Introduction
Human schistosomiasis is a neglected parasitic disease with great relevance to public health. Worldwide, it is estimated that approximately 230–250 people are infected and 700–800 million live in areas that are at risk of infection, mainly in countries located in South America, Asia and Africa (Steinmann et al., Reference Steinmann, Keiser, Bos, Tanner and Utzinger2006; Colley et al., Reference Colley, Bustinduy, Secor and King2014; McManus et al., Reference McManus, Dunne, Sacko, Utzinger, Vennervald and Zhou2018; Wei et al., Reference Wei, Huang, Chen, Chen, Li, Xu and Yang2018; WHO, 2020). In addition, approximately 200 000–280 000 deaths occur each year due to schistosomiasis and its complications (LoVerde, Reference LoVerde2019). The high prevalence of schistosomiasis is mainly related to people living in extreme poverty and poor sanitation, which represent a serious risk to human health (Ismail et al., Reference Ismail, Hong, Babiker, Hassan, Sulaiman, Jeong, Kong, Lee, Cho, Nam, Oh and Lee2014; Bajiro et al., Reference Bajiro, Dana and Levecke2017; Verjee, Reference Verjee2019).
The infection is caused by helminths of the genus Schistosoma (Colley et al., Reference Colley, Bustinduy, Secor and King2014; Stingl and Stingl, Reference Stingl and Stingl2017; WHO, 2020), belonging to the class Trematoda and phylum Platyhelminthes. The main aetiologic agents of this disease, in terms of clinical relevance, are Schistosoma japonicum, Schistosoma mansoni and Schistosoma haematobium (WHO, 2020). In this review, we focus only on S. mansoni and S. japonicum, as they are the main species associated with hepatic and intestinal schistosomiasis (Wilson et al., Reference Wilson, Mentink-Kane, Pesce, Ramalingam, Thompson and Wynn2007; Chen et al., Reference Chen, Peng, Li, Yang, Wang and Chen2013; McManus et al., Reference McManus, Dunne, Sacko, Utzinger, Vennervald and Zhou2018).
There are 2 distinct phases of clinical progression of intestinal schistosomiasis: the acute and the chronic phases (Gobbi et al., Reference Gobbi, Tamarozzi, Buonfrate, van Lieshout, Bisoffi and Bottieau2020). During the early stages of acute phase of schistosomiasis (before parasite oviposition), there is a predominance of the T helper type 1 (Th1) immune response (Pearce et al., Reference Pearce, Caspar, Grzych, Lewis and Sher1991; Hesse et al., Reference Hesse, Modolell, La Flamme, Schito, Fuentes, Cheever, Pearce and Wynn2001; Pearce and MacDonald, Reference Pearce and MacDonald2002; Colley and Secor, Reference Colley and Secor2014). After schistosome oviposition, the immune response becomes strongly polarized to the Th2 profile, which is related to increasing production of interleukin-4 (IL-4), IL-5, IL-9 and IL-13 (Pearce and MacDonald, Reference Pearce and MacDonald2002; Burke et al., Reference Burke, Jones, Gobert, Li, Ellis and McManus2009). This immune environment is responsible for the formation of granulomas in tissues (Grzych et al., Reference Grzych, Pearce, Cheever, Caulada, Caspar, Heiny, Lewis and Sher1991; Brunet et al., Reference Brunet, Finkelman, Cheever, Kopf and Pearce1997; Hoffmann et al., Reference Hoffmann, Cheever and Wynn2000). The granuloma has an important role for the host, because it contains the tissue damage caused by antigens secreted by the schistosome eggs (Hams et al., Reference Hams, Aviello and Fallon2013; Schwartz and Fallon, Reference Schwartz and Fallon2018). In the chronic phase of schistosomiasis, there is an increase in the production of regulatory cells in the granuloma, which can modulate granulomatous inflammation, promoting a minimization of the disease severity (Hesse et al., Reference Hesse, Piccirillo, Belkaid, Prufer, Mentink-Kane, Leusink, Cheever, Shevach and Wynn2004; Lundy and Lukacs, Reference Lundy and Lukacs2013). However, if this inflammatory reaction does not have an adequate modulation, the granulomas progressively may evolve into large areas of fibrosis, responsible for the main pathology of schistosomiasis (Hams et al., Reference Hams, Aviello and Fallon2013; Schwartz and Fallon, Reference Schwartz and Fallon2018), including hepatosplenomegaly (Masi et al., Reference Masi, Perles-Barbacaru, Bernard and Viola2020), portal hypertension (Grieco et al., Reference Grieco, Sulekova, Nardelli, Riggio, Venditti and Taliani2016) and ascites (Fei-Yue et al., Reference Fei-Yue, Hong-Zhuan, Jie, Rui-Hong, Jin-Hua, Xin-Ting and Guang-Hui2017).
Macrophages are cells of the innate immune system that play important roles in controlling infections (Shapouri-Moghaddam et al., Reference Shapouri-Moghaddam, Mohammadian, Vazini, Taghadosi, Esmaeili, Mardani, Seifi, Mohammadi, Afshari and Sahebkar2018), as well as in tissue remodelling processes, both in ontogenesis and wound healing (Kloc et al., Reference Kloc, Ghobrial, Wosik, Lewicka, Lewicki and Kubiak2019). In the course of S. mansoni and S. japonicum infection, either at its initial stage or during the evolution to the chronic phase, macrophages participate in the immune regulation of the disease (Cortes-Selva et al., Reference Cortes-Selva, Elvington, Ready, Rajwa, Pearce, Randolph and Fairfax2018; Ho et al., Reference Ho, Cheng, Huang, Peng, Lee and Cheng2022).
Macrophages can acquire different phenotypes depending on the stimuli to which they are subjected to (Atri et al., Reference Atri, Guerfali and Laouini2018). These cells can be classified into M1 or ‘classically activated’ cells, with pro-inflammatory action, and M2 or ‘alternatively activated’ macrophages, which are considered anti-inflammatory cells (Mills, Reference Mills2015; Ley, Reference Ley2017; Locati et al., Reference Locati, Curtale and Mantovani2020). However, despite the relevance of macrophages in controlling infections, the participation of the functional types of these cells in acute and chronic schistosomiasis is still not well defined. Thus, this review discusses the different molecules and/or pathways of activation and polarization of macrophages during infection by S. mansoni and S. japonicum, leading to a better understanding of the role of these cells in the immunopathology of schistosomiasis. Based on this knowledge, we may help identify potential targets for the development of better treatment strategies to reduce the morbidity of this disease.
Methods and criteria for literature selection
This literature review was performed using recognized databases including Scopus, Google Scholar, ACS, PubMed, Wiley, Scielo, Web of Science, LILACS and ScienceDirect and covered original and review articles published in English from 1966 to 2022. Articles involving in vitro and/or in vivo experiments were included and addressed the main immunological aspects of S. mansoni and S. japonicum infection related to macrophage polarization, activation and effector functions. To search for these articles, combinations of keywords were used, such as ‘Macrophage’, ‘Schistosoma’, ‘macrophage polarization and Schistosoma’, ‘Macrophage and Schistosoma’, ‘Macrophage and Schistosoma mansoni’, ‘Macrophage and Schistosoma japonicum’. Research involving coinfections was not included in this study.
Immunopathology of the definitive host against infection by S. mansoni and S. japonicum
Parasites of the genus Schistosoma have complex life cycles (Fig. 1), with generations of asexual reproducing larvae living in freshwater snails, the intermediate hosts (some species of the genus Biomphalaria for S. mansoni and the genus Oncomelania for S. japonicum) and another stage of sexual reproduction of adult worms in vertebrate hosts (definitive), including humans (McManus et al., Reference McManus, Dunne, Sacko, Utzinger, Vennervald and Zhou2018; Nelwan, Reference Nelwan2019). Each stage of the parasite's life cycle (cercariae, schistosomulae, adult worms and eggs) within the definitive host triggers a series of immune responses, and consequently, clinical signs that can be harmful to humans (Molehin, Reference Molehin2020; Hambrook and Hanington, Reference Hambrook and Hanington2021; Masamba and Kappo, Reference Masamba and Kappo2021). The interactions between the host immune system and the parasite can be divided into 2 phases (Fig. 2): acute phase (after and before parasite oviposition) and chronic phase (Gobbi et al., Reference Gobbi, Tamarozzi, Buonfrate, van Lieshout, Bisoffi and Bottieau2020).

Fig. 1. Life cycle of Schistosoma mansoni and Schistosoma japonicum. (1) The eggs shed in the feces of the definitive host release the miracidia when they come in contact with water (2), which penetrate in soft tissue the intermediate host snail (Biomphalaria spp./Oncomelania spp.). Inside the snail, the miracidia transform into mother sporocysts, which in turn produce daughter sporocysts by asexual reproduction. After around 30 days post-infection, cercariae emerge from the daughter sporocysts and are shedding by the snails in response to the light and heat (4). The cercariae penetrate the skin of the definitive host (5) and later transform into schistosomula. These larvae enter venous blood vessels and are passively carried to the lungs and heart (6). Upon reaching the hepatic portal system, schistosomula mature, become adult worms (male or female) and mate (7). The mated worms migrate to the lower mesenteric veins of the intestine, where the female sheds the eggs. Part of these eggs pass through the intestinal wall and are eliminated in the feces, starting the cycle again. However, some eggs are not eliminated and get trapped in several organs (mainly the liver and intestines), inducing a potent granulomatous inflammatory response, responsible for schistosomiasis pathology. Source: Created with BioRender.com.

Fig. 2. Different immune response profiles during S. mansoni and S. japonicum infection. Source: Created with BioRender.com.
The first clinical manifestations of the acute phase (cercarial dermatitis, oedema and pruritus) begin 48–72 h after cercariae penetrate the host's skin, and occurs mainly in individuals from endemic areas (frequently exposed to infection) (He et al., Reference He, Yu, Yu, Mao and Hu1990, Reference He, Salafsky and Ramaswamy2005; Khammo et al., Reference Khammo, Bartlett, Clothier and Whitfield2002; Ingram et al., Reference Ingram, Bartlett, Brown, Marriott and Whitfield2003; Lambertucci, Reference Lambertucci2010). The first innate immune barrier encountered by cercariae is the skin (Bartlett et al., Reference Bartlett, Brown, Marriott and Whitfield2000; Whitfield et al., Reference Whitfield, Bartlett, Brown and Marriott2003; He et al., Reference He, Salafsky and Ramaswamy2005). This tissue is composed of keratinocytes, whose function is to secrete cytokines with antimicrobial functions (Roupé et al., Reference Roupé, Nybo, Sjöbring, Alberius, Schmidtchen and Sørensen2010; Piipponen et al., Reference Piipponen, Li and Landén2020). Indeed, the keratinocytes are considered the first active cells in response to cercariae infection (Bourke et al., Reference Bourke, Prendergast, Sanin, Oulton, Hall and Mountford2015). These cells rapidly respond to infections by secreting inflammatory cytokines [IL-6, IL-12, tumour necrosis factor-alpha (TNF-α) and IL-1β] to repair damaged tissue (Hogg et al., Reference Hogg, Kumkate, Anderson and Mountford2003a, Reference Hogg, Kumkate and Mountford2003b). When penetrating the host's skin, cercariae also cause an increase in antigen-presenting cells in the innate immune system, such as Langerhans cells and dendritic cells (DCs), as shown in Fig. 2 (Angeli et al., Reference Angeli, Faveeuw, Roye, Fontaine, Teissier, Capron, Wolowezuk, Capron and Trottein2001; Kumkate et al., Reference Kumkate, Jenkins, Paveley, Hogg and Mountford2007; Hambrook and Hanington, Reference Hambrook and Hanington2021), which contribute to a type 1 cellular immune response (He et al., Reference He, Salafsky and Ramaswamy2005; Perona-Wright et al., Reference Perona-Wright, Jenkins and MacDonald2006).
Initial immune responses are activated as a result of excretory/secretory (E/S) products released by the cercariae penetrating glands at the time of penetration into the host's skin (Salter et al., Reference Salter, Lim, Hansell, Hsieh and McKerrow2000; Jenkins et al., Reference Jenkins, Hewitson, Ferret-Bernard and Mountford2005a, Reference Jenkins, Hewitson, Jenkins and Mountford2005b; Curwen et al., Reference Curwen, Ashton, Sundaralingam and Wilson2006; Paveley et al., Reference Paveley, Aynsley, Cook, Turner and Mountford2009). E/S products assist in the immunomodulatory function exerted by cercariae, as well as condition the remodelling of the extracellular matrix, facilitating its penetration into the skin (Janssen et al., Reference Janssen, Silva Santos, Muller, Vieira, De Campos and De Paulo Martins2016; Leontovyč et al., Reference Leontovyč, Ulrychová, Horn and Dvořák2020). Liu et al. (Reference Liu, Ju, Du, Shen, Wang, Li, Zhang, Feng and Hu2015) performed a proteomic analysis of products excreted by S. japonicum cercariae at the time of skin entry and identified a variety of E/S proteins, mainly proteases. Among the enzymes that allow this remodelling, the cercarial elastase of S. mansoni stands out, which is of great importance in the penetration of cercariae into the skin and can degrade a wide variety of macromolecules present in the human integument (Ingram et al., Reference Ingram, Rafi, Eroy-Reveles, Ray, Lambeth, Hsieh, Ruelas, Lim, Sakanari, Craik, Jacobson and McKerrow2012; El-Faham et al., Reference El-Faham, Wheatcroft-Francklow, Price, Sayers and Doenhoff2017).
Parasitic E/S products also promote the activation of prostaglandin E2 (PGE2) and prostaglandin D2-producing keratinocytes (Kaisar et al., Reference Kaisar, Ritter, del Fresno, Jónasdóttir, van der Ham, Pelgrom, Schramm, Layland, Sancho, Prazeres da Costa, Giera, Yazdanbakhsh and Everts2018; Oyesola et al., Reference Oyesola, Shanahan, Kanke, Mooney, Webb, Smita, Matheson, Campioli, Pham, Früh, McGinty, Churchill, Cahoon, Sundaravaradan, Flitter, Mouli, Nadjsombati, Kamynina, Peng, Cubitt, Gronert, Lord, Rauch, von Moltke, Sethupathy and Tait Wojno2021), which are molecules that induce the production of IL-10 via a cyclooxygenase 2-dependent pathway (Ramaswamy et al., Reference Ramaswamy, Kumar and He2000; Harizi et al., Reference Harizi, Juzan, Pitard, Moreau and Gualde2002; Xue et al., Reference Xue, Gyles, Wettey, Gazi, Townsend, Hunter and Pettipher2005). This type of response is responsible for modulating the immune response that favours parasite survival (Angeli et al., Reference Angeli, Faveeuw, Roye, Fontaine, Teissier, Capron, Wolowezuk, Capron and Trottein2001; Hervé et al., Reference Hervé, Angeli, Pinzar, Wintjens, Faveeuw, Narumiya, Capron, Urade, Capron, Riveau and Trottein2003; De Oliveira Fraga et al., Reference De Oliveira Fraga, Torrero, Tocheva, Mitre and Davies2010). Abdel-Ghany et al. (Reference Abdel-Ghany, Rabia, El-Ahwany, Saber, Gamal, Nagy, Mahmoud, Hamad and Barakat2015) suggested that blocking PGE2 might provide partial protection in S. mansoni-infected mice. In addition, during the period when cercariae transform into schistosomules and migrate through the skin, PGE2 acts as a potent vasodilator, helping the passage of these larval forms into circulation (Ruzicka and Printz, Reference Ruzicka and Printz1984).
After penetrating the host's skin, cercariae undergo morphological and biochemical changes, transforming into juvenile forms, known as schistosomula, that reach blood vessels (Brink et al., Reference Brink, McLaren and Smithers1977; Wilson, Reference Wilson1987; Curwen and Wilson, Reference Curwen and Wilson2003). In the bloodstream, the schistosomula is passively transported to the lungs and heart until they finally reach the hepatic portal system, where they develop into adult male or female worms (Miller and Wilson, Reference Miller and Wilson1978; Wheater and Wilson, Reference Wheater and Wilson1979; Nation et al., Reference Nation, Da'dara, Marchant and Skelly2020) (Fig. 1). In this phase before the parasite's oviposition (early stages of acute phase), the host produces a predominantly type 1 immune response, which reaches greater activation between the 3rd and 5th weeks after exposure to cercariae (Dunne and Cooke, Reference Dunne and Cooke2005; Gryseels et al., Reference Gryseels, Polman, Clerinx and Kestens2006). This response is characterized by high production of pro-inflammatory cytokines, such as IL-1, IL-2, IL-6, IL-12, interferon-gamma (IFN-γ) and TNF-α (Fig. 2) (Grzych et al., Reference Grzych, Pearce, Cheever, Caulada, Caspar, Heiny, Lewis and Sher1991; Pearce et al., Reference Pearce, Caspar, Grzych, Lewis and Sher1991; Egesa et al., Reference Egesa, Lubyayi, Tukahebwa, Bagaya, Chalmers, Wilson, Hokke, Hoffmann, Dunne, Yazdanbakhsh, Labuda and Cose2018; Zheng et al., Reference Zheng, Zhang, Chen, Nie, Miller, Gong and Liu2020). Coinciding with the migration and sexual maturation of adult worms, a systemic hypersensitivity reaction occurs in the host, called Katayama syndrome (for S. japonicum) or the toxaemic form (for S. mansoni), which is associated with an intense Th1 response (Neves, 1992; Ross et al., Reference Ross, Vickers, Olds, Shah and McManus2007; Caldas et al., Reference Caldas, Campi-Azevedo, Oliveira, Silveira, Oliveira and Gazzinelli2008; Langenberg et al., Reference Langenberg, Hoogerwerf, Janse, Van Lieshout, Corstjens, Roestenberg, Van Dam, Van Diepen, De Dood, Feijt, Ganesh, Gerritsma, Hardeman, Hokke, Koopman, Kos-Van Oosterhoud, Kruize, Meij, Ozir-Fazalalikhan, Van Schuijlenburg, Smits, Verbeek-Menken, Visser, De Vries, Winkel and Yazdanbakhsh2019). During primary infections in non-immune individuals, the main symptoms related to this systemic inflammation include a high fever accompanied by chills, profuse sweating, asthenia, myalgia, headache and a non-productive cough (Schwartz et al., Reference Schwartz, Rozenman and Perelman2000; Bottieau et al., Reference Bottieau, Clerinx, de Vega, Van den Enden, Colebunders, Van Esbroeck, Vervoort, Van Gompel and Van den Ende2006).
After parasite oviposition (between 5th and 6th weeks post-infection), there is a change in the profile of immune mediators produced by the host, and the immune response becomes predominantly Th2, which is associated with increasing production of IL-4, IL-5, IL-9 and IL-13 (MacDonald et al., Reference MacDonald, Straw, Dalton and Pearce2002; Pearce et al., Reference Pearce, Kane, Sun, Taylor, McKee and Cervi2004; Bartley et al., Reference Bartley, Ramm, Jones, Ruddell, Li and McManus2006; Burke et al., Reference Burke, Jones, Gobert, Li, Ellis and McManus2009). Such changes are responses to soluble egg antigens (SEAs) (Hams et al., Reference Hams, Aviello and Fallon2013), that is composed of a complex mixture of immunostimulatory antigens that are known for their ability to condition DCs to initiate the induction of a Th2 profile (Mouser et al., Reference Mouser, Pollakis, Smits, Thomas, Yazdanbakhsh, De Jong and Paxton2019).
DCs detect, capture and process antigens derived from eggs of S. mansoni (Cervi et al., Reference Cervi, MacDonald, Kane, Dzierszinski and Pearce2004; van Liempt et al., Reference van Liempt, van Vliet, Engering, García Vallejo, Bank, Sanchez-Hernandez, van Kooyk and van Die2007), resulting in their ability to lead to Th2 polarization both in vitro and in vivo (de Jong et al., Reference de Jong, Vieira, Kalinski, Schuitemaker, Tanaka, Wierenga, Yazdanbakhsh and Kapsenberg2002; MacDonald et al., Reference MacDonald, Straw, Dalton and Pearce2002; Perona-Wright et al., Reference Perona-Wright, Jenkins and MacDonald2006). The main antigens responsible for this potent induction of a Th2 response are glycoproteins omega 1 (ω-1) and IPSE (IL-4-inducing principle of S. mansoni eggs)/alpha 1 (α-1) (Schramm et al., Reference Schramm, Gronow, Knobloch, Wippersteg, Grevelding, Galle, Fuller, Stanley, Chiodini, Haas and Doenhoff2006; Meevissen et al., Reference Meevissen, Wuhrer, Doenhoff, Schramm, Haas, Deelder and Hokke2010). Glycoprotein ω-1 is present in both SEAs (Dunne et al., Reference Dunne, Jones and Doenhoff1991) and E/S products from live eggs (Cass et al., Reference Cass, Johnson, Califf, Xu, Hernandez, Stadecker, Yates and Williams2007), and activates DCs (via C-type and Toll-type lectin receptors), which in turn promotes Th2 differentiation, the main source of type 2 cytokines such as IL-4, IL-5 and IL-13 (Everts et al., Reference Everts, Perona-Wright, Smits, Hokke, van der Ham, Fitzsimmons, Doenhoff, van der Bosch, Mohrs, Haas, Mohrs, Yazdanbakhsh and Schramm2009). On the other hand, a previous study (Schramm et al., Reference Schramm, Gronow, Knobloch, Wippersteg, Grevelding, Galle, Fuller, Stanley, Chiodini, Haas and Doenhoff2006) showed that the glycoprotein IPSE/α-1 is exclusively released from mature eggs, but likely possesses the same potential to initiate a Th2 response during S. mansoni infection. IPSE/α-1 binds to immunoglobulin and activates basophils, leading to the release of histamine and facilitating the production of Th2-type cytokines, mainly IL-4 and IL-13 (Schramm et al., Reference Schramm, Mohrs, Wodrich, Doenhoff, Pearce, Haas and Mohrs2007; Meyer et al., Reference Meyer, Mayerhofer, Tripsianes, Blindow, Barths, Mewes, Weimar, Köhli, Bade, Madl, Frey, Haas, Mueller-Dieckmann, Sattler and Schramm2015; Knuhr et al., Reference Knuhr, Langhans, Nyenhuis, Viertmann, Overgaard Kildemoes, Doenhoff, Haas and Schramm2018). Thus, the Th2 response (Fig. 2) is related to low production of IFN-γ and high concentrations of anti-inflammatory cytokines (IL-4, IL-5, IL-10 and IL-13) (Grzych et al., Reference Grzych, Pearce, Cheever, Caulada, Caspar, Heiny, Lewis and Sher1991; Pearce et al., Reference Pearce, Kane, Sun, Taylor, McKee and Cervi2004; Zheng et al., Reference Zheng, Zhang, Chen, Nie, Miller, Gong and Liu2020).
Mechanisms associated with macrophage polarization
Macrophages are cells of the innate immune system that have phagocytic capacity and are involved in the elimination of foreign particles from the body (Gordon and Martinez-Pomares, Reference Gordon and Martinez-Pomares2017; Uribe-Querol and Rosales, Reference Uribe-Querol and Rosales2020) and in the presentation of antigens, constituting an important link between innate and adaptive immunity. These cells are part of the mononuclear phagocytic system and are implicated in tissue homoeostasis and various infectious and inflammatory processes (Rahman et al., Reference Rahman, Vengrenyuk, Ramsey, Vila, Girgis, Liu, Gusarova, Gromada, Weinstock, Moore, Loke and Fisher2017; Shapouri-Moghaddam et al., Reference Shapouri-Moghaddam, Mohammadian, Vazini, Taghadosi, Esmaeili, Mardani, Seifi, Mohammadi, Afshari and Sahebkar2018).
Macrophages are activated during phagocytosis or by contact with molecular patterns associated with pathogenic microorganisms. This activation results in inflammatory responses and increased production of cytokines and/or physicochemical factors and, consequently, can differentiate into various phenotypes depending on the state and changes in the microenvironment (Schmall et al., Reference Schmall, Al-Tamari, Herold, Kampschulte, Weigert, Wietelmann, Vipotnik, Grimminger, Seeger, Pullamsetti and Savai2015; Murray, Reference Murray2017). There are 2 main subtypes of macrophages classified according to the expression of their cell surface markers, production of specific factors and biological activities: classically activated or inflammatory M1 macrophages and alternatively activated or anti-inflammatory M2 macrophages (Parisi et al., Reference Parisi, Gini, Baci, Tremolati, Fanuli, Bassani, Farronato, Bruno and Mortara2018) (Fig. 3). Macrophage subtypes play a role in the initiation and/or progression of many diseases. The M1/M2 paradigm emerged as homologous with the one previously described for Th response profiles, which also presents 2 subtypes: Th cell type 1 (Th1) and type 2 (Th2) (Mills, Reference Mills2015).
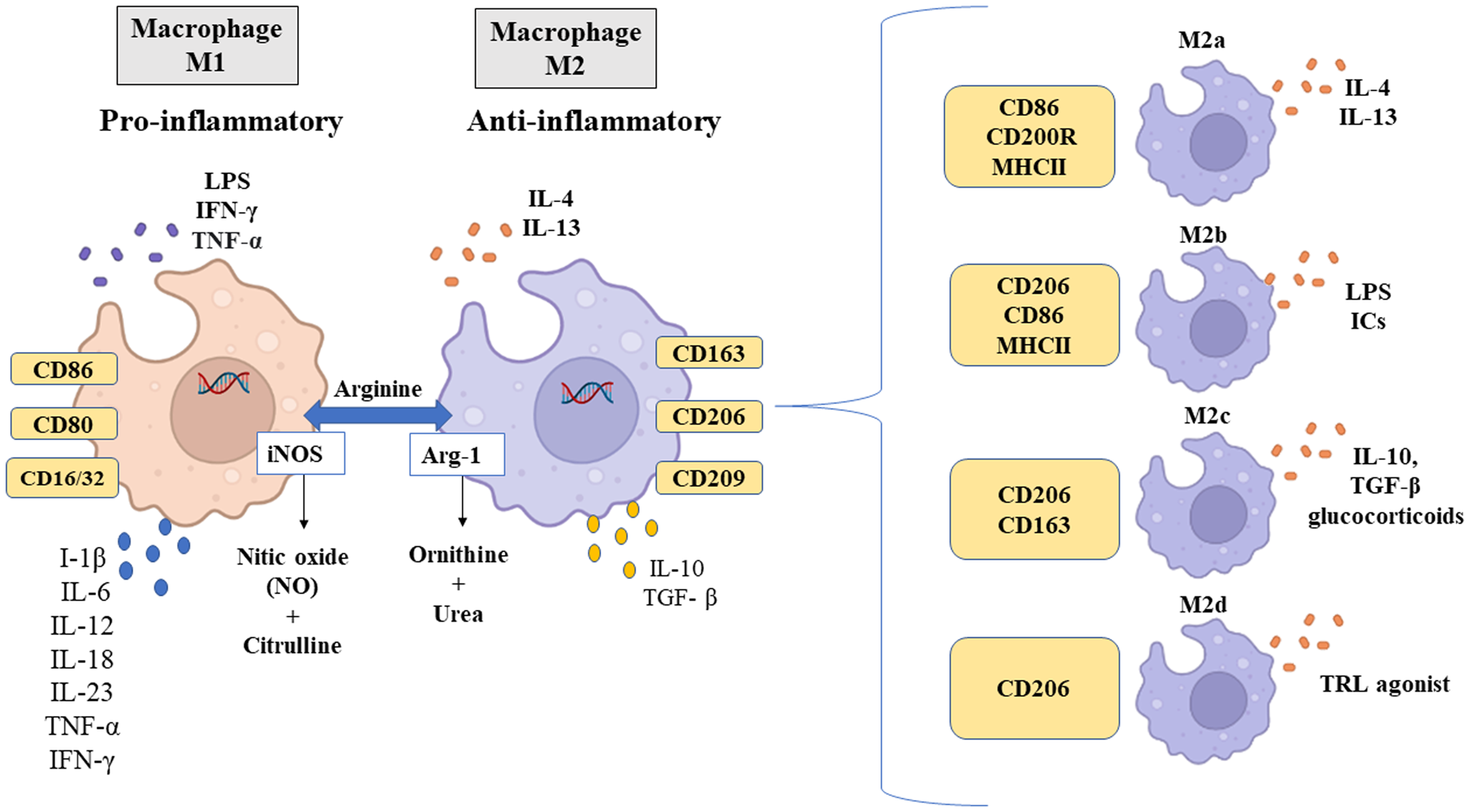
Fig. 3. Different macrophage phenotypes, specific stimuli and markers. Source: Created with BioRender.com.
M1 macrophage subtypes polarize in the presence of Th1 cytokines such as IFN-γ and TNF-α or when exposed to inflammatory molecules such as lipopolysaccharides (LPS) (Yunna et al., Reference Yunna, Mengru, Lei and Weidong2020), through the following mechanisms: (1) JAK/STAT (Janus kinase/signal transducer and transcriptional activator) signalling pathway. IFN-γ activates JAK-inducing phosphorylation of STAT1, which in turn leads to macrophage polarization to M1 (Wang et al., Reference Wang, Shang, Qi, Ba, Lv, Zhou, Wang, Shaxika, Wang and Ma2020); (2) Toll-like receptor (TLR) 4/nuclear factor κB (NF-κB) signalling pathway. LPS binds to TLR4 to activate NF-κB and activator protein 1 (AP-1), promoting the expression of inflammatory factors (Chen et al., Reference Chen, Tang, Fu, Wang, Han and Meng2017b; Ciesielska et al., Reference Ciesielska, Matyjek and Kwiatkowska2021) and (3) cytokine signalling through specific receptors that activate AP-1 (Liu et al., Reference Liu, Zou, Chai and Yao2014).
M1 macrophages are recruited soon after lesion formation and are mainly involved in the initial response to infectious processes (Vannella and Wynn, Reference Vannella and Wynn2017). These increase local inflammation, producing large amounts of pro-inflammatory cytokines, including IL-1β, IL-6, IL-12, IL-18, IL-23, TNF-α and IFN type 1 (Shapouri-Moghaddam et al., Reference Shapouri-Moghaddam, Mohammadian, Vazini, Taghadosi, Esmaeili, Mardani, Seifi, Mohammadi, Afshari and Sahebkar2018), as shown in Fig. 3. The M1 macrophage phenotype expresses high levels of inducible nitric oxide synthase (iNOS), major histocompatibility complex class II (MHC II), CD16/32, CD80 and CD86, as well as chemokines that attract Th1 cells, including CXCL9 and CXCL12 (Orecchioni et al., Reference Orecchioni, Ghosheh, Pramod and Ley2019). Functionally, M1 macrophages are characterized by antimicrobial and antitumour activities and participate in the elimination of infectious agents through the activation of nicotinamide adenine dinucleotide phosphate (NADPH) oxidase and, consequently, the generation of reactive oxygen species (ROS) (Murray et al., Reference Murray, Allen, Biswas, Fisher, Gilroy, Goerdt, Gordon, Hamilton, Ivashkiv, Lawrence, Locati, Mantovani, Martinez, Mege, Mosser, Natoli, Saeij, Schultze, Shirey, Sica, Suttles, Udalova, van, Vogel and Wynn2014).
On the other hand, M2 macrophages are induced by Th2 cytokines IL-4 and IL-13 (Fig. 3), mainly via STAT6 activation (Sica and Mantovani, Reference Sica and Mantovani2012; Enderlin et al., Reference Enderlin Vaz Da Silva, Lehr and Velin2014). This pathway is extremely important, as IL-4 inhibits M1 and induces M2 polarization (He et al., Reference He, Gao, Zhang, Zhou, Cao and Yao2020). Gao et al. (Reference Gao, Zhou, Liu, Wang, Gao, Wu, Zhao, Liu, Wang, Liu, Guo, Shen, Wu and Yuan2015) demonstrated that the expression of STAT6 was positively regulated by curcumin and by the secretion of IL-4 and IL-13, capable of inducing M0 and M1 macrophages to polarize into M2. IL-4 type I and type II receptors also activate STAT6 (Gong et al., Reference Gong, Zhuo and Ma2017), which in turn induces the transcription of typical M2 polarization genes, such as mannose receptor 1, type α resistin (Retnla) and chitinase 3-like 3 (Chi3l3, Ym1) (Martinez and Gordon, Reference Martinez and Gordon2014). M2 polarization can also be induced by IL-10 through STAT3 activation (Yin et al., Reference Yin, Ma, Lin, Lu, Zhang, Chen and Jian2018). However, the STAT6 pathway is considered to activate M2 macrophages (Murray, Reference Murray2017).
The M2 macrophage phenotype has a profile of anti-inflammatory cytokines, characterized by low production of IL-1, IL-6 and TNF-α, and high production of IL-10 and transforming growth factor-beta (TGF-β) (Fig. 3), as well as chemokines CCL1, CCL17, CCL18, CCL22 and CCL24 (Yunna et al., Reference Yunna, Mengru, Lei and Weidong2020). Additionally, this phenotype can be characterized by the expression of arginase 1 (Arg-1), CD163, CD209 and CD206. CD206 interacts with glycoproteins and glycolipids found on the surfaces of pathogens (Suzuki et al., Reference Suzuki, Shirai, Asada, Yasui, Karayama, Hozumi, Furuhashi, Enomoto, Fujisawa, Nakamura, Inui, Shirai, Hayakawa and Suda2018; Xu et al., Reference Xu, Gu, Wang, Jin, Wen, Ma, Tang, Mao, Qian and Lin2019). Thus, CD206 plays a role in immunological recognition of pathogens after antigen internalization and presentation (Hussell and Bell, Reference Hussell and Bell2014). Functionally, M2 macrophages can inhibit inflammation, promote tissue repair and wound healing, prevent parasitic infection and have proangiogenic and profibrotic properties (Jetten et al., Reference Jetten, Verbruggen, Gijbels, Post, De Winther and Donners2014; Braga et al., Reference Braga, Agudelo and Camara2015). Furthermore, because M2 macrophages produce complex cytokines and are characterized by the functional expression of alternative activation markers, they can be divided into 4 subtypes: M2a, M2b, M2c and M2d (Yao et al., Reference Yao, Xu and Jin2019). These subtypes differ from each other based on their cell surface markers, secreted cytokines and biological functions, as is demonstrated in Fig. 3.
M2a macrophages are induced by the cytokine IL-4 or IL-13 and express high levels of CD86, CD200R and MHC II and low levels of CD14 and TLR4 (Yao et al., Reference Yao, Xu and Jin2019). In addition to being major producers of CCL24, CCL17 and CCL22, they use CCR3 and CCR4 receptors, resulting in the recruitment of eosinophils, basophils and Th2 cells, promoting the upregulation of a type 2 immune response (Fraternale et al., Reference Fraternale, Brundu and Magnani2015). M2b-type macrophages are induced by immune complexes, LPS or IL-1 receptor antagonist and are characterized by increased expression of CD206 and CD86 (Viola et al., Reference Viola, Munari, Sánchez-Rodríguez, Scolaro and Castegna2019). Upon activation, this subtype secretes pro- and anti-inflammatory cytokines TNF-α, IL-1β, IL-6 and IL-10 and functions in regulating the immune response and inflammation (Wang et al., Reference Wang, Ye, Zeng and Qiao2019). M2c macrophages are induced by IL-10, TGF-β or glucocorticoids, and express CD206 and CD163, in addition to secreting IL-10, TGF-β, CCL16 and CCL18, which play crucial roles in the phagocytosis of apoptotic cells (Ross et al., Reference Ross, Devitt and Johnson2021). Finally, induced by TLR antagonists, M2d macrophages express high levels of CD206, IL-10 and iNOS, secrete CCL5, CXCL10 and CXCL6 and express low levels of IL-12 and TNF-α (Viola et al., Reference Viola, Munari, Sánchez-Rodríguez, Scolaro and Castegna2019). This subtype also secretes the vascular endothelial growth factor and promotes angiogenesis and tumour progression (Ferrante et al., Reference Ferrante, Pinhal-Enfield, Elson, Cronstein, Hasko, Outram and Leibovich2013). Notably, all subtypes of M2 macrophages express IL-10.
M1 and M2 macrophages can also be differentiated by the way they metabolize arginine, as shown in Fig. 3. M1 macrophages metabolize arginine by the enzyme iNOS to produce nitric oxide (NO) and citrulline; on the contrary, M2 macrophages metabolize arginine by Arg-1 to produce l-ornithine and urea, a precursor molecule of polyamines involved in tissue repair and cell proliferation (Rath et al., Reference Rath, Müller, Kropf, Closs and Munder2014; Yang and Ming, Reference Yang and Ming2014).
The 2 macrophage populations must be balanced to maintain homoeostasis and to protect the organism. Once an imbalance occurs, the exacerbated activity of M1 or M2 macrophages can lead to the development of inflammatory diseases or host immunosuppression (Sica et al., Reference Sica, Erreni, Allavena and Porta2015). However, the remarkable plasticity of macrophages confers significant benefits to the host, especially in the course of chronic helminth infections (Lechner et al., Reference Lechner, Bohnacker and Esser-von Bieren2021) since it limits excessive tissue damage when it is unable to overcome the initial injury. This feature has been well-documented in schistosomiasis.
Participation of M1 and M2 macrophages in the response to Schistosoma infection
Initially, blood monocytes differentiate into macrophages at inflammatory sites (Rückerl and Cook, Reference Rückerl and Cook2019) and exhibit high plasticity as a result of exposure to various stimuli, signalling molecules, nutrients and metabolites in the context of schistosomiasis (Cortes-Selva and Fairfax, Reference Cortes-Selva and Fairfax2021). These phagocytes can exert pro-inflammatory or anti-inflammatory functions (Zhu et al., Reference Zhu, Xu, Chen, Zhou, Zhang, Chi, Li, Song, Liu and Su2014) in different clinical forms of schistosomiasis (acute and chronic phases) (Fig. 2). In the acute phase, macrophages secrete pro-inflammatory cytokines and consequently increase inflammation, recruit more immune cells and promote the formation of the initial granuloma. In the chronic phase, macrophages have an immunoregulatory activity to decrease the damage caused by granulomas (Wolde et al., Reference Wolde, Laan, Medhin, Gadissa, Berhe and Tsegaye2020).
During the life cycle of S. mansoni and S. japonicum, several antigens are excreted by their different evolutive forms (Curwen et al., Reference Curwen, Ashton, Sundaralingam and Wilson2006; Jang-Lee et al., Reference Jang-Lee, Curwen, Ashton, Tissot, Mathieson, Panico, Dell, Wilson and Haslam2007; Acharya et al., Reference Acharya, Da'dara and Skelly2021). For example, Sm16 – a low molecular weight protein that is secreted by S. mansoni cercariae, helps the parasite to enter the host's skin (Brännström et al., Reference Brännström, Sellin, Holmfeldt, Brattsand and Gullberg2009; Sanin and Mountford, Reference Sanin and Mountford2015). Sm29, present in the tegument of schistosomula and adult S. mansoni worms, can induce the maturation and activation of human monocyte-derived DCs (Cardoso et al., Reference Cardoso, Macedo, Gava, Kitten, Mati, de Melo, Caliari, Almeida, Venancio, Verjovski-Almeida and Oliveira2008; Lopes et al., Reference Lopes, Oliveira, Page, Carvalho, Carvalho and Cardoso2019). Sj-C is an example of a protein secreted from the tegument of S. japonicum, which may suppress the presentation of exogenous antigens by DCs (He et al., Reference He, Cai, Ni, Li, Zong and He2011; Chen et al., Reference Chen, He, Hou and He2017a). IPSE/α-1 and ω-1 are examples of proteins secreted by S. mansoni eggs, which help direct a Th2 response (Everts et al., Reference Everts, Perona-Wright, Smits, Hokke, van der Ham, Fitzsimmons, Doenhoff, van der Bosch, Mohrs, Haas, Mohrs, Yazdanbakhsh and Schramm2009; Knuhr et al., Reference Knuhr, Langhans, Nyenhuis, Viertmann, Overgaard Kildemoes, Doenhoff, Haas and Schramm2018). These molecules can induce the activation and modulation of innate and adaptive immune responses and facilitate the evasion of the parasite from the host-defense mechanisms (Jenkins et al., Reference Jenkins, Hewitson, Ferret-Bernard and Mountford2005a, Reference Jenkins, Hewitson, Jenkins and Mountford2005b; Hai et al., Reference Hai, Edwards, Van Zandt, Hoffmann and Christianson2014; Hambrook and Hanington, Reference Hambrook and Hanington2021). Schistosoma antigens can be proteins (such enzymes), polysaccharides and the most commonly used are crude extracts prepared by breaking up worms, larvae or eggs (Doenhoff et al., Reference Doenhoff, Butterworth, Hayes, Sturrock, Ouma, Koech, Prentice and Bain1993; Doenhoff, Reference Doenhoff1998). Thus, it is clear that antigen changes in the microenvironment during schistosomiasis are important for the polarization of macrophages to the M1 or M2 profile (Xu et al., Reference Xu, Zhang, Chen, Zhang, Ji, Wu and Wu2014; Sanin and Mountford, Reference Sanin and Mountford2015; Assunção et al., Reference Assunção, Magalhães, Carneiro, Molinaro, Almeida, Atella, Castro-Faria-Neto and Bozza2017).
According to Tables 1 and 2, we highlight some in vitro and in vivo studies that demonstrate the relationship between the stimulation of S. japonicum and S. mansoni antigens and macrophage polarization. In addition, we also highlight other molecules involved in macrophage polarization in schistosomiasis, providing molecular evidence of great relevance in the process of differentiation of these cells, which will be discussed in this article.
Table 1. Molecules and/or antigens involved in macrophage polarization in Schistosoma mansoni infection

Table 2. Molecules and/or antigens involved in macrophage polarization in Schistosoma japonicum infection

Cercariae and schistosomula antigens can induce an M1 profile
During the penetrating of the human skin, cercariae of S. mansoni and S. japonicum release E/S products, which have remodelling and immunoregulatory functions (Liu et al., Reference Liu, Ju, Du, Shen, Wang, Li, Zhang, Feng and Hu2015; Sanin and Mountford, Reference Sanin and Mountford2015), that facilitate their penetration and subsequent establishment in the host's body, in the form of schistosomula (Janssen et al., Reference Janssen, Silva Santos, Muller, Vieira, De Campos and De Paulo Martins2016). This phase represents the first contact with innate immune responses in the skin, especially Langerhans cells, which are considered tissue-resident macrophages (West and Bennett, Reference West and Bennett2018). These cells phagocytize E/S and secrete pro-inflammatory (IL-6 and IL-12p40) and anti-inflammatory (IL-10) cytokines in a TLR-dependent manner (Jenkins et al., Reference Jenkins, Hewitson, Ferret-Bernard and Mountford2005a, Reference Jenkins, Hewitson, Jenkins and Mountford2005b).
One of the ways in which macrophages are activated is through the action of TLRs. These receptors are a family of pattern recognition receptors that are important for innate immune response (El-Zayat et al., Reference El-Zayat, Sibaii and Mannaa2019). These receptors recognize invading pathogens, trigger innate immune responses and subsequently initiate adaptive immunity against infections, including Gram-positive and Gram-negative bacteria, fungi, viruses and parasites (Lu et al., Reference Lu, Li, Liu, Zhang and Zhang2018). These receptors mediate macrophage recognition by microbial ligands, inducing the expression of microbicidal molecules and cytokines via the adapter protein MyD88 (Jin et al., Reference Jin, Yuan, Chen, Wu, Gong, Yuan, Zeng, Pei and Chen2019). Xu et al. (Reference Xu, Zhang, Chen, Zhang, Ji, Wu and Wu2014) showed that normal cercariae antigen (NCA) and attenuated cercariae antigen (ACA) from S. japonicum induced polarization to the M1 profile, with increased levels of IL-12, CD136/32 and iNOS (Table 2). However, these values decreased when the TLR4 pathway blockers were used. Thus, the authors suggested that the polarization of the M1 profile is dependent on the TLR4 pathway and this may play a protective role in S. japonicum infection (Tang et al., Reference Tang, Shen, Hong, Zhang, Zhai, Fu, Li, Lu and Lin2021).
In fact, the TLR4 pathway is extremely important for the polarization of macrophages to the M1 phenotype, as demonstrated in some studies (Freitas et al., Reference Freitas, Oliveira, Da Silva, Fernandes, Gonçales, Almeida and Roque-Barreira2016; Shi et al., Reference Shi, Luo, Wang, Horst, Bläsius, Relja, Xu, Hildebrand and Greven2020). Sanin and Mountford (Reference Sanin and Mountford2015) demonstrated that Sm16 (a molecule produced by S. mansoni cercariae) is able to block TLR4 and TLR3 pathways in human monocyte, which negatively affect the classic activation of macrophages (M1) in response to IFN-γ (Table 1). This is considered an important mechanism of immune evasion promoted by S. mansoni because it limits the production of NO, which is toxic to the parasite (Shiels et al., Reference Shiels, Cwiklinskiid, Alvarado, Thivierge, Cotton, Santana, Toid, Donnelly, Taggart, Weldonid and Dalton2020).
After complete transformation from a cercariae into a schistosomula, the larva migrates into the bloodstream, travelling through the lungs until reaching maturation in the mesenteric veins. This stage of the cycle is also characterized as a key target for the elimination of infection through innate host immune responses (Houlder et al., Reference Houlder, Costain, Cook and MacDonald2021). Some histological studies conducted in the lungs of mice infected with S. mansoni and S. japonicum showed inflammatory foci consisting of neutrophils, eosinophils and macrophages (Crabtree and Wilson, Reference Crabtree and Wilson1986; Burke et al., Reference Burke, McGarvey, McSorley, Bielefeldt-Ohmann, McManus and Gobert2011).
Macrophages function as cytotoxic cells, mainly in schistosomula (James and Glaven, Reference James and Glaven1989). Oswald et al. (Reference Oswald, Wynn, Sher and James1994) demonstrated that macrophages could produce NO, leading to schistosomula death in animal models independent of the production of pro-inflammatory cytokines. In contrast, Cardoso et al. (Reference Cardoso, Macedo, Gava, Kitten, Mati, de Melo, Caliari, Almeida, Venancio, Verjovski-Almeida and Oliveira2008) determined that the antigen Sm29, present in the integument of S. mansoni schistosomula, induced a Th1-type immune response, with an increase in pro-inflammatory cytokines (IFN-γ, TNF and IL-12) in mice, leading to a reduction in worm burden and liver pathology. James et al. (Reference James, Cheever, Caspar and Wynn1998) demonstrated that IFN-γ was a cytokine of great importance in the activation of macrophages in the lungs for the immunological killing of S. mansoni larvae and played a critical role in protective immunity.
In the early stages of schistosomiasis, lung macrophages may have an M1 phenotypic trait. Menson and Wilson (Reference Menson and Wilson1990) characterized the expression of surface markers in alveolar macrophages associated with the immune response to S. mansoni. The authors demonstrated an increase in IFN-γ expression in the lungs of C57BL/6 mice and suggested that activated macrophages might be responsible for initiating and maintaining focal inflammation that blocks parasite migration (Table 1).
Worm antigens can induce an M1 or M2 profile
During the acute phase of Schistosoma infection, before parasite oviposition (approximately 5–7 weeks post-infection), immune responses are largely of the CD4+ Th1 type, associated with increased numbers of M1 macrophages that produce IL-12, IL-6, TNF-α and NO (Pearce et al., Reference Pearce, Caspar, Grzych, Lewis and Sher1991; Coulson et al., Reference Coulson, Smythies, Betts, Mabbott, Sternberg, Wei, Liew and Wilson1998; Gordon, Reference Gordon2003). These early pro-inflammatory responses are mainly related to the antigens from immature worms (schistosomula) during their migration (Wilson, Reference Wilson1998; Egesa et al., Reference Egesa, Lubyayi, Tukahebwa, Bagaya, Chalmers, Wilson, Hokke, Hoffmann, Dunne, Yazdanbakhsh, Labuda and Cose2018). Activation of these responses may be through binding to TLR and C-type lectin receptors on macrophages; however, further studies are needed to clarify this mechanism of macrophage activation via schistosomula antigens.
In contrast to the schistosomula antigens, the adult worm antigen preparations [soluble worm antigen (SWAP or SWA)] were better explored in experimental studies. Although the antigenic composition is not the same as the live worm, the use of SWAP or SWA constitutes a valuable experimental tool to evaluate many aspects of immune responses promoted by different host cells (Xu et al., Reference Xu, Zhang, Chen, Zhang, Ji, Wu and Wu2014; Zhu et al., Reference Zhu, Xu, Chen, Zhou, Zhang, Chi, Li, Song, Liu and Su2014). This antigen is the easiest to obtain and is essentially an extract based on Tris-HCl or phosphate-buffered saline from mixed male and female worms and prepared in various ways, either by homogenization, sonication or freeze/thaw (or a combination of these) (Grenfell et al., Reference Grenfell, Martins, Silva-Moraes, Barata, Ribeiro, Oliveira and Coelho2012; Neves et al., Reference Neves, Sanson, Wilson and Castro-Borges2015). Some studies have demonstrated that SWAP could induce an M1-like profile (Xu et al., Reference Xu, Zhang, Chen, Zhang, Ji, Wu and Wu2014; Zhu et al., Reference Zhu, Xu, Chen, Zhou, Zhang, Chi, Li, Song, Liu and Su2014). Thus, Zhu et al. (Reference Zhu, Xu, Chen, Zhou, Zhang, Chi, Li, Song, Liu and Su2014), when performing a co-culture of peritoneal macrophages obtained from mice with S. japonicum SWA (Table 2), observed that there was an increase in the expression of specific markers related to M1 (TNF-α, IL-12, CXCL9, CXCL10, CXCL11 and iNOS).
Aiming to understand which mechanisms lead SWAP to induce polarization of the M1 profile, Shen et al. (Reference Shen, Wang, Huang, Zhu, Sun and Duan2021) demonstrated that this antigen promoted the expression of a protein called lipocalin 2 (LCN2) and, consequently, induced the M1 profile of macrophages (Table 2) through the upregulation of the NF-κB signalling pathway. It has already been reported that this protein is increased in macrophages and can potentiate the M1 phenotype of microglia in the central nervous system (Jang et al., Reference Jang, Lee, Kim, Kim, Seo, Lee, Mori, Nakao and Suk2013). The NF-κB signalling pathway can activate macrophages to produce M1 polarization upon LPS induction (Liu et al., Reference Liu, Zhang, Tan, Xu, Zhou, Luo, Li, Huang and Zeng2017). In addition, some studies have shown that this pathway could regulate the expression of LCN2, thereby stimulating the inflammatory response in infectious processes (Zhao and Stephens, Reference Zhao, Elks and Stephens2014; Ghosh et al., Reference Ghosh, Shang, Yazdankhah, Bhutto, Hose, Montezuma, Luo, Chattopadhyay, Qian, Lutty, Ferrington, Zigler and Sinha2017).
In addition to the antigens of adult worms that induce an M1 profile, studies have shown that adult worms of S. mansoni and S. japonicum also release extracellular vesicles (EVs), known as exosomes, which modulate the host immune response (Nowacki et al., Reference Nowacki, Swain, Klychnikov, Niazi, Ivens, Quintana, Hensbergen, Hokke, Buck and Hoffmann2015; Wang et al., Reference Wang, Altinoglu, Takeda and Xu2015; Sotillo et al., Reference Sotillo, Pearson, Potriquet, Becker, Pickering, Mulvenna and Loukas2016; Zhu et al., Reference Zhu, Wang, Lin, Jiang, Cui, Wang, Zhang and Pan2016). Exosomes are membrane-bound vesicles secreted by various types of mammalian cells in normal and diseased states (Avni and Avni, Reference Avni and Avni2021). Exosomes play an important role in cell–cell communication and have been implicated in the regulation of cell development, immune regulation, angiogenesis and cell migration (Raposo and Stoorvogel, Reference Raposo and Stoorvogel2013; Zhu et al., Reference Zhu, Wang, Lin, Jiang, Cui, Wang, Zhang and Pan2016). Wang et al. (Reference Wang, Altinoglu, Takeda and Xu2015) observed that RAW264.7 macrophages, when cultured with exosome-like vesicles isolated from S. japonicum, exhibited an M1 profile (Table 2), due to the increase in the surface markers CD16/32, iNOS and TNF-α. Liu et al. (Reference Liu, Zhu, Wang, Qiu, Chen, Davis and Cheng2019) investigated miRNAs from S. japonicum EVs and found that they increased macrophage proliferation in vitro (RAW264.7) and in vivo (mice and rabbits) as well as TNF-α expression. miRNAs are involved in the regulation of the development, differentiation and activation of immune cells, including macrophages (Montagner et al., Reference Montagner, Dehó and Monticelli2014; Mehta and Baltimore, Reference Mehta and Baltimore2016). Thus, the polarization of M1 induced by schistosome EVs may represent an important mechanism for parasite survival in vertebrate hosts, via modulation of the immune response. However, there are still controversies about the possible role of the schistosome tegument as a source of EVs, because, to date, no study has been performed to prove the exact origin of these vesicles (Wilson and Jones, Reference Wilson and Jones2021).
On the other hand, adult worm products can also bias the M2 profile (Smith et al., Reference Smith, Forman, Mair and Else2018). Indeed, Xu et al. (Reference Xu, Zhang, Chen, Zhang, Ji, Wu and Wu2014) showed that adult S. japonicum worms could induce an M2 macrophage profile. The authors, when stimulating RAW264.7 macrophages with SWAP from S. japonicum, observed an increase in the expression of surface markers (CD16/32 and CD206) and in the production of cytokines (IL-12 and IL-10), suggesting that this antigen could induce both M1 and M2 macrophage profiles. The potential explanation for this could be related to how the antigens of adult worms were obtained since some adult female worms possess eggs in the process of maturation into their uterus/ootype, and consequently, this antigen could have been contaminated with SEAs. However, further studies are needed to understand macrophage polarization by SWAP and its relationship with SEA contamination.
Besides the classical macrophage polarization (M1 and M2), products excreted by schistosomes, such as haemozoin, are also able to induce immunomodulation. Adult worms of S. mansoni acquire nutrients by haematophagy of the host's blood, and this process can form toxic haem for the parasite (Zussman et al., Reference Zussman, Bauman and Petruska1970). However, the schistosomes are able to neutralize the free haem in their intestine through crystallization in haemozoin (Oliveira et al., Reference Oliveira, D'Avila, Torres, Oliveira, Tempone, Rumjanek, Braga, Silva, Dansa-Petretski, Oliveira, De Souza and Ferreira2000). This haemozoin is regurgitated by the worms into the host bloodstream and can be accumulated in the liver (Kloetzel and Lewert, Reference Kloetzel and Lewert1966), which may activate the immune response of the host. From this perspective, a previous study (Truscott et al., Reference Truscott, Evans, Gunn and Hoffmann2013) highlighted that haemozoin formed from S. mansoni is able to maintain the M2 macrophage profile previously activated by IL-4 stimulation, but also exerts specific modulatory effects on these cells (Table 1). These authors showed that haemozoin mediated the suppression of Retnla (resistin-like molecule-α or Fizz1) expression and Retnla protein secretion in the M2 macrophages. The role of Retnla during experimental schistosomiasis is associated with the limitation of Th2 inflammatory response (Pesce et al., Reference Pesce, Ramalingam, Wilson, Mentink-Kane, Thompson, Cheever, Urban, Wynn and Mansfield2009). However, further studies are necessary to better explain the possible impact of haemozoin in the immunopathology of schistosomiasis.
SEAs can induce an M2 profile
After maturation of the adult worms and subsequent oviposition, the activation of a type 2 profile begins in response to the soluble antigens secreted by the eggs of S. mansoni and S. japonicum (Tables 1 and 2) (Cheever et al., Reference Cheever, Hoffmann and Wynn2000; Pearce and MacDonald, Reference Pearce and MacDonald2002; Pearce et al., Reference Pearce, Kane, Sun, Taylor, McKee and Cervi2004; Burke et al., Reference Burke, Jones, Gobert, Li, Ellis and McManus2009; Costain et al., Reference Costain, MacDonald and Smits2018). The type 2 profile of schistosomiasis is characterized by the expansion of Th2 cells, eosinophils and basophils, and increased production of IL-4, IL-5 and IL-13 (Hams et al., Reference Hams, Aviello and Fallon2013; Schwartz et al., Reference Schwartz, Oeser, Prazeres da Costa, Layland and Voehringer2014), as previously described. IL-4 and IL-13 protect hosts against various helminth parasites by signalling through the IL-4Rα chain (Barron and Wynn, Reference Barron and Wynn2011; Jenkins et al., Reference Jenkins, Ruckerl, Cook, Jones, Finkelman, Van Rooijen, MacDonald and Allen2011). The production of these cytokines reduces the inflammation levels produced by the type 1 profile of the initial stage of acute phase, preventing acute pathology, such as intestinal haemorrhage and liver damage; however, the Th2 immune response is responsible for the formation of hepatic and intestinal granulomas (Brunet et al., Reference Brunet, Finkelman, Cheever, Kopf and Pearce1997; Hams et al., Reference Hams, Aviello and Fallon2013; Zheng et al., Reference Zheng, Zhang, Chen, Nie, Miller, Gong and Liu2020).
Granulomas are essential for sequestering toxic antigens produced by eggs and preventing further tissue damage. However, if unregulated by the immune response of the host, granulomas grow excessively and progress to fibrotic stages, which are responsible for severe forms of the disease, such as cirrhosis, portal hypertension, liver failure and even host death (Lenzi et al., Reference Lenzi, Kimmel, Schechtman, Pelajo-Machado, Romanha, Pacheco, Mariano and Lenzi1998; Cheever et al., Reference Cheever, Hoffmann and Wynn2000; Takaki et al., Reference Takaki, Rinaldi, Berriman, Pagán and Ramakrishnan2021). Macrophages are one of the main cellular components of hepatic granulomas (Beljaars et al., Reference Beljaars, Schippers, Reker-Smit, Martinez, Helming, Poelstra and Melgert2014; Schwartz and Fallon, Reference Schwartz and Fallon2018). Recent studies have demonstrated that M2 macrophages play a direct and critical role in fibrosis, granuloma maintenance, tissue repair and host survival (Cortes-Selva et al., Reference Cortes-Selva, Elvington, Ready, Rajwa, Pearce, Randolph and Fairfax2018; Song et al., Reference Song, Yin, Mu, Li, Gao, Zhang, Dong, Mei and Hua2020). Ye et al. (Reference Ye, Huang, Zhang, Mei, Zheng, Li, Chen and Lu2020) showed that M2 macrophage markers (CD200R, Arg-1 and Ym1) were increased in the liver, spleen, large intestine and peritoneal macrophages of S. japonicum-infected mice. Jenkins et al. (Reference Jenkins, Ruckerl, Cook, Jones, Finkelman, Van Rooijen, MacDonald and Allen2011) observed that IL-4/IL-13 signalling via IL-4Rα induces an alternative phenotype in resident macrophages. In this sense, a study performed with macrophages derived from the bone marrow of mice infected with S. mansoni demonstrated that the tumour suppressor gene p16 INK4a was an excellent modulator of the activation and polarization of macrophages induced by IL-4 through the JAK2–STAT1 pathway (Cudejko et al., Reference Cudejko, Wouters, Fuentes, Hannou, Paquet, Bantubungi, Bouchaert, Vanhoutte, Fleury, Remy, Tailleux, Chinetti-Gbaguidi, Dombrowicz, Staels and Paumelle2011).
Egg antigens induce granulomas, consisting mainly of M2 macrophages (Yu et al., Reference Yu, Wang, Wang, Gu, Lei, Tang, Wei, Xu, Wang, Chen, Pu, Qi, Yu, Chen, Zhu, Li, Zhang, Zhou and Su2021). Zhu et al. (Reference Zhu, Xu, Chen, Zhou, Zhang, Chi, Li, Song, Liu and Su2014) showed that peritoneal macrophages obtained from healthy mice, when stimulated with S. japonicum SEAs, expressed high levels of chemokines (CCL2, CCL17 and CCL22), IL-10 and Arg-1. Similarly, Xu et al. (Reference Xu, Zhang, Chen, Zhang, Ji, Wu and Wu2014), after stimulating RAW264.7 macrophages with S. japonicum SEAs, also observed higher levels of IL-10. In chronic schistosomiasis infection, the main function of IL-10 is to control liver damage and regulate antifibrotic processes (Dewals et al., Reference Dewals, Marillier, Hoving, Leeto, Schwegmann and Brombacher2010; Kamdem et al., Reference Kamdem, Moyou-Somo, Brombacher and Nono2018). Previous studies have shown that low levels of IL-10 expression are related to liver fibrosis in S. mansoni-infected patients (Mutengo et al., Reference Mutengo, Mduluza, Kelly, Mwansa, Kwenda, Musonda and Chipeta2018). On the other hand, little is known about the mechanisms by which an SEA preferentially induces M2 macrophage differentiation. Previous studies have demonstrated that an SEA from S. mansoni could induce the expression of the notch Jagged1 ligand in mice and human macrophages, suggesting that Jagged1 might have a specific role in the M2 polarization process of macrophages (Goh et al., Reference Goh, Irvine, Lovelace, Donnelly, Jones, Brion, Hume, Kotze, Dalton, Ingham and Sweet2009) (Table 1). Macrophages found in liver tissues exhibit functional M2 polarization, which is dependent on the activation of notch1/Jagged1 signalling (Zheng et al., Reference Zheng, Zhang, Chen, Zheng, Zheng and Weng2016).
During S. mansoni infection, basophils detect egg IPSE/α-1 glycoprotein and stimulate the production of IL-4 and IL-13, which trigger the alternative activation of human monocytes (Table 1), as observed by the increased expression of CD206 and CD209 (Knuhr et al., Reference Knuhr, Langhans, Nyenhuis, Viertmann, Overgaard Kildemoes, Doenhoff, Haas and Schramm2018). IL-13 is a key cytokine that induces M2 macrophage polarization via the IL-13α1 signalling pathway (Chiaramonte et al., Reference Chiaramonte, Schopf, Neben, Cheever, Donaldson and Wynn1999; Liu et al., Reference Liu, Munker, Müllenbach and Weng2012). Li et al. (Reference Li, Chen, Dang, Wang, Shang, Ma, Wang, Zhang, Luo, Li and Zhao2017) performed a study with corilagin, an active component of many medicinal plants, and found that this component could suppress Schistosoma egg-induced liver fibrosis by inhibiting M2 macrophage polarization (Table 1) in the IL-13Rα1 signalling pathway. Corilagin has great potential to reduce liver fibrosis caused by egg antigens in S. japonicum infection by decreasing the expression of molecules associated with the IL-13/STAT6 signalling pathway in liver M2 macrophages (Du et al., Reference Du, Ma, Zhu, Li, Wang, Li, Chen, Shang, Zhang and Zhao2016).
Signaling via TLR2 may be another way egg antigens polarize M2 macrophages during schistosomiasis. Gong et al. (Reference Gong, Huang, Sun, Yu, Zhang, Xu, Shen and Cao2018) showed that antigens derived from S. japonicum eggs could activate macrophages, which exhibit M2b polarization dependent on NF-κB signalling, mediated by the MyD88/mitogen-activated protein kinase (MAPK) pathway in a TLR2-dependent manner (Table 1). In contrast, Tundup et al. (Reference Tundup, Srivastava, Nagy and Harn2014) showed that the CD14 TLR co-receptor was upregulated in hepatic macrophages after S. mansoni infection and acted as a crucial negative regulator of M2 polarization, possibly as part of a parasitic defense mechanism against granuloma formation (Table 1). Gao et al. (Reference Gao, Chen, Hou, Chen, Ji, Wu and Wu2013) observed that an SEA of S. japonicum, known as SjEA, upregulated programmed death ligand 2 (PD-L2) expression in mouse bone marrow-derived macrophages (BMDCs) via TLR2, which binds PD-1 primarily on CD4+ T cells. This mechanism can help inhibit the T cell response during S. japonicum infection.
Lysophosphatidylcholine (LPC) from S. mansoni eggs can also induce macrophage differentiation into the M2 phenotype (Assunção et al., Reference Assunção, Magalhães, Carneiro, Molinaro, Almeida, Atella, Castro-Faria-Neto and Bozza2017), as shown in Table 1. The authors observed that LPC from S. mansoni activates peroxisome proliferator-activated receptor gamma (PPAR-γ), a transcription factor necessary for M2 polarization, leading to higher expression of Arg-1 and CD206, while increasing the production of IL-10, TGF-β and PGE2 in peritoneal macrophages in vitro. Schistosoma mansoni eggs induced a 7-fold increase in PPAR-γ expression in human liver cell cultures (Anthony et al., Reference Anthony, Mathieson, de Castro-Borges and Allen2010). PPAR-γ, in addition to being of great importance in M2 polarization, can regulate lipid uptake and metabolism (Ahmadian et al., Reference Ahmadian, Suh, Hah, Liddle, Atkins, Downes and Evans2013; Abdalla et al., Reference Abdalla, Napimoga, Lopes, de Macedo Maganin, Cunha, Van Dyke and Clemente Napimoga2020).
Fang et al. (Reference Fang, Wu, Chen, Wu, Yang, Guo, Chen and Wang2015) showed that BMDCs from C57BL/6 mice, when stimulated with a specific S. japonicum egg protein known as SjE16.7, promoted the production of pro- (IL-12, IL-6 and TNF-α) and anti-inflammatory (IL-10) cytokines through the phosphorylation of MAPKs and increased the expression of MHC II on the surface of macrophages. Previous studies have shown that S. mansoni and S. japonicum egg antigens could stimulate the MAPK pathway in macrophages (Wang et al., Reference Wang, Yang, Li, Yu, Brindley, McManus, Wei, Han, Feng, Li and Hu2006; de Andrade et al., Reference de Andrade, de Mourão, Geraldo, Coelho, Silva, Neves, Volpini, Machado-Silva, Araujo, Nacif-Pimenta, Caffrey and Oliveira2014). MAPKs are essential transmitters of extracellular signals that can mediate key cellular processes, including cell differentiation, division and death (Yang et al., Reference Yang, Sharrocks and Whitmarsh2003). Thus, SjE16.7 is a potent macrophage activator. However, in another study, Shen et al. (Reference Shen, Wang, Peng, Liu, Zhang, Zhou, Sun and Wu2019), when using the Sj16 antigen, noticed that it decreased hepatic granulomas in mice infected with S. japonicum and associated this improvement with the suppression of cytokine production, such as IFN-γ, TNF-α, IL-4 and IL- 6. The authors reported that the mechanisms of Sj16 attenuation of hepatic granulomatous inflammation and fibrosis in these infected mice might be related to the induction of macrophages for M2 polarization (Table 2). These authors also demonstrated, by flow cytometry, the increase in the expression of CD206 after stimulation of Sj16 in peritoneal macrophages and leucocytes from the livers of mice. Corroborating these results, Hu et al. (Reference Hu, Wu, Yang and Fung2009) showed that Sj16 decreased the levels of pro-inflammatory cytokines, such as IL-6 and TNF-α, and increased the levels of IL-10 in RAW264.7 macrophages. Vannella et al. (Reference Vannella, Barron, Borthwick, Kindrachuk, Narasimhan, Hart, Thompson, White, Cheever, Ramalingam and Wynn2014) observed that mice infected with S. mansoni showed an increase in M2 macrophages that expressed Arg-1, which attenuated the progression of inflammation and fibrosis (Table 1). Stimulation of RAW264.7 macrophages with another S. japonicum egg protein (SjCP1412) also increased the expression of CD206, Arg-1 and IL-10, which are related to M2-type macrophage differentiation (Ke et al., Reference Ke, Shen, Song, Yu, Kikuchi, Hirayama, Gao, Wang, Yin, Yao, Liu and Zhou2017). Overall, these findings emphasize that M2 macrophages are important in reducing the lesions caused by schistosomiasis through downregulation of the Th1 response and inflammation promoted by egg antigens. Additionally, the role of these cells was previously investigated in a mouse model of liver injury induced by acetaminophen (paracetamol) (Starkey Lewis et al., Reference Starkey Lewis, Campana, Aleksieva, Cartwright, Mackinnon, O'Duibhir, Kendall, Vermeren, Thomson, Gadd, Dwyer, Aird, Man, Rossi, Forrester, Park and Forbes2020). The authors demonstrated that the injection of M2 macrophages in this experimental model was able to rapidly reduce liver damage and inflammation. These data indicate that M2 macrophages may constitute a new potential cell-based therapy for this disease. Based on this, it seems promissory also to apply these cells in the immunotherapy of schistosomiasis.
Interestingly, despite being produced by M1 macrophages, a recent study demonstrated that the production of ROS by egg antigens may be a potential mechanism for M2 macrophage differentiation (Table 2). ROS have several biological activities, such as participation in innate and adaptive immune responses, and can be cytotoxic against pathogens (Canton et al., Reference Canton, Sánchez-Rodríguez, Spera, Venegas, Favia, Viola and Castegna2021). Yu et al. (Reference Yu, Wang, Wang, Gu, Lei, Tang, Wei, Xu, Wang, Chen, Pu, Qi, Yu, Chen, Zhu, Li, Zhang, Zhou and Su2021) observed that a significant increase in ROS in the liver of mice infected with S. japonicum was related to fibrosis and the differentiation of M2 macrophages. The authors hypothesized that their findings were due to NADPH oxidase (NOX2) inhibiting SEA-stimulated ROS production in macrophages, suggesting that NOX might act as the main source of ROS production in SEA-stimulated macrophages. NADPH oxidase is the first source of ROS identified in macrophages (Nathan et al., Reference Nathan and Cunningham-Bussel2013). Macrophages produce large amounts of ROS, primarily through NOX2 activation (Paik et al., Reference Paik, Kim, Aoyama, De Minicis, Bataller and Brenner2014). Thus, the production of ROS induced by schistosome eggs may be a target for the treatment of schistosomiasis.
Future perspectives and final considerations
Findings about the mechanisms behind macrophage activation during different metabolic profiles in human diseases present an exciting prospect, as there are pathologies that have been associated with a particular macrophage phenotype. In this context, the polarization of macrophages in schistosomiasis and their consequent ability to promote an effective immune response seem to be an attractive therapeutic approach associated with conventional chemotherapy treatments.
Overall, the findings highlighted in this review demonstrate the relevance and complexity of understanding the mechanisms involved in macrophage polarization (M1/M2) in schistosomiasis. The S. japonicum and S. mansoni antigens in macrophage polarization are particularly important in this process. These products have been shown to have immunomodulatory effects in different phases of schistosomiasis and are seen as potential therapeutic targets for this disease, especially in the chronic phase. Among the potential therapeutics, the combination of different schistosome antigens can result in higher levels of host protection, stimulating an adequate immune response for either an M1 or M2 profile; however, this can only be achieved after many in vitro and in vivo experiments.
Acknowledgements
We acknowledge the Graduate Program in Health Sciences at the Federal University of Maranhão for providing the support. We would like to acknowledge Editage (www.editage.com) for English-language editing.
Author's contributions
I. C. L. L.: investigation, conceptualization and writing of the original draft; I. C. L. L., G. C. C. G. F., R. A. N., M. G. S. L., V. A. F. S., J. G. M. R., G. S. M., R. C. C., L. A. S., R. N. M. G. and F. R. F. N.: review and editing of original draft. All the authors reviewed and approved the final version of this manuscript.
Financial support
I. C. L. L., G. C. C. G. F., R. A. N. and V. A. F. S. were supported by Coordenação de Aperfeiçoamento de Pessoal de Nível Superior doctoral fellowship. R. N. M. G. and F. R. F. N. were supported by CNPq researcher fellowship. The research was supported by FAPEMA (Universal 01001/19) and by CAPES (Financial code 001).
Conflict of interest
The authors declare no conflict of interest.