Introduction
Visceral leishmaniasis (VL) or kala-azar is a neglected infectious disease caused by infection with the protozoan parasites Leishmania infantum chagasi, Leishmania donovani and Leishmania infantum (Freitas et al., Reference Freitas, Secundino, Pimenta, Parreiras and Duarte2012). This is a potentially fatal disease in most untreated cases (over 95%), closed correlated to poverty, precarious conditions of basic sanitation and limited access to health services (WHO, 2022). In addition to VL being endemic in more than 79 countries, about 50 000–90 000 new cases of this disease occur annually worldwide and 3813 deaths were reported between 2014 and 2020 (Ruiz-Postigo et al., Reference Ruiz-Postigo, Jain, Mikhailov, Maia-Elkhoury, Valadas, Warusavithana and Osman2021). According to the World Health Organization (WHO), these cases were associated with 10 countries, specifically Brazil, China, Ethiopia, Eritrea, India, Kenya, Somalia, South Sudan, Sudan and Yemen (WHO, 2022). While L. infantum is responsible for the disease in North Africa, Europe and Latin America; L. donovani prevails in the East Africa and Indian subcontinent (Ready, Reference Ready2014).
The disease develops after the transmission of metacyclic forms of Leishmania spp. to vertebrate hosts by sand flies, mainly of the genders Phlebotomus spp. and Lutzomyia spp. (Nieto et al., Reference Nieto, Domínguez-Bernal, Orden, De La Fuente, Madrid-Elena and Carrión2011; Dostálová and Volf, Reference Dostálová and Volf2012). At the site of infection, the parasites are phagocytosed by macrophages, within which they survive and multiply by binary fission as amastigote forms (Dostálová and Volf, Reference Dostálová and Volf2012; de Freitas et al., Reference de Freitas, Leoratti, Freire-de-Lima, Morrot and Feijó2016). A broad spectrum of unspecific clinical manifestations is detected during VL development, especially chronic low-grade fever, anorexia, weight loss, weakness and hepatosplenomegaly. In addition, laboratory findings such as pancytopenia, low plasma albumin, high aminotransferase levels and hypergammaglobulinemia are often associated with VL (Serafim et al., Reference Serafim, Malafaia, Silva, Pedrosa and Rezende2010; Mwololo et al., Reference Mwololo, Mutiso, Macharia, Bourdichon and Gicheru2015; WHO, 2016).
The specific treatment of VL is mainly based on pentavalent antimonies (sodium stibogluconate and meglumine antimoniate), followed by paromomycin, oral miltefosine or amphotericin B as a second choice (Corral et al., Reference Corral, Serrano, Moreno, Torrado, Dominguez and Alunda2014; Joice et al., Reference Joice, Yang, Farahat, Meeds, Feng, Li, Boykin, Wang and Werbovetz2017; Alves et al., Reference Alves, Bilbe, Blesson, Goyal, Monnerat, Mowbray, Muthoni Ouattara, Pécoul, Rijal, Rode, Solomos, Strub-Wourgaft, Wasunna, Wells, Zijlstra, Arana and Alvar2018). New nanostructured lipid formulations of amphotericin B (amphotericin liposomal) have shown relevant results in preclinical (Corral et al., Reference Corral, Serrano, Moreno, Torrado, Dominguez and Alunda2014) and clinical (Sundar et al., Reference Sundar, Sinha, Rai, Verma, Nawin, Alam, Chakravarty, Vaillant, Verma, Pandey, Kumari, Lal, Arora, Sharma, Ellis, Strub-Wourgaft, Balasegaram, Olliaro, Das and Modabber2011) studies. However, this treatment is still expensive and often unavailable in several endemic areas (Corral et al., Reference Corral, Serrano, Moreno, Torrado, Dominguez and Alunda2014; Ponte-Sucre et al., Reference Ponte-Sucre, Gamarro, Dujardin, Barrett, López-Vélez, García-Hernández, Pountain, Mwenechanya and Papadopoulou2017). Although these drugs are recommended by the WHO as the reference chemotherapy, marked side-effects (i.e. nausea, vomiting, arthralgia, cardiac dysrhythmias, hepatitis and pancreatitis), limited efficacy, high cost, as well as complex administration (parenteral) make VL chemotherapy a challenging task (Sundar et al., Reference Sundar, Sinha, Rai, Verma, Nawin, Alam, Chakravarty, Vaillant, Verma, Pandey, Kumari, Lal, Arora, Sharma, Ellis, Strub-Wourgaft, Balasegaram, Olliaro, Das and Modabber2011; Hendrickx et al., Reference Hendrickx, Van den Kerkhof, Mabille, Cos, Delputte, Maes and and Caljon2017; Ponte-Sucre et al., Reference Ponte-Sucre, Gamarro, Dujardin, Barrett, López-Vélez, García-Hernández, Pountain, Mwenechanya and Papadopoulou2017). In addition, infections caused by parasites resistant to the reference chemotherapy represent a more recent and worrying barrier to the VL treatment (Mwololo et al., Reference Mwololo, Mutiso, Macharia, Bourdichon and Gicheru2015; Ponte-Sucre et al., Reference Ponte-Sucre, Gamarro, Dujardin, Barrett, López-Vélez, García-Hernández, Pountain, Mwenechanya and Papadopoulou2017). Thus, developing more effective and less toxic treatment protocols for VL is necessary and urgent (Khadem et al., Reference Khadem, Jia, Mou, Feiz Barazandeh, Liu, Keynan and Uzonna2017; Joice et al., Reference Joice, Yang, Farahat, Meeds, Feng, Li, Boykin, Wang and Werbovetz2017).
In the last years, therapy based on drugs association has emerged as an alternative to VL treatment (Mwololo et al., Reference Mwololo, Mutiso, Macharia, Bourdichon and Gicheru2015; Joice et al., Reference Joice, Yang, Farahat, Meeds, Feng, Li, Boykin, Wang and Werbovetz2017; Rebello et al., Reference Rebello, Andrade-Neto, Gomes, de Souza, Branquinha, Santos, Torres-Santos and D'Avila-Levy2019). As combination chemotherapy allows to increase drugs half-life, reduce medication dose, treatment time, systemic toxicity and side-effects (van Griensven et al., Reference van Griensven, Balasegaram, Meheus, Alvar, Lynen and Boelaert2010; Corral et al., Reference Corral, Serrano, Moreno, Torrado, Dominguez and Alunda2014; Bhattacharjee et al., Reference Bhattacharjee, Majumder, Majumdar, Choudhuri, Roy and Majumdar2015; Rebello et al., Reference Rebello, Andrade-Neto, Gomes, de Souza, Branquinha, Santos, Torres-Santos and D'Avila-Levy2019); greater adherence to the treatment protocol and better therapeutic outcomes are proposed (van Griensven et al., Reference van Griensven, Balasegaram, Meheus, Alvar, Lynen and Boelaert2010). With the increase in therapeutic failures after the administration of antimonial drugs and miltefosine, combination chemotherapy is also relevant to reduce Leishmania spp. resistance to treatment (Sundar et al., Reference Sundar, Singh, Rai, Prajapati, Singh, Ostyn, Boelaert, Dujardin and Chakravarty2012; Rijal et al., Reference Rijal, Ostyn, Uranw, Rai, Bhattarai, Dorlo, Beijnen, Vanaerschot, Decuypere, Dhakal, Das, Karki, Singh, Boelaert and Dujardin2013; Ponte-Sucre et al., Reference Ponte-Sucre, Gamarro, Dujardin, Barrett, López-Vélez, García-Hernández, Pountain, Mwenechanya and Papadopoulou2017). This approach has also emerged as a chemotherapy alternative for complicated VL cases, such as patients co-infected with HIV, for which monotherapy does not achieve satisfactory results (Alvar et al., Reference Alvar, Aparicio, Aseffa, Den Boer, Cañavate, Dedet, Gradoni, Ter Horst, López-Vélez and Moreno2008; van Griensven et al., Reference van Griensven, Balasegaram, Meheus, Alvar, Lynen and Boelaert2010; Rebello et al., Reference Rebello, Andrade-Neto, Gomes, de Souza, Branquinha, Santos, Torres-Santos and D'Avila-Levy2019).
Drug combination has been successfully used in the treatment of several other infectious diseases, such as tuberculosis, malaria and leprosy (Nosten and Brasseur, Reference Nosten and Brasseur2002; van Griensven et al., Reference van Griensven, Balasegaram, Meheus, Alvar, Lynen and Boelaert2010; Ramón-García et al., Reference Ramón-García, Ng, Anderson, Chao, Zheng, Pfeifer, Av-Gay, Roberge and Thompson2011). However, as the current evidence is fragmented, it is difficult to establish a clear profile of drugs and protocols administered, as well as to assess their therapeutic relevance for VL. Therefore, we use a systematic review framework to retrieve and analyse the preclinical evidence on the applicability and relevance of leishmanicidal or leishmaniostatic drug combination for the chemotherapeutic management of VL. In addition to mapping the available drug combinations and their spectrum of effectiveness, all preclinical models and treatment protocols used, as well as the risk of bias related to the studies that support the current evidence were critically analysed. By characterizing the rationale underlying the co-administration of different antileishmanial drugs, this systematic review may be relevant to support translational investigations in the search of parasitological cure for this disease.
Materials and methods
Guiding questions
The main questions to be answered in this systematic review were: Are combinations of antileishmanial drugs effective in the treatment of VL? What are the main chemotherapy protocols and primary research outcomes used to determine treatment effectiveness?
Search strategy and selection of primary studies
The PRISMA (Preferred Reporting Items for Systematic Reviews and Meta-analyses) statement was adopted for conducting this systematic review (Hooijmans et al., Reference Hooijmans, Rovers, De Vries, Leenaars, Ritskes-Hoitinga and Langendam2014). In our research strategy, an extensive literature search using 3 comprehensive databases was used: (i) PubMed/Medline, (ii) Scopus and (iii) Web of Science. We used an advanced research strategy based on search filters optimized according to specific algorithms and syntaxes adopted in each database. The search filters were structured in 3 complementary levels as follows: (i) treatment: combination chemotherapy, (ii) disease: VL and (iii) research type: preclinical models in vivo. A search filter was initially developed for PubMed according to the standardized descriptors obtained from the platform's thesaurus MeSH (Medical Subject Headings; https://www.ncbi.nlm.nih.gov/mesh). To expand the recovery of relevant indexed studies and those in the indexing process, the commands [MeSH Terms] and [TIAB] were combined. To detect all animal studies from PubMed, a standardized animal filter was applied (Pereira et al., Reference Pereira, Greco, Moreira, Chagas, Caldas, Gonçalves and Novaes2017). The same search filter used to disease and treatment was adapted to Scopus and Web of Science. A search limit for animal models in Scopus, and language limit (English) for Scopus and Web of Science were applied. No chronological restrictions were adopted in the search strategy. The complete search strategies applied in both databases can be consulted in the Supplementary material (Table 1). The initial selection was independently performed by 3 investigators (DSSB, ACFS and ACS), who screened the title and abstract of all recovered papers. Duplicate studies were removed by comparing the authors, title, year and journal of publication.
Table 1. Search strategies used to identify research registers in PubMed/Medline, Scopus and Web of Sciences databases

a The parasite species were not written in italics and capital letters were suppressed because these variants are not considered in the search algorithms of the databases used.
Inclusion and exclusion criteria
Only studies investigating the effectiveness of drug combination in the preclinical models of VL were included in the systematic review. Irrelevant studies were excluded (not related to the subject) after the initial screening, and all potentially relevant studies were recovered in full-text and evaluated for eligibility. Study exclusion was based on well-defined criteria as follows: (i) studies exclusively based on in vitro, ex vivo or in silico assays; (ii) studies evaluating cutaneous leishmaniasis or unrelated diseases; (iii) secondary studies (i.e. literature reviews, editorials, commentaries, short communication and letters to the editor); (iv) clinical studies; (v) absence of monotherapy as control; (vi) non-pharmacological treatments (i.e. plant extracts, cytokines, peptides) and vaccines; (vii) absence of groups treated with drug combination; and (viii) studies published in other language than English. After identifying all relevant studies in the primary search, we included a secondary screening to enhance the recovery of research records on the subject investigated. Thus, the reference lists of all papers identified in electronic databases and included in the systematic review were manually screened for additional relevant studies. In both search levels, 3 researchers (DSSB, ACS and RVG) independently analysed the eligibility criteria, and disagreements were resolved by arbitration, consulting 2 other researchers (RDN and EAMS).
Study characteristics and data extraction
Qualitative data were extracted from all included articles. For this, standardized spreadsheets (data extraction masks) were built, indicating the essential information to be collected from the reading of the individual study chain. Thus, the information summarized in data extraction masks was categorized as follows: (i) publication characteristics: authors, years and country; (ii) characteristics of the animal models: species, lineage, sex, weight and age; (iii) infection parameters: Leishmania species, strain, number of parasites inoculated, route of inoculation; (iv) treatment protocol: drugs co-administered, dose, frequency, route of administration; (v) complementary in vitro assays: parasitological/toxicity tests for drug interaction assessment; and (vi) primary research outcomes: parasitism, immunological, biochemical and survival results. For parasitism, data available in figures were digitized and the means was obtained using ImageJ software (Schneider et al., Reference Schneider, Rasband and Eliceiri2012) after calibrating each picture to the nearest 0.01 mm.
Reporting quality as a risk of bias
The risk of bias in animal studies was analysed from the SYRCLE's (SYstematic Review Center for Laboratory Animal Experimentation) guidelines, which are based on the Cochrane Collaboration risk-of-bias (RoB) tool for randomized trials (Hooijmans et al., Reference Hooijmans, Rovers, De Vries, Leenaars, Ritskes-Hoitinga and Langendam2014). This instrument is adjusted for bias aspects that play a specific role in animal intervention studies. The objective is to establish consistency and avoid discrepancies in the evaluation of methodological quality in the field of animal experimentation. In order to increase transparency and enforceability, signalling issues have been formulated to facilitate judgment based on the following levels: (1) random sequence generation, (2) baseline characteristics, (3) allocation concealment, (4) random housing, (5) blinding of participants and personnel, (6) random outcome assessment, (7) blinding of outcome assessment, (8) incomplete outcome data, (9) selective outcome reporting, and (10) other bias. The items in the RoB tool were scored with ‘yes’, indicating low risk of bias; ‘no’, indicating high risk of bias; or ‘unclear’, indicating that the item was not reported, and therefore, the risk of bias was unknown.
Results
Research records retrieved
Our primary search strategies recovered 2239 articles from PubMed, Scopus and Web of Science, of which 608 were duplicates. After title and abstract screening, 1631 studies were excluded due to inadequate research topic. Of these, 349 studies were based on only in vitro parasite viability assays, 595 investigated treatments without chemotherapeutic combination, and 238 studies evaluated other diseases. In addition, 386 studies corresponded to non-original papers, 5 papers were based on clinical investigations, and 23 studies were not written in English. Considering papers that investigated drug combination for VL treatment, 40 studies were selected for full-text evaluation. Thus, 14 relevant studies were identified and included in this systematic review (Fig. 1).

Fig. 1. Flow diagram of the systematic review literature search results. Based on PRISMA statement ‘Preferred Reporting Items for Systematic Reviews and Meta-Analyses’. www.prisma-statement.org.
Animal models of visceral leishmaniasis
General characteristics of the animal models used in all studies are showed in supplementary files (Table S1). Most studies were produced in India (35.71%, n = 5), followed by Kenya (14.29%, n = 2). The remaining studies (50%) were conducted in the USA, Italy, UK, Spain, Canada, Belgium and Brazil. BALB/c mice were used as an animal model in most studies (71.43%, n = 10), followed by hamster (21.43%, n = 3), especially the golden lineage (14.29%, n = 2). Hamster lineage was under-reported in 1 study. Dogs belonging to different breeds were used in only 1 study.
The report about the sex of the animals used in the studies shows that females (35.71%, n = 5) and works using males and females together (35.71%, n = 5) were the most found. Male animals were adopted in only 1 study (7.14%, n = 1), while this parameter was under-reported in 3 other studies (21.43%). Mice and hamster age ranged from 4 to 8 weeks, and dogs age ranged from 2 to 10 years old (7.14%, n = 1). This variable was neglected in 6 studies (21.43%). Animal's weight ranged from 18 to 25 g for mice, and 80 to 100 g for hamster. Most studies did not report these data (57.14, n = 8).
Visceral leishmaniasis characteristics
Leishmania donovani species was used in 9 studies (64.29%), followed by L. infantum (35.71%, n = 5). In studies with dogs, the animals were naturally infected and the Leishmania species was not identified. Four different Leishmania strains were used. The strains MHOM/KE/82/LRC-L445/NLB065 and MHOM/MA/67/ITMAP263 were used in 3 studies (21.43%) each, while MHOM/IN/89/GE1F8R and MHOM/ET/67/HU3 strains were found in 2 studies (14.29%) each. The remaining studies used 5 different strains. The strain used to infect the animals was not reported in 1 study (6.25%) (Table S2). Intravenous and intraperitoneal parasites inoculation was used in 5 studies (35.71%) each, followed by intracardiac route in 3 studies (21.43%) and only 1 study reported natural infection (7.14%). The inoculum size ranged from 1 × 106 to 1 × 108 parasites in studies using mice and hamsters (Table S2).
Protocols of combination chemotherapy
Considering all 14 studies, 23 drugs were tested in 40 different combinations (Tables 2 and S3). In mice models, 28 combinations (70%) based on 23 different drugs were used. The most used drug (n = 3, 13.64%) was miltefosine (MTF), followed by sodium antimony gluconate (SAG), diminazene (DIM) and DB766 compound (n = 2, 9.09% combinations each). Glycyrrhizic acid (GA), sodium stibogluconate (SSG), CAL-101 (CAL), amphotericin B (AMB), artesunate (ART), diperoxovanadate (PV6), N-palmitoyl-S-(2, 3-bis (palmitoyloxy)-(2RS)-propyl)-Cys-Ser-Lys4.Hydrochloride (Pam3Cys), buthionine sulfoximine (BSO), chloroquine (CHQ), ketoconazole (KET), lopinavir (LPV), posaconazole (POS) and tufisin (TUF) were also used in combination for VL treatment (Tables 2 and S3). For hamster models, 11 (27.5%) combinations based on 7 different drugs were investigated. The combinations were based on trans-aconitic acid (TAA), SSG, pentamidine (PET), allopurinol (ALO), allicin (ALL), AAMB, paromomycin (PAR) and MTF. Paromomycin and meglumine antimoniate (MEG) were combined to treat dogs with VL (Tables 2 and S3).
Table 2. Control monotherapy, drug combinations, target organs, parasitism suppression and nature of pharmacological interaction

a Drug interaction evaluated from in vitro or in vivo parasitological/cytotoxicity tests.
L, Liver; BM, bone marrow; LN, lymph node; 1, 200016 strain; 2, 200011 strain; 3; resistant strain; 4, susceptible strain; BSO, buthionine sulfoximine; SSG, sodium stibogluconate; MTF, miltefosine; DIM, diminazene; ART, artesunate; PV6, diperoxovanadate; Pam3Cys, N-palmitoyl-S-(2, 3-bis (palmitoyloxy)-(2RS)-propyl)-Cys-Ser-Lys4.Hydrochloride; AMB, amphotericin B; 1,2 and 3,4, studies using 2 different strains.

a Drug interaction evaluated from in vitro or in vivo parasitological/cytotoxicity tests.
L, Liver; BM, bone marrow; LN, lymph node; MTF, miltefosine; DIM, diminazene; AMB, amphotericin B; POS, posaconazole; ART, artesunate; CHQ, chloroquine CAL-101, p110δ-specific pharmacological inhibitors; SAG, sodium antimony gluconate; GA, glycyrrhizic acid; F-TUF, free-tufisin; L-TUF, lipo-tufisin; DB766, 2,5-bis[2-(2-i-propoxy)-4-(2-pyridylimino) aminophenyl] furan hydrochloride. ¨No parasite was detected.

a Drug interaction evaluated from in vitro or in vivo parasitological/cytotoxicity tests.
L, Liver; BM, bone marrow; LN, lymph node; MTF, miltefosine; DB766, 2,5-bis[2-(2-i-propoxy)-4-(2-pyridylimino) aminophenyl] furan hydrochloride; POS, posaconazole; KET, ketoconazole.

a Drug interaction evaluated from in vitro or in vivo parasitological/cytotoxicity tests.
L, Liver; BM, bone marrow; LN, lymph node; S, spleen; MTF, miltefosine; MEG, meglumine antimoniate; PAR, paromomycin; LPV, lopinavir. ¨No parasite was detected.

a Drug interaction evaluated from in vitro or in vivo parasitological/cytotoxicity tests.
L, Liver; BM, bone marrow; LN, lymph node; S, spleen; AMB, amphotericin B; ALL, allicin; ALO, allopurinol; PAR, paromomycin; MTF, miltefosine; PET, pentamidine; MEG, meglumine antimoniate; TAA, trans-aconitic acid. ¨No parasite was detected. (–) Not reported or evaluated. 5,6: Study evaluated the drug combination in different models of infection.
Intraperitoneal (n = 12, 48%) and oral (n = 6, 24%) routes were mainly used for drug administration. Intramuscular, subcutaneous and intravenous routes were reported in 24% of the studies. Only 1 study did not report these data. Most drugs were administered daily (55.56%, n = 10) or in a single dose (16.77%, n = 3). Two studies administered the drugs in alternate days (11.11%), and 2 studies twice a week (11.11%). Only 1 study chose to administer the drugs twice a day (5.56%). Most of the studies (n = 14, 71.42%) used doses below than those recommended for monotherapy (subdoses), to reduce the risk of toxicity (Table 3).
Table 3. Effective drug combinations able to induce an efficient parasite clearance in different animal models of visceral leishmaniasis

a Main outcomes compared to the group treated with monotherapy.
H2O2, Hydrogen peroxide; IFN-γ, interferon gamma; IL, interleukin; NO, nitric oxide; ROS, reactive oxygen species; TGF-β, transforming growth factor beta; TNF-α, tumour necrosis factor alpha. Drugs: BSO, buthionine sulfoximine; SSG, sodium stibogluconate; PAM3cys, N-palmitoyl-S-(2, 3-bis (palmitoyloxy)-(2RS)-propyl)-Cys-Ser-Lys4.Hydrochloride; F-TUF, free-tufisin; L-TUF, lipo-tufisin; MTF, miltefosine; SAG, sodium antimony gluconate; GA, glycyrrhizic acid; CAL-101, p110δ-specific pharmacological inhibitors; AMB, amphotericin B. ¨No parasite was detected.

a Main outcomes compared to the group treated with monotherapy.
Drugs: POS, posaconazole; DB766, 2,5-bis[2-(2-i-propoxy)-4-(2-pyridylimino) aminophenyl] furan hydrochloride; KET, ketoconazole; MTF, miltefosine; LPV, lopinavir; SSG, sodium stibogluconate; PET, pentamidine; ALO, allopurinol; TAA, trans-aconitic acid; All, allicin; AMB, amphotericin B; PAR, paromomycin. ¨ No parasite was detected.
Main outcomes
The main outcomes were shown in Tables 2, 3 and Fig. 2. Preclinical studies demonstrated that combination chemotherapy is effective for VL treatment. In most studies, parasitism was used as a primary outcome to assess the success of antiparasitic chemotherapy. Thus, in the entire dataset with 14 studies, 100% reported a significant reduction in organ parasitism, especially in spleen (n = 11, 44%), liver (n = 11, 44%) and bone marrow (n = 3, 12%). Compared to monotherapy, the improved parasite clearance achieved from drug combination was often associated with the induction of a protective Th1 immunological response, which was mainly evidenced by IL-12, IL-6, TNFα, IFN-γ and IgG2 upregulation (Haldar et al., Reference Haldar, Banerjee, Naskar, Kalita, Islam and Roy2009; Shakya et al., Reference Shakya, Sane, Vishwakarma and Gupta2012a, Reference Shakya, Sane, Haq and Gupta2012b; Bhattacharjee et al., Reference Bhattacharjee, Majumder, Majumdar, Choudhuri, Roy and Majumdar2015). Conversely, combined pharmacological regimens were associated with an attenuated production of Th2 and/or Treg cytokines, such as IL-10, IL-4 or TGFβ upregulation (Haldar et al., Reference Haldar, Banerjee, Naskar, Kalita, Islam and Roy2009; Shakya et al., Reference Shakya, Sane, Vishwakarma and Gupta2012a, Reference Shakya, Sane, Haq and Gupta2012b; Bhattacharjee et al., Reference Bhattacharjee, Majumder, Majumdar, Choudhuri, Roy and Majumdar2015; Khadem et al., Reference Khadem, Jia, Mou, Feiz Barazandeh, Liu, Keynan and Uzonna2017). In addition, beneficial antiparasitic effects were associated with marked modulation of redox metabolism, especially attributed to upregulation of reactive oxygen (ROS) and nitrogen species (RNS) biosynthesis (Haldar et al., Reference Haldar, Banerjee, Naskar, Kalita, Islam and Roy2009; Shakya et al., Reference Shakya, Sane, Vishwakarma and Gupta2012a, Reference Shakya, Sane, Haq and Gupta2012b; Bhattacharjee et al., Reference Bhattacharjee, Majumder, Majumdar, Choudhuri, Roy and Majumdar2015), and increase of hepatic and splenic and reduced total glutathione levels (Carter et al., Reference Carter, Sundar, Spickett, Pereira and Mullen2003). Improved cellular parameters, such as macrophage phagocytic ability and T-cell proliferation, were also associated with a better parasitological control in animals treated with combination chemotherapy than monotherapy (Haldar et al., Reference Haldar, Banerjee, Naskar, Kalita, Islam and Roy2009; Shakya et al., Reference Shakya, Sane, Vishwakarma and Gupta2012a, Reference Shakya, Sane, Haq and Gupta2012b; Khadem et al., Reference Khadem, Jia, Mou, Feiz Barazandeh, Liu, Keynan and Uzonna2017). Improved score and clinical signs (i.e. decrease of hematochemical parameters, cutaneous alterations, anaemia, reduction in the size of spleen and/or lymph nodes and increase in weight), as well as reduction in organs hypertrophy and increase in body weight (Oliva et al., Reference Oliva, Gradoni, Cortese, Orsini, Ciaramella, Scalone, de Luna and Persechino1998) and survival rates (Mutiso et al., Reference Mutiso, Macharia, Barasa, Taracha, Bourdichon and Gicheru2011) were also reported as good markers of drug combination effectiveness.
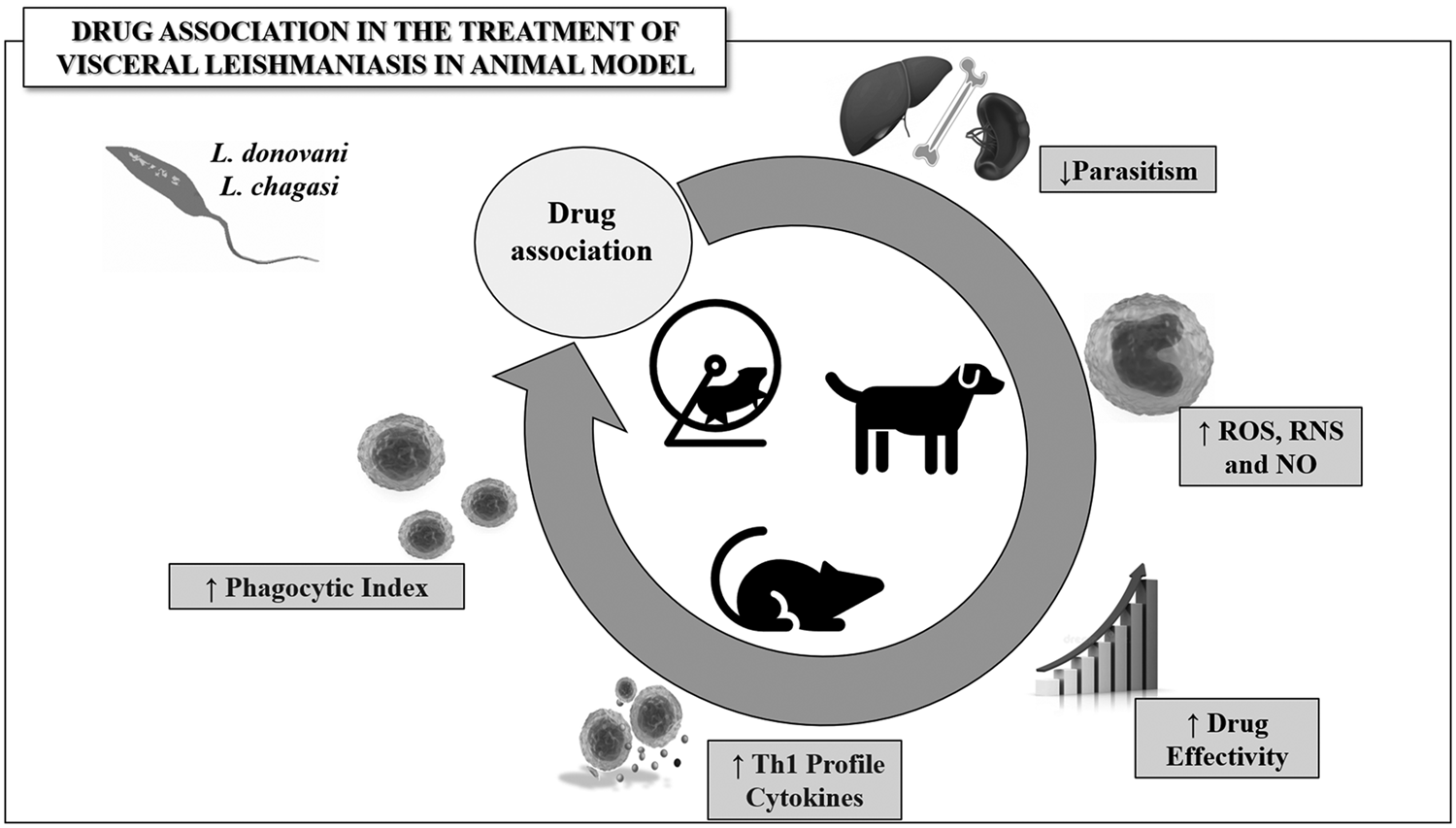
Fig. 2. Representative model of the main outcomes obtained from drug combination used in the treatment of visceral leishmaniasis. Drug combination is potentially useful in chemotherapy effectivity by upregulating the biosynthesis of reactive species and protective Th1 cytokines, stimulating proliferation and activity of immune cells, consequently reducing tissue parasitism in Leishmania spp.-infected animals.
As a critical parameter in drug combination, 8 studies (57.14%) used integrated in vitro models to determine the nature of pharmacological interaction from specific drug combinations. From 4 studies (28.57%), 7 synergistic interactions were reported: (i) glycyrrhizic acid + sodium antimony gluconate, (ii) diminazene + chloroquine, (iii) DB766 + posaconazole (7 different combinations), (iv) DB766 + ketoconazole, (v) trans-aconitic acid + sodium stibogluconate, (vi) trans-aconitic acid + pentamidine and (vii) trans-aconitic acid + allopurinol. Additive interaction was reported in 4 studies (28.57%) with: (i) diperoxovanadate + sodium antimony gluconate, (ii) miltefosine + lopinavir, (iii) allicin + amphotericin B and (iv) DD766 + ketoconazole. Antagonism interaction was reported in 1 study using 2 different combinations with DD766 + ketoconazole. One study (7.14%) investigating paromomycin + miltefosine reported no pharmacological interaction.
Risk of bias
The risk of bias analysed for all studies included in the systematic review is shown in Fig. 3. None of the studies fulfilled all methodological criteria, and a mean of 43.57 ± 6.79% items reported in SYRCLE's toll was covered from all studies reviewed. The criteria not met were predominantly under-reported, indicating an unknown or high risk of bias. The chronological analysis of all studies indicated that the risk of bias exhibited no time-dependent influence, suggesting that methodological limitations have been systematically replicated over the years of research in the area. Eight studies (57.14%) reached a below-average score (Fig. 4). Considering individually each criterion analysed, none of the studies reported information such as experimental blindness (outcome assessment, participants and personnel), allocation concealment or the criteria about randomization (housing and outcome assessment) which resulted in high risk of bias. The random sequence generator was performed in only 1 study (7.14%) (Joice et al., Reference Joice, Yang, Farahat, Meeds, Feng, Li, Boykin, Wang and Werbovetz2017). Therefore, 3 studies (21.43%) reported randomization of animals but did not report the method used (Shakya et al., Reference Shakya, Sane, Vishwakarma and Gupta2012a, Reference Shakya, Sane, Haq and Gupta2012b; Corral et al., Reference Corral, Serrano, Moreno, Torrado, Dominguez and Alunda2014). The baseline characteristics for animal models were reported in most studies (n = 7, 50%). Interestingly, 5 studies started the experiment with animals from different sexes (Carter et al., Reference Carter, Sundar, Spickett, Pereira and Mullen2003; Mutiso et al., Reference Mutiso, Macharia, Barasa, Taracha, Bourdichon and Gicheru2011; Shakya et al., Reference Shakya, Sane, Vishwakarma and Gupta2012a, Reference Shakya, Sane, Haq and Gupta2012b; Mwololo et al., Reference Mwololo, Mutiso, Macharia, Bourdichon and Gicheru2015). Oliva et al. (Reference Oliva, Gradoni, Cortese, Orsini, Ciaramella, Scalone, de Luna and Persechino1998) started the treatment using dogs from different breeds, weight, age and clinical conditions. Incomplete outcome data were adequately addressed in 11 studies (78.57%). Three studies (21.43%) unreported the parasitism data for some treatment groups (Kar et al., Reference Kar, Kar, Bhattacharya and Ghosh1993; Corral et al., Reference Corral, Serrano, Moreno, Torrado, Dominguez and Alunda2014; Khadem et al., Reference Khadem, Jia, Mou, Feiz Barazandeh, Liu, Keynan and Uzonna2017). Other potential sources of bias detected were the absence of information such as strain used, route of drug administration and lineage of animals.

Fig. 3. Results of the risk of bias and methodological quality indicators for all studies included in this systematic review that evaluated the effect of drug combination for the treatment of visceral leishmaniasis. The items covered by the Systematic Review Centre for Laboratory Animal Experimentation (SYRCLE) Risk of Bias assessment were scored with ‘yes’ indicating low risk of bias, ‘no’ indicating high risk of bias, or ‘unclear’ indicating that the item was not reported, resulting in an unknown risk of bias.

Fig. 4. Analysis of the risk of bias in each study included in the systematic review. Based on the SYRCLE's risk of bias tool for animal studies. The dotted line indicates the average score obtained for all studies reviewed.
Discussion
In the present review, our findings are discussed considering 2 guiding questions: (1) Are combinations of antileishmanial drugs effective in the treatment of VL? and (2) What are the main chemotherapy protocols and primary research outcomes used to determine treatment effectiveness? By answering these questions, we identified that specific protocols based on drug combination can be used in realistic and rational strategies to potentiate the effectiveness of antiparasitic chemotherapy in different animal models of leishmaniasis compared to monotherapy. Interestingly, beneficial antiparasitic results were specially determined by the combination of complex pharmacological effects, especially stimulation of leucocyte replication and activity (Haldar et al., Reference Haldar, Banerjee, Naskar, Kalita, Islam and Roy2009; Shakya et al., Reference Shakya, Sane, Vishwakarma and Gupta2012a, Reference Shakya, Sane, Haq and Gupta2012b), upregulation of Th1 cytokines, ROS and RNS production (Haldar et al., Reference Haldar, Banerjee, Naskar, Kalita, Islam and Roy2009; Shakya et al., Reference Shakya, Sane, Vishwakarma and Gupta2012a, Reference Shakya, Sane, Haq and Gupta2012b; Bhattacharjee et al., Reference Bhattacharjee, Majumder, Majumdar, Choudhuri, Roy and Majumdar2015), attenuation of the parasitic load and increase in the cure rates of infected hosts (Kar et al., Reference Kar, Kar, Bhattacharya and Ghosh1993; Carter et al., Reference Carter, Sundar, Spickett, Pereira and Mullen2003; Haldar et al., Reference Haldar, Banerjee, Naskar, Kalita, Islam and Roy2009; Shakya et al., Reference Shakya, Sane, Vishwakarma and Gupta2012a, Reference Shakya, Sane, Haq and Gupta2012b; Corral et al., Reference Corral, Serrano, Moreno, Torrado, Dominguez and Alunda2014; Hendrickx et al., Reference Hendrickx, Van den Kerkhof, Mabille, Cos, Delputte, Maes and and Caljon2017; Khadem et al., Reference Khadem, Jia, Mou, Feiz Barazandeh, Liu, Keynan and Uzonna2017; Joice et al., Reference Joice, Yang, Farahat, Meeds, Feng, Li, Boykin, Wang and Werbovetz2017; Rebello et al., Reference Rebello, Andrade-Neto, Gomes, de Souza, Branquinha, Santos, Torres-Santos and D'Avila-Levy2019).
From our search strategy, we identified that studies investigating combination chemotherapy were mainly concentrated in 5 countries, such as Bangladesh, Brazil, Ethiopia, India and Sudan. Considering that VL exhibits a broad geographic distribution in 98 countries (Alvar et al., Reference Alvar, Vélez, Bern, Herrero, Desjeux, Cano, Jannin and den Boer2012; WHO, 2016), this finding indicates that drug combination is not yet widely explored for VL, although studies in the field have expanded in the last 2 decades. However, the countries in which the studies were developed are consistent with endemic areas for leishmaniasis, since VL cases are especially concentrated in Latin America, Africa and the Middle East (Alvar et al., Reference Alvar, Vélez, Bern, Herrero, Desjeux, Cano, Jannin and den Boer2012; Singh et al., Reference Singh, Hasker, Boelaert and Sundar2016; WHO, 2016). In these areas, social and environmental conditions such as humidity, temperature, rainfall, poverty, hygiene habits and limited access to health services are favourable to vector spread, making it difficult to control the disease (Oryan and Akbari, Reference Oryan and Akbari2016). Interestingly, most of the identified studies originated from India, which is the world leader in VL cases (Alvar et al., Reference Alvar, Vélez, Bern, Herrero, Desjeux, Cano, Jannin and den Boer2012; WHO, 2016). Especially in this country, the governmental strategies associated with combination chemotherapy have been successfully integrated to clinical VL management (WHO, 2015; Oryan and Akbari, Reference Oryan and Akbari2016). Despite the high incidence of VL in South America, only 1 study was identified (Rebello et al., Reference Rebello, Andrade-Neto, Gomes, de Souza, Branquinha, Santos, Torres-Santos and D'Avila-Levy2019). This finding is still poorly understood, although it is potentially related to limited investments in research and development, reinforcing the neglected characteristic of this tropical disease (Hotez et al., Reference Hotez, Bottazzi, Franco-Paredes, Ault and Periago2008; Lindoso and Lindoso, Reference Lindoso and Lindoso2009). A smaller number of studies were also derived from Italy, Belgium, Spain, UK, Canada and USA. In these countries, VL cases have been frequently associated with exotic Leishmania species, which are introduced in these areas by humans and dogs infected and that come from endemic countries (Pérez-Ayala et al., Reference Pérez-Ayala, Norman, Pérez-Molina, Herrero, Monge and López-Vélez2009).
Despite geographical divergence of the studies reviewed, the animal model choice (i.e. BALB/c mice and golden hamster) reflected a convergent research perspective. The genetic similarity from mice inbred strains (i.e. BALB/c and C57BL/6), its high reproductive efficiency and wide availability of analytical tools make these animals attractive models for preclinical research (Loria-Cervera and Andrade-Narvaez, Reference Loria-Cervera and Andrade-Narvaez2014). Although extensively used (Carter et al., Reference Carter, Sundar, Spickett, Pereira and Mullen2003; Haldar et al., Reference Haldar, Banerjee, Naskar, Kalita, Islam and Roy2009; Mutiso et al., Reference Mutiso, Macharia, Barasa, Taracha, Bourdichon and Gicheru2011; Shakya et al., Reference Shakya, Sane, Vishwakarma and Gupta2012a, Reference Shakya, Sane, Haq and Gupta2012b; Mwololo et al., Reference Mwololo, Mutiso, Macharia, Bourdichon and Gicheru2015; Khadem et al., Reference Khadem, Jia, Mou, Feiz Barazandeh, Liu, Keynan and Uzonna2017; Joice et al., Reference Joice, Yang, Farahat, Meeds, Feng, Li, Boykin, Wang and Werbovetz2017), Leishmania-infected BALB/c mice presented limitations associated with disease development. Accordingly, these animals exhibit a natural profile of parasite resistance and, therefore, they do not represent the most adequate model to simulate human disease (Loeuillet et al., Reference Loeuillet, Bañuls and Hide2016; McFarlane et al., Reference McFarlane, Mokgethi, Kaye, Hurdayal, Brombacher, Alexander and Carter2019). On the other hand, the hamster was reported as the best preclinical model for VL in 3 reviewed studies (Melby et al., Reference Melby, Tabares, Restrepo, Cardona, McGuff and Teale2001; Loria-Cervera and Andrade-Narvaez, Reference Loria-Cervera and Andrade-Narvaez2014). Characteristically, these animals exhibit immunopathological similarities of human VL, especially the limited ability in controlling parasites replication in target organs (i.e. liver, spleen and bone marrow) despite a strong Th1 response. Thus, the hamster infection culminates with the typical development of hepatosplenomegaly and hypergammaglobulinemia (Melby et al., Reference Melby, Tabares, Restrepo, Cardona, McGuff and Teale2001; Loria-Cervera and Andrade-Narvaez, Reference Loria-Cervera and Andrade-Narvaez2014). However, the applicability of hamsters is still limited due the scarcity of molecular tools available to investigate the immunological mechanisms associated with resistance or susceptibility to infection by Leishmania spp. (Gupta and Nishi, Reference Gupta and Nishi2011; Loria-Cervera and Andrade-Narvaez, Reference Loria-Cervera and Andrade-Narvaez2014). This limitation has been also observed in the dog model, which was reported in only study. Dogs are a realistic VL model (Quinnell and Courtenay, Reference Quinnell and Courtenay2009), especially considering that these animals are natural reservoirs of Leishmania spp., providing a more accurate preclinical understanding of the disease immunopathology with translational potential (Loria-Cervera and Andrade-Narvaez, Reference Loria-Cervera and Andrade-Narvaez2014). The limited use of dogs is not surprising, as they are generally associated with high animals cost for acquisition and maintenance, need of large facilities, and more restrictive ethical requirements compared to preclinical studies with rodent models (Loria-Cervera and Andrade-Narvaez, Reference Loria-Cervera and Andrade-Narvaez2014).
In addition to the preclinical model, animals' age and sex are still highlighted as potential factors influencing the evolution of parasitic infections. There are consistent disagreements about the ideal age in animal models of Leishmania spp. infection. While some studies reinforce that younger animals are more susceptible to infection (Müller et al., Reference Müller, Hailu, Choi, Abebe, Fuentes, Munder, Modolell and Kropf2008; Boldizsar et al., Reference Boldizsar, Mikecz and Glant2010; Lockard et al., Reference Lockard, Wilson and Rodríguez2019), others pointed that extreme of ages favours the infection due to the curvilinear profile of activation and effectiveness of the innate and acquired immune responses throughout the host life cycles in animals and humans (Boldizsar et al., Reference Boldizsar, Mikecz and Glant2010; Fuentes et al., Reference Fuentes, Fuentes, Alarcón and Palomo2017). As the animals' age was often under-reported, the influence of this parameter on the immune response and on the results of antiparasitic chemotherapy cannot be accurately determined, requiring further investigation. Unlike age, the animals were consistently reported in most studies reviewed, which used female and male animals in a homogeneous proportion. There is evidence that sex hormones can interact with leucocytes such as monocytes/macrophages and lymphocytes, determining idiosyncratic immune patterns in male and female organisms (Snider et al., Reference Snider, Lezama-Davila, Alexander and Satoskar2009; Bhatia et al., Reference Bhatia, Sekhon and Kaur2014; Kovats, Reference Kovats2015). Accordingly, differential immunosuppressive effects are potentially attributed to testosterone and progesterone levels, which can differentially downregulate NFκB cell signal pathway, cytokines production, NK cells and macrophages activity (D'Agostino et al., Reference D'Agostino, Milano, Barbera, Bella, Rosa, Ferlazzo, Farruggio, Miceli, Miele, Castagnneta and Cillari1999; Snider et al., Reference Snider, Lezama-Davila, Alexander and Satoskar2009; Bhatia et al., Reference Bhatia, Sekhon and Kaur2014). Previous studies also indicated that increased levels of these steroidal hormones were associated with a more intense production of Th2/Treg effectors such as IL-4 and IL-10 (Piccinni, Reference Piccinni2000; Snider et al., Reference Snider, Lezama-Davila, Alexander and Satoskar2009), which are recognized by increased host susceptibility to VL (Shakya et al., Reference Shakya, Sane, Haq and Gupta2012b). From this physiological perspective, previous studies indicated that male hamsters infected with are more susceptible to L. donovani infection, exhibiting higher parasite load compared to female counterparts. Thus, male animals are suggested as a more appropriate preclinical model of leishmaniasis, an aspect also related to lower hormonal variability (Travi et al., Reference Travi, Osorio, Melby, Chandrasekar, Arteaga and Saravia2002; Lockard et al., Reference Lockard, Wilson and Rodríguez2019).
Alongside the characteristics of the animal model, genetic and phenotypic variability of Leishmania strains also exerts a marked influence on host–pathogen interaction, time-course of infection and pathological outcomes (Loeuillet et al., Reference Loeuillet, Bañuls and Hide2016; Samarasinghe et al., Reference Samarasinghe, Samaranayake, Kariyawasam, Siriwardana, Imamura and Karunaweera2018). From the studies reviewed, VL was especially induced by L. donovani MHOM/KE/82/LRC-L445/NLB065 and L. infantum MHOM/MA/67/ITMAP263 strains, which proved to be infective and pathogenic. In addition, parasite strains (i.e. L. donovani pentavalent antimonial-resistant MHOM/IN/1989/GE1) with recognized pharmacological resistance to classical leishmanicidal drugs (i.e. pentamidine, sodium antimony gluconate, sodium stibogluconate) were used (Haldar et al., Reference Haldar, Banerjee, Naskar, Kalita, Islam and Roy2009; Bhattacharjee et al., Reference Bhattacharjee, Majumder, Majumdar, Choudhuri, Roy and Majumdar2015). Interestingly, these strains were intentionally used in realistic strategies to identify more efficient chemotherapeutic strategies to overcome drug resistance observed in several cases of VL (Haldar et al., Reference Haldar, Banerjee, Naskar, Kalita, Islam and Roy2009; Bhattacharjee et al., Reference Bhattacharjee, Majumder, Majumdar, Choudhuri, Roy and Majumdar2015). In preclinical models, the parasite inoculum is additionally relevant and must be carefully delimited. Accordingly, the inoculum size can exert a marked impact on the parasite load, tissue parasitism, immunological sensitization, infection severity and mortality rates of the infected host (Loeuillet et al., Reference Loeuillet, Bañuls and Hide2016). In the studies reviewed, the use of medium (1 × 106) or higher (1 × 108) parasite inoculum was effective in inducing a marked parasitism in target organs (i.e. liver, spleen and bone marrow), which was consistent with the human infection (Rolão et al., Reference Rolão, Melo and Campino2004; Oliveira et al., Reference Oliveira, Costa, Chavez-Fumagalli, Valadares, Duarte, Costa, Martins, Gomes, Melo, Soto, Tavares and Coelho2012). There is evidence that poorly sized inoculum (i.e. 103 to 104 parasites) may be inadequate to induce VL, since Th1 immunological effectors can resolve the infection resolution before relevant pathological manifestations develop (Kaur et al., Reference Kaur, Kaur, Garg, Mukherjee, Raina and Athokpam2008; Oliveira et al., Reference Oliveira, Costa, Chavez-Fumagalli, Valadares, Duarte, Costa, Martins, Gomes, Melo, Soto, Tavares and Coelho2012). Conversely, high inoculum (≅107 parasites) can trigger an exacerbated Th2 immune response, which aggravates tissue parasitism and infection severity (Rolão et al., Reference Rolão, Melo and Campino2004; Kaur et al., Reference Kaur, Kaur, Garg, Mukherjee, Raina and Athokpam2008). When evaluating the parasite load induced by different inoculation routes, Kaur et al. (Reference Kaur, Kaur, Garg, Mukherjee, Raina and Athokpam2008) concluded that subcutaneous is less efficient than intradermal, intraperitoneal and intracardiac routes to induce liver parasitism. In this sense, intracardiac and intraperitoneal routes were used in 80% of the studies reviewed. These routes were consistent with the development of a more prominent Th2 phenotype, which was suitable to stimulate tissue parasitism and a persistence infection (Mukherjee et al., Reference Mukherjee, Ghosh and Ghose2003).
From the 14 studies investigating the in vivo models of VL described, 7 studies also evaluated drug combinations in vitro. Most studies were consistent in reporting a potent leishmanicidal effect in vitro (Kar et al., Reference Kar, Kar, Bhattacharya and Ghosh1993; Mutiso et al., Reference Mutiso, Macharia, Barasa, Taracha, Bourdichon and Gicheru2011; Bhattacharjee et al., Reference Bhattacharjee, Majumder, Majumdar, Choudhuri, Roy and Majumdar2015; Mwololo et al., Reference Mwololo, Mutiso, Macharia, Bourdichon and Gicheru2015; Hendrickx et al., Reference Hendrickx, Van den Kerkhof, Mabille, Cos, Delputte, Maes and and Caljon2017; Joice et al., Reference Joice, Yang, Farahat, Meeds, Feng, Li, Boykin, Wang and Werbovetz2017), and only 1 study indicated cytotoxicity on host cells (Rebello et al., Reference Rebello, Andrade-Neto, Gomes, de Souza, Branquinha, Santos, Torres-Santos and D'Avila-Levy2019). According to Huang et al. (Reference Huang, Pei, Liu, Chen, Dou, Shu, Yuan, Lin, Peng, Zhang and Fu2019), in vitro studies are relevant to investigate potential drug interactions. Thus, the combination of drugs with additive and especially synergistic effects generally results in better therapeutic outcomes compared to monotherapy in preclinical models (Huang et al., Reference Huang, Pei, Liu, Chen, Dou, Shu, Yuan, Lin, Peng, Zhang and Fu2019). In this perspective, 23 drugs administered in 40 different combinations were identified from all studies reviewed. These combinations were especially based on the following drugs: (i) BSO + SSG (Carter et al., Reference Carter, Sundar, Spickett, Pereira and Mullen2003); (ii) PV6 + SAG (Haldar et al., Reference Haldar, Banerjee, Naskar, Kalita, Islam and Roy2009); (iii) DIM + ART (Mutiso et al., Reference Mutiso, Macharia, Barasa, Taracha, Bourdichon and Gicheru2011); (iv) Pam3Cys + MTF (Shakya et al., Reference Shakya, Sane, Vishwakarma and Gupta2012a); (v) TUF + MTF (Shakya et al., Reference Shakya, Sane, Haq and Gupta2012b); (vi) GA + SAG (Bhattacharjee et al., Reference Bhattacharjee, Majumder, Majumdar, Choudhuri, Roy and Majumdar2015); (vii) DIM + CHQ (Mwololo et al., Reference Mwololo, Mutiso, Macharia, Bourdichon and Gicheru2015); (viii) CAL-101 + AMB (Khadem et al., Reference Khadem, Jia, Mou, Feiz Barazandeh, Liu, Keynan and Uzonna2017); (ix) DB766 + POS or DB766 + KET (Joice et al., Reference Joice, Yang, Farahat, Meeds, Feng, Li, Boykin, Wang and Werbovetz2017); (x) MTF + LPV (Rebello et al., Reference Rebello, Andrade-Neto, Gomes, de Souza, Branquinha, Santos, Torres-Santos and D'Avila-Levy2019); (xi) PAR + MEG (Oliva et al., Reference Oliva, Gradoni, Cortese, Orsini, Ciaramella, Scalone, de Luna and Persechino1998); (xii) SSG + TAA, ALO + TAA, PET + TAA and SSG + ALO (Kar et al., Reference Kar, Kar, Bhattacharya and Ghosh1993); (xii) AMB + ALL (Corral et al., Reference Corral, Serrano, Moreno, Torrado, Dominguez and Alunda2014) and (xiv) MTF + PAR (Hendrickx et al., Reference Hendrickx, Van den Kerkhof, Mabille, Cos, Delputte, Maes and and Caljon2017).
Based on the results of parasite control compared to antiparasitic monotherapy, superior therapeutic responses were obtained from the following combination strategies: (i) BSO (34 mg kg−1) + SSG (74 mg SBv kg−1) compared to SSG (282 mg SBv kg−1) (Carter et al., Reference Carter, Sundar, Spickett, Pereira and Mullen2003); (ii) PV6 (0.5 μmol 30 g−1) + SAG (50 mg kg−1) compared to SAG (250 mg kg−1) (Haldar et al., Reference Haldar, Banerjee, Naskar, Kalita, Islam and Roy2009); (iii) Pam3Cys (100 μg) + MTF (5 mg kg−1) compared to MTF (20 mg kg−1) (Shakya et al., Reference Shakya, Sane, Vishwakarma and Gupta2012a); (iv) L-TUF (60 μg) + MTF (5 mg kg−1) compared to MTF (20 mg kg−1) (Shakya et al., Reference Shakya, Sane, Haq and Gupta2012b); (v) GA (50 mg kg−1) + SAG (250 mg kg−1) compared to SAG (250 mg kg−1) (Bhattacharjee et al., Reference Bhattacharjee, Majumder, Majumdar, Choudhuri, Roy and Majumdar2015); (vi) CAL-101 (0.05 mg) + AMB (0.1 mg kg−1) compared to AMB (0.1 mg kg−1) (Khadem et al., Reference Khadem, Jia, Mou, Feiz Barazandeh, Liu, Keynan and Uzonna2017); (vii) DB766 (45 mg kg−1) + KET (18 mg kg−1) compared to MTF (10 mg kg−1) (Joice et al., Reference Joice, Yang, Farahat, Meeds, Feng, Li, Boykin, Wang and Werbovetz2017); (viii) LPV (493.2 mg kg−1) + MTF (7.2 mg kg−1) and LPV (246.6 mg kg−1) + MTF (7.2 mg kg−1) compared to MTF (15.4 mg kg−1) (Rebello et al., Reference Rebello, Andrade-Neto, Gomes, de Souza, Branquinha, Santos, Torres-Santos and D'Avila-Levy2019); (ix) PAR (3.5 mg kg−1) + MEG (20 mg Sb kg−1) compared to MEG (30 mg Sb kg−1); (x) SSG (50 mg Sb kg−1) + TAA (400 mg) or PET (8 mg kg−1) + TAA (200 mg) or PET (8 mg kg−1) + TAA (400 mg) and ALO (15 mg kg−1) + TAA (400 mg) compared to monotherapy using SSG (100 mg Sb kg−1), SSG (50 mg Sb kg−1), PET (8 mg kg−1) or ALO (15 mg kg−1) (Kar et al., Reference Kar, Kar, Bhattacharya and Ghosh1993); (xi) AMB (1 mg kg−1) + ALL (5 mg kg−1) compared to AMB (5 mg kg−1) (Corral et al., Reference Corral, Serrano, Moreno, Torrado, Dominguez and Alunda2014); and (xii) MTF (20 mg kg−1) + PAR (350 mg kg−1) or MTF (10 mg kg−1) + PAR (180 mg kg−1) compared to MTF (40 mg kg−1) (Hendrickx et al., Reference Hendrickx, Van den Kerkhof, Mabille, Cos, Delputte, Maes and and Caljon2017).
Interestingly, combination strategies based on MTF and antimony-based drugs (SSG, SAG and MEG) were more frequently used in all studies analysed. In general, this is a rational strategy considering a more favourable time and cost of treatment, reducing the dose (use of subdoses) and toxicity of the combined drugs, which can act through complementary ways to overcome the parasite's pharmacological resistance (Olliaro et al., Reference Olliaro, Guerin, Gerstl, Haaskjold, Rottingen and Sundar2005). Despite the drug combination exhibits a theoretical potential to improve the host response to VL treatment, to find an effective dosage schedule is still a challenging task, which is not always successful. Thus, worse results than those obtained for AMB or MTF monotherapy were reported in 7 studies (Mutiso et al., Reference Mutiso, Macharia, Barasa, Taracha, Bourdichon and Gicheru2011; Shakya et al., Reference Shakya, Sane, Vishwakarma and Gupta2012a, Reference Shakya, Sane, Haq and Gupta2012b; Mwololo et al., Reference Mwololo, Mutiso, Macharia, Bourdichon and Gicheru2015; Hendrickx et al., Reference Hendrickx, Van den Kerkhof, Mabille, Cos, Delputte, Maes and and Caljon2017; Joice et al., Reference Joice, Yang, Farahat, Meeds, Feng, Li, Boykin, Wang and Werbovetz2017; Rebello et al., Reference Rebello, Andrade-Neto, Gomes, de Souza, Branquinha, Santos, Torres-Santos and D'Avila-Levy2019). Therapeutic schemes such as PAR (3.5 mg kg−1) + MEG (30 mg Sb kg−1) for dog model (Oliva et al., Reference Oliva, Gradoni, Cortese, Orsini, Ciaramella, Scalone, de Luna and Persechino1998), DIM (12.5 mg kg−1) + ART (12.5 mg kg−1) in mice (Mutiso et al., Reference Mutiso, Macharia, Barasa, Taracha, Bourdichon and Gicheru2011), and DIM (12.5 mg kg−1) + CHQ (12.5 mg kg−1) also applied in mice (Mwololo et al., Reference Mwololo, Mutiso, Macharia, Bourdichon and Gicheru2015) showed lower or the same effectivity achieved from the monotherapy with the reference drugs MEG, PAR or the experimental drugs used alone, such as DIM, ART or CHQ.
Considering the studies investigating the hamster model, 8 different drugs used in 11 therapeutic combinations were identified. Among these combinations, 7 were successful in reducing parasitism and increasing chemotherapy effectiveness when administered in subdoses (Kar et al., Reference Kar, Kar, Bhattacharya and Ghosh1993; Corral et al., Reference Corral, Serrano, Moreno, Torrado, Dominguez and Alunda2014; Hendrickx et al., Reference Hendrickx, Van den Kerkhof, Mabille, Cos, Delputte, Maes and and Caljon2017). In these studies, it was reported that drug combination was also effective in inhibiting parasite multiplication, transformation and infectivity (Kar et al., Reference Kar, Kar, Bhattacharya and Ghosh1993), as well as achieving parasitological cure (Kar et al., Reference Kar, Kar, Bhattacharya and Ghosh1993; Corral et al., Reference Corral, Serrano, Moreno, Torrado, Dominguez and Alunda2014) without cross-resistance in vivo or in vitro after repeated exposures to combined treatment (Hendrickx et al., Reference Hendrickx, Van den Kerkhof, Mabille, Cos, Delputte, Maes and and Caljon2017). This is a remarkable finding, especially considering that monotherapy strategies currently prescribed to treat VL have been associated with parasite cross-resistance to alternative or second-line leishmanicidal drugs, especially in endemic areas (Rijal et al., Reference Rijal, Ostyn, Uranw, Rai, Bhattarai, Dorlo, Beijnen, Vanaerschot, Decuypere, Dhakal, Das, Karki, Singh, Boelaert and Dujardin2013). Thus, the combination of drugs becomes relevant as a safe and effective alternative to reduce the dose and toxicity of the treatment, ensuring the desirable antiparasitic efficacy (Sundar et al., Reference Sundar, Sinha, Rai, Verma, Nawin, Alam, Chakravarty, Vaillant, Verma, Pandey, Kumari, Lal, Arora, Sharma, Ellis, Strub-Wourgaft, Balasegaram, Olliaro, Das and Modabber2011; Hendrickx et al., Reference Hendrickx, Van den Kerkhof, Mabille, Cos, Delputte, Maes and and Caljon2017). In this since, MEG and PAR coadministration was effective in reducing parasite load in the liver and bone marrow in naturally infected dogs, although this combination did not induce parasitological cure (Oliva et al., Reference Oliva, Gradoni, Cortese, Orsini, Ciaramella, Scalone, de Luna and Persechino1998). However, by attenuating disease severity, MEG plus PAR cannot be disregarded as an alternative combined treatment for natural canine leishmaniasis (Oliva et al., Reference Oliva, Gradoni, Cortese, Orsini, Ciaramella, Scalone, de Luna and Persechino1998). Although this experimental model has been little used, naturally infected dogs of varying breeds are excellent tools to investigate the therapeutic efficacy of drug combinations for VL. Accordingly, the variable genetic and immunological background modulates disease evolution and increases the heterogeneity of the research outcomes, characteristics that bring this model closer to the epidemiological reality associated with the domestic cycle of VL transmission (Quinnell et al., Reference Quinnell, Kennedy, Barnes, Courtenay, Dye, Garcez, Shaw, Carter, Thomson and Ollier2003; de Vasconcelos et al., Reference de Vasconcelos, Furtado, Belo, Morgado and Figueiredo2019).
In addition to the hamster model, 17 drugs administered in 28 different combinations were identified in VL mice models. In general, most studies obtained better therapeutic effects from drug combinations than monotherapy. Accordingly, the beneficial effects achieved from combined therapy were mainly associated with the improvement of the following parameters: half-life or drug retention (Bhattacharjee et al., Reference Bhattacharjee, Majumder, Majumdar, Choudhuri, Roy and Majumdar2015; Joice et al., Reference Joice, Yang, Farahat, Meeds, Feng, Li, Boykin, Wang and Werbovetz2017), subdoses efficacy (Carter et al., Reference Carter, Sundar, Spickett, Pereira and Mullen2003; Bhattacharjee et al., Reference Bhattacharjee, Majumder, Majumdar, Choudhuri, Roy and Majumdar2015; Khadem et al., Reference Khadem, Jia, Mou, Feiz Barazandeh, Liu, Keynan and Uzonna2017; Joice et al., Reference Joice, Yang, Farahat, Meeds, Feng, Li, Boykin, Wang and Werbovetz2017; Rebello et al., Reference Rebello, Andrade-Neto, Gomes, de Souza, Branquinha, Santos, Torres-Santos and D'Avila-Levy2019), immunomodulation (Shakya et al., Reference Shakya, Sane, Vishwakarma and Gupta2012a, Reference Shakya, Sane, Haq and Gupta2012b; Bhattacharjee et al., Reference Bhattacharjee, Majumder, Majumdar, Choudhuri, Roy and Majumdar2015; Khadem et al., Reference Khadem, Jia, Mou, Feiz Barazandeh, Liu, Keynan and Uzonna2017) and activation of oxidative defences (Carter et al., Reference Carter, Sundar, Spickett, Pereira and Mullen2003; Shakya et al., Reference Shakya, Sane, Vishwakarma and Gupta2012a, Reference Shakya, Sane, Haq and Gupta2012b). In addition, parasitological cure was achieved in mice models treated with CAL-101 (0.05 mg) + AMB (0.1 mg kg−1) (Khadem et al., Reference Khadem, Jia, Mou, Feiz Barazandeh, Liu, Keynan and Uzonna2017), and LPV (493.2 mg kg−1) + MTF (7.2 mg kg−1) (Rebello et al., Reference Rebello, Andrade-Neto, Gomes, de Souza, Branquinha, Santos, Torres-Santos and D'Avila-Levy2019). More than two-thirds of the studies reviewed reported low toxicity of the new drug combinations, downregulation of Th2/Treg cytokines (i.e. IL-4/IL-10) and upregulation of antiparasitic effectors, especially ROS and RNS and Th1 cytokines (i.e. IFN-γ, IL-12 and TNF). Thus, success in VL treatment was especially associated with the development of a more effective Th1 phenotype, which potentiated the ROS and RNS production and the elimination of intracellular amastigotes by macrophages (Kaur et al., Reference Kaur, Kaur, Garg, Mukherjee, Raina and Athokpam2008; Khadem et al., Reference Khadem, Jia, Mou, Feiz Barazandeh, Liu, Keynan and Uzonna2017). Considering the analysed pathological outcomes, it becomes evident that the reviewed studies direct drug combinations to stimulate the Th1 phenotype, a rational strategy considering that this phenotype is associated with greater host resistance against Leishmania spp. infection (Haldar et al., Reference Haldar, Banerjee, Naskar, Kalita, Islam and Roy2009; Mutiso et al., Reference Mutiso, Macharia, Barasa, Taracha, Bourdichon and Gicheru2011; Shakya et al., Reference Shakya, Sane, Vishwakarma and Gupta2012a, Reference Shakya, Sane, Haq and Gupta2012b; Bhattacharjee et al., Reference Bhattacharjee, Majumder, Majumdar, Choudhuri, Roy and Majumdar2015; Khadem et al., Reference Khadem, Jia, Mou, Feiz Barazandeh, Liu, Keynan and Uzonna2017). In fact, the immunomodulatory effects of drug combinations seem to be a convergent mechanism associated with the improvement of leishmanicidal defences in mice (Musa et al., Reference Musa, Noazin, Khalil and Modabber2010; Shakya et al., Reference Shakya, Sane, Vishwakarma and Gupta2012a, Reference Shakya, Sane, Haq and Gupta2012b). Thus, current evidence is consistent in demonstrating that curing VL depends simultaneously on the direct ability to kill the parasite and a protective immunological profile, aspects that were simultaneously stimulated in different pharmacological combinations used such as AMB + CAL (Khadem et al., Reference Khadem, Jia, Mou, Feiz Barazandeh, Liu, Keynan and Uzonna2017), MTF + TUF (Shakya et al., Reference Shakya, Sane, Haq and Gupta2012b), MTF + PAM3Cys (Shakya et al., Reference Shakya, Sane, Vishwakarma and Gupta2012a) and SAG + GA (Bhattacharjee et al., Reference Bhattacharjee, Majumder, Majumdar, Choudhuri, Roy and Majumdar2015).
Considering a critical interpretation of the evidence, the objective assessment of methodological quality indicated important elements of bias in the reviewed studies. Even considering the specificities of each research design in the context of the bias analysis, no study fulfilled all methodological criteria, with an average of 43.57% fulfilled criteria. In addition, the studies presented variable methodological score without a temporal influence (year of publication), indicating that elements of bias are systematically replicated in this area of parasitological research, despite methodological advances and the greater availability of more sensitive and specific analytical tools. Surprisingly, over half of the essential criteria to be reported in in vivo animal studies were neglected. There is no doubt that under-reported aspects such as randomization, allocation concealment and complete description of research results undermine the reproducibility, internal and external validity of the reviewed studies, limiting the reliability research evidence. Methodological bias analysis corroborated the low quality of research reports, showing high or unknown risk of bias for most studies and categories evaluated. In general, the description of baseline characteristics (characterization of models and experimental conditions) represented the criteria best performed by studies with murine models. However, studies using dogs had obvious limitations in describing these baseline characteristics. Considering that naturally infected dogs were used, the difficulty to delimit the aspects related to race, sex, weight, age and time of infection is justifiable, representing a methodological limitation inherent to this preclinical model. However, it is imperative that experimental models artificially constructed to simulate human VL overcome the bias factors previously identified from current scientific evidence. In this sense, by mapping the risk of bias in all investigated studies, this review provides objective support to delimit further studies with greater methodological rigor, providing unequivocal evidence on the relevance and effectiveness of drug combinations for VL treatment.
From this systematic review, we identified that despite exhibiting some risk of methodological bias, current evidence indicates that the combination of drugs with different mechanisms of action is potentially relevant to treat VL. In general, combinations based on MTF + CAL-101, MTF + LPV, AMB + ALL, AMB + PAR, TAA + SSG, TAA + ALO and TAA + PET achieved better therapeutic effects compared to monotherapy with the currently prescribed reference leishmanicidal drugs, such as SAG, MEG and SSG. From a mechanistic point of view, the improved therapeutic effects induced by the pharmacological associations were achieved by the combination of direct parasitic toxicity and the upregulation of immunological effectors linked to the Th1 protective phenotype, which increases the host's natural defences against infection by Leishmania spp. By acting in an additive or synergistic way, drug combinations can improve the management of VL, reducing the costs, doses, time and adverse effects associated with the treatment of this infection. Thus, drug combination offers a realistic opportunity to overcome parasitic resistance to leishmanicidal chemotherapy, alleviating infection severity and increasing the parasitological cure rates in Leishmania spp.-infected hosts. However, combinations based on SSG + ALO, PAR + MEG, DB766 + POS, DB766 + KET, DIM + CHQ, MTF + Pam3Cys, MTF + TUF, PV6 + SAG and DIM + ART did not show additional therapeutic benefits compared to monotherapy with AMB and MTF. Thus, drugs used in combination strategies must be carefully and rationally delimited, as they can trigger similar or even worse effects than monotherapy, characterizing relative or absolute contraindications to treat VL.
Supplementary material
The supplementary material for this article can be found at https://doi.org/10.1017/S0031182022000142
Financial support
This work was supported by the agencies: Fundação do Amparo à Pesquisa do Estado de Minas Gerais (FAPEMIG, processes APQ-01211-17, APQ-01895-16, PPM-00077-18 and PPM-00687-17) and Conselho Nacional de Desenvolvimento Científico e Tecnológico (CNPq, processes 303972/2017-3, 305093/2017-7, and 423594/2018-4). This study was financed in part by the Coordenação de Aperfeiçoamento de Pessoal de Nível Superior – Brasil (CAPES) – Finance Code 001. These organizations did not exert influence on the design/conduct of the study, collection/analysis/interpretation of the data, and preparation/review/approval of the manuscript.
Conflict of interest
None.
Ethical standards
Not applicable.