Introduction
Phenolic compounds are widely distributed throughout the plant kingdom, and several hundred are found in edible plants. Phenolic-rich foods have been consistently identified in epidemiological studies as the key components of dietary patterns that reduce the risk of developing chronic diseases, including type 2 diabetes and many cancers( Reference Hu, Rimm and Stampfer 1 – Reference McGinnis and Foege 3 ). However, the adequate bioavailability of phenolic compounds is a prerequisite for the compounds' health benefits( Reference Amarowicz, Carle and Dongowski 4 ). In the human diet, phenolic-rich foods, such as tea and coffee, are usually consumed along with food macronutrients, such as proteins, fats and carbohydrates. For example, the addition of milk to black tea has a long tradition in the Western world, especially in the UK. The co-administered dietary components may have an impact on the bioavailability of phenolic compounds. Most studies have concentrated on the in vitro interactions between polyphenols and proteins. After the pioneering work of Haslam and colleagues( Reference Haslam 5 , Reference Halsam and Lilley 6 ), the interaction of polyphenols with proteins has become a well-studied topic. It has been reported that polyphenol binding to salivary proteins leads to the precipitation of insoluble complexes, causing the perception of an astringent flavour( Reference Baxter, Lilley and Haslam 7 ). Polyphenols are also thought to interact with dietary proteins, plasma proteins and digestive enzymes in the gut( Reference Brunet, Blade and Salvado 8 ). However, the biological fate of protein–polyphenol complexes in vivo is unclear. Recently, we showed that milk protein–polyphenol complexes lead to significant changes in the plasma kinetics profile but do not affect the absorption and bioactivity of polyphenols both in rats and in human subjects( Reference Zhang, Zheng and Liu 9 , Reference Zhang, Jiang and Guo 10 ). It was also found that milk fats can participate in the interactions between proteins and polyphenols during digestion, resulting in remarkable aggregation and thus significantly inhibiting the bioavailability of polyphenols( Reference Zhang, Zheng and Liu 9 , Reference Zhang, Jiang and Guo 10 ). The results suggested that not only a nutrient alone but also certain synergisms between food macronutrients can significantly affect the bioavailability and biological activity of polyphenols. In addition, other researchers have reported that co-consumed carbohydrates can affect the bioavailability of polyphenols( Reference Peters, Green and Janle 11 ). These findings represent novel contributions to the literature and are important for a better understanding of the effects of the interactions of plant phenols with food macronutrients.
Therefore, in the present review, we focused on both the in vitro and the in vivo effects of food macronutrients on the bioavailability and bioactivity of polyphenols. The mechanisms of interactions between polyphenols and food macronutrients are also discussed. A better understanding of these factors is essential to the development of formulation strategies specifically designed to optimise the health benefits of polyphenols.
Nature, intake and health benefits of polyphenols
Nature of dietary phenols
To understand the relationship between the intake of polyphenols and a reduced risk of developing diseases, it is essential to know the nature and distribution of phenolic compounds in our diet. Phenolic compounds can be classified into different groups according to the number of phenol rings in the molecule and the substitution groups of the phenol rings. Distinctions are thus made between phenolic acids, flavonoids, stilbenes and lignans( Reference Manach, Scalbert and Morand 12 ). Among these compounds, flavonoids and phenolic acids are the most abundant polyphenols in the human diet( Reference RiceEvans, Miller and Paganga 13 – Reference Perez-Jimenez, Neveu and Vos 17 ). Attention should also be paid to proanthocyanidins which are believed to be ubiquitous in plant foods. Proanthocyanidins are polyhydroxyflavan oligomers or polymers. The most common monomers are the diastereomers (+)-catechin/( − )-epicatechin, ( − )-gallocatechin/( − )-epigallocatechin and (+)-afzelechin/( − )-epiafzelechin, and the respective oligo- and polymers are called procyanidins, prodelphinidins and propelargonidins. It has been suggested that they account for a significant fraction of the polyphenols ingested in a Western diet( Reference Serrano, Puupponen-Pimiä and Dauer 18 ). Phenolic compounds are secondary metabolites of plants and afford protection against UV radiation, pathogens and herbivores( Reference Harborne and Williams 19 ). The anthocyanin co-pigments in flowers attract pollinating insects( Reference Harborne and Williams 19 ) and are responsible for the characteristic red and blue colours of berries, wines and certain vegetables( Reference Hammerstone, Lazarus and Schmitz 20 – Reference Reinli and Block 26 ). In addition, polyphenols contribute to the organoleptic properties of plant foods, especially by their astringency, bitter taste and participation in haze formation( Reference Le Bourvellec and Renard 27 ). To play these roles, most polyphenols are concentrated in the peels of many fruits and vegetables. It has been shown that for the jujube (Ziziphus jujuba Mill.), a traditional Chinese fruit, the peel has the highest content of total polyphenols( Reference Zhang, Jiang and Ye 28 ). The results are consistent with the data reported by Tomás-Barberán et al. ( Reference Tomás-Barberán, Gil and Cremin 29 ), who found that the peel tissues of nectarines, peaches and plums contained two-fold more flavonoids than other tissues.
Content of polyphenols in food and their absorption after intake
In the human diet, coffee, tea and red wine are major sources of polyphenols because of these liquids' high polyphenol content and relatively large serving sizes. The polyphenol content of main food sources is shown in Table 1 ( Reference Astill, Birch and Dacombe 30 – Reference Rivero, Perez-Magarino and Gonzalez-Sanjose 34 ). Considering the amounts of total phenols in our diet, it has been reported that a well-balanced diet can provide well over 1000 mg of total phenols per d.
Table 1 Main sources of polyphenols in the human diet
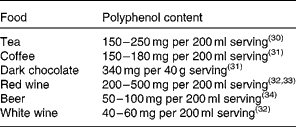
After the intake of polyphenols, aglycones can be absorbed from the small intestine, and the maximal plasma concentrations often reach 1–2 h after ingestion( Reference Aziz, Edwards and Lean 35 – Reference Kivits, van der Sman and Tijburg 37 ). However, most polyphenols in food are in the form of esters, glycosides or polymers that cannot be absorbed in the native form. These substances must be hydrolysed by intestinal enzymes before the substances can be absorbed( Reference Manach, Scalbert and Morand 12 ). In the case of quercetin glucosides, it has been suggested that these compounds could be hydrolysed inside cells by a cytosolic β-glucosidase( Reference Day, DuPont and Ridley 38 ). Another pathway involves lactase phloridzine hydrolase, a glucosidase of the brush-border membrane of the small intestine that catalyses the extracellular hydrolysis of certain glucosides, which is followed by the diffusion of aglycones across the brush border( Reference Day, Cañada and Díaz 39 ). Certain polyphenols with special structures cannot be hydrolysed by intestinal enzymes and thus cannot be absorbed until reaching the colon. Unlike intestinal enzymes, colonic microflora catalyse the breakdown of polyphenols into more simple compounds, such as phenolic acids. For example, when quercetin-3-O-rhamnoside was incubated anaerobically with human intestinal bacteria, quercetin, 3,4-dihydroxyphenylacetic acid and 4-hydroxybenzoic acid were found to be metabolites( Reference Scalbert and Williamson 31 ). Proanthocyanidins are a subclass of polyphenols. Since they are associated with the cell wall in plants by non-covalent or covalent bindings, it is believed that they undergo two main processes in the intestinal tract after intake: (1) partial depolymerisation into their constituent units( Reference Spencer, Chaudry and Pannala 40 ), mainly by the gastric milieu coupled with absorption of the free monomers and small oligomers (dimers, trimers) in the small intestine( Reference Mateos-Martín, Pérez-Jiménez and Fuguet 41 ); and (2) direct fermentation by the intestinal microbiota into smaller metabolites such as phenolic acids and their subsequent absorption( Reference Deprez, Brezillon and Rabot 42 , Reference Rios, Gonthier and Rémésy 43 ). It has been suggested that the chain length of proanthocyanidins has a more crucial role in their microbial metabolism than the dose. Short-chain proanthocyanidins exhibited a strong metabolite formation and a higher concentration of proanthocyanidins could be tolerated without inhibition of metabolite formation( Reference Bazzocco, Mattila and Guyot 44 ). On the other hand, proanthocyanidins with high polymerisation were able to inhibit the conversions by the intestinal microbiota( Reference Aura, Mattila and Hyötyläinen 45 ). The total yields of metabolites decrease significantly with increasing polymerisation( Reference Rios, Gonthier and Rémésy 43 , Reference Bazzocco, Mattila and Guyot 44 , Reference Gonthier, Donovan and Texier 46 ). It is possible for proanthocyanidins to pass through the entire gastrointestinal digestion largely intact, especially if their molecular weight is large( Reference Serrano, Puupponen-Pimiä and Dauer 18 ). Thus, there can be some intact parent compounds( Reference Choy, Jaggers and Oteiza 47 ) and non-absorbable metabolites (probably mid-molecular-weight tannins)( Reference Serrano, Puupponen-Pimiä and Dauer 18 ) after the metabolism of proanthocyanidins that remain in the colonic lumen where they may contribute to the health of the gastrointestinal tract.
After the hydrolysis of polyphenols into free aglycones and before passage into the bloodstream, polyphenols are conjugated by methylation, sulfation or glucuronidation, or a combination( Reference Walle 48 , Reference Karakaya 49 ). This conjugation is a metabolic detoxification process common to many xenobiotics. The conjugation is particularly important for increasing the molecular weight and solubility of phenolic aglycones, which reduces compounds' potential toxic effects and enhances their elimination ability( Reference Day, Bao and Morgan 50 ). The relative contribution of different types of conjugation (methylation, sulfation and glucuronidation) appears to vary according to the nature of the substrate and the dose ingested. Sulfation is generally a higher-affinity, lower-capacity pathway than glucuronidation, so that when the ingested dose increases, a shift from sulfation toward glucuronidation occurs( Reference Koster, Halsema and Scholtens 51 , Reference Piskula 52 ). The balance between the sulfation and the glucuronidation of polyphenols also seems to vary with species, sex and food deprivation( Reference Piskula 52 ).
Health benefits of polyphenols
Antioxidant activity
Polyphenols are strong antioxidants in vitro, mainly due to their low redox potential and capacity to donate electrons or hydrogen atoms( Reference Bors and Saran 53 , Reference Bors, Michel and Stettmaier 54 ). There is ample evidence that the addition of polyphenols or polyphenol-rich food extracts to human plasma or lipoproteins effectively protects endogenous lipids and proteins from oxidation. For example, apple extracts effectively delay the oxidation of α-tocopherol and the formation of lipid hydroperoxides in human plasma( Reference Lotito and Frei 55 ). In addition, colonic metabolites of chlorogenic acids such as m-coumaric acid and dihydroferulic acid showed high antioxidant activity in a study carried out by Gómez-Ruiz et al. ( Reference Gómez-Ruiz, Leake and Ames 56 ). Another study showed that catechins, procyanidins and procyanidin-rich extracts protect plasma components from oxidation( Reference Shafiee, Carbonneau and Urban 57 ).
In vivo, several studies have shown that phenolic compounds can increase the antioxidant capacity of the plasma acutely and after long-term consumption( Reference Crozier, Jaganath and Clifford 58 – Reference Prior, Go and Wu 60 ). This phenomenon has been observed for a wide array of foodstuffs, such as tea( Reference Serafini, Ghiselli and Ferro-Luzzi 61 , Reference van het Hof, de Boer and Wiseman 62 ), red wine( Reference Duthie, Pedersen and Gardner 63 , Reference Serafini, Maiani and Ferro-Luzzi 64 ) and apple juice( Reference Young, Nielsen and Haraldsdottir 65 ). The maximal increase produced by juices is approximately 30 % in the case of elderberry juice, which has a high phenolic content. An increase of 18 % in plasma antioxidant activity was observed after an intake of 100 g dark chocolate( Reference Serafini, Bugianesi and Maiani 66 ). Due to increased plasma antioxidant activity, the consumption of polyphenols with a high-fat meal has been shown to decrease plasma lipid hydroperoxides. Natella et al. ( Reference Natella, Belelli and Gentili 67 ) showed that in eight healthy men who consumed a high-fat meal or meal of potatoes with (active) or without (placebo) 300 mg polyphenols, plasma lipid hydroperoxides were 1·5-fold higher in the placebo meal than in the polyphenol-active meal. Similarly, Nakagawa et al. ( Reference Nakagawa, Ninomiya and Okubo 68 ) reported a reduction in plasma hydroperoxide levels in healthy subjects after acute ingestion of tea extract, equivalent to two cups of tea, and Miura et al. ( Reference Miura, Chiba and Miura 69 ) observed a decreased oxidisability of LDL obtained from subjects who had ingested green tea extract daily for 1 week. In addition, plasma obtained from rats fed quercetin was more resistant to copper sulfate-induced lipid peroxidation than the control plasma on the basis of the accumulation of hydroperoxides and the degradation of α-tocopherol. Based on the fact that quercetin is recovered in rat blood plasma principally as sulfate and glucuronide conjugates, these observations indicate that at least some metabolites of quercetin retain the ability to act as effective antioxidants( Reference da Silva, Piskula and Yamamoto 70 ).
Anticarcinogenic activity
It has been shown that phenylacetic acids possess anticarcinogenic activities( Reference Samid, Hudgins, Shack, Honn, Nigam and Marnett 71 , Reference Thibout, Kraemer and di Benedetto 72 ). After the ingestion of polyphenols, the concentration in the gut should be much higher than in the plasma. For example, the dilution of 500 mg polyphenols with a digestive bolus in the colon would yield a local concentration of 3 mmol/l. Such a high local concentration in the colon may contribute to anticarcinogenic effects( Reference Scalbert and Williamson 31 ). In addition, evidence exists for an inverse relationship between a moderate intake of red wine and the incidence of rectal cancer( Reference Pedersen, Johansen and Gronbaek 73 ), and this could be attributed to the colonic metabolites of malvidin-3-O-glucoside and other anthocyanins, which along with procyanidins are major components in many red wines. Protocatechuic acid is one of the catabolites derived from colonic microbial degradation of cyanidin-O-glucosides( Reference González-Barrio, Edwards and Crozier 74 , Reference Vitaglione, Donnarumma and Napolitano 75 ), and it has apoptotic effects on human gastric adenocarcinoma cells in a time- and dose-dependent manner( Reference Lin, Chen and Huang 76 ). Forester & Waterhouse( Reference Forester and Waterhouse 77 ) found the anthocyanin microbial catabolites gallic acid, 3-O-methyl-gallic acid, vanillic acid, syringic acid and 2,4,6-trihydroxybenzaldehyde have the ability to induce apoptosis and inhibit cell proliferation in the human colon cancer cell. The effects of quercetin metabolites on lung cancer cells have also been investigated. Yang et al. ( Reference Yang, Hsia and Kuo 78 ) demonstrated that quercetin glucuronides were able to inhibit proliferation and induce apoptosis via the caspase-3 cascade in the human lung cancer cell.
Other bioactivities
In addition to their antioxidant and anticarcinogenic properties, polyphenols have several other biological actions. For instance, polyphenols modulate the activity of a wide range of enzymes and cell receptors( Reference Middleton, Kandaswami and Theoharides 79 , Reference Stoclet, Chataigneau and Ndiaye 80 ) and also interfere with the activity and expression of several cell membrane transporters( Reference Martel, Monteiro and Calhau 81 ). In addition, certain flavonoids could act as cytochrome-P450 inhibitors and enhance drug bioavailability. Increased plasma concentrations of many drugs have been shown following co-administration with grapefruit juice( Reference Fuhr 82 ). Certain polyphenols, such as quercetin, are also efficient inhibitors of sulfotransferases( Reference Eaton, Walle and Lewis 83 – Reference Marchetti, De Santi and Vietri 86 ) and thus may have an effect on the function of thyroid hormones, steroids and catecholamines( Reference Coughtrie, Sharp and Maxwell 87 ). Platelet activation and subsequent aggregation play a major role in the pathogenesis of thromboembolic diseases such as myocardial infarction and IHD. Hyperactive platelets with a high predisposition for activation are known to be apparent under conditions such as diabetes and heart disease( Reference Hirsh 88 ). Dihydroferulic and chlorogenic acids partly reversed hyper-reactivity of platelets induced by oxidative stress( Reference Rechner, Smith and Kuhnle 89 , Reference Stalmach, Mullen and Barron 90 ), and could also counteract the negative effects of hormonal stress-induced platelet hyper-reactivity( Reference Rechner and Kroner 91 ). Moreover, certain microbial metabolites of polyphenols, such as 3,4-dihydroxyphenylacetic acid and 4-hydroxyphenylacetic acid, have been suggested to inhibit platelet aggregation( Reference Kim, Jung and Sohng 92 ). From the inflammation perspective, protocatechuic acid has been reported to inhibit monocyte adhesion to TNF-α-activated mouse aortic endothelial cells, and also to reduce intercellular adhesion molecule 1 expression and the nuclear content of p65( Reference Wang, Wei and Yan 93 ). Modulation by quercetin metabolites of endothelial function, which has a strong connection with inflammation, has also been investigated. All quercetin metabolites partially prevented the impairment of the endothelial-derived NO response under conditions of high oxidative stress induced by diethyldithiocarbamic acid, a superoxide dismutase inhibitor, and protected the biological activity of exogenous NO( Reference Del Rio, Rodriguez-Mateos and Spencer 94 ).
Interaction of plant phenols with food macronutrients: in vitro evidence
Interaction of plant phenols with proteins
The interaction of polyphenols with proteins is an old but continuous research topic. Principally, two types of interactions can be classified between phenolic compounds and proteins: covalent and non-covalent binding( Reference Kroll, Rawel and Rohn 95 , Reference Harbourne, Jacquier and O'Riordan 96 ). The formation of covalent bonds between proteins and polyphenols is an irreversible reaction( Reference Sastry and Rao 97 ), involving the oxidation of phenolic compounds and the formation of o-quinones or o-semi-quinones( Reference Kalyanaraman, Felix and Sealy 98 – Reference Nicolas, Richard-Forget and Goupy 100 ) or the cleavage of proanthocyanidin interflavanic bonds in an acid medium with the formation of carbocations( Reference Beart, Lilley and Haslam 101 ). Physiologically and technologically relevant data from the literature suggest that the majority of protein–polyphenol interactions may occur by non-covalent binding( Reference Rawel, Meidtner and Kroll 102 ), although both covalent and non-covalent binding are likely to occur simultaneously, as shown for the binding of chlorogenic acid to proteins( Reference Prigent, Gruppen and Visser 103 ). Three types of non-covalent interactions have been suggested: hydrogen, hydrophobic and ionic bonding( Reference Rawel and Rohn 104 ).
Plasma proteins are the most studied binding targets, especially albumin. Albumin is the primary protein that interacts with polyphenols in the blood. The incubation of quercetin with human plasma revealed that quercetin extensively binds to plasma proteins( Reference Boulton, Walle and Walle 105 ). Smith et al. ( Reference Smith, Halliwell and Aruoma 106 ) found that the binding of polyphenols, such as quercetin, myricetin and morin, to albumin reduces polyphenols' antioxidant activity. Similarly, Rohn et al. ( Reference Rohn, Rawel and Kroll 107 ) showed that the quercetin–albumin interaction decreases total antioxidant activity in comparison with an equivalent amount of free quercetin.
In addition to plasma proteins, interactions may occur between polyphenols and food proteins. In many countries, phenolic-rich foods, such as coffee and tea, are usually consumed with milk. It was observed that the radical-scavenging activity of black tea alone was significantly higher than with milk( Reference Sharma, Kumar and Rao 108 ). Lorenz et al. ( Reference Lorenz, Jochmann and vonKrosigk 109 ) showed that the addition of milk to black tea blunted the tea's beneficial vascular effects, and milk caseins were identified as interfering agents via interaction with tea catechins and the subsequent formation of complexes. Similarly, Hasni et al. ( Reference Hasni, Bourassa and Hamdani 110 ) reported preferred binding sites in α- and β-caseins for tea catechins and suggested that the binding involves hydrogen bonding and hydrophobic interactions. For coffee–milk beverages, an immediate decrease of in vitro bioaccessible polyphenols was found when milk was mixed with coffee, which was also due to the binding of polyphenols to milk proteins( Reference Tagliazucchi, Helal and Verzelloni 111 ). In addition, soya proteins behave in a similar manner to milk caseins, resulting in protein–polyphenol binding and a net loss of antioxidant activity( Reference Rawel, Czajka and Rohn 112 ). This is reasonable considering the structural similarities of soya proteins and milk caseins. A cross-reactivity of milk caseins with soyabean glycinin and β-conglycinin has been described( Reference Rozenfeld, Docena and Añón 113 ). Further support is that polyphenols bind to the proline-rich regions of proteins, with subsequent complexation( Reference Luck, Liao and Murray 114 ), and both soyabean glycinin and β-conglycinin contain proline-rich regions( Reference Maruyama, Maruyama and Mikami 115 ).
In addition to food proteins, polyphenols have been reported to bind to enzymes. Phenolic extracts from a number of plants were found to be effective inhibitors of intestinal α-glucosidase/maltase activity( Reference Matsui, Ueda and Oki 116 ). The inhibitory effect is generally attributed to the ability of polyphenols to bind to the enzymes( Reference Funke and Melzig 117 – Reference Shobana, Sreerama and Malleshi 119 ). Similarly, α-glucosidase activity in vitro was significantly inhibited by anthocyanin-rich extracts of blueberry and blackcurrant( Reference McDougall, Shpiro and Dobson 120 ). Extracts of Salacia reticulata are used to prevent diabetes and obesity in Japan and exhibit pancreatic lipase inhibition, which has been partly attributed to ( − )-epigallocatechin, ( − )-epigallocatechin dimers, and a ‘tannin’ fraction( Reference Yoshikawa, Shimoda and Nishida 121 ). A comprehensive study on the inhibition of pancreatic lipase by tea polyphenols suggested that epigallocatechin 3-O-gallate was an effective inhibitor( Reference Nakai, Fukui and Asami 122 ). Proteases have also been shown to be inhibited by polyphenols. Trypsin was inhibited by phenolic-rich extracts of cocoa, pears and lentils( Reference Quesada, Bartolomé and Nieto 123 ).
Factors influencing the in vitro interactions of polyphenols with proteins are related to the physical and chemical properties of both proteins and polyphenols. It has been suggested that high-molecular-weight polyphenols bind to proteins more effectively( Reference Charlton, Baxter and Khan 124 , Reference Siebert 125 ). In contrast, when the conformation of a polyphenol is constrained, its capacity to interact with proteins is dramatically reduced regardless of the polyphenol's molecular weight( Reference Frazier, Papadopoulou and Green 126 – Reference Deaville, Green and Mueller-Harvey 129 ). In addition, hydrophobicity favours a strong association between proteins and polyphenols( Reference Poncet-Legrand, Edelmann and Putaux 130 ). The ability of proteins to bind to polyphenols also depends on protein size, secondary/tertiary structure and amino acid composition. In general, proteins that interact strongly with polyphenols have a high basic-residue content and a high proline content, are relatively large and hydrophobic, and have a conformationally open and flexible structure( Reference Lu and Bennick 131 – Reference Richard, Vitrac and Merillon 138 ). More details on the structure–affinity relationship of the interactions between polyphenols and proteins can be found in the review by Xiao & Kai( Reference Xiao and Kai 139 ).
Interaction of plant phenols with carbohydrates
A study of a phenolic acid–starch model system indicated that the interactions of phenolic acid with starch significantly contributed to the inhibitory effect of starch hydrolysis( Reference Kandil, Li and Vasanthan 140 ). The carboxyl and hydroxyl groups of phenolic acids are capable of binding to starch and other polysaccharides through hydrogen bonds, chelation or covalent bonds, forming bridges or cross-links( Reference Chi, Chang, Mou, Ho, Lee and Huang 141 ). The effect of tannic acid and catechin on legume starch hydrolysis( Reference Deshpande and Salunkhe 142 ) and the interference of gallic and chlorogenic acids with the starch–iodine reaction have been reported( Reference Sharma, Sharma and Kakkar 143 ). In addition to phenolic acids, various studies have shown that proanthocyanidins can interact with the different polysaccharides( Reference Nitta, Fang and Takemasa 144 , Reference Nishinari, Kim and Fang 145 ). Oligomeric and polymeric procyanidins have the ability to bind non-covalently on apple cell walls by simple incubation in aqueous buffer( Reference LeBourvellec, Guyot and Renard 146 – Reference Le Bourvellec, Bouchet and Renard 148 ). Moreover, the amount of procyanidins bound to the cell wall increases with procyanidin concentrations, degree of polymerisation and percentage of galloylation( Reference LeBourvellec, Guyot and Renard 146 – Reference Le Bourvellec, Bouchet and Renard 148 ). In addition, the apparent affinity constants between procyanidins and polysaccharides were found to decrease as follows: pectins > xyloglucan > starch > cellulose( Reference Le Bourvellec, Bouchet and Renard 148 ). Higher affinities are obtained with pectin, a polysaccharide having the ability to develop a gel-like network, forming hydrophobic pockets to encapsulate procyanidins. It has been reported that complex formation between soluble pectin and tannins reduces the astringency of both fresh juice and a solution of tannins purified from persimmon fruit( Reference Taira, Ono and Matsumoto 149 ). Filamentous and globular polysaccharides, like cellulose and xyloglucan, bind procyanidins weakly. The results are consistent with the results of Renard et al. ( Reference Renard, Baron and Guyot 150 ) which showed that xyloglucan has no marked effect for the procyanidins with a high degree of polymerisation. It was reported that nearly 35 % of the polyphenols in red wine are bound to soluble dietary fibre( Reference Saura-Calixto and Díaz-Rubio 151 ). It has also been reported that polyphenol–pectin complexes show no superoxide-scavenging capacity, have reduced hydroxyl radical-scavenging activity, and have high 2,2-diphenyl-1-picrylhydrazyl radical-scavenging activity, indicating potential changes in functionality because of complex formation( Reference Jacob and Paliyath 152 ). Another cellular study showed that when the extract was ingested along with carbohydrate-rich food, an enhanced uptake of polyphenols was observed( Reference Serra, Macià and Romero 153 ). This finding is in agreement with the results of Schramm et al. ( Reference Schramm, Karim and Schrader 154 ), who reported an enhanced activity of carbohydrate-rich foods and suggested that this effect may be mediated by a yet-unidentified carbohydrate–flavanol transporter.
Interaction of plant phenols with fats
Only a few studies have focused on the in vitro interactions between fats and polyphenols. A positive relationship has been observed between fat concentration and bioaccessibility of polyphenols after in vitro gastropancreatic digestion( Reference Tagliazucchi, Helal and Verzelloni 111 ). Results have indicated that a high fat content in food seems to have a protective effect, as hydrophobic interactions between fats and polyphenols increase the stability of polyphenols during digestion( Reference Ortega, Reguant and Romero 155 ). Similarly, the lipid fraction (rich in PUFA) showed a protective effect on the recovery of phenolic compounds during duodenal digestion. Disruption of the natural matrix under digestive conditions led to the release of PUFA, which could establish interactions with phenolic compounds, enhancing the compounds' recovery and stability during digestion( Reference Ortega, Macià and Romero 156 ). Certain indirect evidence has been provided by Langley-Evans( Reference Langley-Evans 157 ), who found that the addition of soya milk or cows' milk appeared to diminish the antioxidant potential of black tea preparations. This effect was greatest when whole milk was used and appeared to be primarily related to the fat content of the added milk( Reference Langley-Evans 158 ).
Interaction of plant phenols with food macronutrients: in vivo results
Interaction of plant phenols with proteins
Bioavailability refers to the extent to which and rate at which a substance becomes available to target tissue after administration. The clarification of polyphenol bioavailability is important because the polyphenol content and biological activity observed in vitro have little nutritional relevance unless the active metabolites of polyphenols can gain access to appropriate sites within the body( Reference Canon, Pate and Meudec 159 ). So far, few studies have investigated the effect of proteins alone on the bioavailability of phenolic compounds. Most researchers used foodstuffs directly. Milk is the most often chosen food because phenolic-rich foods are usually consumed with milk. Serafini et al. ( Reference Serafini, Ghiselli and Ferro-Luzzi 61 ) found that compared with tea consumed without milk, the antioxidant potential of tea with milk decreased. Mullen et al. ( Reference Mullen, Borges and Donovan 160 ) reported that milk significantly lowered the excretion of urinary flavan-3-ol metabolites. The overall 0–24 h excretion of metabolites corresponded to 18·3 % of intake after ingestion of a cocoa–water beverage compared with 10·5 % after ingestion of a cocoa–milk beverage. Moreover, they emphasised that, with a lower flavan-3-ol content, which is typical of commercial cocoas that are available to the general public, milk does have the capacity to interfere with absorption. Lorenz et al. ( Reference Lorenz, Jochmann and vonKrosigk 109 ) showed that the addition of milk to black tea blunted the tea's beneficial vascular effects. Similarly, yoghurt was also reported to have a marked impact on the urinary excretion of phenolic acids. The overall 0–24 h excretion of phenolic acids was 62 μmol after drinking orange juice alone and this fell markedly to 9·3 μmol when the orange juice was ingested with yoghurt( Reference Roowi, Mullen and Edwards 161 ). There were also several other studies that showed that the absorption of phenolic compounds or increased plasma antioxidant capacity is suppressed when the compounds are consumed with milk( Reference Serafini, Testa and Villano 162 , Reference Duarte and Farah 163 ). Most studies have attributed this inhibitory effect of milk to milk proteins because phenolic compounds can bind to milk proteins through hydrogen bonds and hydrophobic interactions( Reference Charlton, Baxter and Khan 124 , Reference Spencer, Cai and Martin 164 ). However, this speculation is based on in vitro studies. In addition to proteins, there are other macronutrients, such as carbohydrates and fats, in whole milk. These nutrients' possible effects cannot be excluded. In our recent study, after excluding the effects of other macronutrients, it was found that milk proteins led to significant changes in the plasma kinetics profile of polyphenols but did not affect the overall absorption of polyphenols in rats( Reference Zhang, Zheng and Liu 9 ). Similarly, it was also found that milk proteins extended the time needed to reach a maximal plasma polyphenol concentration in human subjects( Reference Zhang, Jiang and Guo 10 ). Casein, which is a major component of milk protein, can form curds in the stomach that delay casein's absorption compared with other proteins( Reference Barth, Pfeuffer and Scholtissek 165 ). It has been suggested that polyphenols can bind to milk proteins and subsequently form complexes( Reference Rawel, Kroll and Hohl 166 , Reference Dupas, Marsset-Baglieri and Ordonaud 167 ). Therefore, the in vivo results may be explained by the formation of protein–polyphenol complexes that remain in the stomach for an extended period of time, thereby delaying the appearance of polyphenols in the blood. Additionally, the absence of an effect on overall polyphenol absorption indicated that polyphenols could be released from the complexes and be absorbed later. Certainly, more studies are needed to evaluate the effect of different types of proteins on the bioavailability and in vivo bioactivity of polyphenols.
Interaction of plant phenols with carbohydrates
Consistent with the in vitro results, it has been shown that sugar and bread increase flavanol AUC values to 140 % of controls. The ability of treated meals to affect AUC values has been found to be positively correlated with carbohydrate content( Reference Schramm, Karim and Schrader 154 ) (Table 2). Moreover, Neilson et al. ( Reference Neilson, George and Janle 168 ) reported that the presence of sucrose in chocolate may positively influence the bioavailability of flavan-3-ols compounds. Several studies have attempted to clarify the mechanism associated with this increase in polyphenol bioavailability. The positive effects observed for carbohydrate-rich foods (bread, sucrose and grapefruit juice) may have been mediated by a carbohydrate-specific effect on gastrointestinal motility and/or secretion( Reference Schramm, Karim and Schrader 154 ). Along with an increase in the absorption of cocoa flavanols, a faster rate of flavanol elimination following sugar treatment has been observed. This observation is consistent with the concept of an increased tubular secretion of xenobiotics with increased solute filtration. Increased solute filtration can result from higher blood solute (xenobiotic) concentrations and an increased glomerular filtration rate( Reference Schramm, Karim and Schrader 154 ). In addition, an indigestible saccharide, difructose anhydride III, has been demonstrated to promote rutin absorption after a single bolus administration using portally and duodenally cannulated rats( Reference Matsumoto, Matsukawa and Chiji 169 ). This disaccharide is an enhancer of paracellular transport in the small intestine( Reference Mineo, Hara and Shigematsu 170 ). Furthermore, difructose anhydride III is fermentable in the large intestine( Reference Afsana, Shiga and Ishizuka 171 ), and it has been suggested that the bacterial fermentation of difructose anhydride III affects the bioavailability of flavonoid glycosides( Reference Tamura, Nishimukai and Shigematsu 172 ). It has also been suggested that fructo-oligosaccharides, which are the most popular non-digestible oligosaccharides and are well established to be readily fermentable( Reference Bomba, Nemcova and Gancarcikova 173 ), increase isoflavone glycoside absorption with the promotion of intestinal fermentation( Reference Uehara, Ohta and Sakai 174 ). The results strongly suggest that increased fermentation by oligosaccharides in the large intestine enhances polyphenol bioavailability. Moreover, the fermentation of oligosaccharides enlarges the intestinal mucosa( Reference Shiga, Nishimukai and Tomita 175 ), suppresses the bacterial degradation of polyphenols in the caecum( Reference Matsukawa, Matsumoto and Shinoki 176 ) and stimulates mucosal blood flow( Reference Younes, Alphonse and Hadj-Abdelkader 177 ), which may also contribute to polyphenol absorption in the large intestine. In addition, the cell walls are major components in fruits and vegetables and their impact cannot be ignored. Proanthocyanidins are associated with cell walls through either weak (hydrophilic/hydrophobic)( Reference Le Bourvellec and Renard 147 , Reference Le Bourvellec, Bouchet and Renard 148 ) or strong (covalent) interactions( Reference Pérez-Jiménez, Arranz and Saura-Calixto 178 ), which may influence their absorption( Reference Touriño, Pérez-Jiménez and Mateos-Martín 179 ). Early studies suggested that condensed tannins were not absorbed from the digestive tract of chicken and sheep( Reference Jimenez-Ramsey, Rogler and Housley 180 , Reference Terrill, Waghorn and Woolley 181 ). Recent rat and human studies suggested that proanthocyanidins with more than three subunits could be more resistant to degradation due to their chemical structure and, therefore, higher levels are observed in the colon( Reference Choy, Jaggers and Oteiza 47 ). Similar results were observed for hydroxycinnamic acid derivatives. In wheat, ferulic acid is ester-linked to position O-5 of the arabinosyl side chains of cell wall arabinoxylans. In fact, the presence of ester-linked monomeric and dimeric phenolics leads to reduced biodegradability of the cell wall polysaccharide, and limits the release of ferulic acid. Diferulates have a dramatic effect on both the rate and extent of polysaccharide degradation. Cross-linking through diferulates may inhibit the binding of endoxylanases, thus limiting the extent of arabinoxylan degradation and consequently the release of esterified ferulic acid( Reference Grabber, Hatfield and Ralph 182 ). Furthermore, cross-linking may prevent localised areas from swelling, excluding key enzymes for dietary fibre degradation( Reference Bunzel, Ralph and Marita 183 ). Likewise, Aprikian et al. ( Reference Aprikian, Duclos and Guyot 184 ) showed that procyanidins with high polymerisation inhibit enzymic degradation of cell walls in apples. Finally, covalently bound phenolics are released only after extensive microbial digestion in the colon( Reference Chesson, Provan and Russell 185 ).
Table 2 In vivo studies assessing the effects of food macronutrients on the bioavailability and bioactivity of polyphenols
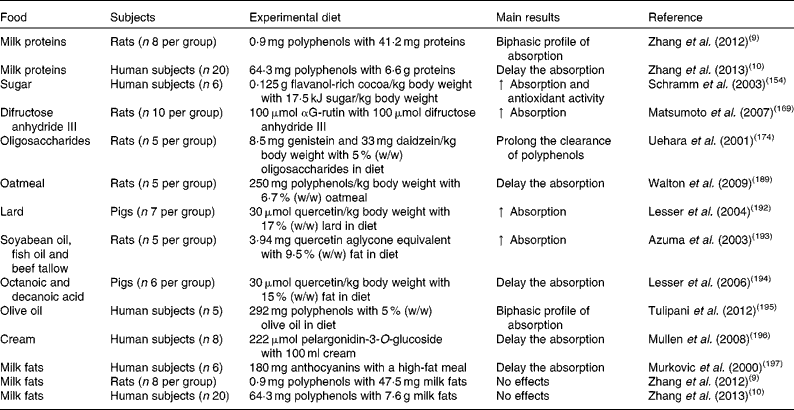
↑ , Increase.
In addition to the effect on absorption, carbohydrates have been observed to change the plasma kinetics profile of polyphenols. Bub et al. ( Reference Bub, Watzl and Heeb 186 ) reported that the mean time required to achieve a maximal plasma concentration of malvidin 3-glucoside was extended after the ingestion of red grape juice compared with red wine in human subjects. The researchers concluded that the delaying effect of the sugar present in the red grape juice could result from the competition of glucose and malvidin 3-glucoside for the Na-dependent GLUT, as reported for quercetin 3-glucoside( Reference Walgren, Lin and Kinne 187 , Reference Arts, Sesink and Hollman 188 ). The viscosities of certain types of carbohydrates also cannot be ignored. Anthocyanins were absorbed and excreted more slowly when administered in an acidified oatmeal solution than in an acidified water solution( Reference Walton, Hendriks and Broomfield 189 ). The addition of sucrose resulted in a slight delay in reaching a maximal plasma concentration. This delay may be partly due to the increase in the viscosity of sucrose( Reference Peters, Green and Janle 11 ). Sucrose formulations were observed to have viscosities approximately six times higher than non-sucrose-containing formulations. Increased viscosity is known to have an impact on gastric emptying and can influence the kinetics of absorption for phenolic compounds in the small intestine( Reference Marciani, Gowland and Spiller 190 ). As non-extractable proanthocyanidins are associated with the food matrix, particularly with insoluble polymers such as dietary fibre, their absorption may be slower compared with that of extractable proanthocyanidins. This deferred release would make non-extractable proanthocyanidin metabolites bioavailable for a long time after intake and may result in long-term health effects( Reference Mateos-Martín, Pérez-Jiménez and Fuguet 41 ). There is supporting evidence that the presence of ferulic acid–arabinoxylans bonds in the food matrix increases the occurrence time of ferulic acid in the organism. The phenomenon may be explained by a slower hydrolysis rate of phenolics–cell wall bonds by intestinal enzymes( Reference Andreasen, Kroon and Williamson 191 ).
Interaction of plant phenols with fats
Many investigations have shown that the co-ingestion of lipids could affect the bioavailability of polyphenols (Table 2)( Reference Lesser, Cermak and Wolffram 192 – Reference Murkovic, Toplak and Adam 197 ). In a pig study, the bioavailability of quercetin was significantly higher in a 17 % fat diet compared with a 3 % fat diet( Reference Lesser, Cermak and Wolffram 192 ). Azuma et al. ( Reference Azuma, Ippoushi and Ito 198 ) observed that the co-administration of lipids (soyabean oil or lecithin) and emulsifiers was able to enhance the intestinal absorption of quercetin in rats. In another rat study, the absorption of catechin was enhanced when green tea was administered as a phospholipid complex rather than as free catechins( Reference Pietta, Simonetti and Gardana 199 ). It has also been shown that more than 4·6 % (w/w) of soyabean oil in diets significantly enhanced the accumulation of quercetin metabolites in the plasma. Fish oil and beef tallow increased this accumulation to an extent similar to that of soyabean oil( Reference Azuma, Ippoushi and Ito 193 ). In addition to fat concentration, the effects of different fat types on the bioavailability of polyphenols have also been assessed. For example, the chain length of fatty acids has been reported to influence the micellarisation of phenolic compounds during digestion. Bioavailability was enhanced by 38 % and 12 % after intake along with medium-chain fatty acid and long-chain fatty acid diets, respectively, compared with the standard diet( Reference Lesser, Cermak and Wolffram 194 ). Moreover, the degree of saturation may affect the bioavailability of polyphenols. Goltz et al. ( Reference Goltz, Campbell and Chitchumroonchokchai 200 ) observed the increased absorption of carotenoids from a vegetable salad with higher levels of lipids and found that unsaturated fat promoted carotenoid absorption to a greater extent than saturated fat. For the mechanism associated with this increase in polyphenol bioavailability, it has been suggested that dietary fat can probably promote the solubility of polyphenols( Reference Lesser, Cermak and Wolffram 192 , Reference Mateo Anson, van den Berg and Havenaar 201 ).
In addition, fat has been reported to affect the absorption kinetics of polyphenols. After the ingestion of oil-enriched tomato sauces, naringenin metabolites showed a biphasic profile of absorption in the plasma, suggesting that the lipid matrix added to the sauce may stimulate reabsorption events via the enterohepatic circulation, thus potentially affecting the apparent plasma half-life of the flavanone before excretion( Reference Tulipani, Martinez Huelamo and Rotches Ribalta 195 ). Additionally, a delay in attaining a maximal plasma concentration was observed in subjects consuming strawberries with cream( Reference Mullen, Edwards and Serafini 196 ). These observations are consistent with an earlier study showing that the time needed to reach a maximal serum level was significantly extended when anthocyanins were consumed together with a high-fat meal( Reference Murkovic, Toplak and Adam 197 ). It was suggested that the fat present in the cream slowed the transit of anthocyanins due to duodenal and ileal brakes activated by fat( Reference Mullen, Edwards and Serafini 196 , Reference Welch, Bruce and Hill 202 ). It has also been shown that a maximal plasma concentration of quercetin was reached significantly later for a medium-chain fatty acid diet than for a long-chain fatty acid or a standard diet( Reference Lesser, Cermak and Wolffram 194 ).
Recently, a novel effect of fat on the bioavailability and antioxidant activity of polyphenols was found. At a relatively low content (3·8 % (w/v) in milk), milk fat alone did not interact with polyphenols and did not have an effect on the bioavailability and antioxidant activity of polyphenols in rats and human subjects( Reference Zhang, Zheng and Liu 9 , Reference Zhang, Jiang and Guo 10 ). Similarly, milk proteins did not affect the absorption and antioxidant activity of polyphenols. However, the combination of milk proteins and fats showed the highest degree of inhibition for both the bioavailability and the antioxidant capacity of polyphenols. To clarify the exact mechanism of this phenomenon, further in vitro and in vivo experiments were performed. The results showed that no significant difference was found in the median diameters of the emulsions between the consumption of polyphenols with milk fat and the consumption of milk fat alone, in accordance with the in vivo results, which did not show any interaction between milk fats and polyphenols. The median diameter of the mixture was significantly higher when polyphenols were consumed along with milk proteins than when milk proteins alone were consumed. The findings suggested the formation of milk protein–polyphenol complexes. However, during digestion, the decreasing trend of the median diameter indicated the breakdown of the milk protein–polyphenol complexes. Nevertheless, when polyphenols, milk proteins and fats were ingested together, a significant increase in the median diameter of the emulsion was found during digestion( Reference Zhang, Zheng and Liu 9 ). Considering that polyphenols can bind to milk proteins( Reference Luck, Liao and Murray 114 ) and that proteins can be adsorbed to the surface of lipid droplets( Reference Ye, Cui and Singh 203 ), the data evidenced the participation of milk fat in the interactions between milk proteins and polyphenols during digestion, resulting in remarkable aggregation. Unlike polyphenol–protein complexes, the complexes formed with proteins and fats did not completely break during digestion and thus impaired the bioavailability and antioxidant activity of the polyphenols. One possible reason is that the aggregation of the fat globules affects the way that gastrointestinal enzymes access substrates( Reference Michalski, Briard and Desage 204 ). Another explanation is that lipase activity is inhibited by phenolic compounds( Reference Ikeda, Tsuda and Suzuki 205 ) and long-chain fatty acids generated during digestion( Reference Borel, Armand and Ythier 206 – Reference Gargouri, Pieroni and Riviere 208 ). The findings show that there are synergisms between food macronutrients in affecting the bioavailability and bioactivity of polyphenols. Based on these results, we propose a hypothesis (Fig. 1) that allows the prediction of the effects of different food macronutrients on the bioavailability and bioactivity of polyphenols.

Fig. 1 A simplified scheme of the physico-chemical processes occurring before and after digestion when different food macronutrients are added to polyphenols. (A colour version of this figure can be found online at http://www.journals.cambridge.org/nrr)
Conclusions and future directions
Epidemiological studies have demonstrated that polyphenols can reduce the risk of many diseases. Because the health benefits of polyphenols are critically based on the compounds' bioavailability, more direct attention should be paid to the factors affecting polyphenols' bioavailability. The evidence collected in the present review suggests that when plant phenols are consumed along with food macronutrients, the bioavailability and bioactivity of polyphenols can be significantly affected. As summarised in Fig. 2, proteins can reduce the in vitro antioxidant activity and significantly change the plasma kinetics profile of polyphenols without affecting the absorption. Carbohydrates can extend the time needed to reach a maximal plasma concentration. Fats can enhance the absorption and change the absorption kinetics of polyphenols. Moreover, as highlighted in the present review, not only a nutrient alone but also certain synergisms between food macronutrients have a significant effect on the bioavailability and biological activity of polyphenols.

Fig. 2 Summary of the in vitro and in vivo effects of food macronutrients on the bioavailability and bioactivity of polyphenols.
In the future, it will be necessary to gain further insight into the interactive effects of food macronutrients on the bioavailability and bioactivity of polyphenols, including, but not limited to, the following. (1) More studies are needed to evaluate the in vivo fate of protein–polyphenol complexes for different types of proteins and the effect on the bioavailability and bioactivity of polyphenols. (2) More studies are necessary to further evaluate the interactions between different macronutrients and whether the nutrients' combination can have a collaborative or antagonistic effect on the healthy benefits of polyphenols. (3) Many studies have shown that many phenolic compounds are present in the plasma, largely in the form of metabolites. Therefore, future research efforts should better assess the effect of interactions with food macronutrients on the metabolism of polyphenols.
Acknowledgements
The present review was a China Postdoctoral Science Foundation-funded project (no. 2012M520463) and supported by the Beijing Higher Institution Engineering Research Center of Animal Product, National Science and Technology Support Program ‘Twelfth Five-Year Plan’ (no. 2012BAD12B08). The funders had no role in the design, analysis or writing of this article.
We acknowledge the contribution of all authors to the writing of the present review and H. Z. for conceiving the article. All authors approved the final manuscript.
The authors declare that they have no conflicts of interest.