Introduction
The African buffalo (Syncerus caffer) plays a role as a reservoir or carrier of a wide number of arthropod-borne pathogens, such as Theileria, Ehrlichia, Babesia and Anaplasma species (Andrew and Norval, Reference Andrew and Norval1989; Allsopp et al., Reference Allsopp, Theron, Coetzee, Dunsterville and Allopp1999; Eygelaar et al., Reference Eygelaar, Jori, Mokopasetso, Sibeko, Collins, Vortser, Troskie and Oosthuizen2015; Machado et al., Reference Machado, Teixeira, Rodrigues, André, Gonçalves, Barbosa da Silva and Pereira2016). Moreover, buffaloes are known to harbour other economically important infectious pathogens, such as Mycobacterium tuberculosis, foot-and-mouth disease virus and Brucella abortus (Godfroid, Reference Godfroid2002; Michel et al., Reference Michel, Bengis, Keet, Hofmeyr, Klerk, Cross, Jolles, Cooper, Whyte, Buss and Godfroid2006; Van Schalkwyk et al., Reference Van Schalkwyk, Knobel, De Clercq, De Pus, Hendrickx and Van den Bossche2016). Although widely distributed throughout sub-Saharan Africa, the African buffaloes are currently confined to protected areas. The species distribution and numbers have been strongly reduced by habitat loss and hunting (IUCN – Downloaded on 29 July 2016).
Many native and exotic animal species have been selected for translocations procedures around the world (Seddon et al., Reference Seddon, Griffiths, Soorae and Armstrong2014; Soorae, Reference Soorae2016). The translocation is defined as the human-mediated movement of living organisms from one area to another (Woodford and Rossiter, Reference Woodford and Rossiter1993). Although the translocation of endangered species has become an important conservation approach, the project success depends to a large extent on the care with which wildlife biologists and veterinarians evaluate the suitability of the chosen release site (Woodford and Rossiter, Reference Woodford and Rossiter1993). Adequate managements are extremely important for the translocation procedures since the introduction of pathogens into naive resident wildlife community or the translocation of animals from free-vectors and pathogens sites to enzootic areas can be catastrophic.
In this context, Bartonella species and haemotropic mycoplasmas (also known as haemoplasmas) emerge as important arthropod-borne pathogens that have an impact in humans and animals’ health (Maggi et al., Reference Maggi, Compton, Trull, Mascarelli, Mozayeni and Breitschwerdt2013a; Breitschwerdt, Reference Breitschwerdt2014).
The Bartonella genus comprises a successful group of Gram-negative bacteria parasites (Birtles, Reference Birtles2005), which infects mainly erythrocytes and endothelial cells from a wide range of animal species, including humans (Breitschwerdt et al., Reference Breitschwerdt, Maggi, Chomel and Lappin2010; Harms and Dehio, Reference Harms and Dehio2012). This success is characterized by the high prevalence of the infection and diversity of host species (Birtles, Reference Birtles2005; Kosoy et al., Reference Kosoy, David and Kung-Sik2012). Currently, five (B. bovis, B. chomelii, B. schoenbuchensis, B. capreoli and B. melophagi) out of the 36 named Bartonella species/subspecies described have been associated with ruminants (Buffet et al., Reference Buffet, Kosoy and Vayssier-Taussat2013; Breitschwerdt, Reference Breitschwerdt2017).
Contrariwise, haemoplasmas are cell wall-less uncultivated epicellular bacteria that attach to red blood cells surface of a wide range of animals, including humans (Neimark et al., Reference Neimark, Johansson, Rikihisa and Tully2001; Maggi et al., Reference Maggi, Chitwood, Kennedy-Stoskopf and DePerno2013b). Among the haemoplasmas, Mycoplasma ovis, Mycoplasma wenyonii and ‘Candidatus Mycoplasma haemobos’ have been recognized as pathogens of domestic ruminants worldwide. In addition, an expanding number of Candidatus to new Bartonella and Mycoplasma species/genotypes have globally been reported in ruminants (Stoffregen et al., Reference Stoffregen, Alt, Palmer, Olsen, Waters and Stasko2006; Watanabe et al., Reference Watanabe, Fujihara, Obara, Matsubara, Yamauchi and Harasawa2010; Sato et al., Reference Sato, Kabeya, Yamazaki, Takeno, Suzuki, Kobayashi, Souma, Masuko, Chomel and Maruyama2012; Maggi et al., Reference Maggi, Chitwood, Kennedy-Stoskopf and DePerno2013b; Dahmani et al., Reference Dahmani, Sambou, Scandola, Raoult, Fenollar and Mediannikov2017).
Although the impact of bartonellae and haemoplasmas in livestock is still unknown, the gaps in the biology of these bacteria warrant further investigation. Indeed, there are limited data on the occurrence, distribution, genetic diversity, pathogenicity and transmission of arthropod-borne agents among wild ruminants.
The elucidation of these bacterial cycles in nature, including the identification of hosts, vectors and the species distribution in a particular ecotope shows great importance (Gutiérrez et al., Reference Gutiérrez, Cohen, Morick, Muncuoglu, Harrus and Gottlieb2014). Additionally, the African buffalo plays a role as reservoirs for vector-borne pathogens and may represent a serious threat to the livestock industry (Andrew and Norval, Reference Andrew and Norval1989; Allsopp et al., Reference Allsopp, Theron, Coetzee, Dunsterville and Allopp1999; Eygelaar et al., Reference Eygelaar, Jori, Mokopasetso, Sibeko, Collins, Vortser, Troskie and Oosthuizen2015; Van Schalkwyk et al., Reference Van Schalkwyk, Knobel, De Clercq, De Pus, Hendrickx and Van den Bossche2016).
Therefore, the present study aimed to investigate the occurrence of Bartonella and haemoplasmas infection in wild buffaloes (S. caffer) submitted to translocation in Sofala province, Mozambique, Africa.
Material and methods
Blood collection of African buffalo
The present study was carried out when Carlos L. Pereira was the Director of Conservation Gorongosa National Park, Mozambique. Animal management and welfare during the fieldwork with Cape buffaloes were conducted in accordance with national legislation on the use of animals for research implemented by the National Administration for the Conservation Areas (ANAC) of Mozambique. In 2011, blood samples were collected from 97 wild African buffalo (S. caffer) in Marromeu Reserve, Mozambique. This reserve is a special buffalo protection area located in the Marromeu district (Sofala Province) (Fig. 1), with an area of 1.500 km2 (http://www.jenmansafaris.com). Sampled animals were apparently healthy young male and female individuals. Approximately 10 mL of blood samples were collected of each animal before they were transferred from Marromeu Reserve (the site where there was contact with cattle) to the Gorongosa National Park (the site where there was no contact with cattle), distant around 300 kilometers from each other. The EDTA–blood were mixed (v/v) with ethanol, transported to the laboratory and posterior kept at −20 °C until sent an aliquot (~1 mL) to Brazil (Machado et al., Reference Machado, Teixeira, Rodrigues, André, Gonçalves, Barbosa da Silva and Pereira2016; Rodrigues et al., Reference Rodrigues, Garcia, Rodrigues, Costa-Martins, Pereira, Pereira, Bengaly, Neves, Camargo, Hamilton and Teixeira2017).
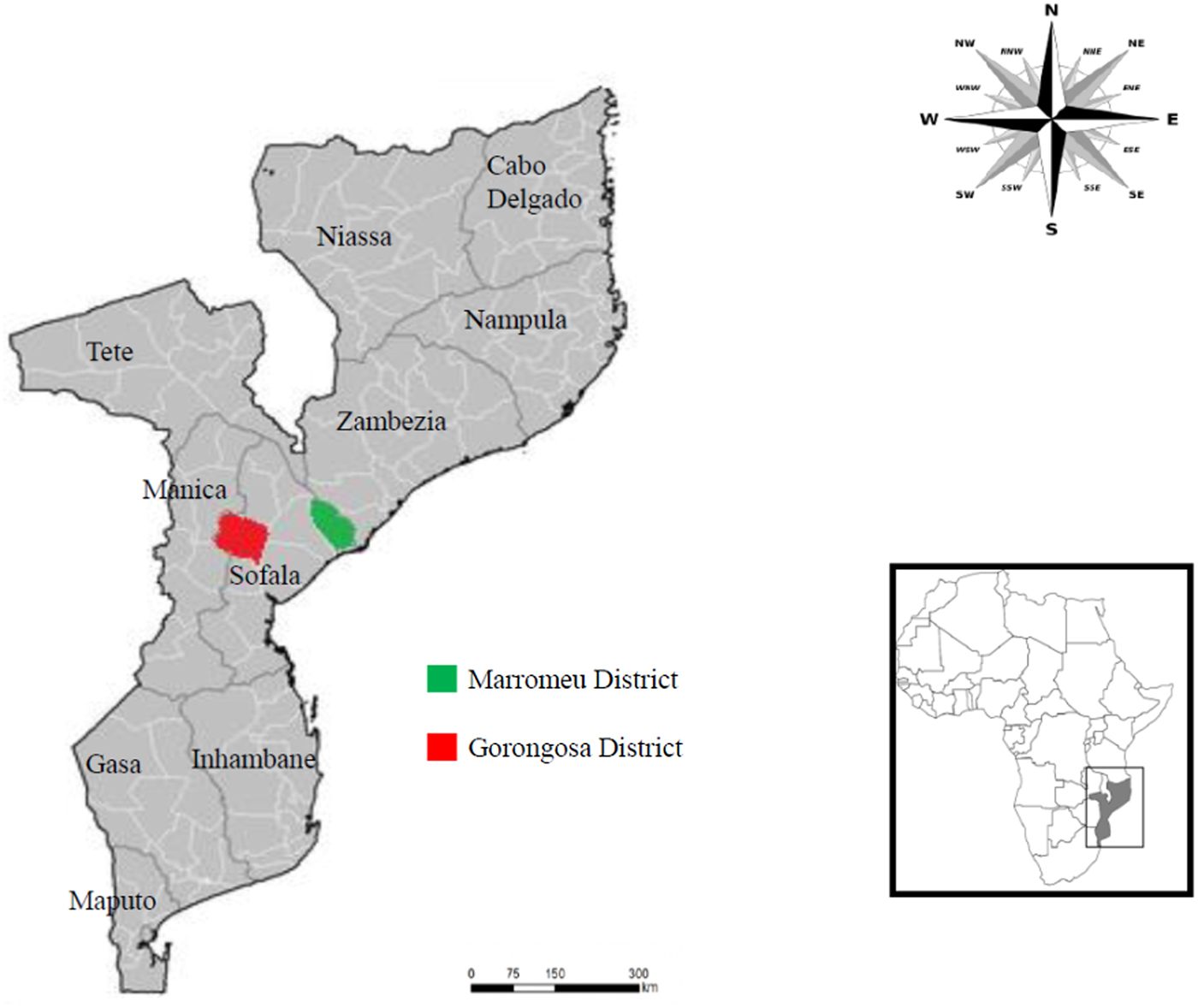
Fig. 1. Map of Mozambique country highlighting the districts where the buffalo were translocated.
Blood samples and DNA extraction
EDTA–blood samples collected from buffaloes were mixed (v/v) with ethanol for further DNA extraction. In Brazil, the blood samples from these buffaloes were incubated in a lysis buffer (1% SDS, 100 mm EDTA at pH 8.0, 20 mm Tris–HCl at pH 8.0 and 350 mg mL−1 of proteinase K) at 37 °C for 18 h and centrifuged at 14.000g for 5 min. The DNA was purified using Wizard Purification Systems (Promega). The concentration of each DNA sample was determined in a NanoDrop 2000c spectrophotometer (Thermo Scientific, San Jose, CA, USA) (Machado et al., Reference Machado, Teixeira, Rodrigues, André, Gonçalves, Barbosa da Silva and Pereira2016).
Molecular diagnosis of Bartonella and haemoplasmas species in African buffalo blood samples
A previously described broad range Taqman quantitative real-time PCR (qPCR) protocol based on nuoG gene was used aiming to detect Bartonella species DNA as previously described (André et al., Reference André, Dumler, Herrera, Gonçalves, de Sousa, Scorpio, de Santis, Domingos, de Macedo and Machado2016). The qPCR amplifications were conducted in low-profile multiplate unskirted PCR plates (BioRad, CA, USA) using a CFX96 Thermal Cycler (BioRad, CA, USA). Serial dilutions were performed aiming to construct standard curves with different concentrations of plasmid DNA (pIDTSMART – Integrated DNA Technologies) (2.0 × 107–2.0 × 100 copies μL−1) encoding an 83 bp insert of nuoG Bartonella henselae (André et al., Reference André, Dumler, Herrera, Gonçalves, de Sousa, Scorpio, de Santis, Domingos, de Macedo and Machado2016). qPCR assays were performed including duplicates of each buffalo DNA sample. All the duplicates showing a difference in Cq values higher than 0.5 were retested. Amplification efficiency (E) was calculated from the slope of the standard curve in each run using the following formula (E = 10–1/slope).
Additionally, two specific conventional PCR (cPCR) protocols based on 16S rRNA were used to amplify M. wenyonii (~530 bp) and ‘Candidatus M. haemobos’ DNA (279 bp) as previously described (Nishizawa et al., Reference Nishizawa, Sato, Fujihara, Sato and Harasawa2010; Su et al., Reference Su, Song, Lin, Yuan, Yang, Zhao, Huang and Zhu2010). Bartonella (KX086714), ‘Candidatus M. haemobos’ (KY328834) and M. wenyonii (KY328836) DNA samples previously obtained from naturally infected rodents and buffaloes (Gonçalves et al., Reference Gonçalves, Favacho, Roque, Mendes, Junior, Benevenute, Herrera, D'Andrea, de Lemos, Machado and André2016; Santos et al., Reference Santos, Brito, Abate, Paixão, Soares, Vieira, Garcia, Vieira and Vidotto2018), respectively, were used as positive controls. Ultra-pure sterile water and blood samples from calves previously tested negative for both pathogens were used as negative controls in all PCR assays described above. In order to prevent PCR contamination, DNA extraction, reaction setup, PCR amplification and electrophoresis were performed in separated rooms.
Molecular characterization of Bartonella and haemoplasma species
Samples from qPCR assays-positive buffalo were submitted to cPCR assays targeting four protein-coding genes with great potential to differentiation among related Bartonella species (La Scola et al., Reference La Scola, Zeaiter, Khamis and Raoult2003; Kosoy et al., Reference Kosoy, Mckee, Albayrak and Fofanov2017), namely gltA (350 bp), rpoB (825 bp), ftsZ (515 bp) and groEL (752 bp) genes, as previously described (Norman et al., Reference Norman, Regnery, Jamenson, Greene and Krause1995; Birtles and Raoult, Reference Birtles and Raoult1996; Renesto et al., Reference Renesto, Gouvernet, Drancourt, Roux and Raoult2001; Zeaiter et al., Reference Zeaiter, Fournier, Ogata and Raoult2002; Paziewska et al., Reference Paziewska, Harris, Zwolinska, Bajer and Sinski2011). Bartonella DNA previously detected in rodents was used as positive control (Gonçalves et al., Reference Gonçalves, Favacho, Roque, Mendes, Junior, Benevenute, Herrera, D'Andrea, de Lemos, Machado and André2016). On the other hand, in order to better characterize the initial PCR assay results, haemoplasma-positive buffalo samples were additionally submitted to a cPCR assay targeting a 16S rRNA larger fragment (~800 bp), as previously described (Maggi et al., Reference Maggi, Chitwood, Kennedy-Stoskopf and DePerno2013b). Mycoplasma haemofelis DNA previously detected in cats in Brazil was used as positive control (Santis et al., Reference Santis, Herrera, Sousa, Gonçalves, Denardi, Domingos, Campos, Machado and André2014).
All cPCR products were purified using Silica Bead DNA Gel Extraction Kit (Fermentas, SP, Brazil). Purified amplified DNA fragments were submitted to sequence confirmation in an automatic sequencer (ABI Prism 310 Genetic Analyser – Applied Byosystem/Perkin Elmer) in both directions using the same primers used for PCR detection. In order to correctly determine the nucleotide composition, the electropherograms were submitted to PhredPhrap analysis (Ewing et al., Reference Ewing, Hillier, Wendl and Green1998). The Phred quality score (peaks around each base call) was established higher than 20 (99% in the accuracy of the base call). Subsequently, the sequences were submitted to BLASTn and phylogenetic analyses.
Phylogenetic analyses of gltA, ftsZ and 16S rDNA sequences
The sequences obtained from gltA and ftsZ Bartonella and 16S rRNA haemoplasmas cPCR assays were identified by BLASTn, using the Megablast (highly similar sequences – using default parameters). Subsequently, obtained sequences were aligned with those retrieved from GenBank database using Clustal/W (Thompson et al., Reference Thompson, Higgins and Gibson1994), adjusted in Bioedit v. 7.0.5.3 (Hall, Reference Hall1999), and submitted to phylogenetic analysis. The maximum likelihood (ML) phylogenetic analysis was inferred with RAxML-HPC BlackBox 7.6.3 (Stamatakis et al., Reference Stamatakis, Hoover and Rougemont2008). Also, in order to perform a robust phylogenetic analysis among the protein-coding gene sequences, the Bartonella gltA and ftsZ nucleotide sequences were submitted to other two methods. Phylogenetic analysis based on Bayesian inference (BI) was done using MrBayes on XSEDE (v. 3.2.6) (the a posteriori probability values higher than 50% were accessed with 106 replicates; the first 25% trees were discarded as burn-in). Finally, these sequences were analysed by neighbour-joining (NJ) using the MEGA5.05 software. The AIC (Akaike information criterion) available on MEGA 5.05 software was applied to identify the most appropriate model of nucleotide substitution.
Results
Occurrence and molecular characterization of Bartonella and haemoplasma species in African buffalo
Out of 97 African buffalo blood samples submitted to Bartonella-qPCR, four (4.1%) were positive. The mean amplification efficiency was E = 90.7% [(ranging from 90.5 to 90.9%); slope = −3.568; r 2 = 0.996]. All blood samples showed low number of Bartonella-DNA copies μL−1 (#45 = 0.046 × 102; #50 = 0.041 × 102; #62 = 0.037 × 102; and #44 = 0.002 × 102 copies μL−1). Among the four blood samples positive to Bartonella in qPCR assay, only the sample #62 showed to be positive in gltA and ftsZ cPCR assays. None blood samples showed positive results in cPCR assays targeting rpoB and groEL genes. The gltA nucleotide sequence amplified in the present study shared 99% identity with B. bovis (KF199897) detected in cattle from Guatemala (Bai et al., Reference Bai, Malania, Castillo, Moran, Boonmar, Chanlun, Suksawat, Maruyama, Knobel and Kosoy2013). On the other hand, the obtained ftsZ nucleotide sequence shared 98% identity with Bartonella sp. (AB703117) previously detected in a Japanese Sika deer (Cervus nippon centralis) (Sato et al., Reference Sato, Kabeya, Yamazaki, Takeno, Suzuki, Kobayashi, Souma, Masuko, Chomel and Maruyama2012).
Additionally, 15.4% (15/97) samples were positive to haemoplasmas. Among them, 14.4% (14/97) and 4.1% (4/97) showed positive results to M. wenyonii, and ‘Candidatus M. haemobos’, respectively. Four (4.1%) samples were simultaneously positive to M. wenyonii and ‘Candidatus M. haemobos’. The two haemoplasmas amplicons sequenced shared 99 and 99% identity with M. wenyonii (KX171205) and ‘Candidatus M. haemobos’ (EF616468) nucleotide sequences detected in cattle from Mexico and Switzerland, respectively. None sample was simultaneously positive to Bartonella and haemoplasmas. The 16S rDNA sequences amplified in the present study showed query coverage ranging from 99 to 100%. All nucleotide sequences submitted to BLASTn and phylogenetic analyses were deposited in GenBank under the following access numbers: Bartonella (MF774324–MF774325) and haemoplasmas (MF981847 and MF992084).
Phylogenetic analysis
The phylogenetic analyses performed among the protein-coding gene sequences (gltA and ftsZ), using different methods (ML, MI and NJ) yielded congruent tree topologies (Figs 2, 3 and S1–S4). Additionally, according to BLASTn analysis, the Bartonella gltA and ftsZ sequences amplified from the same animal (#62) showed a distinct phylogenetic positioning in ML, BI and NJ analyses (Figs 2, 3 and S1–S4). The gltA sequence (MF774324), which shared 99% identity with B. bovis, clustered with other B. bovis sequences, including B. bovis sequences previously amplified in water buffalo from Thailand (Bai et al., Reference Bai, Malania, Castillo, Moran, Boonmar, Chanlun, Suksawat, Maruyama, Knobel and Kosoy2013), showing high support index (ranging from 97 to 100%) (Fig. 2 and S1, S2). On the other hand, although the ftsZ sequence (MF774325) clustered with other ruminant-associated Bartonella sequences, it was not closely related to any of these sequences, remaining in a separate branch in all different methods and supported by a high support index (ranging from 80 to 100%) (Fig. 3 and S3, S4).

Fig. 2. Phylogenetic relationships within the Bartonella genus based on the gltA gene. The tree was inferred by using the maximum likelihood (ML) method with the GTR + G + I model. The sequences detected in the present study are highlighted in bold. The numbers at the nodes correspond to bootstrap values higher than 50% accessed with 1000 replicates. Brucella melitensis was used as an outgroup.

Fig. 3. Phylogenetic relationships within the Bartonella genus based on the ftsZ gene. The tree was inferred by using the maximum likelihood (ML) method with the GTR + G model. The sequences detected in the present study are highlighted in bold. The numbers at the nodes correspond to bootstrap values higher than 50% accessed with 1000 replicates. Brucella melitensis was used as an outgroup.
Also in agreement to BLASTn analysis, the 16S rRNA sequences (MF981847 and MF992084) belonging to M. wenyonii and to ‘Candidatus M. haemobos’ when submitted to ML analysis were phylogenetically positioned near to other M. wenyonii and ‘Candidatus M. haemobos’ sequences, respectively, detected around the world and supported by high bootstrap values (87 and 100%, respectively) (Fig. 4).

Fig. 4. Phylogenetic relationships within the Mycoplasma genus based on a fragment of 800 bp of the 16S rRNA gene. The phylogenetic tree was inferred by using the maximum likelihood method with the GTR + G + I model. The sequences detected in the present study are highlighted in bold. The numbers at the nodes correspond to bootstrap values higher than 60% accessed with 1000 replicates. Mycoplasma pneumoniae was used as an outgroup.
Discussion
In the present study, the occurrence of Bartonella and haemoplasmas species was assessed in African buffaloes translocated from Marromeu to Gorongosa National Park in Mozambique. Although Bartonella and/or haemoplasmas species have been previously reported in domestic animals (dogs, cats and cattle) (Gundi et al., Reference Gundi, Olivier, Davous, Raoult and La Scola2004; Willi et al., Reference Willi, Tasker, Boretti, Doherr, Cattori, Meli, Lobetti, Malik, Reusch, Lutz and Hofmann-Lehmann2006; Dahmani et al., Reference Dahmani, Sambou, Scandola, Raoult, Fenollar and Mediannikov2017), wild animals (rodents, bats and cheetahs) (Kosoy et al., Reference Kosoy, Bay, Lynch, Kuzmin, Niezgoda, Franka, Agwanda, Breiman and Rupprecht2010; Kamani et al., Reference Kamani, Morick, Mumcuoglu and Harrus2013; Krengel et al., Reference Krengel, Meli, Cattori, Wachter, Willi, Thalwitzer, Melzheimer, Hofer, Lutz and Hofmann-Lehmann2013) and haematophagous arthropods (soft ticks and bat flies) (Billeter et al., Reference Billeter, Hayman, Peel, Baker, Wood, Cunningham, Suu-Ire, Dittmar and Kosoy2012; Mediannikov et al., Reference Mediannikov, Diatta, Kasongo and Raoult2014) from African continent, the present work presented, for the first time, the occurrence of these arthropod–bacteria species in S. caffer.
Similarly to the occurrence of Bartonella in water buffalo [Bubalus bubalis (6.8%; 7/103)] from Thailand (Bai et al., Reference Bai, Malania, Castillo, Moran, Boonmar, Chanlun, Suksawat, Maruyama, Knobel and Kosoy2013), a low occurrence was observed among the animals analysed in the present study (4.1%; 4/97). Additionally, the prevalence of haemoplasmas reported in the African buffaloes was lower than that previously reported in buffaloes (32%; 8/25) from China (Su et al., Reference Su, Song, Lin, Yuan, Yang, Zhao, Huang and Zhu2010). However, it is important to highlight that the animals selected in the latter study were showing different clinical signs, such as emaciation, anorexia and decreased milk yields (Su et al., Reference Su, Song, Lin, Yuan, Yang, Zhao, Huang and Zhu2010). In addition to animal health status, the difference in the occurrence of Bartonella and haemoplasmas observed in different countries have been attributed to different factors, such as distribution and abundance of arthropod vectors, and environment and landscape features, which could influence the exposure to these agents (Bai et al., Reference Bai, Malania, Castillo, Moran, Boonmar, Chanlun, Suksawat, Maruyama, Knobel and Kosoy2013).
Syncerus caffer is frequently infested by species of Hyalomma, Rhipicephalus and Amblyomma ticks (Carmichael, Reference Carmichael1976; Anderson et al., Reference Anderson, Ezenwa and Jolles2012; Kariuki et al., Reference Kariuki, Penzhorn and Horak2012). These ticks are responsible for transmission of several pathogens frequently reported in African buffalo, such as Theileria spp., Anaplasma spp., Ehrlichia spp. and Babesia spp. (Andrew and Norval, Reference Andrew and Norval1989; Eygelaar et al., Reference Eygelaar, Jori, Mokopasetso, Sibeko, Collins, Vortser, Troskie and Oosthuizen2015; Machado et al., Reference Machado, Teixeira, Rodrigues, André, Gonçalves, Barbosa da Silva and Pereira2016). Therefore, it suggests that Bartonella and haemoplasma species detected in S. caffer in the present study may be potentially transmitted by these tick species. In addition to possible role of ticks in the transmission of Bartonella, several studies have reported the isolation or molecular detection of Bartonella species in other blood-sucking arthropods associated with wild and domestic ruminants (Chung et al., Reference Chung, Kasten, Paff, Van Horn, Vayssier-Taussat, Boulouis and Chomel2004; Dehio et al., Reference Dehio, Sauder and Hiestand2004; Halos et al., Reference Halos, Jamal, Maillard, Girard, Guillot, Chomel, Vayssier-Taussat and Boulouis2004; Duodu et al., Reference Duodu, Madslien, Hjlelm, Molin, Paziewska-Harris, Harris, Colquhoun and Ytrehus2013; Gutiérrez et al., Reference Gutiérrez, Cohen, Morick, Muncuoglu, Harrus and Gottlieb2014). Regarding the haemoplasma transmission, few studies have accessed the mechanisms of transmission by arthropod vectors. Prullage et al. (Reference Prullage, Williams and Gaafar1993) demonstrated the mechanical transmission of Mycoplasma suis by Stomoxys calcitrans and Aedes aegyptii among susceptible splenectomized pigs. Woods et al. (Reference Woods, Brewer, Hawley, Wisnewski and Lappin2005) showed that Ctenocephalides felis is a possible vector of M. haemofelis and ‘Candidatus M. haemominutum’ among cats. Additionally, bovine-associated Mycoplasma species were molecularly detected in ticks belonging to Dermacentor andersoni (Neimark et al., Reference Neimark, Johansson, Rikihisa and Tully2001), Rhipicephalus (Boophilus) microplus and Haemaphysalis bispinosa species (Mohd Hasan et al., Reference Mohd Hasan, Kho, Koh, Hassan Nizam and Tay2017), among horn (Haematobia irritans), stable (S. calcitrans), horse (Tabanus spp.) (Hornok et al., Reference Hornok, Micsutka, Meli, Lutz and Hofmann-Lehmann2011) and house (Musca domestica) flies, as well as in lice (Haematopinus eurysternus) (Hofmann-Lehmann et al., Reference Hofmann-Lehmann, Meli, Dreher, Gönczi, Deplazes, Braun, Engels, Schüpbach, Jörger, Thoma, Griot, Stärk, Willi, Schmidt, Kocan and Lutz2004). These findings suggested that these arthropods may have an active role in the maintenance and transmission of ruminants-associated Bartonella and haemoplasma species. However, experimental studies aiming to analyse the vectorial competence of selected arthropod species are much needed.
In contrast to the phylogenetic analysis (ML) performed with haemoplasmas sequences amplified from S. caffer blood samples, which clustered with other M. wenyonii and ‘Candidatus M. haemobos’ sequences detected around the world, the Bartonella gltA and ftsZ sequences detected in the same animal (#62) were positioned in different branches in all different methods analysed (ML, BI and NJ). This uncertainty about the phylogenetic positioning could be explained by two hypotheses. Firstly, there would have been a coinfection with different Bartonella species/genotypes (B. bovis identified by gltA sequence, and a new Bartonella genotype closely related to other ruminant-associated Bartonella identified by ftsZ sequence). Alternatively, the amplified sequences might have represented an infection with a genotype that went through recombinant events. Indeed, the latter phenomena have been already reported in Bartonella species from wild rodents (Harrus et al., Reference Harrus, Bar-Gal, Golan, Elazari-Volcani, Kosoy, Morick, Aviador and Baneth2009; Paziewska et al., Reference Paziewska, Harris, Zwolinska, Bajer and Sinski2011), cattle (Gutiérrez et al., Reference Gutiérrez, Cohen, Morick, Muncuoglu, Harrus and Gottlieb2014) and bats (Bai et al., Reference Bai, Hayman, Mckee and Kosoy2015). These distinct possibilities reinforce the great challenge on Bartonella identification based on direct molecular detection in blood, tissue or ectoparasite samples (Gutiérrez et al., Reference Gutiérrez, Cohen, Morick, Muncuoglu, Harrus and Gottlieb2014; Kosoy et al., Reference Kosoy, Mckee, Albayrak and Fofanov2017). Although the multiple loci sequencing approach was attempted in the present study for a better understanding of sequences phylogenetic positioning and possible recombinant events and/or infection by multiple Bartonella species (Kosoy et al., Reference Kosoy, Mckee, Albayrak and Fofanov2017), the unique positive sample (#62) in cPCR assays was negative in PCR targeting additional genes (rpoB and groEL), thus precluding to solve this issue. Additional culturing of isolates would have benefited the differentiation of these genotypes/species (Kosoy et al., Reference Kosoy, Mckee, Albayrak and Fofanov2017).
Considering that buffaloes usually do not show clinical signs of tick-borne diseases, a surveillance on this animal species is much needed since they act as carriers for arthropod-borne pathogens to the livestock (Andrew and Norval, Reference Andrew and Norval1989; Allsopp et al., Reference Allsopp, Theron, Coetzee, Dunsterville and Allopp1999; Eygelaar et al., Reference Eygelaar, Jori, Mokopasetso, Sibeko, Collins, Vortser, Troskie and Oosthuizen2015; Van Schalkwyk et al., Reference Van Schalkwyk, Knobel, De Clercq, De Pus, Hendrickx and Van den Bossche2016). Furthermore, since these animals can migrate over large distances, they could spread different pathogens for susceptible wildlife. In the present study, at least three bovine-associated pathogens, namely B. bovis, M. wenyonii and ‘Candidatus M. haemobos’ were reported in S. caffer. Regarding the pathogenic potential of the above-mentioned agents, while B. bovis have been associated with bovine endocardits (Mailard et al., Reference Mailard, Petit, Chomel, Lacroux, Schelcher, Vayssier-Taussat, Haddad and Bolouis2007; Erol et al., Reference Erol, Jackson, Bai, Sells, Locke, Kosoy, Erol, Jackson, Bai, Sells, Locke and Kosoy2013), bovine haemoplasmas species have been associated with anaemia, transient fever, decreased milk production, anorexia, weight loss and infertility (Smith et al., Reference Smith, Thrall, Smith, Salman, Ching and Collins1990; Su et al., Reference Su, Song, Lin, Yuan, Yang, Zhao, Huang and Zhu2010; Hoelzle et al., Reference Hoelzle, Winkler, Kramer, Wittenbrink, Dieckmann and Hoelzle2011).
In the Marromeu Reserve, the site where animals were caught, buffaloes and cattle used to share the same area. Although little is known about the origin, evolution and dispersion of Bartonella and haemoplasmas species, the low prevalence detected in African buffaloes, mainly to Bartonella, coupled to a wide distribution and the constant detection of these bacterial in cattle around the world, suggest that these pathogens may have been transmitted via arthropod-vectors from cattle to buffaloes. On the other hand, there is no contact among cattle and wildlife in Gorongosa National Park, the site where the animals were released. Therefore, the sylvatic animals residing in this area, mainly other ruminant species may be exposed to B. bovis, M. wenyonii, ‘Candidatus M. haemobos’ and Anaplasma spp., as previously reported (Machado et al., Reference Machado, Teixeira, Rodrigues, André, Gonçalves, Barbosa da Silva and Pereira2016). Although it is difficult to assess the impact of these infections in such population, this situation can be catastrophic to immunologically naïve wildlife (Woodford and Rossiter, Reference Woodford and Rossiter1993).
Even though translocation procedures have intended conservation benefits, this attempt may also impose risks to animal health (IUCN/SSC, 2013). During management procedures, it is important to consider that a translocated animal does not represent a single species, but is rather a biological package containing a selection of viruses, bacteria, protozoa, helminths and arthropods. Different reports have showed the complexity of the translocation procedures, either by diseases introduced through the animals or diseases contracted from the resident animals at the release site (reviewed by Woodford and Rossiter, Reference Woodford and Rossiter1993). Therefore, the veterinary aspects, prior, during and after translocation procedures are extremely important in order to minimize the impact on animal health. Indeed, few studies have been conducted regarding arthropod-borne pathogens in the capture (Marromeu Reserve) and the release (Gorongosa National Park) sites. For instance, Rift Valley fever phlebovirus was detected in buffaloes and cattle sampled in both places between the years of 2013 and 2014 (Moiane et al., Reference Moiane, Mapaco, Thompson, Berg and Albihn2017). Recently, Trypanosoma vivax and T. vivax-like DNA was amplified in buffaloes and tsetse flies (Glossina spp.) captured in Gorongosa between the years of between 2007 and 2014 (Rodrigues et al., Reference Rodrigues, Garcia, Rodrigues, Costa-Martins, Pereira, Pereira, Bengaly, Neves, Camargo, Hamilton and Teixeira2017). These findings reinforce the need for further studies in order to better assess the impact of the translocation process on resident fauna.
Although in the present study we were unable to solve the issue regarding the possible occurrence of co-infection or recombination events by distinct Bartonella species, future studies using cloning of amplicons prior to sequencing and the isolation of bartonellae in blood or chocolate agar may help solving this problem, improving the molecular identification and characterization of this important bacterial group in wild ruminants.
In summary, our study showed the molecular occurrence of B. bovis, a possible new Bartonella genotypes/species or a genotype resulted from recombination events, M. wenyonii and ‘Candidatus M. haemobos’ in African buffaloes submitted to translocation from Marromeu to Gorongosa National Park. Additionally, African buffaloes and other sympatric ruminants living in the release site (Gorongosa National Park) should be evaluated in the future in order to assess the impact of these pathogens in the ecosystem.
Supplementary material
The supplementary material for this article can be found at https://doi.org/10.1017/pao.2018.10.
Acknowledgments
We are grateful to a number of colleagues, students and local people who helped Dr Carlos Lopes Pereira with the fieldwork for animals capture in the Marromeu Reserve.
Financial support
This work was supported by grants from the FAPESP (Fundação de Amparo à Pesquisa do Estado de São Paulo – Process number #2015/14896-1) and CNPq (Conselho Nacional de Desenvolvimento Científico e Tecnológico – Bolsa de Produtividade em Pesquisa – Process number 302420/2017-7) delivered to M.R.A. and the Brazilian Agency CNPq within the PROAFRICA to M.M.G.T.
Conflicts of interest
None.
Ethical standards
Not applicable.