevils.
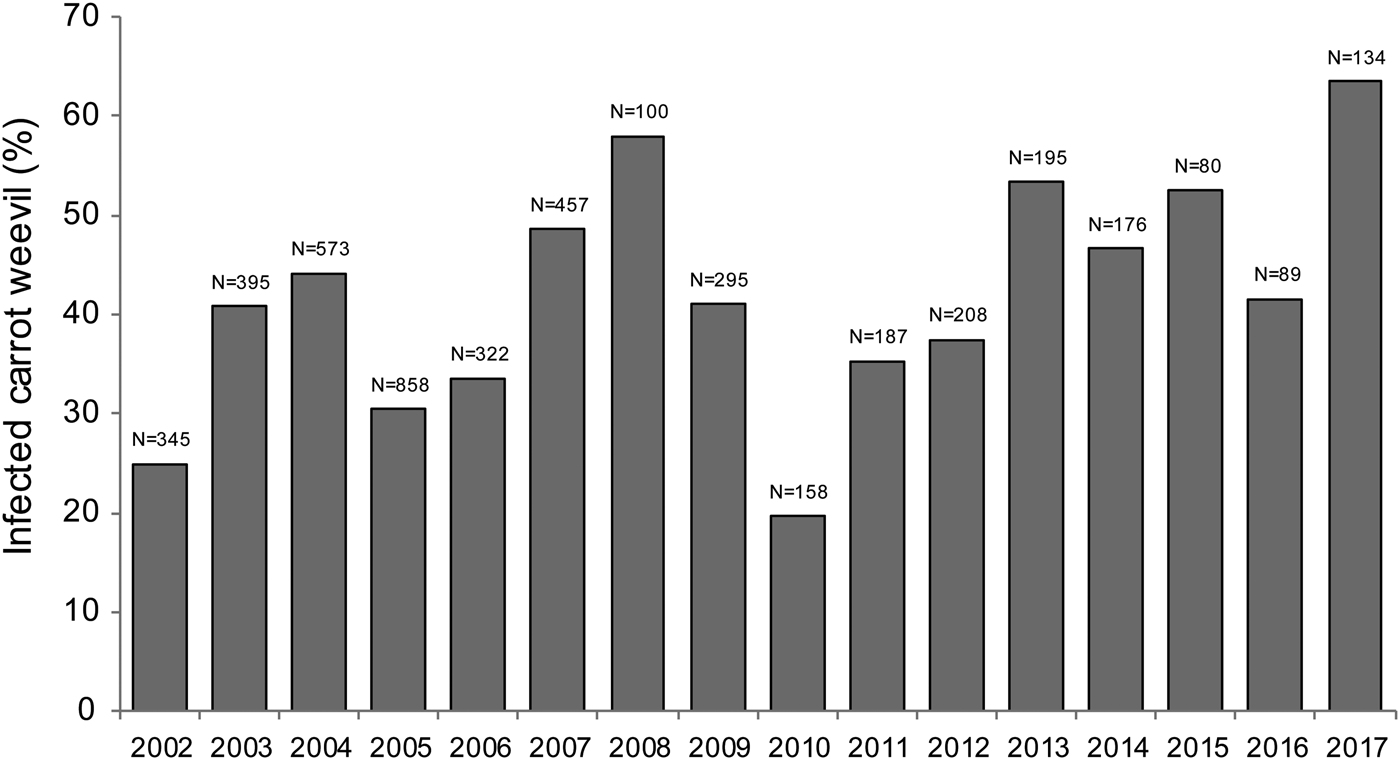
Fig. 1. Infection prevalence of carrot weevil, Listronotus oregonensis, by the parasitic nematode Bradynema listronoti from 2002 to 2017 in Sainte-Clotilde-de-Châteaugay, Quebec, Canada.

Fig. 2. Prevalence of Bradynema listronoti infesting the carrot weevil, Listronotus oregonensis at different sampling dates over 16 years. Each data point corresponds to a sample of at least ten weevils, mean of 36.8 weevils/sample.
Emergence rate of B. listronoti
The emergence of B. listronoti juveniles from field-infected adult weevils was found to be continuous over a period of 45 days with a mean of 5500 nematodes emerging from each weevil during that time. There was no difference in total number of nematodes emerging from male and female weevils [F (1,57) = 0.001, P = 0.97].
Host range and distribution
A total of 830 specimens spread across 21 species of Curculionidae were dissected. All specimens were without parasites, except for specimens of two species where the presence of nematodes was confirmed. Tyloderma nigrum found on Betula alleghaniensis and Apion sp. on Bidens sp. showed an infection prevalence of 27% (8/30) and 4% (2/50), respectively, to a Bradynema-like species (Supplementary Table S1). Identification to the species level was not possible for the specimens found in those two Curculionidae species due to the low quality (degradation of tissues) of the nematodes.
The distribution of B. listronoti was restricted to the Sainte-Clotilde experimental farm. No nematodes were observed by dissection of the 148 carrot weevil individuals (86 males, 62 females) from the other fields.
In vitro interactions
Life stages susceptibility
Among the different developmental stages of the carrot weevil, larvae were found to have higher prevalence of infection suggesting they were more susceptible to infection (56.6 ± 7.9% infected) than adults (7.0 ± 3.4% infected) or pupae (3.9 ± 2.7% infected) [F (2,20) = 34.07, P < 0.0001; Table 1].
Table 1. Carrot weevil life stage susceptibility to infection by Bradynema listronoti and impacts of infection on the average number of eggs laid per female and mortality rate
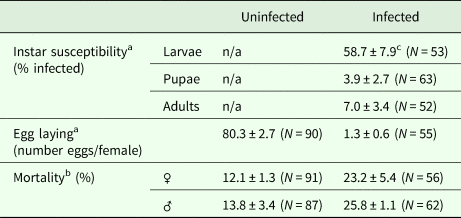
a Over a period of 2 months.
b Over a period of 3 months.
c Mean ± s.e.
Fecundity and sexual transmission
Carrot weevil female fecundity was strongly reduced after infection by B. listronoti. In the presence of nematodes, the average number of eggs laid per female dropped from 80.3 (in uninfected females) to 1.3, representing a 60-fold decrease [F (1,139) = 77.15, P < 0.0001; Table 1]. The few eggs still produced by infected females were laid during the very first days. Dissection of field-collected and reared females at different times (young female before mating, 24 and 48 h after mating) revealed that the presence of nematodes diminishes egg production when infected with B. listronoti (Fig. 3). Mature oocytes were never observed in field-collected weevils infected by B. listronoti (n = 1,795). Anecdotally, we observed that infected female weevils, although sterile, nonetheless attempted to oviposit and deposited nematodes at the oviposition site. Sexual transmission by any infected males was not observed. No nematodes were recovered in females that had copulated with infected males after 30–60 min (n = 20) or 2 months after copulation (n = 20).
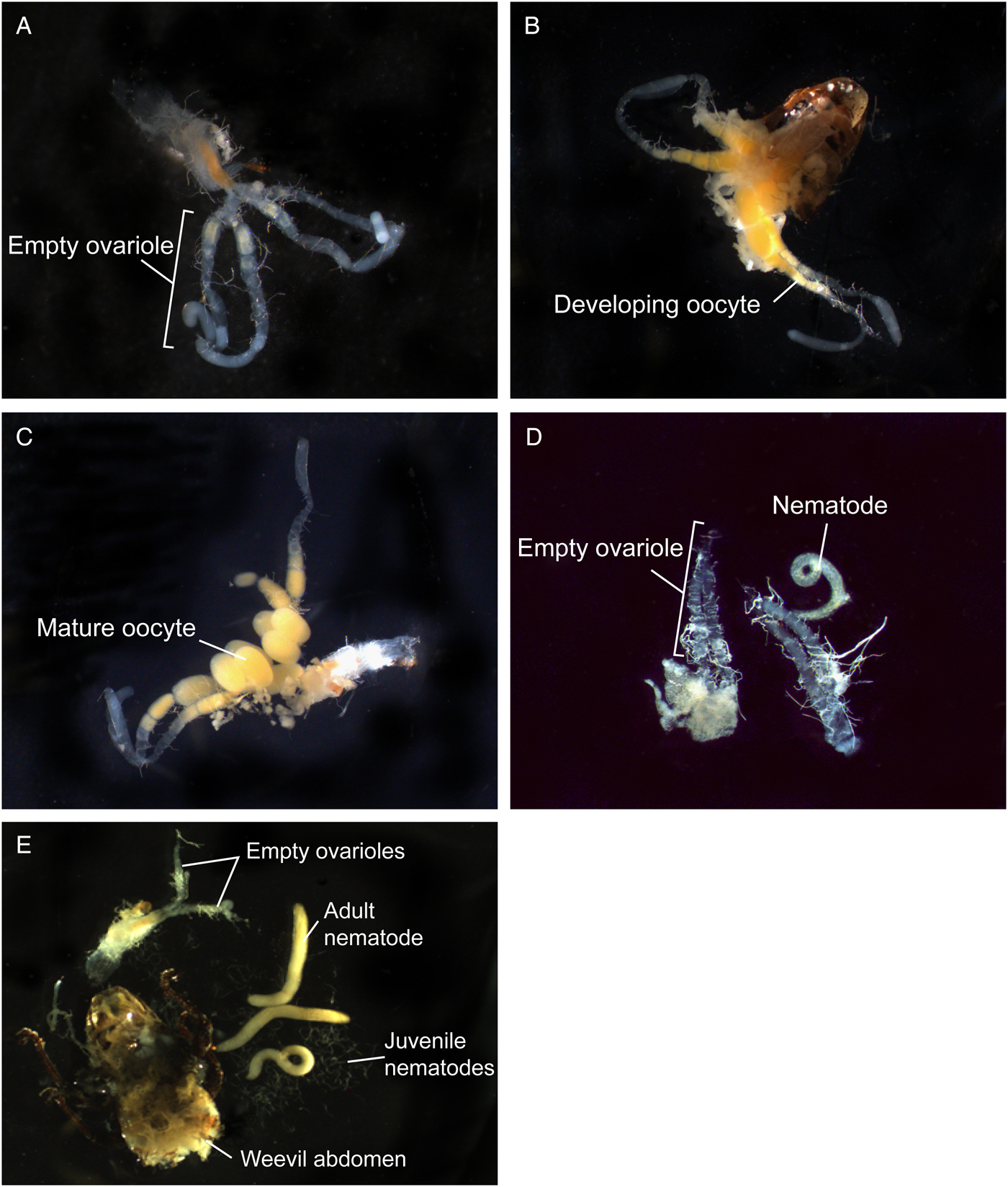
Fig. 3. Effects of infection by Bradynema listronoti on egg development of Listronotus oregonensis: (A) uninfected, unmated 8 days old female; no oocyte (ovarioles empty); (B) uninfected, mated (24 h after copulation) 15 days old female; developing oocytes in ovarioles; (C) uninfected, mated (48 h after copulation) 15 days old female; presence of mature oocytes; (D) field-infected, mated (filled spermatheca) female; no oocyte development in ovarioles; (E) field-infected, mated female, no oocyte development in ovarioles, showing different developmental stages of B. listronoti (nematode).
Mortality rate
The mortality rate was higher for infected weevils [F (1,6) = 16.09, P = 0.0070]. This effect was more pronounced for male weevils [F (1,4) = 11.9, P = 0.0261] than females [F (1,4) = 2.73, P = 0.1740; Table 1].
Molecular interactions
Sequencing of RNA extracts obtained from whole adult females of uninfected and infected carrot weevils generated 151 million 100-bp paired-end reads (NCBI bioproject accession number PRJNA313413). After removal of chimeras, redundant isoforms and transcripts from B. listronoti, the final carrot weevil transcriptome contained 53 328 transcripts. The comparison of transcript abundance between the infected and uninfected weevils yielded 133 differentially expressed genes with fold change ranging from −68.1- to 72.5-fold. From this number, 73 had blast hits and 57 a predicted function (Supplementary Table S2). The analysis of the GO of these genes revealed that the overexpressed were mostly proteases while the downregulated were involved in basal metabolism (structural constituent of cuticle), defence (redox, defence protein) or oviposition (Supplementary Fig. S1).The most overexpressed annotated gene was a serine dehydrogenase with a fold change of 24.6, while the most downregulated gene was a lipase-like gene with a fold change of −25.3 (Supplementary Table S2). Of particular interest, the juvenile hormone esterase E4-like gene was found to be repressed by 6.9-fold and the ebony gene by 8.6-fold.
Discussion
This extensive study of the parasitic nematode B. listronoti and its host, the carrot weevil L. oregonensis, provides evidence for parasitic castration of females. The infection prevalence of weevils under field conditions was high (at least 20%) and persistent over the 16 years of the survey. Under controlled conditions, all developmental stages of the weevil studied were susceptible to B. listronoti, but larvae were significantly more vulnerable than pupae or adults with a 57% infection prevalence after 1 week of exposure. Although the mortality was significantly higher for infected weevils, the percentage mortality was low (<25%) and the effect was not noticeable until after 30 days. The most marked effect of B. listronoti infection on weevils was the reduction of female fecundity (>60 × decrease in egg production). This change is associated with an inhibition of the maturation of the reproductive system (reduced number of eggs) that may have resulted from modulation of gene expression in weevils infected by the nematode.
Parasitic castration
The number of eggs laid by carrot weevil females was strongly reduced after infection by B. listronoti. Actually, only a few eggs were produced by infected weevils in the in vitro experiment and were all laid during the first days. It is thought that these weevils were infected only a few days before egg laying, during the pupal or pre-oviposition stages. This is supported by the rapid cessation of egg laying and the low number of nematodes found in these females after 2 months (not shown). On the other hand, no mature oocytes were observed in the field specimens infected by B. listronoti. It is probable that, in the rare instances where a female is infected at the later stages, the number of juvenile nematodes is too low to be recorded when oocytes are still present or oocytes too degenerated to be seen along with nematodes. Dissection of infected females provided evidence that the presence of nematodes can stop the maturation of the female gonads. Bradynema listronoti can thus be considered as a castrating parasite involving a physiological modification of the female host gonad (Baudoin, Reference Baudoin1975). This may result in juvenilization of the host which could have other impacts on weevil behaviour such as a reduction in mate searching. The percentage of infection was similar between male and female carrot weevil adults.
Furthermore, some castrators have been shown to secrete hormones directly into the host or to interfere with the host endocrinological organs (Baudoin, Reference Baudoin1975; Hurd, Reference Hurd2001). Transcriptomic analyses in this study revealed that this parasitic castration may have been triggered by the inhibition of reproductive hormones. We found that two genes associated with reproductive processes, the juvenile hormone esterase E4-like and ebony, were repressed by 7- and 9-fold, respectively, in weevils infected by B. listronoti. The juvenile hormone plays a gonadotropic role in insect reproduction, where it triggers the functioning of organs such as the ovary, accessory glands and fat body (Gruntenko and Rauschenbach, Reference Gruntenko and Rauschenbach2008). The ebony gene is implied in N-b-alanyldopamine synthase activity which regulates dopamine content (Hodgetts and Konopka, Reference Hodgetts and Konopka1973). Dopamine is an inhibitor of juvenile hormone production. Repression of these genes suggests that the weevil female may be regulating reproductive hormones in response to infection, as shown in other studies (Hurd, Reference Hurd1998; Beckage, Reference Beckage1991). This hypothesis will have to be validated in future experiments by knocking down these genes.
In entomopathogenic nematodes, it was demonstrated that symbiotic bacteria in nematodes suppress the host immune system by the repression of phospholipase A2, PLA2 (Kim et al., Reference Kim, Ji, Cho and Park2005). PLA2 is involved in the biosynthesis of eicosanoids that play a crucial role in non-self-recognition in insects (Howard et al., Reference Howard, Miller and Stanley1998; Stanley-Samuelson et al., Reference Stanley-Samuelson, Jensen, Nickerson, Tiebel, Ogg and Howard1991). Here we show that the gene coding for ‘phospholipase a2b precursor’ was highly repressed (>23-fold) suggesting that the immune system in infected weevils may be compromised. In addition, a gene coding for an ‘inducible metalloproteinase inhibitor’ was found to be overexpressed (by 14-fold). The product of this gene, discovered in Galleria mellonella (Lepidoptera: Pyralidae) (Clermont et al., Reference Clermont, Wedde, Seitz, Podsiadlowski, Lenze, Hummel and Vilcinskas2004), is currently the only known specific inhibitor of metalloproteinases found in animals. Its role is to inhibit the activity of metalloproteinases secreted by pathogenic organisms as virulence factors to degrade immune-relevant polypeptides of the infected host. This suggests that, even if a part of its immune response is inhibited, the beetle is still responding to the presence of infection.
Biological control
Carrot production has a long history of integrated pest management. In North America, a vast programme was developed in the early 1980s to manage several pests, including the carrot weevil, while minimizing the use of pesticides (Boivin, Reference Boivin, Mason and Gillespie2013). However, with the reinforcement of environmental laws and the objective to reduce pesticide use, the industry is challenged to improve production. Carrot weevil has been the object of several biological control studies (see Boivin, Reference Boivin1999 for review), but its management could still be improved. Also, the northern growing regions were until recently restricted with a single generation of carrot weevil. However, the occurrence of a second generation causing damage to the crop is progressively increasing and becoming a concern (Boivin, Reference Boivin, Mason and Gillespie2013). With the current perspective of climate change, this situation is expected to worsen. In this context, the discovery of B. listronoti and its possible development as a biological control agent is very significant.
Other nematodes species have been tested against carrot weevil, and some, like Steinernema carpocapsae or Heterorhabditis bacteriophora, reduced the oviposition and the survival of L. oregonensis (Boivin and Bélair, Reference Boivin and Bélair1989; Bélair and Boivin, Reference Bélair and Boivin1995; Miklasiewicz et al., Reference Miklasiewicz, Grewal, Hoy and Malik2002). The entomopathogenic nematode species kill their host very efficiently and very quickly when they are in continuous contact with it. However, soil applications of this nematode alone failed to achieve a level of control similar to insecticides and appeared vulnerable to desiccation at lower relative humidity (Bélair and Boivin, Reference Bélair and Boivin1995).
While the entomopathogenic nematodes belonging to the Steinernematidae kill their host through the inoculation of deleterious bacteria, B. listronoti infection seems to hamper the expansion of carrot weevil populations through the sterilization of female due to an atrophied reproductive system. Even if the mortality induced by B. listronoti infection was low (<25%), its effect on oviposition was very significant, causing a >60-fold decrease in number of eggs laid. And because B. listronoti has a low impact on carrot weevil mortality, it is likely to spread by weevil movements. Notably, the infected female weevils, although sterile, nonetheless attempted to oviposit and deposited nematodes at the oviposition site. Because other uninfected females are likely to use the same site for oviposition, this behaviour should contribute to the spread of the nematode. Furthermore, our data demonstrate the continuous emergence of a high number of B. listronoti juveniles from infected adult weevils over 45 days and this should allow the dissemination of infection over a long time frame, a key factor in biological control. Bradynema listronoti is unique compared with commercially available entomopathogenic nematodes in being host-specific. It may therefore help to preserve the natural community of curculionids (some of which are beneficial for the control of several weeds) and other arthropods if used for biological control. Further studies are needed to confirm its effectiveness under commercial production conditions.
Supplementary material
The supplementary material for this article can be found at https://doi.org/10.1017/S0031182018002056.
Acknowledgements
The authors would like to thank Marie-Ève Poirier, Danielle Thibodeau, Julie Frenette, Joël Lafond-Lapalme, Yvon Fournier, Éléonore Tremblay and Nathalie Dauphinais for technical assistance. The authors are also very grateful to the reviewers for their meticulous reading of the manuscript and valuable comments.
Financial support
This research was funded by an internal grant from Agriculture and Agri-Food Canada.
Conflict of interest
None.
Ethical standards
Not applicable.
Author ORCIDs
Benjamin Mimee 0000-0002-9601-6140