Introduction
Variable harlequin frogs Atelopus varius are brightly coloured Critically Endangered frogs occurring in Costa Rica and Panama that have declined significantly as a result of chytridiomycosis, a disease caused by the fungal pathogen Batrachochytrium dendrobatidis (Bd; Escobedo-Galván et al., Reference Escobedo-Galván, Wyatt, González-Maya, Schipper, Belant and Fischer2013; McCaffery et al., Reference McCaffery, Richards-Zawacki and Lips2015; Lewis et al., Reference Lewis, Richards-Zawacki, Ibáñez, Luedtke, Voyles, Houser and Gratwicke2019). Most species in the genus Atelopus are now threatened with extinction (La Marca et al., Reference La Marca, Lips, Lötters, Puschendorf, Ibáñez and Rueda-Almonacid2005; Scheele et al., Reference Scheele, Pasmans, Skerratt, Berger, Martel and Beukema2019), leading the Panama Amphibian Rescue and Conservation Project to develop captive management and ex situ reproduction programmes of extant populations of Atelopus in Panama (Gratwicke et al., Reference Gratwicke, Ross, Batista, Chaves, Crawford and Elizondo2016; Lewis et al., Reference Lewis, Richards-Zawacki, Ibáñez, Luedtke, Voyles, Houser and Gratwicke2019). Persisting wild populations have been observed in some warmer climates that are less suitable for the fungus, leading to the formulation of a ‘climate refuge’ hypothesis (Puschendorf et al., Reference Puschendorf, Hoskin, Cashins, McDonald, Skerratt, Vanderwal and Alford2011; McCaffery et al., Reference McCaffery, Richards-Zawacki and Lips2015), in which it is proposed that reduced Bd transmission could allow Atelopus populations to persist in spite of significant Bd-related mortality (Lampo et al., Reference Lampo, Señaris and García2017). In addition, some persisting populations have evolved anti-Bd skin secretions that could explain their persistence (Voyles et al., Reference Voyles, Woodhams, Saenz, Byrne, Perez and Rios-Sotelo2018), and recent migration and gene flow are evident in these populations, raising the potential of genetic rescue through outbreeding (Byrne et al., Reference Byrne, Richards-Zawacki, Voyles, Bi, Ibáñez and Rosenblum2021). However, it was recently demonstrated that skin secretions of the Panamanian golden frog Atelopus zeteki could increase susceptibility to chytridiomycosis (Gass & Voyles, Reference Gass and Voyles2022), and thus understanding specific components of skin defence is required (reviewed in Woodhams et al., Reference Woodhams, McCartney, Walke and Whetstone2023).
The threat of Bd in Panama has not abated, but captive-bred animals have been a valuable tool in efforts to understand the disease and test potential solutions in ex situ contexts (Lewis et al., Reference Lewis, Richards-Zawacki, Ibáñez, Luedtke, Voyles, Houser and Gratwicke2019; Becker et al., Reference Becker, Brophy, Barrett, Bronikowski, Evans and Glassey2021). Rapid declines of animals following Bd outbreaks have led to the disappearance of highly susceptible species before in situ disease dynamics could be adequately studied (McCaffery et al., Reference McCaffery, Richards-Zawacki and Lips2015), and studies of small persisting populations are challenging because of sample size limitations (Perez et al., Reference Perez, Richards-Zawacki, Krohn, Robak, Griffith and Ross2014). A few studies of Bd in wild Atelopus populations suggest that thermal context is important in persistence and disease dynamics (Richards-Zawacki, Reference Richards-Zawacki2010; Lampo et al., Reference Lampo, Señaris and García2017; Sauer et al., Reference Sauer, Fuller, Richards-Zawacki, Sonn, Sperry and Rohr2018). Carefully designed in situ studies could shed valuable light on the potential for interventions that could favour those frogs facing disease threats (Scheele et al., Reference Scheele, Hunter, Grogan, Berger, Kolby and McFadden2014). However, conducting in situ studies of released animals is not straightforward, and captive-bred animals face many threats in addition to disease as they transition back into the wild. Furthermore, concern has been expressed that susceptible species that can develop high Bd loads could act as supershedders of Bd, and so their release could have negative impacts on other species living at the release site (DiRenzo et al., Reference DiRenzo, Langhammer, Zamudio and Lips2014).
There are many unique, taxa-specific challenges when performing a reintroduction of A. varius frogs. For example, A. varius is aposematically coloured and diurnal and probably relies on its toxins for predator defence. Atelopus varius is known to possess tetrodotoxin (TTX), a powerful water-soluble toxin thought to be of bacterial origin, and when reared in captivity the production of these chemical defences is reduced or eliminated (Daly et al., Reference Daly, Padgett, Saunders and Cover1997). This raises questions about both the mechanism of production and how long it will take a reintroduced frog to regain its chemical defences. The recovery time of natural skin defences is an important question for future reintroductions, as without these defences, predators could potentially pose a greater threat to reintroduced animals than the chytrid fungus. We hypothesize that skin toxicity will recover upon exposure to natural diets and/or symbiotic bacteria.
Another issue to consider is that wild A. varius are known to be territorial, and both sexes exhibit homing behaviour to within 1 m of their original territory if displaced (Crump, Reference Crump1986). When conducting surveys, males are more frequently encountered on stream banks than females (Crump, Reference Crump1988), and it is unclear whether these differences are because of habitat preferences, skewed sex ratios or differences in detectability. After the emergence of Bd at a site, the chances of re-encountering females increased, suggesting that this water-borne disease could have more rapidly reduced stream-dwelling males than females that are less closely linked to streamside habitats (McCaffery et al., Reference McCaffery, Richards-Zawacki and Lips2015). When placing captive-bred animals into the wild, understanding sexually dimorphic dispersal behaviour could be critical to disease ecology, site fidelity and sex-based recapture probabilities.
Intensively monitored pilot releases have been recommended to facilitate understanding of the threats faced by animals after reintroduction, but poor recapture rates are a recurring problem in many amphibian reintroduction projects, limiting the potential for effective research or adaptive management (Linhoff et al., Reference Linhoff, Soorae, Harding, Donnelly, Germano and Hunter2021). Post-release monitoring of reintroduced populations is challenging because of low or variable detection probabilities and high numbers of unobservable individuals. Developing methods to monitor released animals effectively is therefore an essential requirement to advance in situ conservation of this threatened species.
The primary objective of this study was to conduct the first release trial of A. varius. Our goals were to (1) improve our ability to monitor populations post-release, (2) observe dispersal patterns as individuals transition from captivity to the wild, (3) increase our understanding of immediate threats to released animals and (4) observe whether natural skin toxin defences can be restored after a transition to the wild through implementing outdoor mesocosm trials. A synchronous objective to the release trial was to observe Bd dynamics in wild amphibian communities at the release site compared to a similar site where no release was conducted.
Study area
We conducted the study in the Donoso area, Colón Province, Republic of Panama, within the Río Caimito basin at 50–140 m elevation. The natural vegetation of this area comprises tropical lowland evergreen rainforest, subjected to c. 4,500 mm mean annual precipitation (IGNTG, 2016). Estimated precipitation is 2,500–3,000 mm during the rainy season (mid May to mid December) and 1,000–1,200 mm during the dry season (mid December to mid May; ETESA Hidrometerología, Reference idrometerología2020). A large open-pit mining operation is being developed in the Donoso area. We selected two accessible first-order perennial streams with protected, forested catchments within the known range of A. varius that appeared to be suitable habitat even though they are not known historical localities for the species. The nearest known historical locality for A. varius was c. 10 km to the south. We simultaneously monitored the amphibian community at the release stream (8.91582°N, 80.66270°W) and a control stream c. 650 m away (8.91186°N, 80.65733°W).
Methods
Provenance of animals
We selected 470 captive-bred A. varius individuals for the release trial. The individuals were 1–2 years old and were derived from seven clutches, representing 14 lowland founder animals from the Donoso area that had been collected as part of the Panama Amphibian Rescue and Conservation Project. These comprised 279 females (mean weight 4.5 ± SD 1.7 g, mean snout to vent length 39 ± SD 5 mm) and 191 males (mean weight 3.0 ± SD 0.9 g, mean snout to vent length 33 ± SD 4 mm). Pre-release, we swabbed and confirmed as negative for Bd one frog from each holding tank housing up to 10 same-sex individuals, and a veterinarian (EK) examined all individual animals.
Tracking protocol
We fitted 12 males and 18 females with radio transmitters to observe the dispersal patterns of the frogs and compare the movements of males and females. We used 0.31 g LB-2X transmitters (Holohil Systems Ltd, Carp, Canada; 6–12% of the body mass of the animals) with a 21 day battery life and a mean detection range of 80–120 m depending on habitat and topography. We attached the transmitter to the waist of the frog using cotton threaded through 1 mm (inner diameter)–2 mm (outer diameter) surgical silicone tubing (Plate 1; Bartelt et al., Reference Bartelt, Peterson, Landler, Ringler, Hödl and Crawford2000; Rowley & Alford, Reference Rowley and Alford2007; Pašukonis et al., Reference Pašukonis, Loretto, Landler, Ringler and Hödl2014). The transmitter of larger frogs had an additional tubing attachment around the chest. A test on a captive frog showed that the cotton thread degraded in c. 30 days, serving as an auto-release mechanism (Rowley & Alford, Reference Rowley and Alford2007). We released animals at 5–10 m intervals along the 150 m stream transect on 17 January 2018. Weather permitting, we attempted to locate animals once daily using a Biotracker radio receiver (Lotek, Ontario, Canada), and we documented all recapture locations using a GPS with a mean error of 7 ± SD 3 m at the release site. We searched with the radio telemetry equipment up to c. 30 m upstream and c. 30 m downstream of the release site. After 11 days, we removed radiotracking devices at the first recapture and released the frogs. We mapped dispersal paths using QGIS 3.10.2 (QGIS Development Team, 2021). We were able to calculate the 11- or 12-day dispersal distance for seven males and eight females using the Haversine formula (Robusto, Reference Robusto1957), to determine the distance moved between the release location and the last post-release encounter 11 or 12 days later. The dispersal distances between male and female frogs did not meet assumptions of normality or homogeneity of variance for parametric tests, so we compared them using a non-parametric Wilcoxon rank-sum test in R 4.0 (R Core Team, 2021).
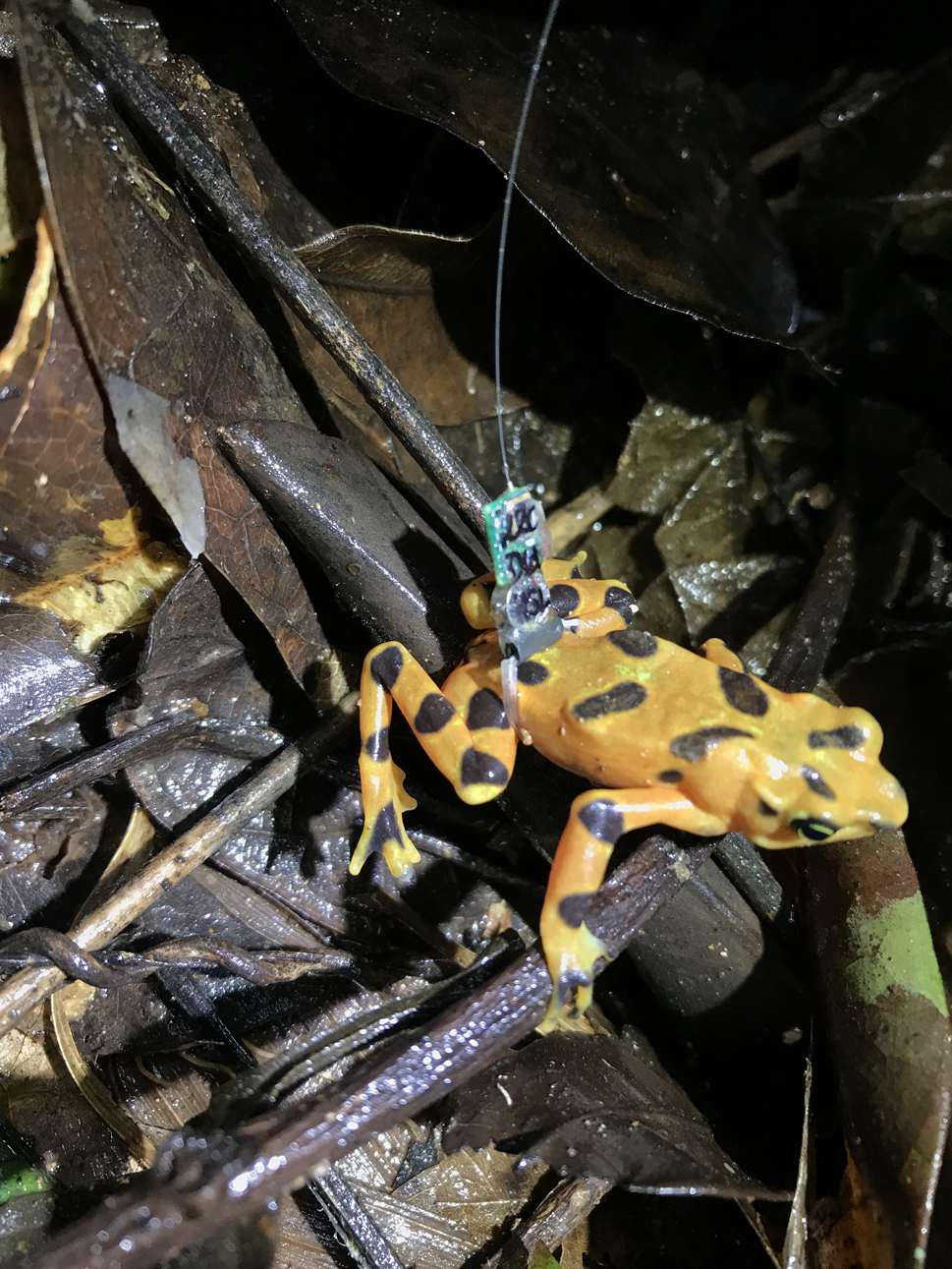
Plate 1 Variable harlequin frog Atelopus varius fitted with a 0.3 g radio transmitter.
Mark–recapture
We adopted a robust recapture design for this study as it assumes open populations, allowing for immigration, mortality and recruitment between intensive concurrent resampling periods that assume closed populations (Pollock, Reference Pollock1982). This method has been successfully used to monitor wild Atelopus populations of 50–100 animals (Tarvin et al., Reference Tarvin, Peña and Ron2014). We uniquely marked frogs for recapture analysis through a combination of a single toe-clip and coloured visual implant elastomer (NW Marine Technology, Anacortes, USA), injected into the palm of a hand or foot (Dodd, Reference Dodd2010). We took individual dorsal and ventral photos of each frog as a back-up to validate individual IDs if necessary using pattern-mapping (Dodd, Reference Dodd2010). We released 428 frogs for the mark–recapture study. We divided these into 15 groups (28–30 frogs in each group) that we freed along the margins of the release stream split into 15 pre-marked transect segments every 10 m along a 150 m transect on 17 January 2018 (Supplementary Material 1). We selected six gravid females visually determined to be at grade 5 on the Atelopus gravidity scale of Bronson et al. (Reference Bronson, Guy, Murphy, Barrett, Kouba, Poole and Kouba2021). We housed each gravid female in its own small enclosure and paired her with an unrelated male the night before release, and we released the four males that amplexed the gravid females as amplectant pairs; we released all others individually. We established three survey transects at the release site: transect 1 was directly on the stream and where the animals were released; transects 2 and 3 were 10 m either side of transect 1, in parallel. One trained surveyor walked transects 2 and 3 in a single direction, searching c. 2 m either side in a standard visual encounter survey, with effort standardized by transect length (Heyer et al., Reference Heyer, Donnelly, Foster and McDiarmid1994). Two trained surveyors walked the stream transect, with each focusing on their side of the stream and bank. We resampled the transects by visually resurveying for Atelopus only on seven occasions, each consisting of 3 consecutive sampling days on which we walked each transect once per day. We conducted these diurnal visual encounter surveys immediately following release, at 10 days after release and, subsequently, at c. monthly intervals for 5 months. We analysed recapture data using Rcapture 1.4.3 in R, following a robust design (Pollock, Reference Pollock1982; Rivest & Baillargeon, Reference Rivest and Baillargeon2019). We swabbed all recaptured frogs from day 10 onwards to check for Bd.
Skin toxicity
We evaluated recovery of TTX skin toxicity using three groups of frogs. We euthanized immediately the first group of five frogs (pre-release) to examine TTX quantities in captivity, and we housed individually the second group of 12 frogs in 12 outdoor terrestrial mesocosms filled to depths of 10–15 cm with leaflitter collected from the field environment. Each mesocosm was an 80 × 76 × 45 cm cage constructed from 0.7 cm gauge firm plastic mesh. We euthanized five frogs at day 27, and we euthanized the remaining seven frogs at day 79. We euthanized frogs using a topical overdose of 20% benzocaine anaesthetic Orajel (AVMA, 2007; Cecala et al., Reference Cecala, Price and Dorcas2007). We preserved dissected skins from euthanized frogs in 50 ml Falcon tubes filled with methanol and stored at −80 °C prior to extraction and analysis.
We used an analytical method adapted from Bane et al. (Reference Bane, Hutchinson, Sheehan, Brosnan, Barnes, Lehane and Furey2016), as follows: we filtered crude extracts from all samples through a 1 ml Norm-Ject disposable syringe attached to an Acrodisc CR 13 mm, 0.2 μm polytetrafluoroethylene membrane filter. We evaporated the methanol from the crude extracts in vacuo in a DR 120 SpeedVac with the drying rate on low. We stored the dried crude extracts at −80 °C until ready for liquid chromatography–mass spectrometry analysis, at which point we reconstituted them in 100 μl of high-performance liquid chromatography-grade methanol and transferred them via micropipette into 0.25 ml polypropylene autosampler vials for liquid chromatography–mass spectrometry analysis. We analysed the crude extracts via high-performance liquid chromatography using a Shimadzu LC-20 liquid chromatograph equipped with a Phenomenex Luna HILIC column (3 μm, 150 × 4.6 mm) and an Applied Biosystems SCIEX API 2000 triple quadrupole mass spectrometer. We separated the samples with a binary mobile phase flowing at 0.4 ml/min consisting of water (1 l) with formic acid (75 μl) and ammonium hydroxide (300 μl; solvent A) and 70:30 water:acetonitrile (1 l) with formic acid (100 μl; solvent B). The gradient was as follows: 70% B (2 min hold) ramped to a mobile phase concentration of 30% B over 3 min (5 min hold) before being ramped back to 70% B for the remainder of the run. Each run lasted 15 min. We determined the behaviour of TTX using a commercially available TTX standard (Toronto Research Chemicals, North York, Canada); under these conditions, the retention time was c. 6.7 min. We performed mass spectrometry analyses using positive electrospray ionization in single quadrupole scan mode or multiple reaction monitoring mode scanning for transitions of 320→ 302 and 320→ 162 (Tsujimura & Yamanouchi, Reference Tsujimura and Yamanouchi2015). For the multiple reaction monitoring mode scan, we optimized the declustering potentials and collision energies for each transition at 30 and 50 V, respectively. We obtained all spectra under Analyst 1.6.2 (SCIEX, Framingham, USA) control. This method successfully detected small TTX quantities from other amphibian species (K. Minbiole & J.A. Tasca, unpubl. data, 2019), and it would have isolated TTX previously reported from two wild A. varius populations (Kim et al., Reference Kim, Kim and Yotsu-Yamashita2003) or 4-epi-TTX, which is usually found in nature to be present in smaller quantities than TTX. Other TTX or saxitoxin analogues (e.g. zetekitoxin AB), often found in Atelopus species and reviewed previously (Pearson & Tarvin, Reference Pearson and Tarvin2022), would not have been detected using this method.
We used a complementary targeted amplicon approach to determine the proportion of skin bacteria that could produce TTX on frogs in the mesocosms on pre-release and days 28 and 79. Skin bacteria have been collected and analysed previously (Kueneman et al., Reference Kueneman, Bletz, Becker, Gratwicke, Garcés and Hertz2022). We assembled a sequence file of 16S rRNA gene sequences of known TTX-producing bacteria from Vaelli et al. (Reference Vaelli, Theis, Williams, O'Connell, Foster and Eisthen2020), where the bacteria were cultured from the toxic rough-skinned newt Taricha granulosa. The T. granulosa and matching A. varius bacterial sequences are provided in Supplementary Materials 2 & 3.
Amphibian community dynamics
We conducted one diurnal and one nocturnal visual encounter survey along a 150 m stream transect in the release (i.e. transect 1) and control streams (Heyer et al., Reference Heyer, Donnelly, Foster and McDiarmid1994), 6–7 days prior to the release and, subsequently, at c. monthly intervals for 5 months. These surveys were additional to the ones focused only on Atelopus. Two trained personnel visually searched the stream and c. 2 m on either side for amphibians, one working on either stream bank. We captured all amphibians using a freshly gloved hand (or using a fresh plastic bag) and then swabbed them to detect Bd. We calculated the community species richness at each site over the six repeated diurnal and nocturnal samples during the 155-day monitoring period using the vegan package in R (Oksanen et al., Reference Oksanen, Blanchet, Friendly, Kindt, Legendre and McGlinn2019).
Detection of B. dendrobatidis
We swabbed the skin of individual frogs using a sterile MW113 rayon swab, with 60 passes total (i.e. 10 midventral, 10 on each flank, 10 on each thigh, 5 on the ventral side of each foot). We stored the swabs dry in cryovials that were frozen at −20 °C prior to DNA extraction and quantitative PCR analysis. The extraction of DNA from swabs followed Hyatt et al. (Reference Hyatt, Boyle, Olsen, Boyle, Berger and Obendorf2007). We performed the quantitative PCR analysis according to the protocol of Boyle et al. (Reference Boyle, Boyle, Olsen, Morgan and Hyatt2004) with some modifications, as indicated herein. We used 20 μl reactions (Kriger et al., Reference Kriger, Hines, Hyatt, Boyle and Hero2006b) in a Roche LightCycler 96 system. Each reaction contained 5 μl of sample and 15 μl of a master mix. The master mix contained 10 μl of Roche FastStart Essential DNA Probes Master, 0.25 μl of Roche LightCycler uracil-DNA glycosylase to avoid PCR carryover contamination, 0.8 μl of 18 μM ITS1-3 Chytr and 0.8 μl of 18 μM 5.8S Chytr primer solutions, 1 μl of 5 μM ChytrMGB2 probe solution, 2 μl of 10X Exo IPC mix and 0.4 μl of 50X Exo IPC DNA TaqMan Exogenous Internal Positive Control Reagents (Applied Biosystems No. 4308323) for uncovering false negatives from reaction inhibition (Hyatt et al., Reference Hyatt, Boyle, Olsen, Boyle, Berger and Obendorf2007). We tested the samples in triplicate, along with five Bd-negative and one or two Bd-positive controls on every plate (Boyle et al., Reference Boyle, Boyle, Olsen, Morgan and Hyatt2004; Kriger et al., Reference Kriger, Hero and Ashton2006a). We considered samples with two or three positive reactions to be Bd positive. We repeated a few samples to confirm these results. We quantified the infection load of Bd-positive samples based on the number of zoospore equivalents (Boyle et al., Reference Boyle, Boyle, Olsen, Morgan and Hyatt2004). We used tenfold serial dilutions of the standards that ranged from 0.1 to 10,000 zoospore equivalents of the JEL 423 Bd strain (originally isolated in Panama). We averaged the quantified values and multiplied them by 100 to compensate for the extraction and dilution of the samples.
Results
Tracking data
After release, we obtained 151 relocations of radiotracked animals, with a mean of 5 relocations and 8.3 days of tracking time per animal (Supplementary Material 4). Radiotracked frogs dispersed rapidly beyond the resurvey area, and by day 9 or 10 only c. 50% of radiotracked frogs were found inside the area associated with transects within the general release area (Table 1). Data were not recorded on days 4 and 5 because of heavy rainfall. All radiotracked frogs tested negative for Bd on the last day of sampling prior to removal of the transmitter. Females dispersed further than males (Wilcoxon rank sum test W = 49, P = 0.013), 62% of females (5/8) dispersed > 50 m in the first 11 or 12 days following release and two of these females dispersed > 100 m. Only 14% of males (1/7) dispersed > 50 m in that period (Table 2, Fig. 1). The mean dispersal distances of females (n = 8) and males (n = 7) were 65.0 and 22.7 m, respectively (Table 2). Of 30 tracked frogs, we recovered only half at the end of the first 10 days. We recovered one detached transmitter on day 1. We could not detect five frogs after day 2, and we last saw seven frogs on days 6–8. We traced one of the frogs that disappeared on day 8 to a Savage's thin-toed frog Leptodactylus savagei hole and so it could have been predated. One frog had a malfunctioning transmitter but was incidentally encountered on days 3 and 7.
Table 1 Number and per cent of radiotracked variable harlequin frogs Atelopus varius found inside the mark–recapture survey area in Panama (Fig. 1) after release day.

Table 2 Dispersal distance of 15 variable harlequin frogs calculated between the site of release and the last recapture on day 11 or 12 (Fig. 2). The median dispersal distances of females (n = 8) and males (n = 7) were 55.5 and 14.0 m, respectively. Females dispersed further than males (Wilcoxon rank-sum test W = 49, P = 0.013). All frogs tested negative for Batrachochytrium dendrobatidis on the last recapture.


Fig. 1 The release site in Panama, showing the paths of 15 radiotracked variable harlequin frogs Atelopus varius (7 males, 8 females) within the first 11 or 12 days of release (Table 2). Frogs dispersed rapidly beyond the mark–recapture area (colours indicate individuals). Females dispersed a mean of 65 m, and males a mean of 23 m.
Mark–recapture
We recaptured 78 unique individuals of the 428 non-radiotracked frogs that we released (Table 3), the last of which we saw 36 days following release. We had 35 recaptures on day 1, which decreased to 23 recaptures on day 2 and 25 recaptures on day 3. Taken together with the rapid dispersal of frogs out of the radiotracking study area (Table 1), it appears that the assumption of a closed population for the first recapture period was not satisfied. The closed population estimate for that period was 133 animals (M0 = 133.6 ± SE 24.5, Akaike information criterion = 36.50) or 30% of the known number of released animals (Table 3). We did not record sufficient numbers of recaptures in subsequent recapture periods to implement the planned robust recapture design (Table 3). Two of 13 of the frogs recaptured on day 10 tested positive for Bd, and we detected no frogs on days 11 or 12 (Supplementary Material 5), possibly because of heavy rain and reduced detectability. We are uncertain as to the fate of these individuals as we were unable to observe them. Anecdotally, we observed courtship behaviour amongst untracked animals, with male frogs calling and fighting from rocks in the stream, and on day 7 we observed one male amplexing a female (Plate 2). We also observed that following release some individuals became a darker green colour, probably reducing their detectability.

Plate 2 Male (top) and female (bottom) amplectant pair of A. varius observed following male–male aggressive interactions with an undepicted male on day 7 following release.
Table 3 Variable harlequin frog recaptures following the release day of 17 January 2018 (i.e. day 0) when we released 428 individuals for the mark–recapture study. We resurveyed all transects on three concurrent days in each recapture period. In recapture period 2 it is probable that heavy rains on days 11 and 12 affected the detectability of the frogs and we did not attempt a population estimate.

Skin toxicity
We could not detect TTX on any of the captive-bred A. varius prior to release (n = 5). Frogs did not appear to regain toxicity in mesocosms, as all animals euthanized at day 27 (n = 5) and at day 79 (n = 7) also tested negative for TTX. A targeted amplicon sequencing approach detected bacteria on the skin matching known TTX producers described previously (Vaelli et al., Reference Vaelli, Theis, Williams, O'Connell, Foster and Eisthen2020). The proportion of reads was < 10% of the bacterial community and increased substantially with time from < 1% in captivity to c. 8% in the outdoor mesocosms. The sequences from A. varius that matched with TTX producers are described in Supplementary Material 3 and included four genera: Pseudomonas, Aeromonas, Shewanella and Sphingopyxis. By comparison, the proportion of skin bacteria predicted to contribute to anti-Bd defence function increased from c. 40–60% over the same period in the mesocosms (Fig. 2; based on microbiome sequencing reported in Kueneman et al., Reference Kueneman, Bletz, Becker, Gratwicke, Garcés and Hertz2022).

Fig. 2 Per cent of the bacterial community (mean reads ± SE) thought to (a) produce tetrodotoxin (TTX) or (b) inhibit growth of Batrachochytrium dendrobatidis (Bd) on the skin of A. varius held in outdoor mesocosms based on targeted amplicon sequencing described in Kueneman et al. (Reference Kueneman, Bletz, Becker, Gratwicke, Garcés and Hertz2022).
Amphibian community dynamics
During the amphibian community surveys, 83.7 person-hours resulted in 28 species and 230 individuals being found at the two sites. The Chao 1 species richness estimates were similar for both sites: 24.6 ± SE 2.2 for the release site and 23.6 ± SE 2.2 for the control site. The number of frogs and number of species encountered varied over the survey period. The pattern of change in both community metrics was similar at both the release and control sites (Fig. 3), indicating that the cause of the observed changes occurred at both sites and was unlikely to be a result of the release of Atelopus. Bd prevalence was variable, probably because of the low total numbers of frogs swabbed on each sampling occasion, but Bd prevalence was highest at both sites immediately preceding release, exceeding 40% at both sites then declining at both sites, which had a similar mean Bd prevalence of c. 23% (Table 4, Fig. 3). The observed high Bd prevalence at the time of release was probably associated with weather conditions. On the first sampling occasion immediately preceding release, we observed two dead frogs (Craugastor talamancae and Pristimantis sp.) with high Bd loads exceeding 1 million zoospore equivalents (Supplementary Material 6). Approximately 3–18% of the frogs we encountered in some months (January, February and May) had high Bd loads exceeding 100,000 zoospore equivalents (Supplementary Material 6), including a released individual of A. varius.

Fig. 3 Ten-day rainfall totals, number of species observed, total number of all A. varius counted and Batrachochytrium dendrobatidis (Bd) prevalence in frog communities (Table 4) on 150-m long stream transects along the release and control streams that were surveyed both diurnally and nocturnally at regular intervals before and after the release day of 17 January 2018.
Table 4 Summary of community survey data at the release and control sites ranked by total number of variable harlequin frogs sampled. Binomial 95% CIs are shown for species for which we recorded > 10 individuals. Chao 1 species richness estimates for the release site and control site were similar: 24.6 ± SE 2.2 and 23.6 ± SE 2.2, respectively. Bold indicates species where at least one individual had a Batrachochytrium dendrobatidis (Bd) load > 100,000 zoospore equivalents (Fig. 3). Full data are in Supplementary Material 6.

Discussion
The goal of this study was to understand how captive-bred A. varius transition into a wild situation. Frogs did not regain any detectable skin toxicity in the first 2.5 months post-release. We found that frogs dispersed rapidly and were difficult to re-encounter unless they had a working radio transmitter or were housed in a mesocosm. The low re-encounter rates prevented us from gaining insight into the fate of these animals. Some released animals rapidly contracted Bd, but within the wild frog community Bd prevalence rates at the release site and a control site were similar during the post-release observation period. We planned our release trial around the early dry season in Panama, but unseasonable continuous heavy rain negatively affected our work. Heavy rain on some census days prevented us from conducting radiotracking and probably reduced the detectability of frogs during visual encounter surveys. During a subsequent visit to the release site c. 1 year later (3 July 2019), we recorded the presence of three frog species but did not observe individuals of A. varius along the three surveyed transects or in surrounding areas.
The radiotracking method offered a labour-intensive way to track a limited number of frogs (≤ 30) for a short duration. Nonetheless, 50% of the radiotracked animals disappeared in just 10 days, possibly due to transmitter failure, predation or dispersal beyond the area of detectability. This method was suitable for mapping dispersal over longer distances. We were able to discern sex-based dispersal differences, but the GPS location, with an error of 7 m ± SD 3 m, was not sufficiently precise to measure finer-scale movements and territory establishment within 10 m, which would have required an intensive manual quadrat survey grid (Crump & Pounds, Reference Crump and Pounds1989) or a high-resolution forest mapping approach (Ringler et al., Reference Ringler, Mangione, Pašukonis, Rainer, Gyimesi and Felling2016). Male A. varius and A. zeteki are usually encountered in higher densities along streams (Crump, Reference Crump1986; McCaffery et al., Reference McCaffery, Richards-Zawacki and Lips2015). Males are more territorial and defend their territories through calls, semaphoring behaviour and aggressive interactions (Lindquist & Hetherington, Reference Lindquist and Hetherington1996). It is probable that males are simply more detectable than females, but it is also possible there is a sex-ratio skew in the population (Crump, Reference Crump1988; Crump & Pounds, Reference Crump and Pounds1989); however, our observations suggest that the females are less tied to stream banks and could disperse further.
We released many animals for the mark–recapture study: the equivalent of 285 frogs per 100 m stream segment. This number is large even for pre-Bd Atelopus populations. One A. varius site using mark–recapture documented 5–16 individuals/100 m, and another A. zeteki site found 34–75 individuals/100 m (McCaffery et al., Reference McCaffery, Richards-Zawacki and Lips2015). We released frogs at much higher densities, hoping that even with significant dispersal outside the core release area, a sufficiently high density would remain in the core recapture transects to conduct effective mark–recapture evaluations of the population. However, our transect search-based mark–recapture population estimate methods were not sufficient to recapture frogs even with the large number of animals released. Both the dispersal area and density of frogs within the release area changed rapidly (Fig. 1). It is possible that the detectability of the frogs changed as some brightly coloured animals assumed an olive colouration post-release. As frogs settled into their new environment, they probably found hiding spots that further reduced their detectability, which could only be overcome with the aid of a radio transmitter (O. Garcés & E. Lassiter, pers. obs., 2018). Even when contained in mesocosms, these animals were not conspicuous and required considerable effort to find (O. Garcés & E. Lassiter, pers. obs., 2018). A more appropriate recapture methodology would have involved a visual encounter survey design that accommodated a more intensive search method (such as zigzag searches between numbered stakes or intensive quadrat sampling; Heyer et al., Reference Heyer, Donnelly, Foster and McDiarmid1994), encompassing a much larger projected dispersal area. Another alternative would have been developing a recapture survey method that quantifies search effort and accommodates a changing dispersal area determined by radiotracking a subset of frogs. Regardless, recapturing individuals is likely to remain the greatest limiting factor for monitoring future release trials of Atelopus.
One of the problems with releasing an aposematically coloured animal without chemical defences back into the wild is likely to be predation. We did not observe direct predation on the frogs, but it can be inferred from the anecdotal observation of a frog disappearing into a burrow containing a large L. savagei and from the rapid disappearance of 50% of the radio transmitters that frogs could have been captured by predators. Captive-raised Atelopus lack TTX (Daly et al., Reference Daly, Padgett, Saunders and Cover1997; present study), and it is currently unclear how TTX is acquired, although it is postulated to be derived from the frogs' diet or from symbiotic bacteria (Yotsu-Yamashita & Tateki, Reference Yotsu-Yamashita and Tateki2010). The absence of previous information on the skin toxins of wild A. varius from the study area precludes comparisons of the natural levels of TTX present in their skin. Studies of Californian newts have shown they can demonstrate TTX increases in captivity (Hanifin et al., Reference Hanifin, Brodie and Brodie2002) and experience rapidly inducible changes in TTX defences over short periods (Bucciarelli et al., Reference Bucciarelli, Shaffer, Green and Kats2017), and that TTX could be bacterially derived (Vaelli et al., Reference Vaelli, Theis, Williams, O'Connell, Foster and Eisthen2020). Similarly, it was shown previously that association with toxic parents was necessary to induce detectable TTX in Chinese red-bellied newts Cynops orientalis (Mebs & Yotsu-Yamashita, Reference Mebs and Yotsu-Yamashita2021). Captive-bred Atelopus appear to rapidly regain their wild microbiome when held in wild environments (Estrada et al., Reference Estrada, Medina, Gratwicke, Ibáñez and Belden2022; Kueneman et al., Reference Kueneman, Bletz, Becker, Gratwicke, Garcés and Hertz2022), but after 79 days there was no detectable TTX in the skin of our study animals, thus indicating the value of research into artificial restoration of skin defences prior to release, with dietary provisioning (Kudo et al., Reference Kudo, Chiba, Konoki, Cho and Yotsu-Yamashita2017) or probiotics. Although putative TTX-producing bacteria were detected at high abundances (up to 8% of reads at day 79) in the mesocosms compared to the 1–2% of reads from the same bacteria detected in toxic rough-skinned newts T. granulosa (Vaelli et al., Reference Vaelli, Theis, Williams, O'Connell, Foster and Eisthen2020), our results indicate that different strains of bacteria or additional factors might be needed to induce toxicity.
The threat of Bd in Panama has not been mitigated, but there is ongoing research into the evolution of anti-Bd skin secretions and exploration of genetic rescue possibilities (Voyles et al., Reference Voyles, Woodhams, Saenz, Byrne, Perez and Rios-Sotelo2018; Byrne et al., Reference Byrne, Richards-Zawacki, Voyles, Bi, Ibáñez and Rosenblum2021). Rather than setting explicit recovery goals, the aims of this release trial were focused on comprehending the other threats faced by Atelopus frogs as they transition back into the wild and on understanding Bd dynamics in the released frogs and the community. Our expectation, based on laboratory studies of A. zeteki, was that Bd-related mortality would not begin until c. 2–4 months after exposure (Becker et al., Reference Becker, Harris, Minbiole, Schwantes, Rollins-Smith and Reinert2011, Reference Becker, Walke, Cikanek, Savage, Mattheus and Santiago2015, Reference Becker, Brophy, Barrett, Bronikowski, Evans and Glassey2021; Langhammer et al., Reference Langhammer, Lips, Burrowes, Tunstall, Palmer and Collins2013), but this is highly variable depending on Bd load, temperature and hydric environment (Bustamante et al., Reference Bustamante, Livo and Carey2010). The released frogs mostly disappeared before we could make any observations, but Bd prevalence in the surrounding frog community was high, probably because of the weather conditions (wet and cool), with two dead Bd-positive non-Atelopus frogs observed, and 2/13 of the released A. varius were Bd positive by day 10. This was an unseasonably rainy period despite us planning for the release to occur in the dry season. The month of January 2018 received 1,167 mm of rain in this area (Fig. 3), whereas rainfall amounts were more characteristic in the years before and after the trial, with 89 mm in January 2017 and 54 mm in January 2019 (Empresa de Transmisión Eléctrica, S.A. rainfall data, Station 105003, Coclé del Norte, Panama).
One of the concerns about releasing highly susceptible frogs into the environment is that they could develop high Bd loads and become supershedders, which could be detrimental to the persisting frog community as a result of them increasing the environmental disease load (DiRenzo et al., Reference DiRenzo, Langhammer, Zamudio and Lips2014). However, a follow-up experimental study did not validate the supershedder hypothesis (DiRenzo et al., Reference DiRenzo, Tunstall, Ibáñez, deVries, Longo, Zamudio and Lips2018). When comparing our control stream and release stream, we observed that disease loads prior to release were high and declined over time in a pattern probably driven by climatic effects. However, we did not observe any differences in Bd prevalence at the two streams. Any statistical comparisons will require replication, and we present our data (Supplementary Materials 6) for future meta-analysis.
It is evident that surplus individuals from captive collections of threatened amphibians afford numerous research opportunities to enable us to understand the threats they face and to study these animals ex situ and in situ (Lewis et al., Reference Lewis, Richards-Zawacki, Ibáñez, Luedtke, Voyles, Houser and Gratwicke2019). This study revealed that we will need to refine our methods and approaches to study captive amphibians released into the wild. Although the use of mesocosms has proven to be a useful and efficient method for conducting experiments and monitoring Atelopus individuals within their natural habitats (Estrada et al., Reference Estrada, Medina, Gratwicke, Ibáñez and Belden2022; Klocke et al., Reference Klocke, Estrada, Mataya, Medina, Baitchman and Belden2023; this study), our study also showed that simply increasing the numbers of frogs released into the wild is not sufficient to ensure repeated observations of individuals using visual encounter survey methods. This release trial demonstrated that even if we had a species of frog that shows resilience to the amphibian chytrid fungus in captive settings, we do not have satisfactory tools to perform adequate post-release monitoring in the wild. Radiotracking Atelopus individuals is an expensive and labour-intensive method that has potential for use (Klocke et al., Reference Klocke, Estrada, Mataya, Medina, Baitchman and Belden2023; this study), but it requires further testing and improvement. Moreover, we lack a good understanding of the other potential threats facing amphibians previously acclimated to captive conditions. We recommend continued release trials of captive-bred frogs with intensive post-release monitoring methods using an adaptive management framework to advance the nascent field of amphibian reintroduction ecology. Given that 62 of 94 Atelopus species are considered to require ex situ conservation measures for species management (IUCN, 2022) and that the action plans of regional conservation initiatives place captive survival insurance colonies for future reintroduction amongst their conservation priorities (Valencia & Fonte, Reference Valencia and Fonte2021, Reference Valencia and Fonte2022), we anticipate that our findings will be useful for other ex situ conservation efforts for Atelopus species.
Acknowledgements
We thank Minera Panama, a subsidiary of First Quantum Minerals, for financial and logistical support and for permission to conduct this research on their land concession, and Blanca Araúz for facilitating this work. The Panama Amphibian Rescue and Conservation Project is a collaborative project between the Cheyenne Mountain Zoo, the Houston Zoo, Zoo New England and the Smithsonian Institution, who collectively provided core operational support for this project, and the laboratory facility holding the animals was established by the National Science Foundation Field Stations and Marine Laboratories Improvement Grant # 1227005. We thank the National Geographic Society, the Mohammed Bin Zayed Species Conservation Fund, the Smithsonian Women's Committee and the Secretaría Nacional de Ciencia, Tecnología e Innovación for grants supporting this research. AH was financially supported by a German Science Foundation (DFG) research grant (HE 7562/1-1) and a Smithsonian Institution Postdoctoral Fellowship. DCW was supported by the US National Science Foundation (IOS-1845634 and BII 2120084). We thank Milagro González, Yeisson Muñoz, Nair Cabezón, Lanki Cheucarama, Rigoberto Díaz and Nancy Fairchild for their work on captive husbandry and their care of the animals; Angie Estrada and Daniel Medina for lending us the mesocosms designed by them; and Juan P. Ríos for his assistance in the field. We thank the Empresa de Transmisión Eléctrica, S.A. for providing rainfall data from the Red Hidrometeorológica Nacional.
Author contributions
Study design, grant writing: BK, BG, RI; captive animal care, breeding: JG, HR; fieldwork: BK, OG, EL, JG, AH, EK, HR, BG, RI; laboratory analysis: EI, KM, JAT, DGW; data analysis: BK, DGW, BG, RI; writing: BK, LL, DGW, BG, RI; supervision: BG, RI; revision: all authors.
Conflicts of interest
The funders acknowledged above had no role in the study design, data collection and analysis, decision to publish or preparation of the manuscript.
Ethical standards
This study abided by the Oryx guidelines on ethical standards. We obtained the approval 2016-0311-2019-A10 from the Smithsonian Tropical Research Institute Animal Care and Use Committee and the scientific permits SE/APB-1-17 and SE/APH-1-18 from the Panamanian Ministerio de Ambiente to conduct this study.
Data availability
The data supporting the findings of this study are available within the supplementary materials.