Introduction
The Mediterranean Sea, despite occupying only 0.8% of the global ocean's surface, is recognized as a hotspot of biodiversity, hosting around 7.5% of the world marine species (Coll et al., Reference Coll, Piroddi, Steenbeek, Kaschner, Ben Rais Lasram, Aguzzi, Ballesteros, Bianchi, Corbera, Dailianis, Danovaro, Estrada, Froglia, Galil, Gasol, Gertwagen, Gil, Guilhaumon, Kesner-Reyes, Kitsos, Koukouras, Lampadariou, Laxamana, López-Fé de la Cuadra, Lotze, Martin, Mouillot, Oro, Raicevich, Rius-Barile, Saiz-Salinas, San Vicente, Somot, Templado, Turon, Vafidis, Villanueva and Voultsiadou2010). Within this species richness, the Mediterranean ecosystems also hide an important component of the marine environment, represented by trophically transmitted helminth endoparasites (TTHs). These organisms constitute a dominant community of both the pelagic and benthic food webs. Several digenean trematodes, cestodes, nematodes and acanthocephalans, previously listed (Euzet, Reference Euzet1959; Petter and Radujkovic, Reference Petter and Radujkovic1989; Bona et al., Reference Bona, Buriola, Cerioni, Di Cave, Orecchia, Paggi, Minelli, Ruffo and La Posta1995; Paggi, Reference Paggi and Rellini2008; Radujkovic and Sundic, Reference Radujkovic and Sundic2014), constitute the TTHs biodiversity of Mediterranean Sea. However, although recent decades have seen a considerable progress in the taxonomic classification of marine parasites through an integrative approach, involving both morphological and molecular methods, a significant number of TTHs species still remains unidentified, and our knowledge of their ecology is incomplete.
This review focuses on the current state of knowledge on the biodiversity and ecology of certain TTHs among nematodes and cestodes from the Mediterranean Sea, whose taxonomic status has been assessed by both morphological and genetic/molecular methodologies. The chosen TTHs exhibit complex life cycles, with free-living phases and use of several host organisms at different trophic levels. Crustaceans, molluscs and fish can act as intermediate and paratenic hosts, while large organisms of mid to high trophic levels, such as cetaceans, sea turtles, large teleosts and elasmobranchs, are definitive hosts.
The Mediterranean ecosystem faces a wide range of anthropogenic stressors, such as global warming, overexploitation of fish resource, bycatch, intense maritime transport, eutrophication and land-based pollutants/contaminants (Mandić and Piraino, Reference Mandić and Piraino2023). There is increasing evidence that these stressors dramatically affect the demography, migration patterns and spawning sites of marine organisms, including the hosts involved in the life cycle of the TTHs here considered. Consequently, these changes could also impact the dynamics of parasite populations (Marcogliese, Reference Marcogliese2005; Lafferty et al., Reference Lafferty, Allesina, Arim, Briggs, De Leo, Dobson, Dunne, Johnson, Kuris, Marcogliese, Martinez, Memmott, Marquet, McLaughlin, Mordecai, Pascual, Poulin and Thieltges2008; Timi and Poulin, Reference Timi and Poulin2020). Understanding the situation and dynamics of TTHs–host associations in the Mediterranean basin can offer a further perspective in the monitoring of the overall ‘health status’ of this marine environment, and estimate its vulnerability to various human-induced pressures.
We first present the rationale behind choosing certain groups of TTHs as ‘sentinel’ species. Then, by examining the ecological aspects of the chosen TTHs, the review critically evaluates their significance as indicators of trophic web functioning and stability in the Mediterranean Sea. Indeed, being intimately related to trophic transmission and specific to their hosts, these helminth endoparasites require stable trophic webs, as well as a wide range and population size of suitable hosts to complete their life cycles (Marcogliese, Reference Marcogliese2005; Mattiucci and Nascetti, Reference Mattiucci and Nascetti2008). Any anthropogenic stressor that could lead to decline in the host populations of the chosen TTHs could also impact the population size of their endoparasites (Marcogliese, Reference Marcogliese2005). As a consequence, a loss of genetic variability can occur in the parasites' gene pool as the result of genetic drift phenomena (Bullini et al., Reference Bullini, Nascetti, Paggi, Orecchia, Mattiucci and Berland1986; Mattiucci and Nascetti, Reference Mattiucci and Nascetti2008). In this regard, the review aims to provide examples of temporal and spatial variations in infection levels and genetic variability of some among the chosen TTHs. Estimates of parasitic load and genetic variability of some TTHs are here proposed as indirect indicators of trophic web stability of marine ecosystem.
Furthermore, the host–parasite associations of the chosen TTHs can be significantly influenced by abiotic environmental factors, which are impacted by ongoing climate change (Palm, Reference Palm and Mehlhorn2011). Indeed, the rising sea levels and increasing temperatures in Mediterranean waters (Marriner et al., Reference Marriner, Kaniewski, Pourkerman and Devillers2022) can trigger shifts in water circulation and variation in salinity. These, among other abiotic perturbations, can directly affect the hatching of eggs and the first free-living larval stage of TTHs, as well as influence the presence and abundance of first intermediate hosts (i.e. invertebrates) of these parasites. These same environmental perturbations can also alter the migratory routes for feeding and/or reproduction of both intermediate/paratenic and definitive hosts of TTHs. In this context, this study aims to highlight the role of some TTHs as an ecological tool for monitoring the consequences of warming Mediterranean marine environment. Finally, the review aims to provide examples, so far existing, where TTHs would serve as environmental indicators of the impact of pollutants originating from offshore sea activities and coastal areas.
What are criteria to choose certain TTHs as ‘sentinel’ parasites?
To date, the use of marine parasites as biological indicators in Mediterranean waters has primarily centred on their linkage to fisheries. In this context, distribution and relative proportions of parasite species with known geographical ranges have been employed as part of a holistic approach, as biological tags for identifying fish stocks (Palm, Reference Palm and Mehlhorn2011; Timi and Mackenzie, Reference Timi and Mackenzie2015; Mattiucci et al., Reference Mattiucci, Cimmaruta, Cipriani, Abaunza, Bellisario and Nascetti2015a). Indeed, some TTHs (i.e. Anisakis spp. and trypanorhynch larvae) proved to be valid indicators of distinct Mediterranean biological and genetic stocks as opposed to those from the NE Atlantic waters, of pelagic and demersal fish species, such as the European hake Merluccius merluccius, the Atlantic horse mackerel Trachurus trachurus, the swordfish Xiphias gladius (reviewed in Mattiucci et al., Reference Mattiucci, Cimmaruta, Cipriani, Abaunza, Bellisario and Nascetti2015a) and the European pilchard Sardina pilchardus (Caballero-Huertas et al., Reference Caballero-Huertas, Palomba, Frigola-Tepe, Muñoz, Mattiucci and Viñas2022). In the use of parasites as tags, phylogeographic aspects of the parasite species have also been included to determine fish host populations (Mattiucci et al., Reference Mattiucci, Giulietti, Paoletti, Cipriani, Gay, Levsen, Klapper, Karl, Bao, Pierce and Nascetti2018a). However, comparatively, less emphasis has been spent on exploring the potential use of marine TTHs as indicators of trophic web stability and ecosystem functioning.
The TTHs here considered were chosen based on the following criteria: (1) being endemic or widely distributed in the Mediterranean Sea. They should be easily visible and collectable, with documented presence in Mediterranean regions and have been reported in scientific literature or studied within the past 2 decades, in order to follow, whenever possible, their population dynamics; (2) having a known life cycle in its general pathway. The chosen parasite species should have, at least, confirmed definitive and/or intermediate/paratenic hosts inhabiting the Mediterranean Sea. Further, it should be easily identifiable at the trophic levels (feeding relationships) of the host species involved in the parasite's life cycle; (3) being specialist rather than generalist species vs definitive hosts: i.e. the parasitic species should display a preference for definitive hosts, rather than infecting a wide range of host species not ecologically correlated; (4) not having a high pathogenic role to their hosts; (5) being common in their definitive host or/and intermediate/paratenic hosts. Their density should have been studied, possibly, even at spatial scale; (6) being well-characterized at both the morphological and molecular level. The chosen species should have been studied by an integrative approach, including both morphological and genetic methodologies, allowing their clear recognition; (7) whenever possible, having access to historical comparative data on their occurrence in Mediterranean waters.
What are the most reported TTHs from organisms of mid-high trophic levels?
The existing literature consistently highlights that the predominant taxa of TTHs in Mediterranean waters, aligning with those specified indicator criteria, includes nematodes and cestodes. In the last decades, these helminths have attracted scientific attention due to their constant presence, mainly at their larval stage, in commercially important marine organisms, such as fish and molluscs, from the Mediterranean Sea (Cancellieri et al., Reference Cancellieri, Amicone, Cicero, Milani, Mosca, Palomba, Mattiucci and Bonaiuto2023). Extensive studies of certain TTHs have been motivated by the zoonotic role played by some nematode species (i.e. Anisakis pegreffii, Campana-Rouget and Biocca, Reference Campana-Rouget and Biocca1955; see Nascetti et al., Reference Nascetti, Paggi, Orecchia, Smith, Mattiucci and Bullini1986) (reviewed in Mattiucci et al., Reference Mattiucci, Cipriani, Levsen, Paoletti and Nascetti2018b), which are widespread in Mediterranean wild fisheries. Other TTHs such as larval Hysterothylacium, Sulcascaris and/or trypanorhynch cestodes, even though not considered zoonotic, could by their presence make seafood unappealing to the consumer, thereby compromising the quality and value of the products (Bao et al., Reference Bao, Cipriani, Giulietti, Drivenes and Levsen2020; Rudders et al., Reference Rudders, Roman, Fisher and McDowell2023). Sporadic reports of human infections by Hysterothylacium aduncum (Rudolphi, 1802) Deardorff and Overstreet, 1981 exist in literature (Yagi et al., Reference Yagi, Nagasawa, Ishikura, Nakagawa, Sato, Kikuchi and Ishikura1996). However, in these records, pathological findings and molecular identification of the recovered parasite were not described. Similarly, metacestodes of the chosen TTHs are not considered zoonotic parasites. However, there is speculation that Trypanorhyncha larvae, i.e. Gymnorhynchus gigas (Cuvier, 1817) Rudolphi, 1819 and Molicola horridus (Goodsir, 1841) Dollfus, 1935, invading fish host muscles, might release proteins triggering allergic reactions in humans (Gòmez-Morales et al., Reference Gòmez-Morales, Ludovisi, Giuffra, Manfredi, Piccolo and Pozio2008). Although these proteins have not yet been identified in marine metacestodes, it is generally known that proteins from zoonotic larval helminths involved in host tissue invasion, such as in the infection by A. pegreffii, could lead to IgE-hypersensitization in the immune response of accidental human hosts (Mattiucci et al., Reference Mattiucci, Colantoni, Crisafi, Mori-Ubaldini, Caponi, Fazii, Nascetti and Bruschi2017a; Palomba et al., Reference Palomba, Paoletti, Colantoni, Rughetti, Nascetti and Mattiucci2019, Reference Palomba, Rughetti, Mignogna, Castrignanò, Rahimi, Masuelli, Napoletano, Pinna, Giorgi, Santoro, Schininà, Maras and Mattiucci2023a).
In addition, over the last 30 years, the implementation of molecular epidemiological surveys on these TTHs, carried out in wild fisheries, bycatches and stranded animals along the Mediterranean coasts, has substantially enhanced our knowledge regarding their ecological aspects. This includes life cycles, host range and geographical distribution within Mediterranean waters (Palm et al., Reference Palm, Waeschenbach, Olson and Littlewood2009; Mattiucci et al., Reference Mattiucci, Cipriani, Levsen, Paoletti and Nascetti2018b, Reference Mattiucci, Bello, Paoletti, Webb, Timi, Levsen, Cipriani and Nascetti2019; Marcer et al., Reference Marcer, Tosi, Franzo, Vetri, Ravagnan, Santoro and Marchiori2020). Many TTHs species rely on cetaceans, sea turtles, elasmobranchs and large teleostean fish as definitive hosts. Studies on these parasites in such ‘charismatic’ hosts have drawn particular attention, since these hosts are recognized as ‘flagship species’, many of which are endangered, protected or subject to specific conservation measures.
In light of these considerations, significant scientific works have been generated on these TTHs, which are the central focus of this review. Thus, we used the bulk of published information on chosen key parasites to outline their current distribution (Fig. 1), life cycle (Fig. 2) and infection values (Fig. 3) in the Mediterranean basin, and provide examples concerning their possible use as ecological indicators of the Mediterranean marine ecosystem.

Figure 1. Trophically transmitted helminth endoparasite (TTHs) distributions mapped into the FAO (major fishing regions) (area 37) of the Mediterranean Sea.

Figure 2. Schematic representation of the life cycle of the TTHs here considered. (A) Anisakidae; (B) Raphidascarididae; (C) Gymnorhynchidae; (D) Lacistorhynchidae; (E) Calliobothriidae; (F) Onchobothriidae. On the right, anthropogenic stressors, affecting the trophic web levels through which the TTHs move their life cycle, are reported.
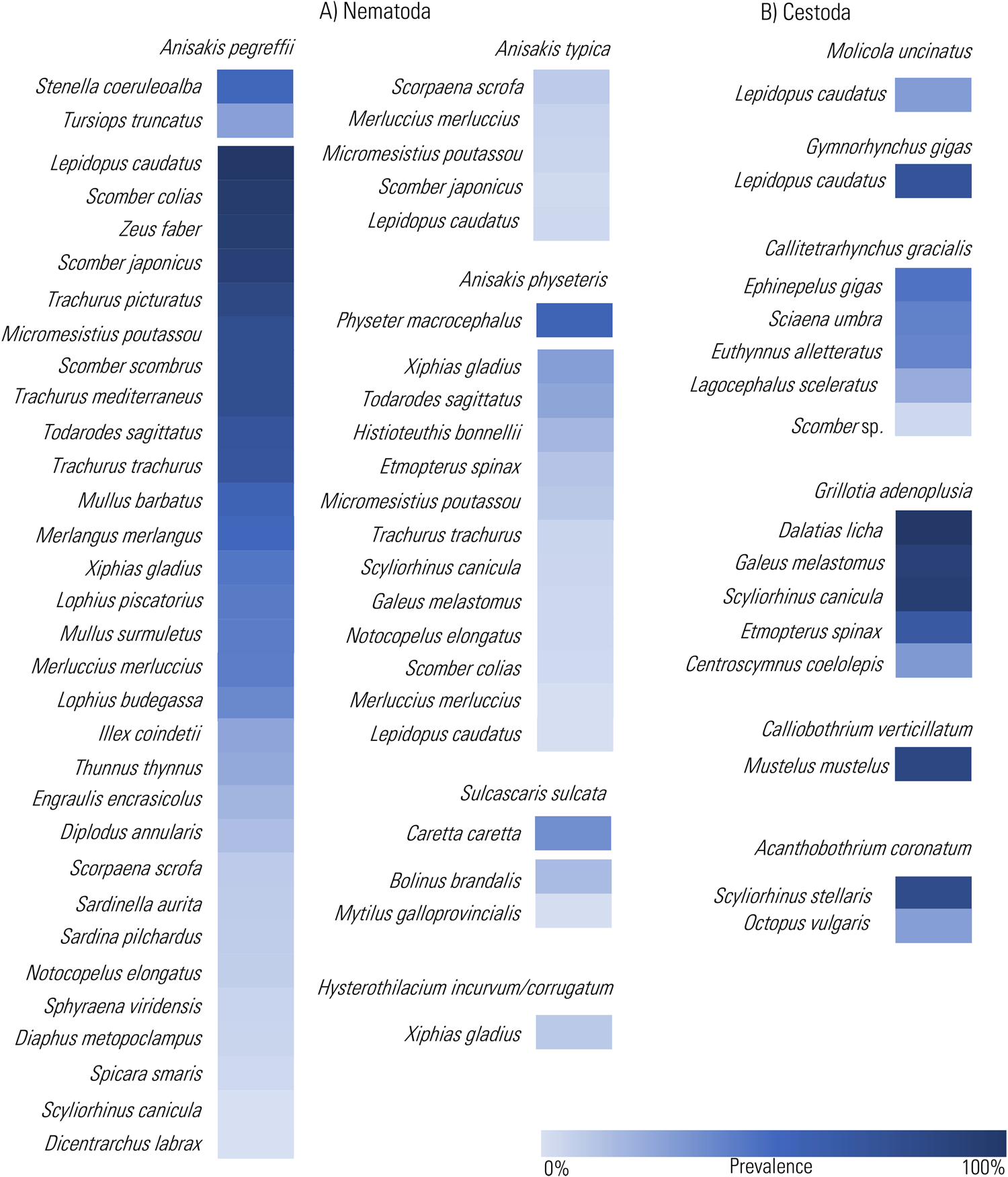
Figure 3. Heat Map representation of the prevalence values of the infection by the chosen TTH species among nematodes (A) and cestodes (B), so far recorded in definitive and intermediate/paratenic hosts from the Mediterranean Sea (data from cited references in Supplementary Tables 1 and 2 and through the text). The prevalence values are presented in decreasing order.
Chosen TTHs among nematodes: biodiversity and ecology
The predominant groups among nematodes found at adult stage in large organisms of the marine Mediterranean mid-upper food webs are species of ascaridoid nematodes belonging to the genera Anisakis, Sulcascaris and Hysterothylacium. A parasite-host list is reported in the supplementary Table 1. These genera include species having a complex life cycle, involving various marine organisms at different levels of the pelagic and/or benthic trophic webs (Fig. 2A and B). Their life cycle encompasses 4 larval stages before they reach the adult form (Klimpel and Palm, Reference Klimpel and Palm2011) (Fig. 2A). Adults of Anisakis species live in the gastric chambers of marine mammals, mainly cetaceans; Sulcascaris species are found in sea turtles; while Hysterothylacium species lodge in the stomach of large teleostean fish. Female worms release fertilized unembryonated eggs into the seawater through the stools of their definitive hosts. They embryonate in seawater, maturing within the egg. The larvae moult once or twice and emerge as ensheathed, free-swimming form (see Gomes et al., Reference Gomes, Quiazon, Itoh, Fujise and Yoshinaga2023). After a so far poorly defined free-living stage, ascaridoid larvae of these genera continue their life cycle when ingested by the first intermediate hosts. These are mostly small crustaceans, including krill for Anisakis spp. (Køie et al., 1993, 2001). Euphausiid species such as the North Pacific krill Euphausia pacifica, E. similis, Nyctiophanes couchii and the Arctic krill Thysanoessa raschii have been found infected by Anisakis simplex (s.s.) (Rudolphi, 1809) (see Nascetti et al., Reference Nascetti, Paggi, Orecchia, Smith, Mattiucci and Bullini1986) and A. pegreffii along the Spanish Atlantic coast (Gregori et al., Reference Gregori, Roura, Abollo, González and Pascual2015; Cresson et al., Reference Cresson, Bourgau, Cordier, Couvreur, Rouquette and Gay2023). Pelagic and benthic crustaceans such as harpacticoid copepods, amphipods, isopods and mysids have been reported as intermediate hosts of Hysterothylacium from the Atlantic and Pacific Oceans (Køie, Reference Køie1993; Klimpel and Rückert, Reference Klimpel and Rückert2005). However, knowledge concerning the first intermediate hosts of Anisakis and Hysterothylacium in Mediterranean waters is currently lacking. For Sulcascaris, bivalve molluscs and gasteropods serve as intermediate hosts in Mediterranean (Marcer et al., Reference Marcer, Tosi, Franzo, Vetri, Ravagnan, Santoro and Marchiori2020; Santoro et al., Reference Santoro, Marchiori, Palomba, Degli Uberti, Marcer and Mattiucci2020a, Reference Santoro, Palomba and Modica2022a). In particular, the Mediterranean scallop Pecten jacobeus and the queen scallop Aequipecten opercularis were recognized as intermediate hosts in the Adriatic Sea (Marcer et al., Reference Marcer, Tosi, Franzo, Vetri, Ravagnan, Santoro and Marchiori2020). Similarly, the Mediterranean mussel Mytilus galloprovincialis and the purple dye murex Bolinus brandaris were found to serve as intermediate hosts in the Tyrrhenian Sea (Santoro et al., Reference Santoro, Marchiori, Palomba, Degli Uberti, Marcer and Mattiucci2020a, Reference Santoro, Palomba and Modica2022a) (Supplementary Table 1; Fig. 2A). These host species are commonly found as part of the diet of loggerhead sea turtle Caretta caretta in these regions (Lazar et al., Reference Lazar, Gračan, Katić, Zavodnik, Jaklin and Tvrtković2010; Santoro et al., Reference Santoro, Marchiori, Iaccarino, Uberti, Cassini, Di Nocera, Cerrone, Galiero and Marcer2019).
In the case of Anisakis and Hysterothylacium, the first intermediate hosts harbouring the third stage larvae (L3) are then eaten by various paratenic hosts, such as pelagic, demersal, benthic fish and cephalopods (Mattiucci et al., Reference Mattiucci, Cipriani, Levsen, Paoletti and Nascetti2018b; Tedesco et al., Reference Tedesco, Gustinelli, Caffara, Patarnello, Terlizzi and Fioravanti2018) (Supplementary Table 1; Fig. 2A and B). Other marine organisms, such as ctenophores, chaetognaths, polychaetes and ophiuroids, can also become infected by ingesting infected crustaceans and act as second intermediate or transport hosts for Hysterothylacium (Køie, Reference Køie1993). When L3 are ingested by paratenic hosts, they can either remain encapsulated outside the visceral cavity, or migrate to the host muscle tissue in the case of A. pegreffii, A. simplex (s.s.) and Anisakis typica (s.l.) (Diesing, 1860) (e.g. Mattiucci et al., Reference Mattiucci, Cipriani, Levsen, Paoletti and Nascetti2018b; Levsen et al., Reference Levsen, Svanevik, Cipriani, Mattiucci, Gay, Hastie, Bušelić, Mladineo, Karl, Ostermeyer, Buchmann, Højgaard, González, Pascual and Pierce2018a; Cipriani et al., Reference Cipriani, Giulietti, Shayo, Storesund, Bao, Palomba, Mattiucci and Levsen2022a). In contrast, there is evidence that the L3 stages of Hysterothylacium and some anisakid species (i.e. Anisakis physeteris Baylis, 1923) do not commonly migrate into the host's muscle tissue. Anisakis spp. and Hysterothylacium spp. at L3 stage occur in several Mediterranean pelagic and mesopelagic fish and squid species (Supplementary Table 1; Fig. 2A). Co-infections with L3 Anisakis and Hysterothylacium in the same fish species in Mediterranean waters have been frequently reported (see Supplementary Table 1).
Fish and squid hosts trophically pass the parasite to their definitive hosts at the higher levels of the pelagic/benthic food webs. Once within suitable definitive hosts, namely cetaceans for Anisakis spp. and teleostean fish for Hysterothylacium spp., the L3 moults to L4 and then matures into the adult form of the parasite.
Currently, Mediterranean waters harbour at least 12 cetacean species (Notarbartolo di Sciara, Reference Notarbartolo di Sciara2016). The Anisakis species so far detected in stranded cetaceans along the coasts of the Mediterranean Sea are A. pegreffii, A. simplex (s.s.), A. ziphidarum Paggi et al., Reference Paggi, Nascetti, Webb, Mattiucci, Cianchi and Bullini1988, A. typica sp. A and A. physeteris (Supplementary Table 1).
The TTH A. pegreffii meets the criteria for a potential ‘ecological indicator’ of anthropogenic changes in the Mediterranean Sea. Initially described at the adult stage in the Mediterranean monk seal Monachus monachus by Campana-Rouget and Biocca, Reference Campana-Rouget and Biocca1955, it has not been subsequently detected in this host species. However, it is common among Mediterranean populations of cetaceans in the Delphinidae family (Supplementary Table 1). Mature female and male A. pegreffii have been frequently genetically identified in various delphinid species, such as the striped dolphin Stenella coeruleoalba, the bottlenose dolphin Tursiops truncatus and the Risso's dolphin Grampus griseus, stranded in different localities across the Central and South Mediterranean Sea (Blažekovićet al., Reference Blažeković, Lepen Pleić, Đuras, Gomerčić and Mladineo2015; Mattiucci et al., Reference Mattiucci, Cipriani, Levsen, Paoletti and Nascetti2018b; Cipriani et al., Reference Cipriani, Palomba, Giulietti, Marcer, Mazzariol, Santoro, Alburqueque, Covelo, López, Santos, Pierce, Brownlow, Davison, McGovern, Frantzis, Alexiadou, Højgaard, Mikkelsen, Paoletti, Nascetti, Levsen and Mattiucci2022b). A few immature A. pegreffii have been genetically identified in a dwarf sperm whale Kogia sima, off the Tyrrhenian Sea coast (Santoro et al., Reference Santoro, Di Nocera, Iaccarino, Cipriani, Guadano Procesi, Maffucci, Hochscheid, Blanco, Cerrone, Galiero, Nascetti and Mattiucci2018a), as well as, in the Cuvier's beaked whale Ziphius cavirostris from the Aegean Sea (Cipriani et al., Reference Cipriani, Palomba, Giulietti, Marcer, Mazzariol, Santoro, Alburqueque, Covelo, López, Santos, Pierce, Brownlow, Davison, McGovern, Frantzis, Alexiadou, Højgaard, Mikkelsen, Paoletti, Nascetti, Levsen and Mattiucci2022b). In addition, few adults were found in the sperm whale Physeter macrocephalus (Mattiucci et al., Reference Mattiucci, Nascetti, Bullini, Orecchia and Paggi1986) (Supplementary Table 1). These findings support the hypothesis that A. pegreffii exhibits host preference and thus an evolutionary adaptation, within ‘oceanic dolphins’ rather than for other cetacean families, as the result of host–parasite co-speciation events (Mattiucci and Nascetti, Reference Mattiucci and Nascetti2008).
Anisakis physeteris is another chosen taxon in this review. Over the past decade, A. physeteris has consistently been found in stranded sperm whales along the Tyrrhenian and Ionian coasts (Mattiucci et al., Reference Mattiucci, Nascetti, Bullini, Orecchia and Paggi1986; Cipriani et al., Reference Cipriani, Palomba, Giulietti, Marcer, Mazzariol, Santoro, Alburqueque, Covelo, López, Santos, Pierce, Brownlow, Davison, McGovern, Frantzis, Alexiadou, Højgaard, Mikkelsen, Paoletti, Nascetti, Levsen and Mattiucci2022b), the Adriatic Croatian coast (Blažeković et al., Reference Blažeković, Lepen Pleić, Đuras, Gomerčić and Mladineo2015) and the Aegean Sea (Cipriani et al., Reference Cipriani, Palomba, Giulietti, Marcer, Mazzariol, Santoro, Alburqueque, Covelo, López, Santos, Pierce, Brownlow, Davison, McGovern, Frantzis, Alexiadou, Højgaard, Mikkelsen, Paoletti, Nascetti, Levsen and Mattiucci2022b) (Supplementary Table 1). Additionally, massive infections by this parasite species were noted during 2 stranding events of sperm whales in 2009 and 2013 along the southeastern coast of Italy (Mazzariol et al., Reference Mazzariol, Centelleghe, Cozzi, Povinelli, Marcer, Ferri, Di Francesco, Badagliacca, Profeta, Olivieri, Guccione, Cocumelli, Terracciano, Troiano, Beverelli, Garibaldi, Podestà, Marsili, Fossi, Mattiucci, Cipriani, De Nurra, Zaccaroni, Rubini, Berto, de Quiros, Fernandez, Morell, Giorda, Pautasso, Modesto, Casalone and Di Guardo2018). In the 2009 event, only 2 of 9 stranded sperm whales along the Apulia coast were found infected by a large amount (several hundreds of worms) of A. physeteris. In the subsequent event on the Adratic Sea coast, all 3 stranded sperm whales were infected with over 600 specimens of the parasite (Mazzariol et al., Reference Mazzariol, Centelleghe, Cozzi, Povinelli, Marcer, Ferri, Di Francesco, Badagliacca, Profeta, Olivieri, Guccione, Cocumelli, Terracciano, Troiano, Beverelli, Garibaldi, Podestà, Marsili, Fossi, Mattiucci, Cipriani, De Nurra, Zaccaroni, Rubini, Berto, de Quiros, Fernandez, Morell, Giorda, Pautasso, Modesto, Casalone and Di Guardo2018; Cipriani et al., Reference Cipriani, Palomba, Giulietti, Marcer, Mazzariol, Santoro, Alburqueque, Covelo, López, Santos, Pierce, Brownlow, Davison, McGovern, Frantzis, Alexiadou, Højgaard, Mikkelsen, Paoletti, Nascetti, Levsen and Mattiucci2022b). Adult worms of A. physeteris were also identified in an individual of a dwarf sperm whale stranded along the Central Tyrrhenian Sea coast (Santoro et al., Reference Santoro, Di Nocera, Iaccarino, Cipriani, Guadano Procesi, Maffucci, Hochscheid, Blanco, Cerrone, Galiero, Nascetti and Mattiucci2018a). A host preference for odontocetes belonging to the Physeteridae family is evident for A. physeteris, which could be attributed to host–parasite co-evolutionary aspects and host feeding ecology (Mattiucci and Nascetti, Reference Mattiucci and Nascetti2008). Anisakis physeteris has been sporadically found as L3 in fish species, at low infection rates. Large, deep-dwelling cephalopods might act as reservoir hosts for this anisakid species, playing a crucial role in completing its life cycle, maintaining its distribution and infection levels. Indeed, a high parasitic load of the L3 stage of A. physeteris has been genetically recognized in deep sea squids of the Mediterranean Sea belonging to the family Histioteuthiidae (Palomba et al., Reference Palomba, Mattiucci, Crocetta, Osca and Santoro2021a). These squids are preferred prey items of sperm whales. This finding, along with rare records of L3 of A. physeteris in pelagic and demersal fish species, suggests that A. physeteris has a life cycle typical of bathypelagic waters (Mattiucci et al., Reference Mattiucci, Cipriani, Levsen, Paoletti and Nascetti2018b) (Supplementary Table 1). Supporting this hypothesis, stranded individuals of sperm whales in 2013 had stomachs filled with beaks of the simply angel squid Ancistroteuthis lichtensteinii, the jumbo squid Dosidicus gigas and the umbrella squid Histioteuthis bonnellii (Mattiucci and Garibaldi, personal observation). Similarly, a dwarf sperm whale stranded on Tyrrhenian Sea coast with a high parasitic burden of A. physeteris had beaks of the umbrella squid and the reverse jewel squid H. reversa (Santoro et al., Reference Santoro, Di Nocera, Iaccarino, Cipriani, Guadano Procesi, Maffucci, Hochscheid, Blanco, Cerrone, Galiero, Nascetti and Mattiucci2018a). Likewise, a stranded sperm whale from Greece infected by A. physeteris contained up to 30 000 beaks of 12 squid species known to occur in the Mediterranean Sea (Rendell and Frantzis, Reference Rendell and Frantzis2016).
An additional anisakid taxon that appears to meet the aforementioned indicator criteria is A. typica (s.l.). Initially genetically characterized in Central Atlantic waters (Brazilian coast) and from Indian Ocean (Mattiucci et al., Reference Mattiucci, Paggi, Nascetti, Portes Santos, Costa, Di Beneditto, Ramos, Argyrou, Cianchi and Bullini2002), this morphospecies has recently been recognized as a complex of sibling species, as inferred from genetic and molecular markers (see Cipriani et al., Reference Cipriani, Giulietti, Shayo, Storesund, Bao, Palomba, Mattiucci and Levsen2022a). In fact, 2 distinct sister species, provisionally designated as A. typica sp. A and A. typica sp. B, were genetically distinguished (Cipriani et al., Reference Cipriani, Giulietti, Shayo, Storesund, Bao, Palomba, Mattiucci and Levsen2022a). Both species were sympatrically identified at the larval stage in fish species from the Indian Ocean. This complex of species is distributed in warmer temperate and tropical waters of the world, spanning a geographical range from 35–40°N to 36°S. According to the updated finding, it appears that only 1 sibling species, i.e. A. typica sp. A, is currently present in the Mediterranean Sea. So far, it has been found to occur in warmer Mediterranean waters, such as off the far southeastern coast of Crypus, Crete and the Aegean Sea (Mattiucci et al., Reference Mattiucci, Abaunza, Ramadori and Nascetti2004, Reference Mattiucci, Giulietti, Paoletti, Cipriani, Gay, Levsen, Klapper, Karl, Bao, Pierce and Nascetti2018a, Reference Mattiucci, Cipriani, Levsen, Paoletti and Nascetti2018b) and from the Tunisian coast (Farjallah et al., Reference Farjallah, Slimane, Busi, Paggi, Amor, Blel, Said and D'Amelio2008). This species has been found at the adult stage in a striped dolphin from Cyprus (Mattiucci and Nascetti, Reference Mattiucci and Nascetti2008) and at larval stage in fish hosts of pelagic and mesopelagic domain (Mattiucci et al., Reference Mattiucci, Abaunza, Ramadori and Nascetti2004, Reference Mattiucci, Cipriani, Levsen, Paoletti and Nascetti2018b; Cipriani et al., Reference Cipriani, Sbaraglia, Paoletti, Giulietti, Bellisario, Palomba, Bušelić, Mladineo, Nascetti and Mattiucci2018a) (Supplementary Table 1; Fig. 3).
Concerning the occurrence of other species of Anisakis in Mediterranean Sea, records of A. ziphidarum are sporadic (see Mattiucci et al., Reference Mattiucci, Cipriani, Levsen, Paoletti and Nascetti2018b). Its geographical range is mainly linked to the distribution of its definitive hosts, i.e. the beaked whales (see Mattiucci et al., Reference Mattiucci, Cipriani, Levsen, Paoletti and Nascetti2018b). Its first record in the Mediterranean Sea was in an individual of the Cuvier's beaked whale off the Ionian coast (Paggi et al., Reference Paggi, Nascetti, Webb, Mattiucci, Cianchi and Bullini1998) (Supplementary Table 1). It is rarely reported, at L3 stage, in pelagic and demersal fish species, worldwide (see Mattiucci et al., Reference Mattiucci, Cipriani, Levsen, Paoletti and Nascetti2018b). Instead, in the Mediterranean basin, the species was genetically recognized in the deep-dwelling myctophid meso-benthopelagic spothead lantern fish Diaphus metopoclampus caught in the eastern Ionian Sea (Gaglio et al., Reference Gaglio, Battaglia, Costa, Cavallaro, Cammilleri, Graci, Buscemi, Ferrantelli, Andaloro and Marino2018) (Supplementary Table 1). Habitat modelling for populations of the Cuvier's beaked whale in the Mediterranean Sea (Podestà et al., Reference Podestà, Azzellino, Cañadas, Frantzis, Moulins, Rosso, Tepsich and Lanfredi2016) confirms its preference for deep waters (>200 m). These findings suggest that the life cycle of A. ziphidarum involves deep sea species, such as deep-sea squids. Its scarcity in Mediterranean records (Supplementary Table 1) can be attributed to the rare populations of beaked whales, the suitable definitive hosts of A. ziphidarum (Mattiucci and Nascetti, Reference Mattiucci and Nascetti2008) so far recorded in the region (IUCN, 2020), as well as to the limited parasitological studies so far focused on deep sea species. Therefore, this TTH parasite was not chosen as possible sentinel parasite in this review.
Anisakis simplex (s.s.) has an Arctic-Boreal distribution (see Mattiucci et al., Reference Mattiucci, Cipriani, Levsen, Paoletti and Nascetti2018b), and has been rarely documented in Mediterranean waters. Here, A. simplex (s.s.) has been occasionally detected at the larval stage in several fish species in the Alboran Sea, the westernmost portion of the Mediterranean Sea (see Mattiucci et al., Reference Mattiucci, Cipriani, Levsen, Paoletti and Nascetti2018b; Molina-Fernández et al., Reference Molina-Fernández, Adroher and Benítez2018; Roca-Geronès et al., Reference Roca-Geronès, Alcover, Godínez-González, Montoliu and Fisa2021), which is considered as part of the Atlantic waters than the Mediterranean Sea (Hidalgo et al., Reference Hidalgo, Annane, Filali, Mekhazni, Ferhani, Mennad, Mattiucci, Cariani, Idrissi, Mokhtar, Wahbi, Giraldez, Garcia, Johnstone, Laiz-Carrión, Abaunza, Pérez, Garcia, Lafuente, Sanchez, Sammartino, Dominguez, Palomba, Khemeri, Gaamour, Feki, Fiorentino, Farrugio, Vasconcellos and Hernández2018). Spot occurrence of A. simplex (s.s.) has been also documented in the eastern part of the Mediterranean Sea. The presence of this species in the basin is likely linked to the migratory routes of the intermediate/definitive hosts transporting the parasite, such as the bluefin tuna Thunnus thynnus and the striped dolphin (Mladineo and Poljak, Reference Mladineo and Poljak2014; Blažeković et al., Reference Blažeković, Lepen Pleić, Đuras, Gomerčić and Mladineo2015). Adult specimens of A. simplex (s.s.) are extremely rare in the Mediterranean. This is likely due to the Mediterranean Sea temperature as a limiting abiotic factor for the eggs hatching and first stage larval survival of the parasite (Gomes et al., Reference Gomes, Quiazon, Itoh, Fujise and Yoshinaga2023). Only 1 adult, found in co-infection with several specimens of A. pegreffii, was recorded in a striped dolphin from off Sicilian coast (Cavallero et al., Reference Cavallero, Costa, Caracappa, Gambetta and D'Amelio2014) (Supplementary Table 1).
Among the endoparasites of cold-blooded marine animals, the anisakid Sulcascaris sulcata (Rudolphi, 1819) Hartwich, 1957 has been chosen in this review. Adults of Sulcascaris have been described in the gastric lumen of 3 marine turtle species: the loggerhead sea turtle, the green turtle Chelonia mydas and Kemp's ridley sea turtle Lepidochelys kempii in Atlantic and Pacific waters (Berry and Cannon, Reference Berry and Cannon1981). In the Mediterranean Sea, 3 sea turtle species (i.e. the leatherback turtle Dermochelys coriacea, the green turtle and the loggerhead turtle) have been recorded (Casale and Margaritoulis, Reference Casale and Margaritoulis2010). From this basin, S. sulcata has been reported in loggerhead sea turtles from the Adriatic (Manfredi et al., Reference Manfredi, Piccolo and Meotti1998; Gračan et al., Reference Gračan, Buršic, Mladineo, Kučinic, Lazar and Lackovic2012; Santoro et al., Reference Santoro, Marchiori, Iaccarino, Uberti, Cassini, Di Nocera, Cerrone, Galiero and Marcer2019; Marangi et al., Reference Marangi, Carlino, Profico, Olivieri, Totaro, Furii, Marzano and Papini2020; Gentile et al., Reference Gentile, Amato, Gustinelli, Fioravanti, Gambino, Randazzo, Caracappa, Vicari and Arculeo2021) and Tyrrhenian Sea (Santoro et al., Reference Santoro, Marchiori, Iaccarino, Uberti, Cassini, Di Nocera, Cerrone, Galiero and Marcer2019), as well as from North African coastline (Sey, Reference Sey1977), with prevalence varying from 18 to 33% (Supplementary Table 1; Fig. 3).
Worms of the genus Hysterothylacium have been mostly recorded at adult stage in the stomachs of large teleostean predators inhabiting the Mediterranean waters. Within the genus, Hysterothylacium incurvum (Rudolphi, 1819) Deardorff and Overstreet, 1980 and H. corrugatum (s.l.) Deardorff and Overstreet, 1981 are dominant and specialized nematodes of the swordfish, having this fish as their definitive host (Supplementary Table 1; Fig. 2B). Their occurrence in swordfish has been documented both in the Mediterranean (Mattiucci et al., Reference Mattiucci, Garcia, Cipriani, Santos, Nascetti and Cimmaruta2014, Reference Mattiucci, Cimmaruta, Cipriani, Abaunza, Bellisario and Nascetti2015a, Reference Mattiucci, Cipriani, Paoletti, Nardi, Santoro, Bellisario and Nascetti2015b) as well from other geographical areas included in the host range, such as the North-East and equatorial-tropical Atlantic waters (Garcia et al., Reference Garcia, Santos, Damiano, Nascetti and Mattiucci2008, Reference Garcia, Mattiucci, Damiano, Santos and Nascetti2010). However, no data are currently available for the larval occurrence of these species in intermediate/paratenic hosts. The presence of numerous squid beaks in swordfish stomachs examined during parasitological surveys leads to the hypothesis that the L3 might occur in deep-sea squid species that have not yet been investigated (Garcia et al., Reference Garcia, Santos, Damiano, Nascetti and Mattiucci2008; Mattiucci et al., Reference Mattiucci, Cimmaruta, Cipriani, Abaunza, Bellisario and Nascetti2015a).
Regarding the occurrence of other Hysterothylacium species, there is only limited evidence of adults in other large teleostean species (Table 1) (Ortis and Paggi, Reference Ortis, Paggi and Rellini2008). However, combined parasitological surveys and molecular analyses have identified L3 of H. aduncum (s.l.) in pelagic and demersal fish from Mediterranean waters (Supplementary Table 1). Hysterothylacium aduncum is currently the most common species, found at larval stage in more than 40 fish hosts (Petter and Radujkovic, Reference Petter and Radujkovic1989) (Supplementary Table 1). Larval stages of Hysterothylacium spp. have also been detected in various cephalopods, particularly oegopsid squids (Tedesco et al., Reference Tedesco, Bevilacqua, Fiorito and Terlizzi2020a, and references therein). Despite the abundance of larval stage reports, data on the infection by H. aduncum at the adult stage from the Mediterranean Sea are missing (Supplementary Table 1). Whereas this parasite species has been constantly recorded at adult stage in gadoid fish from NE Atlantic waters (see Levsen et al., Reference Levsen, Cipriani, Palomba, Giulietti, Storesund and Bao2022 and references therein). Consequently, despite its large occurrence at larval stage in pelagic and demersal Mediterranean food webs, H. aduncum was not chosen as possible sentinel parasite in this review, for the lack of consistent data on its definitive hosts.
Table 1. Chosen TTHs as sentinels of food-webs stability, environmental pollution and sea temperature change in the Mediterranean Sea

Metrics: measurable characteristics of sentinel parasites as tools for ecosystem monitoring. A good ( + +), moderate ( + ) or low ( + /–) potential level of contribution of the parasite species to a specific change, according to the currently assessed data. nd: not enough data.
Chosen TTHs among cestodes: biodiversity and ecology
Current knowledge on the species diversity of cestodes maturing in mid-upper trophic webs in the Mediterranean environment primarily concerns those from elasmobranchs. However, it is characterized by a mix of old and limited historical records. A parasite-host list is reported in the supplementary Table 2. Most of the observations report the parasite occurrence in intermediate/paratenic teleostean fish. Recent investigations, employing an integrative taxonomy approach, are consistently revealing a wide distribution and diversity of these cestodes in the Mediterranean. In particular, the trypanorhynch tapeworms stand out as the most extensively reported. Other groups such as Tetraphyllidea, Oncoproteocephalidea, Rhinebothriidea and Phyllobothriidea have historical records, but have received relatively limited recent attention (Supplementary Table 2). Indeed, according to historical data, the Mediterranean Sea seems to represent a hotspot of diversity for trypanorhynchs, harbouring around the 14% of the currently recognized species worldwide. The most represented families (by number of species) belong to Lacistorhynchidae (14 species), Eutetrarhynchidae (12 species), Tentaculariidae (5 species), Gymnorhynchidae (4 species) and Sphyriocephalidae (4 species) (Supplementary Table 2). These families account for about 85% of all trypanorhynch species found to date in the Mediterranean.
In general, trypanorhynchs are characterized by a complex life cycle (Fig. 2C and D), involving various marine organisms across different marine trophic levels. It has been suggested that different species have evolved different life-cycle strategies, occupying different ecological niches, also in terms of vertical distribution (Palm, Reference Palm2004). It is noteworthy that many of them exhibit a strong preference for a particular definitive host (Palm and Caira, Reference Palm and Caira2008), following the most common feeding ecology and depth range of this host. In general, their life cycle stages typically consist of a procercoid, followed by a plerocercus, or in some species, a plerocercoid or merocercoid, and ultimately the adult stage. Two hypothetical life cycle patterns have been proposed (Palm, Reference Palm2004). In the first scenario, the life cycle encompasses 3 hosts, with copepods acting as the first host and invertebrates as the second intermediate host, as observed in the Eutetrarhynchidae (Palm, Reference Palm2004). Alternatively, copepods can be the first host, and pelagic euphausiids can be the second intermediate host, as observed in Gymnorhynchidae. In the second pattern, the life cycle might involve 3 hosts, with copepods as first hosts and fish as obligatory second intermediate hosts, as is the case for many of the Lacistorhynchidae. A life cycle fully elucidated in nature is that of Grillotia erinaceus (van Beneden, 1858) Guiart, 1927 (Lacistorhynchidae). Operculate eggs released in sea water shed free-swimming coracidia in 5 days. The copepods Acartia longiremis or Pseudocalanus elongates serve as first intermediate hosts. The plerocercus develops in fish (especially in Gadiformes) while Raja spp. serve as definitive hosts (Palm, Reference Palm2004). The life cycles of Lacistorhynchidae can also extend to 4 hosts, where copepods are the first host, followed by schooling fish and large predatory fish as the second and third intermediate hosts, respectively. For example, among the Lacistorhynchidae, Callitetrarhynchus gracilis (Rudolphi, 1819) Pintner, 1931 exhibits a 4-host trophic chain life cycle. The plerocercus with small form generally occurs in smaller fish species, as clupeids, while the large form is present in larger fish, as scombrids, paralichthyids and balistids (Palm, Reference Palm2004).
This review has focused on 4 trypanorhynch species. They were chosen due to the new insights gained about their occurrence in intermediate/paratenic hosts, and on occasion, in definitive hosts of marine organisms inhabiting the Mediterranean Sea. The chosen species are: Molicola uncinatus (Linton, 1924) Palm, Reference Palm2004 and G. gigas, belonging to the family Gymnorhynchidae; Grillotia adenoplusia (Pintner, 1903) Palm, Reference Palm2004, and C. gracilis, which are representatives of the family Lacistorhynchidae.
Molicola uncinatus has been documented across various global regions. Notably, the larval stages have been found in scombriformid fish from diverse locations, including Cape Campbell (Cook Strait, New Zealand), the South African coast and Mediterranean Sea (see Palm, Reference Palm2004; Palomba et al., Reference Palomba, Santoro, Aco Alburqueque, Cipriani and Mattiucci2021b; Santoro et al., Reference Santoro, Palomba, Aco Alburqueque and Mattiucci2022b). However, as a sporadic finding, it was also reported in Tetraodontiformes and Carangiformes (Palm, Reference Palm2004). More specifically, in the Mediterranean Sea, this species has been genetically identified along the coast of Malta, in the silver scabbardfish Lepidopus caudatus (Palomba et al., Reference Palomba, Santoro, Aco Alburqueque, Cipriani and Mattiucci2021b) and in the Atlantic pomfret Brama brama from the Tyrrhenian and Ionian Seas (Santoro et al., Reference Santoro, Palomba, Aco Alburqueque and Mattiucci2022b). The high parasitic burden (A ≈ 28.8) of M. uncinatus within the silver scabbardfish strongly indicates the successful functioning of the parasite's life cycle in the Mediterranean (Fig. 3). The definitive host for the species is the common thresher Alopias vulpinus (Palm and Caira, Reference Palm and Caira2008). Even if M. uncinatus seems to show a strong species-specific association with this definitive host, however, no infection data about this cestode are so far available from stranded common thresher in the Mediterranean Sea. The population of the common thresher, found between surface waters and depths of 360 m in the Mediterranean Sea, is currently classified as critically endangered (IUCN). Also in the Mediterranean Sea, another significantly prevalent species is G. gigas. Here, it has been observed, at adult stage, in members of the Lamnidae, i.e. the great white shark Carcharodon carcharias, the porbeagle shark Lamna nasus and also the angular roughshark Oxynotus centrina of the Oxynotidae.
Larval G. gigas have been observed in the Atlantic pomfret, with high levels of infection from both Tyrrhenian and Ionian Seas, and the silver scabbardfish captured in the Central Mediterranean Sea (Fig. 3) (Manfredi et al., Reference Manfredi, Gandini and Traldi1993; Giarratana et al., Reference Giarratana, Muscolino, Beninati, Ziino, Giuffrida, Trapani and Panebianco2014; Palomba et al., Reference Palomba, Santoro, Aco Alburqueque, Cipriani and Mattiucci2021b; Santoro et al., Reference Santoro, Palomba, Aco Alburqueque and Mattiucci2022b).
The larvae of M. uncinatus and G. gigas may coexist in sympatry and syntopy. However, identifying Gymnorhynchidae larvae based solely on morphological criteria can be a challenging or sometimes impossibile, explecially when their tentacles are partially or completly invaginated, hindering the examination of crucial taxonomic features. In such cases, the use of molecular analysis becomes essential for precise species identification. In the Mediterranean basin, another species recently reported is Gymnorhynchus isuri Robinson, 1959 found in the sunfish Mola mola (Santoro et al., Reference Santoro, Palomba, Mattiucci, Osca and Crocetta2020b). Originally documented in the north, southwestern and northeast Atlantic, as well as the Tasman Sea in the southwestern Pacific, G. isuri is known to infect the shortfin mako Isurus oxyrinchus and the blue shark Prionace glauca (Palm et al., Reference Palm, Waeschenbach, Olson and Littlewood2009; Penadés-Suay et al., Reference Penadés-Suay, Jarque-Rico, Tomás and Aznar2022). The occurrence of this species in Mediterranean waters is probably connected to the migratory pathways of sunfish (Santoro et al., Reference Santoro, Palomba, Mattiucci, Osca and Crocetta2020b).
Seven species of the genus Grillotia belonging to the family Lacistorynchidae have been mainly recorded in the Mediterranean basin (Beveridge and Campbell, Reference Beveridge and Campbell2013; Dallarés, Reference Dallarés2016) (Supplementary Table 2). Among these, G. heptanchi (Vaullegeard, 1899) Dollfus, 1942 and G. adenoplusia have been observed as adults in sharks, while G. erinaceus has been found as adults in skates and rays (Beveridge and Campbell, Reference Beveridge and Campbell2013). Until a decade ago, only limited literature was available on Grillotia infections in the Mediterranean, with only G. erinaceus having undergone molecular identification. However, because of its sporadic and old records in Mediterranean region, G. erinaceus has not been considered in this review. Recent advancements in molecular systematics have expanded knowledge of Grillotia species in the Mediterranean Sea. Plerocerci of Grillotia were detected in the angler fish Lophius piscatorius (Santoro et al., Reference Santoro, Uberti, Corrado, Cutarelli, Iaccarino, Di Nocera, D'Amore, De Luca, Cerrone, Capuano and Galiero2018b) and in deep-dwelling sharks (Dallarés, Reference Dallarés2016; Santoro et al., Reference Santoro, Crocetta and Palomba2021b). Morphological and molecular studies, recently performed on these plerocerci, recognized them as belonging to the species G. adenoplusia (Isbert et al., Reference Isbert, Dallarés, Grau, Petrou, García-Ruiz, Guijarro, Jung and Catanese2023). Specifically, plerocerci of this cestode have been documented in the blackmouth catshark Galeus melastomus, the Portuguese dogfish Centroscymnus coelolepis and the velvet belly lanternshark Etmopterus spinax from the Western Mediterranean coast of Spain (Dallarés, Reference Dallarés2016; Dallarés et al., Reference Dallarés, Padrós, Cartes, Solé and Carrassón2017a, Reference Dallarés, Pérez-del-Olmo, Montero and Carrassón2017b; Isbert et al., Reference Isbert, Dallarés, Grau, Petrou, García-Ruiz, Guijarro, Jung and Catanese2023). No reports of Grillotia specimens were detected in the small-spotted catshark Scyliorhinus canicula from the same area. The prevalence of infection ranged from 17 to 100%, with a maximum abundance value of 84.6 recorded in the blackmouth catshark (Fig. 3).
Likewise, in the Tyrrhenian Sea, the velvet belly lanternshark, the blackmouth catshark and the small-spotted catshark have also been found to host these plerocerci (Santoro et al., Reference Santoro, Crocetta and Palomba2021b). In these cases, prevalence ranged from 82.0 to 100% with an abundance of 181.65 in the blackmouth catshark(Santoro et al., Reference Santoro, Bellisario, Crocetta, Degli Uberti and Palomba2021a) (Fig. 3). The presence of this TTH has been associated with the consumption of myctophid fish, which are known to prey on copepods, suggesting that these could potentially serve as intermediate hosts for G. adenoplusia (Dallarés, Reference Dallarés2016; Santoro et al., Reference Santoro, Bellisario, Tanduo, Crocetta and Palomba2022c). The bluntnose sixgill shark Hexanchus griseus was established as the definitive host for G. adenoplusia (Beveridge and Campbell, Reference Beveridge and Campbell2013).
In this review, C. gracilis, a member of the Lacistorhynchidae family, has also been considered. According to Palm (Reference Palm2004), C. gracilis has a trophically linked 4-host life cycle. In the Eastern Mediterranean Sea, larger fish species such as the bullet tuna Auxis rochei, the little tunny Euthynnus alletteratus, greater amberjack Seriola dumerili and the bluefin tuna act as hosts for the plerocerci of C. gracilis (Abdelsalam et al., Reference Abdelsalam, Abdel-Gaber, Mahmoud, Mahdy, Khafaga and Warda2016; Gabel et al., Reference Gabel, Unger, Theisen, Palm, Bat-Sheva Rothman, Yitzhak, Morov and Stern2022; Morsy et al., Reference Morsy, Dajem, Al-Kahtani, El-kott, Ibrahim, Hamdi, Al-Doaiss, Abumandour, El-Mekkawy, Massoud, Adel and El-Kareem2022). High infection levels by this parasite have been reported in these hosts. For instance, the species infects the little tunny off the coast of Alexandria with a prevalence of 38.7% (Abdelsalam et al., Reference Abdelsalam, Abdel-Gaber, Mahmoud, Mahdy, Khafaga and Warda2016). Notably, the parasite has also been identified in the little tunny populations in Turkey, where the prevalence soars to 91.3% (Akmirza, Reference Akmirza2006) (Fig. 3). Adults of C. gracilis were found in carcharhinid sharks (Carcharhinus spp.), although these have yet to be identified in the Mediterranean Sea.
The Tetraphyllidea, from which 3 new orders have recently emerged, namely Rhinebothriidea, Onchoproteocephalidea and Phyllobothriidea (Caira and Jensen, Reference Caira and Jensen2014), has traditionally been considered as one of the most speciose orders, worldwide. To date, reports of those cestodes infecting Mediterranean elasmobranchs are mostly based on morphological data, with few exceptions (Supplementary Table 2). The most recently studied species in the Mediterranean Sea are the tetraphyllidean Calliobothrium verticillatum (Rudolphi, 1819) (Fig. 2E) and the onchoproteocephalid Acanthobothrium coronatum (Rudolphi, 1819) Blanchard, 1848 (Fig. 2F) (Tedesco et al., Reference Tedesco, Gustinelli, Caffara, Marsella, Toffan and Fioravanti2020b, Reference Tedesco, Caffara, Gustinelli, Fiorito and Fioravanti2020c; Santoro et al., Reference Santoro, Crocetta and Palomba2021b).
Originally described from an unspecified shark species of Italy, C. verticillatum, the most representative species of its genus, has since been documented in the common smooth-hound Mustelus mustelus from the Northeastern Atlantic. Later, Euzet (Reference Euzet1959) reported it infecting the Mediterranean common smooth-hound. Tedesco et al. (Reference Tedesco, Gustinelli, Caffara, Marsella, Toffan and Fioravanti2020b) recently confirmed the occurrence of this host–parasite relationship in Mediterranean waters, specifically in the Adriatic Sea. Given these reports, the species demonstrates a high level of specificity with respect to its definitive host species. Indeed, C. verticillatum matures in the spiral valve of common smooth-hound. In this host, it produces hexacanth embryos that are released from gravid proglottids. The next stage involves the decapod grey hermit crab Pagurus pollicaris as first intermediate host, which ingests hexacanths. Inside the body of the hermit crab, the embryo undergoes development, first into a procercoid and then into a plerocercoid larva (see Tedesco et al., Reference Tedesco, Gustinelli, Caffara, Marsella, Toffan and Fioravanti2020b) (Fig. 2E). Currently, no information is available on intermediate hosts for this cestode species in the Mediterranean Sea.
Knowledge regarding species of the genus Acanthobotrium in the Mediterranean are generally outdated, with few exceptions, such as in the case of A. coronatum. Previously known for its non-specific associations with elasmobranch hosts, A. coronatum has recently been discovered to exclusively thrive in the nursehound Scyliorhinus stellaris from the Tyrrhenian Sea (Santoro et al., Reference Santoro, Bellisario, Fernández-Álvarez, Crocetta and Palomba2022d). This knowledge has been recently acquired from a parasitological investigation which thoroughly explored a diverse array of potential host species (Santoro et al., Reference Santoro, Bellisario, Fernández-Álvarez, Crocetta and Palomba2022d). Generally, members of the onchoproteocephalidean family, to which A. coronatum belongs, present a 3–5 host life cycle (Fig. 2F). This encompasses copepods as first, molluscs as second intermediate hosts, while fish act as intermediate or paratenic hosts (Jensen and Bullard, Reference Jensen and Bullard2010). A comparative molecular analysis, including sequences of A. coronatum from the nursehound and sequences of an unidentified Acanthobothrium collected from the common octopus Octopus vulgaris, has identified this cephalopod as a suitable intermediate host of A. coronatum (Tedesco et al., Reference Tedesco, Caffara, Gustinelli, Fiorito and Fioravanti2020c; Santoro et al., Reference Santoro, Bellisario, Fernández-Álvarez, Crocetta and Palomba2022d). In support of this, the common octopus has been confirmed as primary prey item of the nursehound (Santoro et al., Reference Santoro, Bellisario, Fernández-Álvarez, Crocetta and Palomba2022d).
TTHs as ‘sentinels’ of anthropogenic impacts in marine ecosystems
Marine helminth parasites with complex life cycles can be directly or indirectly affected by abiotic (i.e. sea water temperature, salinity, pollution) and biotic (i.e. host-species demography) factors. The autoecology of TTHs is the result of a long adaptation to their environment and their hosts, often including both homeothermic and heterothermic organisms. Thus, environmental changes, such as those caused by anthropogenic impacts, could significantly alter the dynamics of these organisms deeply embedded in the marine ecosystem. For this reason, TTHs interactions with the environment and their intermediate/definitive hosts constitute delicate biodiversity-functioning relationships. These relationships can be easily disrupted, either directly due to environmental changes or indirectly as a result of alterations in host dynamics (Marcogliese, Reference Marcogliese2005; Hudson et al., Reference Hudson, Dobson and Lafferty2006; Mattiucci and Nascetti, Reference Mattiucci and Nascetti2008; Palm, Reference Palm and Mehlhorn2011; Byers, Reference Byers2021; Selbach et al., Reference Selbach, Mouritsen, Poulin, Sures and Smit2022) (Fig. 2). Stressors affecting host population dynamics have the potential to compromise the transmission pathways of parasites, leading to changes in parasite distribution and number, even on a regional scale.
The possible contributions of TTHs as ‘sentinels’ of anthropogenic changes are proposed in Table 1. Measurable attributes to be used as metrics in future monitoring, at both spatial and temporal scale level, include those concerning their distribution, infection levels, relative proportions and genetic diversity. Some examples of the metrics evaluated in chosen TTHs from the Mediterranean Sea are here presented.
Infection levels of TTHs as indicators of food-web stability
Successful completion of TTHs life cycles requires suitable marine environmental conditions and stable food chains in which parasites are dependent on their hosts, to survive, develop and reproduce. Thus, the main factor shaping the distribution and infection levels of a parasite species in a local marine ecosystem is the availability of appropriate definitive and intermediate hosts, which in turn, maintains a high probability of multi-host parasite transmission (Cirtwill et al., Reference Cirtwill, Stouffer, Poulin and Lagrue2016). Among the drivers responsible for infection dynamics of chosen TTHs in the Mediterranean Sea, the distribution and the demography of both their definitive (i.e. cetaceans, sea turtles, elasmobranchs) and intermediate/paratenic (i.e. crustaceans, fish and molluscs) hosts play a determinant role. These factors contribute, in turn, to maintain the effective population size of a TTH species at regional level.
The infection levels of A. pegreffii in its paratenic fish host species (i.e. the European hake) vary significantly across fishing grounds in the western and eastern Mediterranean Sea. Indeed, in recent years, the observed infection rates with the parasite species in fish sampled from the Central and Southern Adriatic Sea were notably high (P≈95%; A≈128) than those recorded in the same fish host species sampled from Tyrrhenian Sea (Cipriani et al., Reference Cipriani, Sbaraglia, Paoletti, Giulietti, Bellisario, Palomba, Bušelić, Mladineo, Nascetti and Mattiucci2018a). Historical data indicate that the European hake, collected from the same area (i.e. Adriatic Sea) and same sized fish, did not show high infection rates by A. pegreffii (P≈73.3; A≈6.1) (Mattiucci et al., Reference Mattiucci, Abaunza, Ramadori and Nascetti2004). It can be hypothetically considered that environmental changes could have impacted parasite infection levels in this region. Oceanographic currents, along with nutrient-rich upwelling, could support a high population of planktonic organisms, the first hosts in the life cycle of the parasite. This abundance of hosts also benefits small pelagic fish such as anchovies and sardines, which serve as paratenic hosts for the parasite in the Adriatic. Moreover, notable populations of local bottlenose dolphins, the definitive host of the parasite species, have been observed, with these dolphins heavily preying on anchovy and hake (Holcer, Reference Holcer2012). Substantial A. pegreffii infections have indeed been detected in dolphins stranded along the Croatian coast (Blažeković et al., Reference Blažeković, Lepen Pleić, Đuras, Gomerčić and Mladineo2015). Consequently, these are optimal conditions for completion of the A. pegreffii life cycle, sustaining the elevated infection levels so far found in commercially important fish species (Cipriani et al., Reference Cipriani, Sbaraglia, Paoletti, Giulietti, Bellisario, Palomba, Bušelić, Mladineo, Nascetti and Mattiucci2018a, Reference Cipriani, Sbaraglia, Palomba, Giulietti, Bellisario, Bušelić, Mladineo, Cheleschi, Nascetti and Mattiucci2018b). Nonetheless, these abiotic and biotic factors and anthropogenic influence appear to be also responsible for the increased parasite infection in this Mediterranean Sea fishing ground. Indeed, an ‘anthropogenic shortcut’ in the life cycle of the parasite could occur as a consequence of the widespread practice of eviscerating fish directly onboard vessels and discarding their viscera at sea. This practice, aimed at removing viscera from heavily Anisakis larvae-infected fish, whose presence could compromise the value of the fish at landing, might lead to the inadvertent direct introduction of A. pegreffii larvae into the sea. Despite current Italian regulations suggesting proper on-land disposal, the discarded fish entrails carrying live A. pegreffii larvae serve as potential food for other fish and cetaceans, thereby facilitating the parasite's life cycle. As a result, dolphins following trawlers and consuming these discarded entrails and larvae, can accumulate more A. pegreffii. This contributes to a persistent high parasite burden of A. pegreffii in the Adriatic Sea's coastal fisheries, such as those so far reported (Mladineo and Poljak, Reference Mladineo and Poljak2014; Cipriani et al., Reference Cipriani, Sbaraglia, Paoletti, Giulietti, Bellisario, Palomba, Bušelić, Mladineo, Nascetti and Mattiucci2018a, Reference Cipriani, Sbaraglia, Palomba, Giulietti, Bellisario, Bušelić, Mladineo, Cheleschi, Nascetti and Mattiucci2018b).
In contrast, A. pegreffii has shown in the European hake, so far, lower values of prevalence and abundance in the same fish species from other Mediterranean Sea regions, for instance: from Ligurian Sea P ≈ 27.6, A ≈ 0.54; Central Tyrrhenian Sea P ≈ 44.2, A ≈ 1.39; Alboran Sea P ≈ 26.2, A ≈ 0.73. Interestingly, a temporal analysis from 1998 to 2019 of A. pegreffii infections in the European hake from the Atlantic Spanish coast (an area comprised into the parasite's geographical range) revealed an increasing trend in both prevalence and abundance of the infection (Diez et al., Reference Diez, Chust, Andonegi, Santurtún, Abaroa, Bilbao, Maceira and Mendibil2022). The authors correlated this trend with a corresponding rise in stock biomass of fish species, such as the European hake, the European anchovy Engraulis encrasicolus and the Atlantic mackerel Scomber scombrus, which also serve as suitable intermediate/paratenic hosts for A. pegreffii (Diez et al., Reference Diez, Chust, Andonegi, Santurtún, Abaroa, Bilbao, Maceira and Mendibil2022). Therefore, the higher fish host demography in Spanish Atlantic waters (Diez et al., Reference Diez, Chust, Andonegi, Santurtún, Abaroa, Bilbao, Maceira and Mendibil2022) contributes to a larger parasite population size and higher levels of infection. Furthermore, the same parasite species, in its populations in Austral regions (i.e. Argentine and New Zealand waters) exhibits higher levels of prevalence and abundance (Hossen et al., Reference Hossen, Wassens and Shamsi2021; Irigoitia et al., Reference Irigoitia, Palomba, Braicovich, Lanfranchi, Denuncio, Gana, Mattiucci and Timi2021). This suggests that the transmission route of the parasite life cycle in these extra-Mediterranean waters, included in the range of distribution of the parasite species (see Mattiucci et al., Reference Mattiucci, Cipriani, Levsen, Paoletti and Nascetti2018b), is maintained at a higher intensity.
This would be related to the substantial demography of its suitable definitive (Hammond et al., Reference Hammond, Macleod, Berggren, Borchers, Burt, Cañadas, Desportes, Donovan, Gilles, Gillespie, Gordon, Hiby, Kuklik, Leaper, Lehnert, Leopold, Lovell, Nils Øienm, Paxton, Ridoux, Rogan, Samarra, Scheidat, Sequeira, Siebert, Skov, Swift, Taske, Teilmann, Van Canneyt and Vázquez2013; Magera et al., Reference Magera, Mills Flemming, Kaschner, Christensen and Lotze2013) and intermediate/paratenic hosts in those waters, probably characterized by more stable ecosystem dynamics. Conversely, we could hypothesize that the Mediterranean pelagic and mesopelagic trophic webs, exploited by A. pegreffii, may be less stable and therefore unable to maintain a population size comparable to those in other regions. In support of this observation, we should consider the population status of the most common definitive hosts of A. pegreffii in the Mediterranean, i.e. dolphins. Despite recent conservation efforts aimed at monitoring and protecting marine mammals in Mediterranean waters (for instance, the Santuario dei Cetacei, ‘Pelagos’ in the basin area between the Ligurian and Tyrrhenian Sea), the population size of certain dolphin species is lower than in other oceanographic areas (IUCN, 2020), thus supporting smaller parasite population abundance values. Indeed, unfortunately, Mediterranean marine mammals and sea turtles are currently affected by various anthropogenic disturbances, such as fisheries by-catch and plastic pollution (IUCN, 2020). For instance, sperm whales stranded in 2009 along the Italian Ionian Sea were found uninfected by anisakid worms, and were instead loaded with a large quantity of plastic waste (Mattiucci and Marcer, personal observation). In addition, sperm whale mortalities have been reported as caused by plastic ingestion (De Stephanis et al., Reference De Stephanis, Giménez, Carpinelli, Gutierrez-Exposito and Cañadas2013). Meanwhile, the high infection rate with A. physeteris found in both Mediterranean sperm whales (Mazzariol et al., Reference Mazzariol, Centelleghe, Cozzi, Povinelli, Marcer, Ferri, Di Francesco, Badagliacca, Profeta, Olivieri, Guccione, Cocumelli, Terracciano, Troiano, Beverelli, Garibaldi, Podestà, Marsili, Fossi, Mattiucci, Cipriani, De Nurra, Zaccaroni, Rubini, Berto, de Quiros, Fernandez, Morell, Giorda, Pautasso, Modesto, Casalone and Di Guardo2018) and dwarf sperm whales (Santoro et al., Reference Santoro, Di Nocera, Iaccarino, Cipriani, Guadano Procesi, Maffucci, Hochscheid, Blanco, Cerrone, Galiero, Nascetti and Mattiucci2018a) seems to indicate a well-established life cycle of the parasite in the Mediterranean Sea. Thus, the occurrence of A. physeteris could be used as an ecological indicator to monitor the stability of trophic webs. These would encompass the aforementioned physeteriids and deep-sea squid species such as histiotheuthids. These squids are not directly impacted by overfishing activities, and have a significant parasite burden (on average, A ≈ 18) (Palomba et al., Reference Palomba, Mattiucci, Crocetta, Osca and Santoro2021a) (Fig. 3).
Infections by the anisakid S. sulcata in the Mediterranean Sea are strongly linked to the abundance of its definitive host, the loggerhead sea turtle and the availability of its prey. Indeed, juveniles and adults of this sea turtle species are present throughout the entire basin, concentrating their foraging activity along the North Adriatic Sea and Central Mediterranean shelf; lower frequencies are reported from the Southern Ionian and Sicilian areas (Casale et al., Reference Casale, Hochscheid, Kaska and Panagopoulou2020). Host migratory activity throughout the Mediterranean plays a significant role in shaping the transmission and infection levels observed with S. sulcata in the North Adriatic and Tyrrhenian Seas (Santoro et al., Reference Santoro, Marchiori, Iaccarino, Uberti, Cassini, Di Nocera, Cerrone, Galiero and Marcer2019; Marcer et al., Reference Marcer, Tosi, Franzo, Vetri, Ravagnan, Santoro and Marchiori2020). In addition, the differential foraging habits of juvenile and adult loggerhead sea turtles, as well as their distinct habitats in these basins, are responsible for defining the distribution of S. sulcata. The prevalence of S. sulcata was found to be significantly higher in loggerhead sea turtles from the Adriatic Sea with respect to the Tyrrhenian Sea. However, while in the Adriatic Sea both early and large juveniles of the loggerhead sea turtles were found infected, in the Tyrrhenian Sea only larger individuals were parasitized. These differences seem to be the result of regional environmental habitat characteristics, and prey availability for the loggerhead sea turtle in these basins (Santoro et al., Reference Santoro, Marchiori, Iaccarino, Uberti, Cassini, Di Nocera, Cerrone, Galiero and Marcer2019). Both juveniles and adult loggerhead sea turtles are known to feed on the benthic organisms in the Northern Adriatic Sea, here including bivalves and gastropods. Adults forage instead in shallower coastal waters of the Tyrrhenian Sea on benthic molluscs, such as the Mediterranean mussel (Casale et al., Reference Casale, Abbate, Freggi, Conte, Oliverio and Argano2008) which are intermediate hosts of S. sulcata (Santoro et al., Reference Santoro, Marchiori, Iaccarino, Uberti, Cassini, Di Nocera, Cerrone, Galiero and Marcer2019; Marcer et al., Reference Marcer, Tosi, Franzo, Vetri, Ravagnan, Santoro and Marchiori2020). Thus, in the Adriatic Sea, the benthic trophic web seems to support a higher probability for juveniles to become infected by S. sulcata. The increasing trend showing high parasitic load (A = 100) found in adult sea turtles also suggests that the parasite accumulates in its definitive hosts (Santoro et al., Reference Santoro, Marchiori, Iaccarino, Uberti, Cassini, Di Nocera, Cerrone, Galiero and Marcer2019). Thus, the abundance of the loggerhead sea turtle recently recorded in the Mediterranean (Di Matteo et al., Reference Di Matteo, Cañadas, Roberts, Sparks, Panigada, Boisseau, Moscrop, Fortuna, Lauriano, Holcer, Peltier, Ridoux, Raga, Tomás, Broderick, Godley, Haywood, March, Snape, Sagarminaga and Hochscheid2022) seems to play a role in sustaining the population size of its specific anisakid endoparasite.
Significant differences in the infection levels with the adult raphidascarid nematodes H. incurvum and H. corrugatum (s.l.), specific endoparasites of the swordfish, were observed between the Mediterranean Sea and Atlantic waters (Mattiucci et al., Reference Mattiucci, Garcia, Cipriani, Santos, Nascetti and Cimmaruta2014). These differences suggest the existence of distinct stocks of the swordfish in these waters, as also indicated by the population genetics of the fish host stocks (see Mattiucci et al., Reference Mattiucci, Cimmaruta, Cipriani, Abaunza, Bellisario and Nascetti2015a, Reference Mattiucci, Cipriani, Paoletti, Nardi, Santoro, Bellisario and Nascetti2015b). High infection rates, in terms of both prevalence and mean intensity, were recorded for Hysterothylacium spp. in swordfish from the Atlantic tropical-equatorial waters. Indeed, from this area, H. incurvum showed, on average, prevalence, P≈ 90% and mean intensity, Im ≈ 39.7; while the species H. corrugatum (s.l.) reached, on average, the value of P ≈ 87.3%, and Im ≈ 20. Similarly, in the Atlantic Iberian swordfish population, H. incurvum showed P = 100% and, on average Im ≈ 18.6, while H. corrugatum (s.l.) reached the values of P = 100% and on average Im ≈ 25.7 (Garcia et al., Reference Garcia, Mattiucci, Damiano, Santos and Nascetti2010). Conversely, lower levels of infection were observed, over the same temporal but at different spatial scale, in the Mediterranean stock: H. incurvum: P ≈ 14.3, on average, Im ≈ 5.3; H. corrugatum (s.l.): P ≈ 14%, on average Im ≈ 5.8 (Mattiucci et al., Reference Mattiucci, Garcia, Cipriani, Santos, Nascetti and Cimmaruta2014). These differences in the parasitic load of Hysterothylacium species in the swordfish populations could be attributed to variations in the foraging patterns of the swordfish in different areas. This variation is likely linked to the availability of food items, mostly deep-sea squid species, which serve as suitable intermediate hosts for these species of Hysterothylacium. The presence of squid beaks in the gastric contents of swordfish from tropical-equatorial waters heavily infected by these Hysterothylacium species further supports this hypothesis (Garcia et al., 2011) (Fig. 3). Thus, the composition and parasitic burden with Hysterothylacium spp. in swordfish stocks from different fishing grounds are likely the outcome of a combination of factors, including the population size of their swordfish definitive host as well as the availability and demography of its favourite prey items. In accordance, while the North Atlantic swordfish population benefited from management actions that have rebuilt its stock (Neilson et al., Reference Neilson, Arocha, Calay, Mejuto, Ortiz, Scott, Smith, Travassos, Tserpes and Andruschchenko2013), the Mediterranean swordfish stocks are still under management to avoid a possible stock collapse in the next generations (Tserpes et al., Reference Tserpes, Tzanatos and Peristeraki2011). Thus, within the framework of a holistic approach in fisheries management, the parallel monitoring of infection levels with these TTHs would provide an indication of habitat or stock deterioration of the Mediterranean swordfish.
Concerning cestodes, in the Mediterranean Sea, specifically within the Gulf of Naples, a notable prevalence and abundance (P ≈ 53.6; abundance, A ≈ 5.32) of the cestode A. coronatum has been recently documented (Santoro et al., Reference Santoro, Crocetta and Palomba2021b, Reference Santoro, Bellisario, Fernández-Álvarez, Crocetta and Palomba2022d). This parasite species has the nursehound shark as its suitable definitive host. This elasmobranch species is demersal, primarily dwelling along the continental shelves and slopes. The nursehound shark's diet predominantly comprises a variety of benthic organisms, with cephalopods often as a substantial dietary component (Santoro et al., Reference Santoro, Bellisario, Fernández-Álvarez, Crocetta and Palomba2022d). The nursehound shark's dietary preferences and its ecology are significant factors influencing the parasite's transmission route. Thus, the high prevalence of A. coronatum in the Central Tyrrhenian area, off the Gulf of Naples seems to indicate a stable population size of its definitive host in this geographical area. Supporting this, the Santa Croce Bank in the Gulf of Naples is recognized as the main known Mediterranean breeding ground for the nursehound shark (de Sabata and Clò, Reference De Sabata and Clò2013). A similar trend of infection levels (P = 85%, A = 7.3) by C. verticillatum has been documented in the common smooth-hounds captured in the Tyrrhenian Sea (our unpublished data) (Fig. 3). This could suggest the presence of a stable coastal trophic web, with the species-specific common smooth-hound as the definitive host and the pagurus as intermediate one.
Intraspecific genetic variability of TTHs as an indirect indicator of food web stability
The demographic dynamics of host populations and the environmental abiotic factors also have a role in shaping the genetic structure and diversity of nematode and cestode populations (Mattiucci and Nascetti, Reference Mattiucci and Nascetti2008; Isbert et al., Reference Isbert, Dallarés, Grau, Petrou, García-Ruiz, Guijarro, Jung and Catanese2023).
For instance, it has been postulated that multi-host parasites with life cycles completed in both poikilothermic and homeothermic hosts (for instance, Anisakis spp.) exhibit higher levels of genetic polymorphism and heterozygosity compared with single-homeothermic host species (Bullini et al., Reference Bullini, Nascetti, Paggi, Orecchia, Mattiucci and Berland1986). Such intraspecific genetic variation is fundamental for the species adaptive capacity to changing environments (May, Reference May1994). In the case of multi-host parasites, it was indeed hypothesized that within the gene pool of a parasite species, a given allele may confer greater fitness in one stage of the life cycle, while another allele may function optimally in another group of hosts of its life cycle (Bullini et al., Reference Bullini, Nascetti, Paggi, Orecchia, Mattiucci and Berland1986). In addition, estimates of genetic variability can shed light on the effect of ecological traits and bottleneck events that may have occurred historically within the gene pools of multi-host helminths, potentially as a result of anthropogenic-driven environmental changes (Mattiucci and Nascetti, Reference Mattiucci and Nascetti2008). Indeed, assessment of genetic variability parameters – i.e. the estimates of genetic polymorphism, rare alleles and expected heterozygosity (He) – of a parasite species from an oceanographic area can assist with evaluation of the parasite population status, and provide an indicator of stable or changing environmental conditions. Any habitat perturbation directly disrupting the abiotic conditions for egg hatching, or, through a ‘domino effect’, any habitat perturbation that decreases host numbers could negatively impact local parasite populations. Consequently, this sequence of events increases the probability of genetic erosion within the parasite population gene pool resulting in the loss of general polymorphism due to genetic drift phenomena and loss of rare alleles (Mattiucci and Nascetti, Reference Mattiucci and Nascetti2008; Pauls et al., Reference Pauls, Nowak, Bálint and Pfenninger2013; Almeida-Rocha et al., Reference Almeida-Rocha, Soares, Andrade, Gaiotto and Cazetta2020). In agreement with this hypothesis, a significant positive correlation between the genetic variability values of a parasite population and its abundance (as a proxy of population size of the parasite species) of TTHs parasites and their hosts has been documented (Zarlenga et al., Reference Zarlenga, Hoberg, Rosenthal, Mattiucci and Nascetti2014). An attempt to positively correlate genetic variability values with the effective population size (Ne) of a parasite species as a conservation measure has been also recently speculated for species of nematomorphs (De Vivo et al., Reference De Vivo, Chen and Huang2023). A research on anisakid species from Antarctic waters recorded significantly high values of intraspecific genetic variability in Contracaecum osculatum sp. D and C. osculatum sp. E, with high infection rates observed in their paratenic (icefish) and definitive (the Weddell seal, Leptonychotes weddellii) hosts, surpassing those found in sibling species of the C. osculatum (s.l.) complex from the Arctic Seas (Mattiucci and Nascetti, Reference Mattiucci and Nascetti2008). In addition, a 3-decade temporal analysis found stable values in both infection levels and genetic variability in these Antarctic anisakids (Mattiucci et al., Reference Mattiucci, Cipriani, Paoletti, Nardi, Santoro, Bellisario and Nascetti2015b, Reference Mattiucci, Paoletti, Cipriani, Webb, Timi, Nascetti, Klimpel, Kuhn and Mehlhorn2017b). This trend of stability can be attributed to an undisturbed Antarctic marine habitat, fostering stable trophic webs with abundant hosts maintaining high anisakid population sizes, with less probability of genetic erosion events in their gene pools (Mattiucci et al., Reference Mattiucci, Cipriani, Paoletti, Nardi, Santoro, Bellisario and Nascetti2015b, Reference Mattiucci, Paoletti, Cipriani, Webb, Timi, Nascetti, Klimpel, Kuhn and Mehlhorn2017b; Palomba et al., Reference Palomba, Libro, Di Martino, Roca-Geronès, Castrignanò, Canestrelli and Mattiucci2023b).
In contrast, possible occurrence of genetic erosion was reported in a Mediterranean population of A. pegreffii after a 20-year (1986–2004) study. There was a loss of rare alleles at various polymorphic loci (i.e. allozymes) (Mattiucci and Nascetti, Reference Mattiucci and Nascetti2008). This was attributed by the authors to a likely demographic decline of the parasite, possibly due to habitat disturbance and host species population disruption, consequently altering the trophic web required by the parasite to complete its life cycle. A similar genetic analysis was conducted in the Austral (New Zealand waters) populations of the same parasite species, showing instead a stable genetic polymorphism (Mattiucci and Nascetti, Reference Mattiucci and Nascetti2008). More recent investigations of the Mediterranean populations of A. pegreffii, based on a substantial number (N = 11) of highly polymorphic DNA microsatellite (SSRs) loci, originally developed for the A. simplex (s.l.) complex (Mattiucci et al., Reference Mattiucci, Bello, Paoletti, Webb, Timi, Levsen, Cipriani and Nascetti2019; Bello et al., Reference Bello, Paoletti, Webb, Nascetti and Mattiucci2020), provided further support to the alarming decrease of genetic variability in this parasite. According to those SSR loci, preliminary estimates of the genetic variability at intraspecific level over a spatial scale level, in a large number of individuals (N ≈ 150), analysed for each Mediterranean and extra-Mediterranean sample of A. pegreffii, have indicated lower mean heterozygosity values (He) in the Mediterranean populations (on average, He ≈ 0.67), compared with the levels observed in parasite populations from an extra-Mediterranean area, such as New Zealand waters (on average, He ≈ 0.75). Similarly, intraspecific genetic diversity estimated in A. pegreffii at a polymorphic mitochondrial gene locus (i.e. mtDNA cox2) showed a much lower nucleotide diversity (π) and haplotype number (Nh) (π ≈ 0.0059; Nh = 52) in a population from the Adriatic Sea compared with that recorded in its extra-Mediterranean population from New Zealand (π ≈ 0.0102; Nh = 113) waters. These findings suggest that the Mediterranean populations of A. pegreffii are facing genetic erosion events, probably linked to an overall parasite demographic fluctuation. In contrast, a higher level of stability characterizes the trophic webs maintaining A. pegreffii life cycle in the Austral waters off New Zealand. This stability is likely maintained by a broader range and demography of suitable hosts in the Austral region, which in turn, permits the maintenance of higher A. pegreffii population size, as above mentioned, with lower probability of genetic drift phenomena in the population's gene pool. These observations once again suggest how vulnerable the Mediterranean ecosystem could be in the face of recent global changes and anthropogenic impacts, as suggested by the likelihood of genetic variability loss in parasite's gene pool.
The Mediterranean population of A. physeteris has also displayed a high level of genetic diversity estimated at mitochondrial gene level, with a Tyrrhenian sample showing a considerable number of haplotypes and nucleotide diversity values (Nh = 39; π ≈ 0.0086) (Cipriani et al., Reference Cipriani, Palomba, Giulietti, Marcer, Mazzariol, Santoro, Alburqueque, Covelo, López, Santos, Pierce, Brownlow, Davison, McGovern, Frantzis, Alexiadou, Højgaard, Mikkelsen, Paoletti, Nascetti, Levsen and Mattiucci2022b). This finding seems to be positively correlated with the high parasitic load so far observed in the Mediterranean populations of physeteriids (Santoro et al., Reference Santoro, Di Nocera, Iaccarino, Cipriani, Guadano Procesi, Maffucci, Hochscheid, Blanco, Cerrone, Galiero, Nascetti and Mattiucci2018a; Mazzariol et al., Reference Mazzariol, Centelleghe, Cozzi, Povinelli, Marcer, Ferri, Di Francesco, Badagliacca, Profeta, Olivieri, Guccione, Cocumelli, Terracciano, Troiano, Beverelli, Garibaldi, Podestà, Marsili, Fossi, Mattiucci, Cipriani, De Nurra, Zaccaroni, Rubini, Berto, de Quiros, Fernandez, Morell, Giorda, Pautasso, Modesto, Casalone and Di Guardo2018). Despite the fact that comparative spatial and/or temporal genetic data are not available for this parasite, and that nuclear genes should be investigated, the genetic variability values and parasitic burden observed seem to indicate a stable trophic web which sustains the life cycle of A. physeteris.
Genetic studies of Mediterranean S. sulcata at 3 gene loci (i.e. ITS region of rDNA, mtDNA cox1 and cox2) revealed an overall genetic similarity between parasite populations from Adriatic and Tyrrhenian waters, without geographical clustering of parasite from different basins (Marcer et al., Reference Marcer, Tosi, Franzo, Vetri, Ravagnan, Santoro and Marchiori2020). This could likely be the consequence of the long-span migrations recorded for the loggerhead sea turtles throughout the Mediterranean Sea (Marcer et al., Reference Marcer, Tosi, Franzo, Vetri, Ravagnan, Santoro and Marchiori2020). However, the existence of a certain number of haplotypes (Nh = 38) observed at the mtDNA cox1 gene locus in the Mediterranean population of S. sulcata was estimated (our unpublished data). This value would be positively related to the parasite population size maintained by both juveniles and adult loggerheads. Although a spatial and temporal comparison of the genetic diversity of S. sulcata from extra-Mediterranean waters cannot currently be performed, these findings represent the base for future genetic comparisons. Such a comparison that may be feasible with the possible existence of a sibling species of S. sulcata from the Eastern China Sea off Japan, showing a high level of genetic differentiation from Mediterranean isolates, was recently suggested (Sata and Nakano, Reference Sata and Nakano2023). Unfortunately, only 2 sequences were deposited from GenBank from Eastern China Sea, making it impossible to infer genetic variability values. Further genetic studies, potentially adopting a multigenotyping approach, will unravel the population genetic structure and genetic diversity of S. sulcata (s.l.) worldwide, and even clarify the possible existence of sibling species within the taxon. This information, combined with the definitive host's phylogeographical data, will be useful to monitor the host migratory routes, foraging areas and nesting sites in Mediterranean waters.
A recent molecular epidemiological study from different geographical areas of the Mediterranean Sea and Atlantic waters revealed a substantial genetic variability for G. adenoplusia. Specimens of G. adenoplusia were obtained from the benthic shark (E. spinax) in the eastern and western Mediterranean, as well as the Atlantic Ocean (Isbert et al., Reference Isbert, Dallarés, Grau, Petrou, García-Ruiz, Guijarro, Jung and Catanese2023). The study revealed a noteworthy intraspecific occurrence of numerous haplotypes (Nh = 17) with 18 polymorphic sites in the large subunit ribosomal DNA (28S rDNA) gene (Isbert et al., Reference Isbert, Dallarés, Grau, Petrou, García-Ruiz, Guijarro, Jung and Catanese2023). Interestingly, in the Mediterranean, the parasite showed higher abundance in intermediate hosts sampled from the Balearic and Cyprus populations, also corresponding with the highest record of polymorphic sites and haplotypes (the latter from the Balearic Sea) (Isbert et al., Reference Isbert, Dallarés, Grau, Petrou, García-Ruiz, Guijarro, Jung and Catanese2023). This observation supports the hypothesis that, even in the case of this heteroxenous cestode, genetic variability is positively correlated with the parasite population size at the local level. Indirectly, this could imply that in the Mediterranean Sea, the deep-sea food webs maintain a higher stability than pelagic realm. Concerning Grillotia spp., further genetic markers and additional sampling will be necessary to elucidate the genetic structure and intraspecific genetic variation of this taxa, to assess its host range and explore its utility as an indicator of deep-sea environment stability.
TTHs as sentinels of sea temperature rise
The biogeographical range and infection dynamics of host–parasite associations involving the chosen TTHs and their hosts are not only determined by biotic factors, but also profoundly influenced by abiotic environmental parameters, such as sea water temperature and salinity. These are strongly impacted by climate changes. The Mediterranean Sea has shown a consistent increase of sea surface temperature (SST) from 1979 to 2003 (overall average increase of 1.4°C), particularly in the eastern part of the basin (MedECC, 2020). Indeed, it is defined as a ‘hot spot’ for climate change (Pastor et al., Reference Pastor, Valiente and Palau2018). Temperature increase and water-level rise can provoke changes in water circulation and variations in salinity, representing key environmental factors for the marine organisms. These changes present limiting factors for the egg hatching and free-living larval stages of parasites, but also influence the abundance of first intermediate hosts and the migratory routes for feeding and/or reproduction of both intermediate/paratenic and definitive hosts. For instance, the hatching of eggs and the free-living larval stage of each anisakid requires an optimal thermal range (Diez et al., Reference Diez, Chust, Andonegi, Santurtún, Abaroa, Bilbao, Maceira and Mendibil2022; Gomes et al., Reference Gomes, Quiazon, Itoh, Fujise and Yoshinaga2023). An increase in sea water temperature as recorded for the Mediterranean Sea (MedECC, 2020) could potentially impact their survival and lead to a shift in parasite distribution to areas with more favourable abiotic conditions. These phenomena could lead to a change in the fitness of certain parasite species in a determined marine area and alter its distribution in different intermediate and definitive hosts.
This is, for instance, the case in the parasite Anisakis typica (s.l.) whose distribution has been monitored over the past 2 decades in the Mediterranean Sea. Historically, this parasite showed a relative proportion of 100% in the European hake populations in the far eastern warmer Mediterranean Sea, i.e. around Cyprus waters and a relative proportion of around the 14 and 2.3%, in co-infection with other Anisakis spp. in hakes fished off Crete and from the Ionian Sea, respectively (Mattiucci et al., Reference Mattiucci, Abaunza, Ramadori and Nascetti2004) (Fig. 4). In recent years, the occurrence of A. typica sp. A in this species from the eastern Mediterranean basin has increased, and it was also recorded at increasing proportions (around 25%) in Central Mediterranean (off the Malta coast) in co-infection with A. pegreffii and A. physeteris (Palomba and Mattiucci personal observation) (Fig. 4). In previous molecular epidemiological studies, this parasite was never reported from this basin (see Mattiucci et al., Reference Mattiucci, Giulietti, Paoletti, Cipriani, Gay, Levsen, Klapper, Karl, Bao, Pierce and Nascetti2018a, Reference Mattiucci, Cipriani, Levsen, Paoletti and Nascetti2018b) (Fig. 4). Water temperatures in the Levantine, Aegean and northwest Ionian Seas have experienced the most significant SST increase in recent years (MedECC, 2020). In addition, warming was also recorded in central Mediterranean waters. This prompts speculation that the temperature increase could have contributed to the western-bound range shift of this parasite during recent years. Such temperature trends could increase egg hatching probability, thereby increasing the probability of transmission to the next suitable hosts. Further epidemiological surveys carried out in these fishing grounds may confirm this trend. Westward expansion of TTHs such as A. typica sp. A from the Levantine Seas to the southern Ionian and Central Mediterranean (Sicilian coasts) could serve as a further indicator of a warming Mediterranean Sea. The finding of A. typica sp. A in the eastern part of the Mediterranean Sea could be attributed to the so-called ‘Lessepsian migration’ of marine organisms, including parasites, from the Indian Ocean to the eastern waters of Mediterranean Sea through the Suez Canal. In a warmer Mediterranean basin, this parasite species could encounter environmental conditions resembling their current habitat, permitting to establish its life cycle.
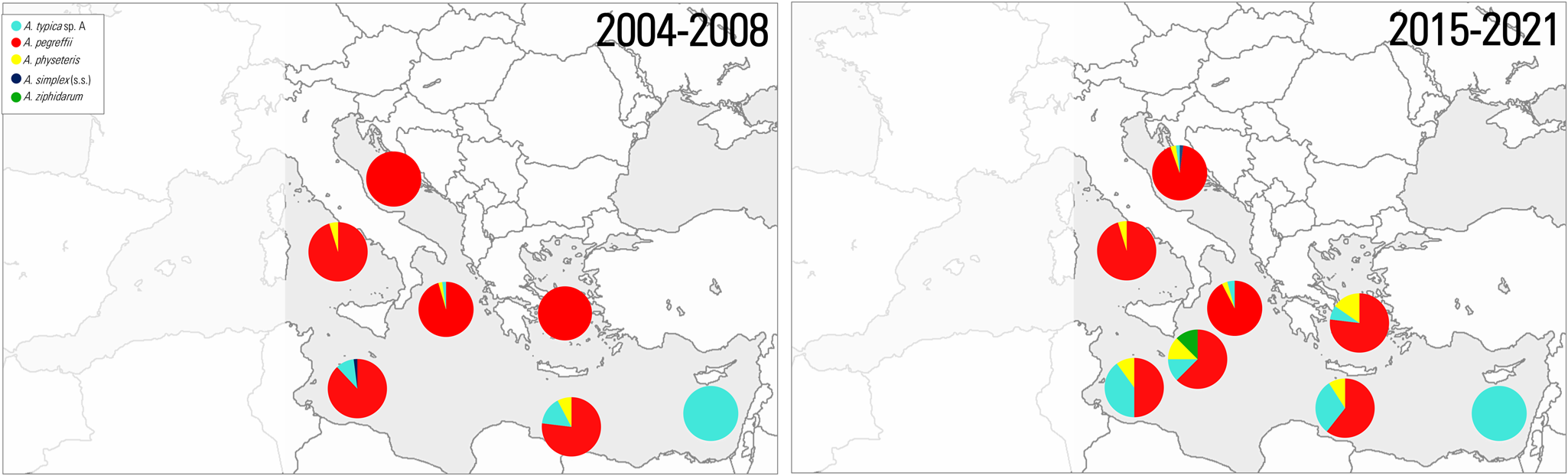
Figure 4. Relative proportions of Anisakis spp. from historical (2004–2008) and current (2015–2021) data observed in a fish species (i.e. European hake Merluccius merluccius) from different fishing grounds of the Mediterranean Sea (data from Mattiucci et al., Reference Mattiucci, Abaunza, Ramadori and Nascetti2004, Reference Mattiucci, Cipriani, Levsen, Paoletti and Nascetti2018b; Farjallah et al., Reference Farjallah, Slimane, Busi, Paggi, Amor, Blel, Said and D'Amelio2008; Mattiucci and Nascetti, Reference Mattiucci and Nascetti2008; Cipriani et al., Reference Cipriani, Smaldone, Acerra, D'Angelo, Anastasio, Bellisario, Palma, Nascetti and Mattiucci2015 and unpublished data).
Similarly, some recent findings indicate a potential northbound expansion of the geographical range of A. pegreffii into NE Atlantic waters. Previously limited to the northern part of the Atlantic Spanish coast, recent findings of its larval stages in the Atlantic cod, Gadus morhua and the Atlantic mackerel from the North Sea (Gay et al., Reference Gay, Bao, MacKenzie, Pascual, Buchmann, Bourgau, Couvreur, Mattiucci, Paoletti, Hastie, Levsen and Pierce2018; Levsen et al., Reference Levsen, Svanevik, Cipriani, Mattiucci, Gay, Hastie, Bušelić, Mladineo, Karl, Ostermeyer, Buchmann, Højgaard, González, Pascual and Pierce2018a, Reference Levsen, Cipriani, Mattiucci, Gay, Hastie, MacKenzie, Graham, Cecilie, Svanevik, Højgaard, Nascetti, González and Pascual2018b) suggest a northward expansion. The lower limit of sea temperature tolerance of A. pegreffii along the Iberian Atlantic waters seems to be around 15°C (Diez et al., Reference Diez, Chust, Andonegi, Santurtún, Abaroa, Bilbao, Maceira and Mendibil2022). Therefore, the ongoing rising sea temperature in NE Atlantic waters could permit the completion of A. pegreffii life cycle also in Northern regions. As a consequence, tracking the distribution of this parasite not only serves as an indicator of temperature shift in Atlantic waters but might also to predict the spread of this zoonotic parasite to other regions.
In the case of the loggerhead sea turtle, harbouring the anisakid S. sulcata, a westward movement of the host, likely due to SST increase, has been recently described (Mancino et al., Reference Mancino, Canestrelli and Maiorano2022). Thus, future data on the distribution and genetic analysis of S. sulcata can yield insights into turtle migration routes, foraging and nesting territories. Hence, monitoring the distribution and infection patterns of this chosen TTH would contribute to assessing the status of the loggerhead sea turtle and the trophic web within which S. sulcata completes its life cycle. Thus, this TTH could serve as a valuable indicator for monitoring this flagship host and the associated coastal and marine ecosystems, under climate change scenarios.
Temperature could also directly impact the eggs, coracidia and procercoids of trypanorhynch tapeworms (Sakanari and Moser, Reference Sakanari and Moser1985). A notable example was reported by MacKenzie (Reference MacKenzie1987). The author reported a dramatic decline in cestode populations, i.e. Grillotia smarisgora (Wagener, 1854) and Lacistorhynchus tenuis (Van Beneden, 1858) Pintner, 1913 in 2 fish hosts (Atlantic mackerel and the Atlantic herring Clupea harengus) in European waters over 8 years. The exact reasons behind these transmission failures remain puzzling; however, they are likely linked to shifts in abiotic environmental factors that could have affected the demography of the first intermediate host of the parasites. Intriguingly, the decline in cestode prevalence coincided with the conclusion of a North Atlantic hydrographic phenomenon known as the ‘1970s salinity anomaly’ (MacKenzie, Reference MacKenzie1987). Unfortunately, the Mediterranean Sea region lacks analogous reported instances in cestodes due to the scarcity of historical data for these parasites. Nonetheless, in recent years, a high rate of infection with trypanorhynch larvae such as species of genera Grillotia, Molicola and Gymnorhynchus in their intermediate hosts – not previously recorded – has been observed (e.g. Santoro et al., Reference Santoro, Uberti, Corrado, Cutarelli, Iaccarino, Di Nocera, D'Amore, De Luca, Cerrone, Capuano and Galiero2018b, Reference Santoro, Bellisario, Crocetta, Degli Uberti and Palomba2021a, Reference Santoro, Palomba, Aco Alburqueque and Mattiucci2022b; Palomba et al., Reference Palomba, Santoro, Aco Alburqueque, Cipriani and Mattiucci2021b) (Fig. 3) (Supplementary Table 2). It could be speculated that the changes observed over the last years in Mediterranean waters (increase in SST, salinity, switching currents) could have altered the conditions for the survival and spread of these parasites. These changes could have triggered egg hatching, increased the abundance of the first intermediate hosts or potentially influenced the migration routes, food availability and reproductive cycles of their definitive elasmobranch hosts. Thus, changes in the abiotic conditions of Mediterranean Sea may have facilitated life cycle completion in these cestodes.
Furthermore, shifts in the geographic distribution of definitive and intermediate hosts of certain helminth parasites, driven by global climate changes, could create new parapatric and sympatric areas between isolated but genetically related parasite species. This could lead to a potential increase in hybridization events between closely related species. An example of this phenomenon is observed in the sympatric area of the Alboran Sea, where the 2 sibling species, i.e. A. simplex (s.s.) and A. pegreffii, coexist (see Mattiucci et al., Reference Mattiucci, Cipriani, Levsen, Paoletti and Nascetti2018b). Preliminary results obtained by a multinuclear genes approach (Mattiucci et al., Reference Mattiucci, Acerra, Paoletti, Cipriani, Levsen, Webb, Canestrelli and Nascetti2016, Reference Mattiucci, Bello, Paoletti, Webb, Timi, Levsen, Cipriani and Nascetti2019; Palomba et al., Reference Palomba, Paoletti, Webb, Nascetti and Mattiucci2020 and unpublished data) have shown a differential distribution of hybrid genotype categories at the respective temperature tolerance limits of the 2 parental taxa within their overlapping geographic areas, such as the Alboran Sea waters and the Iberian Atlantic coast (Mattiucci et al., Reference Mattiucci, Palomba, Aco Alburqueque, Belli, Cipriani, Bello, Timi, Levsen, Webb and Nascetti2022 and unpublished data). Hypotheses about the possible enhanced fitness of hybrid genotype categories with respect to the parental taxa remain speculative but cannot be ruled out. Thus, monitoring the distribution of A. pegreffii and A. simplex (s.s.) and their hybrid genotype categories in these sympatric areas will constitute valuable predictive metrics for tracking climate change in these peculiar oceanographic basins.
TTHs as sentinels of environmental pollution
The Mediterranean marine environment receives a plethora of contaminants from both anthropogenic activities at sea and coastal areas affected by inland agricultural, industrial and urban sources. These pollutants can undergo biomagnification within marine food webs, leading to increased concentrations at higher trophic levels, depending on the feeding habits of predators (Sures and Nachev, Reference Sures and Nachev2022; Sures et al., Reference Sures, Nachev, Schwelm, Grabner and Selbach2023).
One interesting aspect of the interaction between some TTHs endoparasites and pollutants is the positive effect related to the presence of certain endoparasites, which seem to be able to mitigate some pollutant stressors for their host species. Indeed, the capacity of cestodes – and a lesser extent of nematodes – to accumulate pollutants in their tissues is well known, at concentrations higher than those found in the host's tissues (Sures and Nachev, Reference Sures and Nachev2022). This supports the evidence that infected hosts may experience less severe effects of pollutants compared to non-infected hosts (Sures and Nachev, Reference Sures and Nachev2022).
In this context, trypanorhynch cestodes, i.e. C. gracilis merocercoid larvae, infecting the flesh of Mediterranean fish from Egypt, were analysed for heavy metals (Cu, Pb and Cd) using atomic absorption spectrophotometry. The results indicated significantly higher concentrations of heavy metals in the parasite tissues compared with the muscles of its fish host. The study concluded that C. gracilis larvae could be used as bio-indicator of heavy metal pollution, adding further evidence to the usefulness of parasites in monitoring environmental impacts (Mahmoud et al., Reference Mahmoud, Alhindy and Fahmy2015).
A positive correlation between high abundance levels of parasites and microplastics has been recorded in European anchovies and the European pilchard from western Mediterranean waters (Pennino et al., Reference Pennino, Coll, Albo-Puigserver, Fernández-Corredor, Steenbeek, Giráldez, González, Esteban and Bellido2020). The authors noted that the Gulf of Lions, which showed the highest frequency of ingested microplastics, also had a higher infection rate with Anisakis in sardine and anchovies. These findings could be linked to the fact that both Anisakis spp. and microplastic are bioaccumulated in the fish host during the fish life history, through predation and feeding. However, a specific synergic effect between parasites infection and microplastic was not described.
Concentrations of mercury and polychlorinated biphenyls were also measured in the European hake infected with Anisakis larvae (Mille et al., Reference Mille, Soulier, Caill-Milly, Cresson, Morandeau and Monperrus2020). The authors found a positive correlation between the parasitic burden with Anisakis spp. and the methylmercury contamination in hake. This suggests that the presence of methylmercury can weaken the host's immune system enough to affect parasite infection intensity (Mille et al., Reference Mille, Soulier, Caill-Milly, Cresson, Morandeau and Monperrus2020). Indeed, it has been supposed that pollutants such as heavy metals and PCBs can influence host–parasite interactions (Marcogliese and Pietrock, Reference Marcogliese and Pietrock2011; Sures and Nachev, Reference Sures and Nachev2022). This, in turn, could exacerbate the pathological effect provoked by the parasite species on the host, whose immune response capacity may be compromised due to a particular pollutant, perhaps with a concomitant decline in its local population. Generally speaking, TTHs do not seem to have a pathogenic impact on their definitive hosts, likely due to the result of long co-evolutionary host–parasite adaptations. However, there are instances where TTHs have been linked to pathological conditions. For example, gastric lesions caused by adult A. simplex (s.l.) complex specimens have been reported in stranded delphinids from eastern Mediterranean waters (Hrabar et al., Reference Hrabar, Bočina, Gudan Kurilj, Đuras and Mladineo2017). However, the possibility of a synergistic pathological effect due to the presence of environmental pollutants and these endoparasites cannot be ruled out. Further investigation including experimental infection studies, using also additional innovative methodologies, is needed.
Conclusions and future directions
This comprehensive review represents an update on the biodiversity and ecology of chosen TTHs occurring in the Mediterranean Sea, as well as the first attempt to use them to detect the consequences of anthropogenic-driven impacts on marine food webs. The findings so far acquired on the distribution and parasitic burden of some TTHs underscore that marine ecosystem stability, with stable food webs and abundant host populations, is associated with large parasitic populations. Consequently, the overall abundance of parasites and the genetic variability of specialized multi-host parasites, such as some TTHs here considered, can reflect the state of trophic webs in the Mediterranean marine ecosystem.
However, in some cases, we have detected that the population size of some TTHs (for instance, A. pegreffii, H. corrugatum and H. incurvum) is lower with respect to other extra-Mediterranean populations. These findings suggest that, in comparison with other oceanographic areas, the trophic webs involving hosts through which those parasites complete their life cycles in the Mediterranean are somehow altered. Probably, the Mediterranean Sea, being an enclosed and vulnerable ecosystem, is rapidly responding to ongoing global environmental changes, and is thus less resilient to these stressors. However, paradoxically,a high parasite burden suggests a well-preserved and functioning ecosystem.
The findings also suggest that the metrics here proposed, i.e. estimates of genetic variability and infection rates of parasites, could serve as indicators for monitoring the status of diverse marine trophic webs and, more broadly, the ‘health state’ of the Mediterranean Sea, over both temporal and spatial scales. In the light of current global anthropogenic changes, it will be advisable to study their effects also on the alteration of intraspecific genetic diversity, since this represents the basis for any evolutionary change, and it is a fundamental level of biodiversity. Despite the increasing number of studies examining the effects of global changes on the biodiversity of parasite species (Fiorenza et al., Reference Fiorenza, Wendt, Dobkowski, King, Papaionou, Rabinowitz, Samhouri and Wood2019), very few studies focus on genetic diversity and are eventually related to the terrestrial ecosystem relevance (Gagne et al., Reference Gagne, Crooks, Craft, Chiu, Fountain-Jones, Malberg, Carver, Funk and Vande Wouude2020; Gupta et al., Reference Gupta, Robin and Dharmarajan2020). Future studies that will incorporate the intraspecific genetic variability of TTHs into investigations related to anthropogenic stressors will offer novel integrated avenues. These studies include the possibility to analyse population genetic structure based on genome-wide diversity, such as by dd-RADseq analysis, and genotyping individuals at climate-adaptive loci from different geographical populations. These investigations will also be possible with increased availability of genomic and transcriptomic resources for chosen TTHs (e.g. Cavallero et al., Reference Cavallero, Lombardo, Su, Salvemini, Cantacessi and D'Amelio2018; Palomba et al., Reference Palomba, Libro, Di Martino, Rughetti, Santoro, Mattiucci and Castrignanò2022, Reference Palomba, Libro, Di Martino, Roca-Geronès, Castrignanò, Canestrelli and Mattiucci2023b). These studies will enable evaluation of the evolutionary potential of parasite species, populations and genotypes also in the context of global change scenarios.
Recent insights emphasize that parasites with multiple obligate hosts could face a higher risk of extinction in a given geographical area due to potential losses or decreases in suitable hosts, leading to bottleneck events in their life cycle (Carlson et al., Reference Carlson, Hopkins, Bell, Doña, Godfrey, Kwak, Lafferty, Moir, Speer, Strona, Torchin and Wood2020; Sures et al., Reference Sures, Nachev, Schwelm, Grabner and Selbach2023). This situation has been observed among the TTHs in the Mediterranean Sea. Stranding events of cetaceans, sea turtles and sharks in the Mediterranean Sea can occur for several reasons, including natural causes such as illness, environmental factors, as well as anthropogenic causes including bycatch, vessel strikes, plastic ingestion or acoustic trauma. In this context, the General Fisheries Commission for the Mediterranean (GFCM) has adopted several recommendations aimed at preserving these species. For instance, GFCM/42/2018/2 and GFCM/44/2021/16 propose mitigation measures for the conservation of teleostean and elasmobranch species in the Mediterranean. These recommendations support data collection and monitoring of these vulnerable ‘flagship species’. Consequently, obtaining insights not only into threatened ‘flagship’ host species, but also on the endoparasites they harbour becomes crucial for predicting variations in distribution, population sizes, migration patterns and health status of ‘flagship’ host species in response to environmental changes, such as rising sea temperatures and pollutants. The joint effect of contaminant biomagnification and parasites in definitive host species of the chosen TTHs from the Mediterranean Sea remains poorly studied. Future transcriptomic investigations, focusing on stressor-exposed host conditions, potentially in comparison with co-infecting TTH parasites, may elucidate the pathogenic pathways modulated during parasite and pollutant exposures.
Finally, considering the observed trends of increasing SSTs in the Mediterranean, there is evidence that global warming is also impacting the distribution of some parasite species. The anisakids A. typica (s.l.) and S. sulcata, well adapted to warmer water temperatures of the eastern Mediterranean basin, are both showing a westward expansion, achieving higher parasitic loads in definitive and intermediate hosts in the warming waters of central Mediterranean Sea. Therefore, these TTHs might serve as ‘sentinels’ for tracking climate changes. Overall, it appears fundamental to apply an integrative approach in future studies to gain a comprehensive understanding of stress effects on TTHs to monitoring changes in the dynamics of host–parasite associations of the marine Mediterranean ecosystem.
Supplementary material
The supplementary material for this article can be found at https://doi.org/10.1017/S0031182023000951.
Data availability statement
Data availability is not applicable to this review article.
Acknowledgements
We would like to dedicate this review to Dr Giuseppe Nascetti, Professor Emeritus at Tuscia University , actually retired, for his scientific contributions in the field of ecology and evolution of the marine parasites considered in this review. The authors are grateful to 2 anonymous referees for their constructive and insightful comments to the first version of the MS, that have helped to improve this manuscript. We thank Dr Steve C. Webb (Cawthron Institute, New Zealand) for his valuable comments and corrections to the final version of the MS.
Author contributions
S. M., M. P., M. S. and P. C. conceived and designed the study. M. P., P. T., E. M., R. A.-A., B. B. and M. S. conducted data gathering; S. M., M. P., P. T., E. M., M. S. and A. G. wrote sections of the article; S. M., M. P., M. F., F. M., D. C., M. S. and P. C. reviewed the manuscript. All authors approved the last version of the manuscript.
Financial support
Part of the research was carried out by grants: - Sapienza-University of Rome, Tuscia University and Stazione Zoologica Anton Dorhn in the frame of the research ‘PARAsites do matter: looking for ‘SIGNAls’ of Thermal change and habitat distUrbance of Mediterranean maRine Ecosystem through a genetic and transcriptomic approach of a trophic-web transmitted parasite (PARA-SIGNATURE) carried out by 'Ministry of University and Research (MUR)-PRIN 2022'; - Sapienza-University of Rome, Grandi Progetti, Ateneo 2020; - Stazione Zoologica Anton Dorhn and Sapienza-University of Rome, in the frame of the research ‘Food-web transmitted endoparasites and their hosts: an integrative approach to investigate the “state” of biodiversity of the marine ecosystem from off Calabria coast’ carried out under the project ‘Centro Ricerche ed Infrastrutture Marine Avanzate in Calabria (CRIMAC)’, funded by FSC 2014–2020 – Piano Stralcio Ricerca e Innovazione 2015–2017 – Programma Nazionale Infrastrutture di Ricerca (PNIR), linea d'azione 1. Cofinanziamento Infrastrutture di Ricerca (IR); - Tuscia University, Young Independent Research Groups (YIRG); - Research project implemented under the National Recovery and Resilience Plan (NRRP), Mission 4 Component 2 Investment 1.4 – Call for tender No. 3138 of 16 December 2021, rectified by Decree n.3175 of 18 December 2021 of Italian Ministry of University and Research funded by the European Union – Next Generation EU. Project code CN_00000033, Concession Decree No. 1034 of 17 June 2022 adopted by the Italian Ministry of University and Research, CUP J33C22001190001 (Department of Veterinary Medical Sciences, Alma Mater Studiorum Università di Bologna), J83C22000860007 (Department of Ecological and Biological Sciences, University of Tuscia), C63C22000520001 (Stazione Zoologica ‘Anton Dohrn’), Project title ‘National Biodiversity Future Center – NBFC’; University of Padova, Progetti di Ateneo (CPDA149521/14).
Competing interests
None.
Ethical standards
Not applicable.