Introduction
The past few years have seen a renewed interest in dietary long-chain n-3 PUFA (LC-n-3-PUFA) and their possible beneficial effects in numerous chronic diseases( Reference Cicero, Reggi and Parini 1 , Reference Wendel and Heller 2 ).
n-3 PUFA are hydrocarbon chains containing two or more double bonds. They belong to the n-6 or n-3 class depending on the position of the first double bond starting from the terminal methyl group. The parent fatty acids (FA) of the two n-6 and n-3 PUFA families are linoleic acid (LA; 18 : 2n-6) and α-linolenic acid (ALA; 18:3n-3), both considered dietary essential FA, since they cannot be endogenously synthesised by mammals. High amounts of LA are present in vegetable oils, seeds and nuts, whereas ALA is especially contained in leafy vegetables, seeds, walnuts, soyabeans, flaxseed and seed oils. Once entered into our cells, both FA are metabolised by a series of elongation and desaturation reactions to LC-PUFA. LA is converted to arachidonic acid (AA; 20 : 4n-6), whereas ALA to EPA (20 : 5n-3), and finally to DHA (22 : 6n-3). However, the endogenous production of EPA and DHA from ALA is not very efficient, especially at some ages and conditions; thus, the consumption of fish represents an alternative and more efficient route to enrich our tissues with these FA( Reference Deckelbaum and Torrejon 3 ).
The antineoplastic effects of n-3 PUFA have been studied for over 20 years in our laboratory by performing human trials( Reference Anti, Marra and Armelao 4 – Reference Palozza, Anti and Franceschelli 6 ), or preclinical studies on experimental animals( Reference Calviello, Palozza and Piccioni 7 – Reference Vecchini, Ceccarelli and Susta 10 ) and cultured cells( Reference Calviello, Di Nicuolo and Gragnoli 11 – Reference Fasano, Serini and Piccioni 16 ). All the results obtained have concurred to demonstrate the anti-neoplastic role of EPA and DHA. In particular, two clinical trials performed in the early 1990s showed that dietary supplementation with both EPA and DHA was able to normalise the atypical cell proliferation pattern present in the colonic mucosa of subjects at high risk for colon cancer( Reference Anti, Marra and Armelao 4 , Reference Anti, Armelao and Marra 5 ). We also demonstrated that EPA and DHA treatments delayed the development and reduced the growth of murine and human cancer cells transplanted in syngeneic animals( Reference Calviello, Palozza and Piccioni 7 – Reference Vecchini, Ceccarelli and Susta 10 ) or in nude mice( Reference Calviello, Di Nicuolo and Gragnoli 11 ).
So far, multiple biological mechanisms have been suggested to explain the inhibitory effects of LC-n-3 PUFA on cancer cell growth, and, particularly, their ability to induce apoptosis in cancer cells( Reference Calviello, Palozza and Piccioni 7 , Reference Calviello, Di Nicuolo and Gragnoli 11 , Reference Calviello, Di Nicuolo and Serini 12 , Reference Serini, Trombino and Oliva 14 – Reference Sun, Hu and Gu 21 ). Several molecular pathways are thought to be involved in their growth-inhibitory effect (Fig. 1). For instance, it has been proven that they induce deep alterations in the composition and function of lipid membrane microdomains (rafts and caveolae; mechanism A in Fig. 1). It has been suggested that such perturbations may alter the function of resident proteins (receptors, enzymes, transporters, adaptors; mechanism B in Fig. 1), and, in turn, the intracellular molecular responses associated with them( Reference Ma, Seo and Switzer 22 ). Moreover, it is well documented that they are able to deeply alter the cellular metabolism of eicosanoids (mechanism C in Fig. 1), bioactive molecules originating from EPA and AA and involved in cell signalling. These FA are cleaved from cell membrane phospholipids by phospholipase A2, and further metabolised by the enzymes cyclo-oxygenases (COX) and lipo-oxygenases, which convert them into PG, leukotrienes and thromboxanes. EPA competes with AA for these enzymes, but whereas the eicosanoids derived from AA have powerful inflammatory and proliferative activity, those derived from EPA exert scarce pro-inflammatory activity and, instead, have anti-proliferative effects( Reference Hardman 23 ). Recently, new enzymically derived products of EPA and DHA (resolvins and protectins) were identified (mechanism D in Fig. 1)( Reference Serhan 24 ). They show powerful activity in vitro and in vivo at nanomolar or picomolar concentrations in the resolution phase of inflammation or by acting as neuroprotective agents( Reference Serhan, Clish and Brannon 25 – Reference Mukherjee, Marcheselli and Serhan 27 ). Since the pathogenic role of inflammation in the development of many kinds of tumours( Reference Gonda, Tu and Wang 28 ) was well established, the antineoplastic activity of n-3 PUFA has also been related to the action of these bioactive molecules. Furthermore, although there is still much debate as to whether n-3 PUFA may function in our tissues as pro-oxidants or antioxidants( Reference Serini, Fasano and Piccioni 29 ), most of the findings concur to demonstrate that n-3 PUFA are able to sensitise cells to reactive oxygen species (ROS) through their high peroxidability, due to the presence in their hydrocarbon chain of multiple double bonds (mechanism E in Fig. 1)( Reference Mazière, Conte and Degonville 30 ). This mechanism has been invoked by a number of studies correlating the cytotoxic action of n-3 PUFA in cancer cells in vitro with their pro-oxidant potential( Reference Ding, Vaught and Yamauchi 31 – Reference Germain, Lavandier and Chajès 33 ), and the formation of cytotoxic metabolic intermediates( Reference Gleissman, Yang and Martinod 34 ). Many reports have also demonstrated the ability of n-3 PUFA to modulate the expression of several proteins and transcription factors involved in the molecular pathways associated with cell proliferation and survival (mechanism F in Fig. 1)( Reference Calviello, Serini and Palozza 35 ). The multiplicity of mechanisms activated by these FA may be disorienting. However, it is possible to hypothesise( Reference Calviello, Serini and Palozza 35 ) that the activation of some of them (such as mechanisms A and E in Fig. 1) may be more directly related to n-3 PUFA. These mechanisms could be considered as starting points for the activation of further LC-n-3 PUFA-dependent secondary mechanisms (such as mechanisms B, C, D and F in Fig. 1).
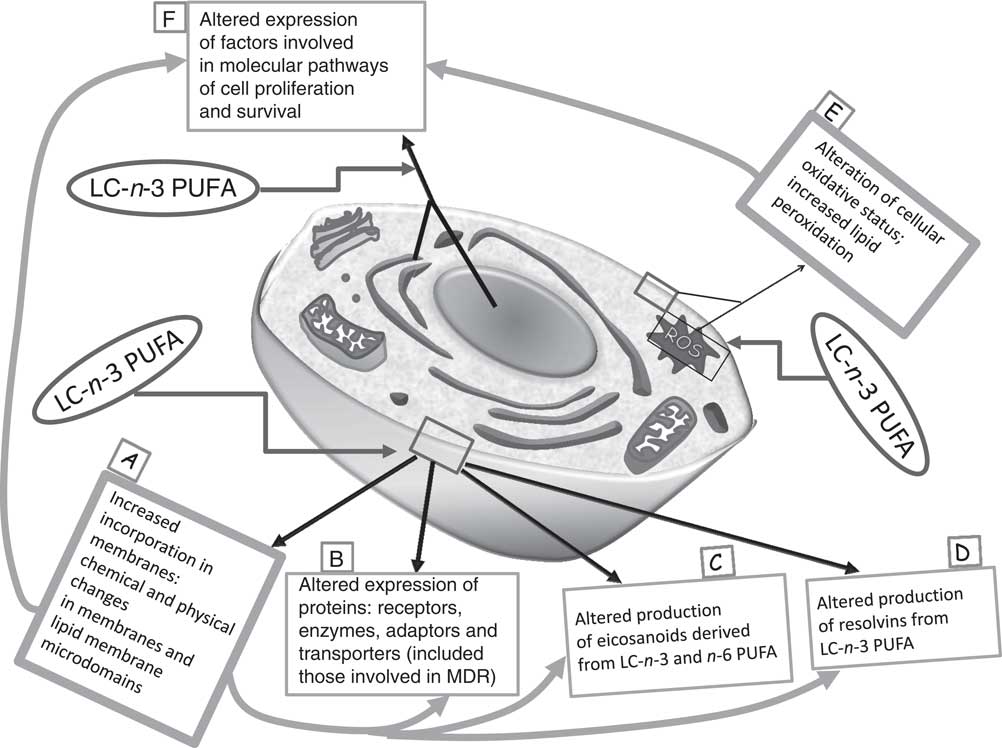
Fig. 1 Main mechanisms proposed to explain the inhibitory effect of long-chain (LC)-n-3 PUFA on cancer cell growth. It is possible to hypothesise that the activation of the molecular mechanisms A and E (in bold boxes, ‘unifying mechanisms’) may be more directly related to the treatment with n-3 PUFA. As a consequence, other mechanisms (in thin boxes: B, C, D and F) may be induced. MDR, multidrug resistance; ROS, reactive oxygen species.
The idea of a possible preventive usage of LC-PUFA against cancer has become quite acceptable, since within an established range of doses (0.5–2 g/d), most of the published data have proven that their intake cannot be considered dangerous, or induce toxic or carcinogenic effects( Reference Calviello, Su and Weylandt 36 ). Since they are usual components of our diet, a preventive use could be simply realised by increasing the consumption of n-3 PUFA-rich foods or by supplementing them in capsules containing marine oils or EPA and/or DHA in a purified form. On the other hand, it is unlikely that dietary supplementation alone could be an effective therapy for cancer patients. Instead, because of their pleiotropic effects, and their ability to modulate many biological processes and molecular pathways in the cells, an interesting hypothesis has been considered. It suggests that n-3 PUFA could be administered in combination with the currently used anticancer agents, with the main aim to enhance the efficacy of these therapeutic treatments, as well as to reduce their side effects. Several years ago, we reviewed all the studies that had evaluated this new combined therapeutic strategy up to that point( Reference Calviello, Serini and Piccioni 37 ). However, additional and interesting papers have been published on this subject meanwhile. Moreover, we are recently witnessing an upsurge of interest in n-3 PUFA as anti-inflammatory and anti-neoplastic agents. Thus, in the present review we will update the analysis of the existing data regarding the beneficial effects exerted by n-3 PUFA in combination with anti-neoplastic drugs and radiation.
Methodology
Literature search
A systematic literature search of the PubMed database was conducted in the period from July 2014 to July 2015 to identify published peer-reviewed original research articles regarding in vitro studies, and in vivo animal and human interventional studies on n-3 PUFA combined with conventional (chemotherapy or radiotherapy) cancer therapies. The main search terms used for the search of titles and abstracts were: ‘omega-3 PUFA’ or ‘n-3 PUFA’ or ‘docosahexaenoic acid’ or ‘eicosapentaenoic acid’ or ‘fish oil’; and ‘animal studies’, or ‘human studies’, or ‘in vitro studies’, or ‘in vivo studies’; and ‘chemotherapy’ or ‘radiotherapy’ or ‘antineoplastic drug’ and ‘cancer’ or ‘tumor’; and ‘combination’ or ‘combined treatment’. We mostly identified full-text articles written in English. The papers were chosen without restriction of time. Of course, whatever the criteria used to choose papers to be analysed, it is useful to be reminded that, as recently emphasised by Song et al. ( Reference Song, Parekh and Hooper 38 ), there still remains the tendency of researchers and journal editors to prefer the outcomes showing significant findings. This, however, represents a bias in all fields of published literature.
Presentation of data
The in vitro studies were first considered, followed by the preclinical animal studies and the human clinical interventional trials. The results and the molecular mechanisms invoked were critically analysed. The results were also summarised in several comprehensive tables reporting the information for each therapeutic combination studied, the concentrations/dose of both anticancer drugs/radiation and n-3 PUFA/fish oils (FO), the cellular/animal models used or the types of clinical trials performed, and the anticancer as well as the biological and molecular effects obtained.
In vitro studies
A broad range of experimental in vitro studies, mainly performed on colon and breast cancer cell lines, as well as on leukaemia cells, have demonstrated an enhanced efficacy of treatments with conventional antineoplastic agents (either drugs or radiation) when they were administered in combination with n-3 PUFA. Just a few experimental studies were also performed by using cancer cells originating from other tissues. We will analyse the studies in four separate paragraphs on the basis of the cancer cells used (colon cancer cells, breast cancer cells, leukaemia/lymphoma and myeloma cells, and other kinds of cancer cells). This paragraph division is related to the fact that the antineoplastic treatments administered in combination were usually specific for each kind of cancer cells, that is, those generally employed against the human cancers derived from those cells.
Colon cancer cells
All of the results obtained by adding LC-n-3 PUFA to colon cancer cells treated in vitro with anti-neoplastic agents have concurred to demonstrate a remarkable enhancement in the ability of cells to undergo apoptosis (Table 1 and Table 2). In most of the papers analysed, the possible molecular changes underlying this effect were also investigated. Several years ago, we demonstrated that DHA was able to enhance the efficacy of 5-fluorouracil (5-FU), one of the most widely used drugs in the therapy of this type of cancer. DHA induced a synergistic growth-inhibitory and pro-apoptotic effect in four different types of human colon cancer cells, and this effect was independent on cell p53 status( Reference Calviello, Di Nicuolo and Serini 12 ). This result is interesting, since it is known that therapy with 5-FU is less effective in patients bearing p53-mutant colon cancers, and also because the anti-tumour effect was observed with concentrations of 5-FU lower than those commonly found in the serum of patients treated with 5-FU (in the range 0·1–1 μm)( Reference Calviello, Di Nicuolo and Serini 12 ). This finding suggested that n-3 PUFA in vivo could increase the therapeutic potential of low doses of 5-FU, and, thus, avoid the undesired side effects associated with high 5-FU doses. More recently, Granci et al. ( Reference Granci, Cai and Lecumberri 39 ) confirmed these results, showing that a FO emulsion (corresponding to about 45 µm-EPA+DHA) enhanced the pro-apoptotic effect of 1 µm-5-FU in HT-29 cells, whereas a soyabean oil emulsion was ineffective. The authors ascribed this effect to the ability of the FO emulsion to activate the Bax-dependent apoptotic pathway. Also Jordan & Stein( Reference Jordan and Stein 40 ) investigated the effect of a combination of 5-FU and a FO-based lipid emulsion rich in n-3 FA in Caco-2 colon cancer cells. In agreement with our results, they found that the combination FO emulsion/5-FU additively inhibited cell growth, both decreasing cell cycle progression and inducing apoptosis.
Table 1 Colon cancer cells in vitro: effects of n-3 PUFA in combination with conventional antineoplastic agents

5-FU, 5-fluorouracil; FO, fish oil; Δψm, mitochondrial membrane potential; CK20, cytokeratin 20; MUC2, mucin 2; CSC, cancer stem cells; CSLC, cancer stem-like cells; PARP, poly(ADP-ribose) polymerase; AKT, protein kinase B; PTEN, phosphatase and tensin homolog; p-XSC, 1,4-phenylenebis(methylene)selenocyanate; COX-2, cyclo-oxygenase-2; iNOS, inducible NO synthase; TRAIL, TNF-related apoptosis-inducing ligand; ROS, reactive oxygen species
Table 2 Colon cancer cells in vitro: effects of n-3 PUFA in combination with conventional antineoplastic agents

TRAIL, TNF-related apoptosis-inducing ligand; CHOP, C/EBP homology protein; Δψm, mitochondrial membrane potential; cIAP, cellular inhibitor of apoptosis protein; XIAP, X-linked inhibitor of apoptosis protein; PERK, protein kinase RNA-like endoplasmic reticulum kinase; PDT, photodynamic therapy; ROS, reactive oxygen species; RNS, reactive N species; cEPA, conjugated EPA; Pols, DNA polymerases; COX-2, cyclo-oxygenase-2; PARP, poly(ADP-ribose) polymerase; P-gp, P-glycoprotein; CAR, constitutive androstane receptor; RXR, retinoid X receptor; HMGCoAR, 3-hydroxy-3-methylglutaryl-coenzyme A reductase; MRP1, multidrug resistant related protein 1.
The same n-3 PUFA/5-FU combination was investigated recently in colon cancer stem-like cells (CSLC). It was observed that EPA increases the sensitivity of the CD133+ CSLC to 5-FU( Reference De Carlo, Witte and Hardman 41 ). This may represent a highly innovative frontier in the field of new possible therapeutic strategies using antineoplastic agents/n-3 combinations. A growing body of evidence supports the hypothesis that human cancer is a stem cell disease, and that only the CSLC fraction possesses cancer-initiating potential, as well as the ability to sustain a tumour( Reference Presnell, Petersen and Heidaran 42 , Reference Reya, Morrison and Clarke 43 ). Moreover, it has been shown that these cells have a role in the acquisition of chemotherapy resistance and tumour relapse. Interestingly, it has been observed that EPA is able to down-regulate the expression of CD133( Reference De Carlo, Witte and Hardman 41 ), a marker used to isolate populations of colon cancer cells that retain stem cell properties from both established cell lines and primary cell cultures. On the contrary, EPA increased the expression of differentiation markers in colonic epithelium, such as cytokeratin 20 and mucin 2( Reference De Carlo, Witte and Hardman 41 ). Moreover, in a recent study performed in chemoresistant colon cancer cells highly enriched in CSC, it was shown that EPA improved the ability of 5-FU+oxaliplatin (FuOx) not only in inhibiting colon cancer cell growth and colonosphere formation, but also in reducing CSC population. Also in this case the suppression of cancer cell growth was the result of increased apoptosis, as evidenced by poly(ADP-ribose) polymerase (PARP) cleavage( Reference Vasudevan, Yu and Banerjee 44 ).
Moreover, on the basis of previous epidemiological and preclinical studies showing that diets rich in Se had the potential to reduce the incidence of colon cancer( Reference El-Bayoumy, Rao and Reddy 45 ), Narayanan et al. ( Reference Narayanan, Narayanan and Desai 46 ) evaluated the effect of a combination of DHA with 1,4-phenylene bis(methylene) selenocyanate, a synthetic organoselenium compound less toxic and more effective than inorganic Se. Interestingly, it was observed that the combined treatment efficiently inhibited colon cancer cell growth by enhancing apoptosis( Reference Narayanan, Narayanan and Desai 46 ). They suggested that the effect could be related to the down-regulation of several genes involved in colon cancer cell growth (i.e. COX-2, inducible NO synthase, cyclin D1, β-catenin and NF-κB-p65).
Of great interest are also the results showing that DHA is able to sensitise human colon adenocarcinoma HT-29 cells to the apoptosis induced by TNF-related apoptosis-inducing ligand (TRAIL)( Reference Vaculová, Hofmanová and Andera 47 , Reference Skender, Hofmanová and Slavík 48 ). This is a member of the TNF family and a very promising anti-cancer agent( Reference Nagane, Huang and Cavenee 49 ), since it possesses the unique property to induce cancer cell death while sparing most normal cells( Reference Nagane, Huang and Cavenee 49 ). However, its clinical application has been so far hampered due to the resistance that some cancer cells show to TRAIL-induced apoptosis( Reference Zhang, Nguyen and Thomas 50 ). For this reason, possible combinations of TRAIL with apoptosis inducers able to synergise with it and, thus, sensitise apoptosis-resistant cancer cells have been intensively studied over the last 10 years( Reference Refaat, Abd-Rabou and Reda 51 ). Based on the results obtained, the combinatory strategy is considered very promising, even though to be still verified in clinical settings. Particularly, Skender et al. ( Reference Skender, Hofmanová and Slavík 48 ) recently showed that DHA sensitised cancer cells to the pro-apoptotic effect of TRAIL thanks to its ability to activate Bax and Bak, whose down-regulation had been associated with resistance to TRAIL( Reference Ravi and Bedi 52 , Reference Gillissen, Wendt and Richter 53 ). Moreover, DHA acted also by activating the endoplasmic reticulum stress response and up-regulating CCAAT-enhancer-binding protein homologous protein (CHOP). This is one of the pathways considered crucial to be targeted in a combinatory treatment with TRAIL( Reference Refaat, Abd-Rabou and Reda 51 ).
The exposure to photodynamic therapy or radiation induces apoptosis in colon cancer cells, and the combined treatment with DHA, EPA or conjugated EPA was shown to enhance it( Reference Kello, Mikes and Jendzelovský 54 – Reference Manda, Kriesen and Hildebrandt 57 ). The mechanism proposed to explain the pro-apoptotic effect of these combinations was increased oxidative stress and lipid peroxidation, which may also activate the inflammatory response( Reference Kello, Mikes and Jendzelovský 54 – Reference Manda, Kriesen and Hildebrandt 57 ), as well as the increased DNA damage due to decreased expression of DNA polymerases( Reference Kumamoto-Yonezawa, Sasaki and Suzuki 55 ).
In agreement, it was recently shown that treatment of different colon cancer cells with DHA was able to enhance sulindac-sulfide-induced apoptosis in vitro through an enhanced cleavage of PARP and caspase-8 activation( Reference Lim, Lee and Lee 58 ). This finding is interesting, since sulindac analogues are compounds belonging to the group of non-steroidal anti-inflammatory drugs, and are well known for being extremely efficacious in reducing colon cancer risk( Reference Shi, He and An 59 ). This finding was confirmed in the same paper also in vivo, by showing that the simultaneous intraperitoneal treatment with sulindac and DHA was able to suppress the growth of tumours derived from colorectal cancer cells transplanted in nude mice more efficiently than the treatment of each single compound administered alone.
Finally, the treatment of Caco-2 colon adenocarcinoma with both EPA + DHA was recently observed( Reference Kuan, Walker and Luo 60 ) to significantly enhance the pro-apoptotic effect of paclitaxel, a well-known antineoplastic agent used in the treatment of different types of cancers. The authors found that both DHA and EPA were able to reduce the expression of the drug transporter protein P-glycoprotein (P-gp), involved in the development of multidrug resistance (MDR) during cancer progression. This findings is of interest, since the resistance of tumour cells to conventional anticancer drugs represents a problem for the success of cancer chemotherapy( Reference Ullah 61 ). It is known that MDR can be caused in part by the expression of three drug transporter proteins: ABCB1/P-gp, ABCC1/multidrug resistance protein-1 (MRP1) and ABCG2/breast cancer resistance protein, belonging to the ATP-binding cassette (ABC) superfamily and possessing the function of ATP-dependent drug-efflux pumps( Reference Szakács, Paterson and Ludwig 62 ). Moreover, it has been shown that the activity of P-gp and MRP1 is increased by high amounts of cholesterol in the plasma membrane and detergent-resistant membranes( Reference Bacso, Nagy and Goda 63 – Reference Klappe, Hinrichs and Kroesen 65 ). In keeping, Gelsomino et al. ( Reference Gelsomino, Corsetto and Campia 66 ) recently reported that n-3 PUFA were able to sensitise MDR colon cancer cells to doxorubicin by a mechanism involving the down-regulation of endogenous cholesterol synthesis. These results demonstrate that MDR may be targeted by n-3 PUFA in colon cancer cells, and that the inhibition of MDR may represent one of the main pathways through which these FA may enhance the antineoplastic efficacy of some of the conventional chemotherapeutic drugs.
Breast cancer cells
In contrast to what is observed in colon cancer cells, the increased sensitivity of breast cancer cells to chemotherapeutic drugs administered in combination with LC-n-3 PUFA (Table 3) has been almost totally ascribed to the enhanced cytotoxic pro-oxidant effect of the drugs. Reasonably, this may be due to the use of drugs that themselves act mainly through a pro-oxidant mechanism. In two articles( Reference Germain, Chajès and Cognault 67 , Reference Mahéo, Vibet and Steghens 68 ) doxorubicin was used, and it was observed that DHA enhanced oxidative stress-dependent cytotoxicity induced by this drug, and increased the production of lipid peroxidation products in breast cancer cells (MDA-MB-231 and MCF-7dox, a doxorubicin-resistant cell line, derived from the parental MCF-7 cells). These findings were further confirmed by the observation( Reference Vibet, Goupille and Bougnoux 69 ) that the enhanced cytotoxicity induced by DHA in anthracycline-treated MDA-MB-231 breast cancer cells was concomitant with a decreased activity and expression of glutathione peroxidase, an antioxidant enzyme playing a key role against hydrogen and lipid peroxides. It was also observed( Reference Menendez, Lupu and Colomer 70 ) that DHA was able to synergistically enhance the taxane cytotoxicity in the highly metastatic MDA-MB-231 cells treated with paclitaxel or docetaxel. In this case, however, the pro-apoptotic effects of n-3 PUFA could be involved, since the main mechanism through which taxanes are believed to exert cytotoxicity and anti-tumour activity is microtubule stabilisation and induction of cell cycle arrest and apoptosis. According with all these findings, it has been reported that DHA is able to improve the growth-inhibitory effect of doxorubicin in oestrogen receptor-negative MDA-MB-231 cells, and that the underlying mechanism involved the activation of the CD95-triggered apoptosis( Reference Ewaschuk, Newell and Field 71 ). Interestingly, however, it has been recently reported that also taxanes may exert their cytotoxic effect through the activation of intracellular oxidative stress( Reference Meshkini and Yazdanparast 72 ). Nevertheless, in this case it was not the oxidative stress induced by both PUFA and the taxane, but the decreased expression of Her-2/neu oncoprotein to be invoked by the authors to explain the strong synergistic cytotoxic effect exerted by DHA. The effect was visible also in the taxane-resistant SK-BR-3 and BT-474 breast cancer cells( Reference Menendez, Lupu and Colomer 70 ). Instead, lipid peroxidation and oxidative stress were suggested again to be responsible for the reduced viability of SK-BR-3 cells treated with EPA in combination with As2O3 ( Reference Wirtitsch, Roth and Bachleitner-Hofmann 73 ).
Table 3 Breast cancer cells in vitro: effects of n-3 PUFA in combination with conventional antineoplastic drugs

MCF-7dox*, doxorubicin-resistant MCF-7 cells; ROS, reactive oxygen species; GPx, glutathione peroxidase; Her-2/neu, human epidermal growth factor receptor-2; FADD, Fas-associated death domain-containing protein; LOV, lovastatin.
In some studies on breast cancer cells, n-3 PUFA were chemically conjugated with other drugs in an effort to examine new innovative therapeutic strategies. For instance, in an additional study, Siddiqui et al. ( Reference Siddiqui, Zerouga and Wu 74 ) synthesised a conjugated EPA or DHA moiety with propofol, and these conjugates were tested in MDA-MB-231 breast cancer cells for their ability to modulate cell migration, adhesion and apoptosis. Propofol (2,6-diisopropylphenol) is the most extensively used anaesthetic–sedative agent possessing the characteristic to be non-toxic to humans also at high levels (50 μg/ml). It has been also reported that concentrations of propofol in the range of 20–50 μm possess anticancer properties( Reference Mammoto, Mukai and Mammoto 75 , Reference Tsuchiya, Asada and Arita 76 ). Interestingly, the propofol–DHA or propofol–EPA conjugates( Reference Siddiqui, Zerouga and Wu 74 ) were able to significantly inhibit cell adhesion and migration and to induce apoptosis in the cells more than DHA, EPA or propofol alone, but the authors did not investigate the molecular mechanism underlying these effects. Moreover, DHA was recently conjugated with lovastatin( Reference Siddiqui, Harvey and Xu 77 ), and the lovastatin–DHA (LOV-DHA) conjugate was able to induce apoptosis in MDA-MB-231 cells at concentrations as low as 1 µm. Statins are drugs inhibiting the activity of the enzyme 3-hydroxy-3-methylglutaryl-CoA (HMG-CoA) reductase, a key enzyme in the synthesis of cholesterol( Reference Goldstein and Brown 78 ). They have also been involved in the regulation of cell proliferation, differentiation, apoptosis and invasion( Reference Kusama, Mukai and Iwasaki 79 – Reference Collisson, Kleer and Wu 81 ), and they were shown to inhibit the proliferation of different kinds of cancer cells, including breast cancer( Reference Wei, Mi and Zhou 82 – Reference Klawitter, Shokati and Moll 84 ). Interestingly, while the addition of mevalonate reversed the effect of lovastatin, it did not modify the growth-inhibitory effects of the LOV-DHA conjugate, suggesting that the conjugate acted through a HMG-CoA reductase-independent pathway( Reference Siddiqui, Harvey and Xu 77 ).
Prostate cancer cells
A clear molecular mechanistic approach has been used in the studies performed on prostate cancer cells treated with LC-n-3 PUFA combined with different drugs, but the mechanisms proposed are highly variable (Table 4). Narayanan et al. ( Reference Narayanan, Narayanan and Reddy 85 ) found that a combination of DHA and celecoxib at low doses (2·5 μm each) powerfully prevented prostate cancer cell growth. The authors suggested that DHA could exert its effect through the modulation of COX-2, NF-κB, the nuclear receptors PPAR-γ and retinoid X receptor, all these factors being involved in prostate carcinogenesis( Reference Narayanan, Narayanan and Reddy 85 ). The effects induced by DHA/celecoxib were significantly higher than those obtained with the two compounds given alone, even at higher concentrations. In an additional study, these authors( Reference Narayanan, Narayanan and Bosland 86 ) found that DHA in combination with celecoxib was also able to increase Hsp70 levels and p53 transport into the nucleus in prostate cancer cells, thus providing an additional COX-2-independent anti-tumour mechanism. This effect of DHA is in line with what has been observed in previous studies, which had revealed that the increased expression of heat shock protein isoforms could induce anti-tumour activities in the presence of wild-type p53( Reference Wang, Grossmann and Young 87 , Reference Lipinski, Pelech and Mountain 88 ).
Table 4 Prostate cancer cells in vitro: effects of n-3 PUFA in combination with conventional antineoplastic drugs

COX-2, cyclo-oxygenase-2; RXR, retinoid X receptor; FADD, Fas-associated death domain-containing protein; AKT1, protein kinase B1; TRAF3, TNF receptor-associated factor 3; MAP2k4, mitogen-activated protein kinase kinases 4.
Interestingly, the down-regulation of NF-κB-related genes was reported in either hormone receptor-positive or -negative LNCaP, DU145 and PC3 cells treated with DHA and docetaxel, and it was associated with the synergistic induction of apoptosis by the two compounds( Reference Shaikh, Brown and Schofield 89 ). This kind of combination is of great interest, since the results of two recent clinical trials have shown taxanes, docetaxel in particular, to be highly efficient drugs for the therapy of advanced and hormone-refractory prostate cancer( Reference Petrylak 90 , Reference Collins, Trowman and Norman 91 ). However, docetaxel is cytotoxic and can induce deleterious side effects, such as myelosuppression and granulocytopenia, which limit the dose that can be administered to patients( Reference Yared and Tkaczuk 92 ). Thus, it could be hypothesised that the combined treatment with DHA could allow a decrease in the doses of docetaxel, but still to obtain the growth-inhibitory effects obtained with higher doses.
Leukaemia, lymphoma and myeloma cells
Similarly to what was observed in breast cancer cells, the main molecular mechanisms proposed in most of the papers investigating anti-neoplastic agents/LC-n-3 PUFA combinations in leukaemia, lymphoma and myeloma cells are enhanced cellular oxidative stress and lipid peroxidation (Table 5 and Table 6). In one of these studies( Reference Sturlan, Baumgartner and Roth 93 ), n-3 PUFA have been combined with As2O3, which possesses a broad spectrum of antileukaemic activity due to its ability to induce apoptosis via intracellular production of ROS( Reference Chou and Dang 94 ). Since this compound is not effective at the same degree in all types of leukaemic cells, the search for agents enhancing As2O3 efficacy has recently gained great interest. In this study, Sturlan et al. ( Reference Sturlan, Baumgartner and Roth 93 ) observed that DHA is able to enhance cytotoxicity of As2O3 in HL-60 cells through the induction of apoptosis, and the effect was ascribed to the synergism of the two combined agents in producing intracellular ROS and toxic lipid peroxidation products. In fact, the enhanced efficacy of the combination was abolished by treatment with vitamin E or by the substitution of DHA with oleic acid (a non-peroxidisable FA)( Reference Sturlan, Baumgartner and Roth 93 ). Other studies confirmed the synergistic cytotoxic effect of this combination in leukaemic as well as in solid tumour cells( Reference Wirtitsch, Roth and Bachleitner-Hofmann 73 , Reference Brown, Bellon and Nicot 95 ). It is important to underline that in one of these studies( Reference Brown, Bellon and Nicot 95 ), where DHA was used in combination not only with As2O3, but also IFN-γ and emodin, clinically achievable doses of DHA and emodin allowed reduction in As concentrations by 100-fold, preserving high toxicity in tumour cells( Reference Brown, Bellon and Nicot 95 ). Similarly, enhanced cytotoxicity was observed and the pro-oxidant mechanism was invoked in a range of leukaemia or lymphoma cells when DHA was used in combination with other antineoplastic drugs (adriamycin, methotrexate, doxorubicin, dexamethasone, mitomycin and imatinib)( Reference Guffy, North and Burns 96 – Reference Kinsella and Black 99 ). Similarly, it was shown( Reference Fahrmann and Hardman 100 ) that treatments with EPA or DHA enhanced the sensitivity of the B-leukaemic cell lines to the cytotoxic effect of doxorubicin, vincristine and fludarabine by promoting their arrest in the cell cycle G2/M phase and inducing their death.
Table 5 Leukaemia cells in vitro: effects of n-3 PUFA in combination with conventional antineoplastic drugs

Δψm, Mitochondrial membrane potential; ROS, reactive oxygen species; TBARS, thiobarbituric acid-reactive substances; IFN-α, interferon α; AKT, protein kinase B; Bcr-Abl HL-60*, Bcr-Abl protein expressing HL-60 cells; MDA, malondialdehyde.
Table 6 Lymphoma and myeloma cells in vitro: effects of n-3 PUFA in combination with conventional antineoplastic drugs

ROS, reactive oxygen species; PKC-δ, protein kinase Cδ.
Another combination recently investigated in lymphoma cells was hyperthermia together with n-3( Reference Cui, Piao and Kondo 101 ). Hyperthermia has been used as an anticancer therapeutic strategy for several years( Reference Overgaard 102 ), but an important limitation of this treatment is that it often does not sufficiently kill neoplastic cells. In their paper, Cui et al. ( Reference Cui, Piao and Kondo 101 ) showed that combining hyperthermia (44°C for 10 min) with treatment with 20 µm-DHA induced significant apoptosis in human myelomonocytic lymphoma U937 cells. Again, increased ROS production, together with mitochondria dysfunction, and protein kinase C-δ activation were shown to be the mechanisms underlining the effects of this combination.
To finish, it is noteworthy, in the recent remarkable finding of Abdi et al. ( Reference Abdi, Garssen and Faber 103 ), that n-3 PUFA are able to sensitise also multiple myeloma cells to bortezomib. Multiple myeloma is a malignant neoplasm of terminally differentiated B cells infiltrating the bone marrow, and most patients, even when treated with more innovative drugs such as this one, develop intrinsic drug resistance or become chemoresistant in the course of treatment. Both primary cells from myeloma patients and different human myeloma cell lines have been shown to develop resistance to bortezomib( Reference Markovina, Callander and O’Connor 104 , Reference Ri, Iida and Nakashima 105 ). The mechanism of antineoplastic action of bortezomib in multiple myeloma involves the inhibition of the NF-κB signalling pathway( Reference Hideshima, Chauhan and Richardson 106 ), and many reports have demonstrated a marked suppression of this pathway by n-3 PUFA( Reference Patterson and Georgel 107 ). As a matter of fact, it was found that EPA and DHA affected multiple signalling pathways in multiple myeloma cells, including NF-κB, Notch, Hedgehog, oxidative stress and Wnt( Reference Abdi, Garssen and Faber 103 ). Finally, it is worth noticing also that EPA and DHA enhanced the sensitivity of multiple myeloma cells to bortezomib but did not affect the viability of normal peripheral mononuclear cells.
Other kinds of cancer cells
Besides the studies performed in colon, breast and prostate cancer cells, and in leukaemia, lymphoma and myeloma cells, only a few studies have been performed in cells deriving from other tissues (Table 7). Most of these studies also showed that the synergistic cytotoxic effect of conventional antineoplastic agents and LC-n-3 PUFA combined together could be explained on the basis of their pro-oxidant effect. For instance, a significant cytotoxic effect mediated through enhanced lipid peroxidation and ROS production was observed in drug-resistant neuroblastoma cells treated with n-3 PUFA in combination with several antineoplastic drugs, such as vincristine( Reference Ikushima, Fujiwara and Todo 108 ), As2O3, cisplatin, doxorubicin and irinotecan( Reference Lindskog, Gleissman and Ponthan 109 ). Similarly, increased oxidative stress-dependent cytotoxicity was observed( Reference Rudra and Krokan 110 ) in glioblastoma and bronchial carcinoma cells treated with combinations of DHA and doxorubicin. However, in a more recent study( Reference Wang, Bhat and Doucette 111 ) specific oligo-array analyses demonstrated that the enhanced effectiveness of etoposide administered in combination with DHA to brain tumour cells (medulloblastoma and glioblastoma) was related to a markedly reduced expression of several genes involved in cell proliferation, survival, invasion, DNA damage repair and angiogenesis. Conversely, in agreement with the oxidative stress hypothesis, it has been recently shown that EPA is able to sensitise U251 human glioblastoma cells to radiation by a mechanism involving an enhancement of lipid peroxidation in treated cells( Reference Manda, Kriesen and Hildebrandt 112 ).
Table 7 Cancer cells of different originFootnote * in vitro: effects of n-3 PUFA in combination with conventional antineoplastic drugs

PDT, photodynamic therapy; ROS, reactive oxygen species; NCG/VCR1, vincristine-resistant NCG cells; Δψm, mitochondrial membrane potential; PRKDC, protein kinase, DNA-activated, catalytic polypeptide; PIK3R1, phosphoinositide-3-kinase, regulatory subunit 1; MAPK14, mitogen-activated protein kinase 14; NF-κB1, NF-κB light polypeptide gene enhancer in B-cells 1; NF-κBIA, NF-κB inhibitor α; 5-FU, 5-fluorouracil; FAS, fatty acid synthase.
* Other than colon, breast, prostate, leukaemia, lymphoma and myeloma cells.
Moreover, a synergistic activity of DHA and COX-2 inhibitors (celecoxib and indomethacin) combinations was observed( Reference Chiu, Tong and Ooi 113 ) in human melanoma cells. Similarly, the synergism of 5-FU and DHA combination was reported in human gastric carcinoma cells( Reference Zhuo, Zhang and Mu 114 ), where the combination induced apoptosis by modulating the expression of several apoptosis-related factors, including Bax, Fas, Bcl-2, Bcl2L12 and caspase-9.
On the whole, it can be concluded that most of the studies investigating the beneficial effects of n-3 PUFA in combination with antineoplastic agents in vitro indicate increased lipid peroxidation and oxidative stress as the main mechanisms involved. Other studies, however, and mainly the most recent ones, identified also other more specific molecular pathways. Certainly, the possibility that the oxidative stress-related cytotoxicity induced by n-3 PUFA may exalt the effect of antineoplastic agents that by themselves are inducers of oxidative stress (doxorubicine, taxanes, radiation) is easy to understand. However, in our opinion, it would be recommendable, when investigating mechanisms in vitro, not to use high concentrations of n-3 PUFA (i.e. concentrations that are able to produce maximal or near-maximal cytotoxicity) to avoid cytotoxicity exclusively related to necrosis due to high levels of oxidative stress. Conversely, low levels of ROS production and oxidative stress may function as specific intracellular signals that may induce plenty of cellular pathways involved in apoptosis, differentiation, autophagy and other cellular processes related to cell growth. Moreover, in future studies it would be important to focus also on specific effects that n-3 PUFA may exert on molecular pathways already involved in the sensitivity or resistance to specific antineoplastic agents used in the combinations under study.
In vivo animal studies
A great effort has also been expended in studying the efficacy of n-3 PUFA in combination with conventional antineoplastic drugs in animal models. We will analyse in the two following paragraphs the results obtained by the animal models mainly used, that is, human cancer cells transplanted in nude mice (or murine tumours transplanted in syngeneic rats or mice), and chemically induced carcinogenesis in rats.
Cancer cells transplanted in animals
Several studies have contributed to demonstrate that the addition of FO or LC-n-3 PUFA to the diet of animals following tumour transplantation increases the efficacy of conventional cancer chemotherapy. Most of them were performed using human breast cancer cells xenografted in nude mice (Table 8), and associated the decreased tumour rate with an altered oxidative status and increased lipid/protein peroxidation( Reference Hardman, Barnes and Knight 115 – Reference Shao, Pardini and Pardini 117 ) (for further details, see Table 8). In some of the studies( Reference Hardman, Moyer and Cameron 118 , Reference Huan, Cui and Teng 119 ) the mechanisms underlying the improved anti-tumour effect of the combination studied were not investigated. However, in another study( Reference Shao, Pardini and Pardini 120 ) performed by combining cyclophosphamide and a FO (menhaden oil) it was observed that the improved anti-tumour effect was related to the increased activity of one of the key cyclophosphamide-activating enzymes, CYP2B1, found significantly enhanced in the liver and tumour of menhaden oil-treated animals. It was suggested that this effect could be related to the formation of more pharmacologically active drug metabolites that could enhance cyclophosphamide anti-tumour response. Interestingly, FO-containing diets were also found to reduce deleterious intestinal, haematological and cardiac side effects of various chemotherapeutic treatments( Reference Shao, Pardini and Pardini 120 – Reference Germain, Lavandier and Chajès 122 ). It has also been shown that the chemical binding of the n-3 PUFA linolenic acid to the moiety of one of the chemotherapic drugs (doxorubicin) confers to it tumour specificity( Reference Huan, Cui and Teng 123 ), thus making linolenic acid a good candidate for possible use as a targeting molecule also for other chemotherapic agents.
Table 8 Animals implanted with cancer cells: effects of n-3 PUFA in combination with conventional antineoplastic drugs

s.c., Subcutaneous; FO, fish oil; i.v., intravenous; FOC, fish oil concentrate; GPx, glutathione peroxidase; SOD, superoxide dismutase; i.p., intraperitoneal; CAT, catalase; GSTPx, glutathione S-transferase peroxidise; DOX-LNA, doxorubicin–α-linolenic acid conjugate; ADH, alcohol dehydrogenase.
The remaining in vivo transplantation models were mostly performed by injecting murine colon and lung cancer cells, or murine leukaemia cells in syngeneic animals treated with FO or EPA and/or DHA( Reference Wynter, Russell and Tisdale 124 – Reference Barnés, Prox and Christison-Lagay 130 ) (Table 9). Again, in two of these studies( Reference Xue, Le Roy and Sawyer 127 , Reference Yam, Peled and Shinitzky 128 ) enhanced oxidative stress and lipid peroxidation were involved, and several other studies( Reference Wynter, Russell and Tisdale 124 – Reference Cha, Meckling and Stewart 126 ) were only observational and, even though they found a positive effect of n-3 PUFA combined with epothilone, 5-FU, cyclophosphamide, doxorubicin or arabinosylcytosine, they did not investigate the possible molecular mechanisms involved.
Table 9 Animals implanted with cancer cells: effects of n-3 PUFA in combination with conventional antineoplastic drugs

s.c., Subcutaneous; EPO, epothilone; GCB, gemcitabine; 5-FU, 5-fluorouracil; CYC, cyclophosphamide; i.v., intravenous;. AraC, arabinosylcytosine; i.p., intraperitoneal; CPT-11, irinotecan; FO, fish oil; GSH, reduced glutathione; GSSG, oxidised glutathione; PLA2, phospholipase A2.
An interesting therapeutic approach was the covalent binding of DHA to different anti-tumour drugs. In a model of implanted lung cancer DHA was covalently conjugated to paclitaxel( Reference Bradley, Webb and Anthony 131 ). The conjugate, besides being less toxic with respect to paclitaxel alone, was able to induce a complete tumour regression, while paclitaxel alone induced only a partial regression. Since DHA–paclitaxel remained in tumours for long times at high concentrations, and it was slowly converted to the cytotoxic metabolites of paclitaxel, it was suggested that the conjugate could also kill residual tumour cells that eventually re-enter the cell cycle. Similarly, an enhanced tumour sensitivity was observed by Wang et al. ( Reference Wang, Li and Jiang 132 ) when 10-hydroxycamptothecin was conjugated with DHA and administered to mice bearing Lewis lung carcinoma, L1210 leukaemia and 38 adenocarcinoma cells.
Chemically induced carcinogenesis
The possible adjuvant effect of n-3 PUFA in the therapy of breast cancer has been investigated also using the N-methylnitrosourea-induced carcinogenesis model in rats (Table 10). These studies were performed using radiation or drugs that act mainly through a pro-oxidant effect, and this is probably the reason why the main mechanisms found to be associated with the enhanced anti-tumour effects induced by n-3 PUFA were the altered oxidative status and/or lipid peroxidation( Reference Colas, Mahéo and Denis 133 – Reference Hajjaji, Besson and Bougnoux 136 ). It should be noted that this mechanism was observed either treating the animals with quite low doses of n-3 PUFA (about 0·7 g/d DHA, see Colas et al.( Reference Colas, Mahéo and Denis 133 )), or with doses several times higher (about 4·5 g/kg EPA+DHA, present in the dose of FO administered by Manni et al. ( Reference Manni, Xu and Washington 134 , Reference Manni, Richie and Xu 137 ) (see United States Environmental Protection Agency( 138 ) for the calculation of the exact doses of LC-n-3 PUFA administered).
Table 10 Animal models of experimental carcinogenesis: effects of n-3 PUFA in combination with conventional antineoplastic agents

NMU, N-methylnitrosourea; s.c., subcutaneous; i.p., intraperitoneal; FO, fish oil; GSH, reduced glutathione; i.v., intravenous; GPx, glutathione peroxidise.
The hypothesis that a pro-oxidant mechanism was involved also when n-3 PUFA were supplemented in combination with radiation( Reference Colas, Paon and Denis 135 ) was also supported by the finding that the addition of vitamin E to the DHASCO diet inhibited the beneficial effect of DHA in rats bearing breast cancer( Reference Colas, Paon and Denis 135 ). In a more recent study( Reference Manni, Richie and Xu 137 ) performed by supplementing FO alone or in combination with tamoxifen the authors evaluated systemic and preneoplastic mammary gland biomarkers known to play a key role in the progression to invasive cancer. They found that the FO-rich diets significantly reduced Ki67 expression in hyperplastic lesions, but in this case it was found that dietary FO and/or tamoxifen did not modify systemic oxidative stress biomarkers or lipid peroxidation. On the other hand, the authors observed that in the FO-fed rats the tissue levels of 8-isoprostane were even markedly reduced. Thus, they hypothesised that the effect was related to the FO-induced AA depletion in the mammary gland, and suggested that the protective effect of n-3 PUFA in this experimental model was not mediated by changes in the levels of oxidative stress but by the suppression of AA-specific pathways( Reference Manni, Richie and Xu 137 ).
Moreover, in a study( Reference Hajjaji, Couet and Besson 139 ) a DHA-enriched diet was reported to significantly reduce the doxorubicin-induced body weight loss, a finding very frequently observed in cancer patients with advanced disease and associated with a poor prognosis.
In conclusion, our recommendation for future combination studies in animals is to choose n-3 PUFA doses placed in the lower range (2–8 g/kg body weight EPA+DHA) of all the dosages used so far( Reference Fasano, Serini and Cittadini 140 ). As a matter of fact, we recently examined( Reference Fasano, Serini and Cittadini 140 ) all the doses of n-3 PUFA used in animal studies and concluded that, in more recent times, similarly to what happened for the clinical studies, there has been a tendency to use doses comprised in the lower range. These lower doses do not induce tissue cytotoxicity and necrosis, often driven by high levels of lipid peroxidation and oxidative stress, but could modulate in a more specific way crucial molecular pathways/receptors related to inflammation, cell growth, differentiation, angiogenesis and cell invasion( Reference Fasano, Serini and Cittadini 140 ).
Clinical studies
Despite the strong evidences of the beneficial antineoplastic effect of n-3 PUFA in combination with conventional antineoplastic agents both in cell culture and animal studies, the number of clinical trials performed so far is still extremely small (Table 11, Table 12 and Table 13). We are not examining here the possible reasons as for why more clinical trials have not been performed, having comprehensively discussed this important topic in several previous reviews of ours( Reference Serini, Innocenti and Piccioni 141 , Reference Calviello and Serini 142 ). Moreover, besides the scarce number, most of the trials performed so far did not address the possible ability of n-3 PUFA to increase the efficiency of the drug/radiation therapy administered simultaneously. On the contrary, they investigated the potential of the dietary n-3 PUFA supplementations to reduce cachexia in advanced stages of cancer, or the toxic side-effects of the antineoplastic treatments. For instance, four clinical studies( Reference van der Meij, Langius and Smit 143 – Reference Murphy, Mourtzakis and Chu 146 ) conducted in patients with advanced non-small cell lung cancer (NSCLC) fall in this category. The first one( Reference van der Meij, Langius and Smit 143 ) (Table 11) was a double-blind study conducted in stage III NSCLC patients receiving a multimodality treatment in order to investigate the effects of an oral nutritional supplement containing both EPA and DHA on nutritional status and inflammatory markers in patients. The results were promising, since the oral n-3 PUFA-containing supplement resulted in the preservation of body weight and fat-free mass during chemotherapy (Table 11). The effects were directly related to the supplementation, since this effect was particularly evident in those patients showing an increased plasma phospholipid EPA concentration, which was assessed as an objective marker of compliance with the intervention. Moreover, the n-3 PUFA-supplemented group showed lower levels of the circulating pro-inflammatory cytokine IL-6. Similar results were obtained in another multicentre, randomised, double-blind trial( Reference Finocchiaro, Segre and Fadda 144 ) where NSCLC patients undergoing chemotherapy were treated with an oral supplementation of EPA and DHA for about 2 months. Also in this case n-3 PUFA exerted anti-inflammatory effects by inducing a progressive decrease in C-reactive protein (CRP) and IL-6 levels during chemotherapy. Moreover, they inhibited the formation of ROS and lipid peroxidation products taking place in plasma during chemotherapy. Another human study( Reference Murphy, Mourtzakis and Chu 145 ), performed in patients with NSCLC from the time of initiation to the completion of first-line chemotherapy, evaluated the effects of intervention with an oral supplementation with FO (or gelatin capsules containing the same amount of EPA present in the FO) in combination with standard chemotherapy (carboplatin/vinorelbine or carboplatin/gemcitabine). Body weight, skeletal muscle and adipose tissue were examined (Table 11), and it was demonstrated that the patients in the FO/EPA group were able to maintain their body weight, while the control group showed an average weight loss of 2·5 kg throughout the chemotherapy period. Also in this case, patients showing the highest increase in plasma EPA concentration after supplementation had the greatest gain in muscle mass. Only one study in patients with advanced NSCLC( Reference Murphy, Mourtzakis and Chu 146 ) had the objective to evaluate whether combining chemotherapy (carboplatin with vinorelbine or gemcitabine) with a dietary supplementation with FO could increase the effect of chemotherapy. It was observed that in the FO patients the chemotherapy efficiency increased, as demonstrated by the significant increase in the One-Year Survival Index and in the two parameters Response Rate and Clinical Benefit (both calculated on the basis of the complete response, partial response, and stable disease after four cycles of chemotherapy). An analogous trial( Reference Trabal, Leyes and Forga 147 ) was performed in patients diagnosed with stage IV colorectal cancer receiving standard chemotherapy and supplemented with EPA (Table 11). It had the primary goal to evaluate if the dietary EPA could avoid the side effects of the therapy. Thus, the patients were assessed for their nutritional status, dietary intake and chemotherapy compliance. In this case, even though we have to underline that the sample of patients enrolled was very small (thirteen patients in total), the results unequivocally showed that only those receiving the EPA supplement significantly increased their body weight. Instead, the evaluation of their quality of life remained unchanged. Interestingly, however, the patients receiving only the standard chemotherapy had to interrupt the treatment for its high toxicity, whereas the EPA-supplemented group continued the treatment, thus suggesting the positive effect of EPA on chemotherapy tolerability. Nevertheless, for the very small number of patients, this study would need confirmation by a larger controlled randomised trial.
Table 11 Human clinical trials: effects of n-3 PUFA in combination with conventional antineoplastic drugs

NSCLC, non-small cell lung cancer; CIS, cisplatin; DTX, docetaxel; BV, bevacizumab; REE, resting energy expenditure; GCB, gemcitabine; CRP, C-reactive protein; ROS, reactive oxygen species; CBCDA, carboplatin; VNB, vinorelbine; VP-16, etoposide; FO, fish oil; 5-FU, 5-fluorouracil; OXA, oxaliplatin; FOL, folinic acid; CAP, capecitabine; GHS/QoL, global health status/quality of life.
Table 12 Human clinical trials: effects of n-3 PUFA in combination with conventional antineoplastic drugs

5-FU, 5-fluorouracil; LCV, leucovorin; i.v., intravenous; FO, fish oil; PMNC, polymorphonuclear cells; CAP, capecitabine; OXA, oxaliplatin; CRP, C-reactive protein; ARA, arachidonic acid; CCRT, concurrent chemoradiotherapy; CIS, cisplatin; CYC, cyclophosphamide; EPI, epirubicin.
Table 13 Human clinical trials: effects of n-3 PUFA in combination with conventional antineoplastic drugs

PTX, paclitaxel; GCB, gemcitabine; OS, overall survival; PFS, progression-free survival; PDGF, platelet-derived growth factor; FGF, fibroblast growth factor; CCRT, concomitant chemotherapy; CIS, cisplatin; CBCDA, carboplatin; MMC, mytomicin C; 5-FU, 5-fluorouracil; CY, cytarabin; IDA, idarubicin; DRC, daunorubicin; EP, etoposide phosphate; MXT, mitoxantron; BV, bevacizumab; FO, fish oil.
Beneficial effects on the general health conditions of cancer patients dietary supplemented with FO in combination with standard chemotherapy after surgical removal of several kinds of tumours (mainly gastrointestinal) were also recently reported by Bonatto et al. ( Reference Bonatto, Oliveira and Nunes 148 ) (Table 12). In this case, the authors analysed neutrophil number and functions and observed that the FO supplementation was able to prevent the chemotherapy-induced decrease in these parameters( Reference Bonatto, Oliveira and Nunes 148 ), as well as cachexia-induced body-weight loss. In agreement, a randomised, prospective, controlled clinical trial( Reference Mocellin, Pastore e Silva Jde and Camargo Cde 149 ) recently performed demonstrated that the dietary FO supplementation administered to colorectal cancer patients for 9 weeks was able to significantly improve CRP values, CRP/albumin status, and plasma FA profile during chemotherapy. These findings are of interest, since they further support the hypothesis that an increased intake of n-3 PUFA may suppress the permanent serum inflammatory status and the high serum levels of inflammatory cytokines observed in cancer patients, and related to cachexia and poor prognosis( Reference Szkaradkiewicz, Marciniak and Chudzicka-Strugała 150 , Reference Chung and Chang 151 ). Moreover, whereas the FO patients gained body weight, those receiving only chemotherapy lost it, even though the difference did not reach significance (P=0·72). Again, however, this study, even though highly promising, had the main limitation of having enrolled a very small number of patients (only eleven patients). However, a further confirmation of the anti-inflammatory effect of n-3 PUFA during chemotherapy came from a larger recent randomised controlled trial (Table 12) performed on patients with oesophageal squamous cell carcinoma treated with chemo- (5-FU and cisplatin) and radiotherapy( Reference Sunpaweravong, Puttawibul and Ruangsin 152 ), and supplemented with a combination of n-3 FA, glutamine and arginine. Also in this case a significant decrease in the levels of serum CRP and TNF-α was reported in patients receiving enteral nutrition providing PUFA.
n-3 PUFA supplementation has also been investigated in breast cancer patients with visceral metastases and treated with an anthracycline-based chemotherapy( Reference Bougnoux, Hajjaji and Ferrasson 153 ) (Table 12). The trial was one small (twenty-five patients) open-label single-arm phase II study analysing as the primary outcome the efficacy of an oral DHA supplementation. They found that DHA, besides not inducing adverse side effects, increased both the time to progression (P=0·02) and the overall survival (P=0·007) of patients with respect to the control group, and the effects were particularly remarkable in the women showing the highest DHA incorporation into plasma phospholipids. Certainly, as the authors themselves stated, this study also warrants confirmation through a larger placebo-controlled randomised phase III clinical trial stratified on patients’ DHA incorporation.
Instead, in another randomised double-blind placebo-controlled trial, breast cancer patients were subjected to paclitaxel-based chemotherapy and supplemented with FO all through the chemotherapy treatment, as well as 1 month after the end of therapy (Table 13). The authors found a significantly decreased incidence of chemotherapy-induced peripheral neuropathy in n-3 FA-supplemented patients (P=0·029)( Reference Ghoreishi, Esfahani and Djazayeri 154 ). Even though also this study examined a small sample (fifty-seven patients), this result is very promising since, in spite of the increased survival rate of breast cancer patients due to the recent development of new therapeutic strategies, peripheral neuropathy associated with paclitaxel chemotherapy often impairs patients’ quality of life and induces oncologists to change or even to stop the treatment( Reference Chaudhry, Rowinsky and Sartorius 155 ). Likewise, another interesting finding was obtained in a recent randomised, double-blind, placebo-controlled pilot study (thirty-eight patients) performed in postmenopausal breast cancer survivors after chemotherapy with aromatase inhibitors. The authors demonstrated that a short-term, high-dose n-3 PUFA-rich FO supplementation was able to significantly reduce bone resorption (P<0·05)( Reference Hutchins-Wiese, Picho and Watkins 156 ). This effect is interesting since, together with decreased bone mineral density, bone resorption represents an undesired side-effect induced by aromatase inhibitors in breast cancer patients( Reference Santen 157 ).
Also patients with advanced pancreatic cancer were treated with gemcitabine and intravenous (i.v.) n-3-rich Lipidem® emulsion (B Braun, Melsungen) in a single-arm phase II trial (thirty-two patients)( Reference Arshad, Chung and Steward 158 ).The authors observed that the serum concentrations of platelet-derived growth factor and fibroblast growth factor decreased significantly with treatment over time. This finding, in spite of the small number of patients examined, is of note since increased serum concentrations of these pro-angiogenic factors have been correlated with the development of most human solid tumours( Reference Rahbari, Reissfelder and Mühlbayer 159 ). Moreover, these changes were associated with an improved overall survival and a significantly higher progression-free survival. Positive results were also obtained in a recent and larger multicentre study (Table 13), performed on head and neck and oesophageal cancer patients (111 patients)( Reference Fietkau, Lewitzki and Kuhnt 160 ). They were treated with enteral n-3 nutrition and concurrent chemo-radiotherapy, and a significant improvement in the nutritional status (evaluated as Kondrup score; P=0·0165) was observed (Table 13).
Conversely, significant differences were not found between the control and n-3 groups in one pilot phase II trial recently performed to investigate the protective effect that an i.v. supplementation of n-3 PUFA may exert in reducing the incidence and severity of enterocolitis developing in thirteen patients with acute myeloid leukaemia or at high risk of myelodysplastic syndrome undergoing myeloablative chemotherapy( Reference Bükki, Stanga and Tellez 161 ) (Table 13). In this case, an inverse probability of treatment-weighted comparison with a historical control group of consecutive AML patients was performed. The authors found that the CI of incidence rate ratios of serious adverse events comparing experimental and control were wide. Therefore, they were not able to exclude a clinically relevant increase or decrease in the rate of serious adverse events. However, the authors themselves suggested that more adequately designed randomised trials of moderate to large size could better determine whether the intervention with n-3 PUFA may be beneficial.
Certainly, from the analysis of all the trials performed so far, it is impressive to notice how the methods used to enrich cells and tissues of cancer patients with n-3 PUFA (in the form of dietary supplements, i.v. inoculation or enteral nutrition) are absolutely heterogeneous, making the comparison between the outcomes very complicated. Moreover, most of the studies are small and for this reason await confirmation by future larger studies. However, in spite of these limitations, and although the number of these human clinical trials remains quite scarce, most of the results analysed strongly suggest multiple advantages deriving from adding n-3 PUFA to anticancer therapies. In particular, some of these results suggest that this therapeutic approach could be extremely useful in order to: (a) reduce weight loss and cachexia in cancer patients receiving chemotherapy( Reference van der Meij, Langius and Smit 143 – Reference Murphy, Mourtzakis and Chu 145 , Reference Trabal, Leyes and Forga 147 , Reference Bonatto, Oliveira and Nunes 148 , Reference Fietkau, Lewitzki and Kuhnt 160 ); (b) decrease inflammation related to either the cancer condition or the antineoplastic therapy( Reference van der Meij, Langius and Smit 143 , Reference Finocchiaro, Segre and Fadda 144 , Reference Mocellin, Pastore e Silva Jde and Camargo Cde 149 , Reference Sunpaweravong, Puttawibul and Ruangsin 152 ); (c) enhance the tolerability of highly cytotoxic chemotherapeutic drugs( Reference Trabal, Leyes and Forga 147 , Reference Bonatto, Oliveira and Nunes 148 , Reference Ghoreishi, Esfahani and Djazayeri 154 , Reference Hutchins-Wiese, Picho and Watkins 156 ); and (d) improve the efficiency of the antineoplastic treatments( Reference Murphy, Mourtzakis and Chu 146 , Reference Bougnoux, Hajjaji and Ferrasson 153 , Reference Arshad, Chung and Steward 158 ).
DHA–paclitaxel conjugate studies
Finally, we cannot omit from our analysis another therapeutic approach used in some clinical trials, which involves the use of DHA–paclitaxel, a conjugate molecule synthesised by covalently linking DHA to the chemotherapeutic drug paclitaxel. One of these studies( Reference Wolff, Donehower and Carducci 162 ) was a phase I trial that examined the toxicity and pharmacokinetics of DHA–paclitaxel in patients bearing different kinds of advanced refractory solid tumours. The patients were treated with DHA–paclitaxel as a 2 h i.v. infusion every 3 weeks at doses ranging from 200 to 1100 mg/m2. It was demonstrated that DHA–paclitaxel was able to strongly increase the pharmacokinetics of paclitaxel alone at the dose of 175 mg/m2. Moreover, the authors found that the DHA–paclitaxel conjugate was less cytotoxic in terms of neutropenia, alopecia and peripheral neuropathy. Similarly, another phase I clinical trial( Reference Fracasso, Picus and Wildi 163 ) demonstrated that DHA–paclitaxel administered weekly to a maximum dose of 600 mg/m2 was well tolerated, and that a weekly continuous infusion was more active than a treatment performed with the taxane alone every 3 weeks or weekly. A prospective, open-label, non-randomised, multi-institutional phase II study( Reference Payne, Ellis and Dunlop 164 ) was also performed to assess the anti-tumour activity and safety of DHA–paclitaxel (900 and 1100 mg/m2, as i.v. infusion every 3 weeks) as first-line treatment of patients with advanced NSCLC. However, in this case DHA–paclitaxel administered as a single-agent showed little activity in patients with advanced NSCLC, with about 40 % patients achieving either stable disease or a partial response after treatment. A phase II open-label study( Reference Jones, Hawkins and Eatock 165 ) was also performed using DHA–paclitaxel administered at the dose of 1100 mg/m2 by i.v. infusion every 21 d in patients with locally advanced or metastatic adenocarcinoma of the oesophagus, oesophago-gastric junction or stomach, which had not been previously treated. However, the results demonstrated that the DHA–paclitaxel treatment induced only a partial response (9·4 %), and that the haematological toxicity was comparable with that induced by paclitaxel alone (neutropenia in 93 % of patients). Three similar clinical studies( Reference Homsi, Bedikian and Kim 166 – Reference Homsi, Bedikian and Papadopoulos 168 ) were also conducted in melanoma patients. In particular, Homsi et al. ( Reference Homsi, Bedikian and Kim 166 ) administered DHA–paclitaxel (900 mg/m2) or dacarbazine (1000 mg/m2) every 3 weeks in metastatic melanoma patients not treated before, and found that its efficacy was not higher than that of dacarbazine, even though DHA–paclitaxel was well tolerated. Similarly, Bedikian et al. ( Reference Bedikian, DeConti and Conry 167 ), in a phase III study in patients bearing a metastatic malignant melanoma and treated with DHA–paclitaxel or dacarbazine, did not find any difference in the patient survival and response rate between the two treatments. Actually, the authors found higher myelosuppression in patients receiving DHA–paclitaxel. Finally, a phase II open-label study( Reference Homsi, Bedikian and Papadopoulos 168 ) was conducted by treating patients with metastatic uveal melanoma (previously treated or not with standard chemotherapy) with DHA–paclitaxel at the dose of 500 mg/m2 per week for 5 consecutive weeks. As a single-agent therapy, DHA–paclitaxel was found to be safe and well tolerated, but its efficacy was observed only in 32 % of patients achieving stable disease. In conclusion, the outcomes of the clinical studies performed with the DHA–paclitaxel conjugate appear controversial with respect to both the preclinical studies and the few clinical studies in which n-3 PUFA were given in combination with other chemotherapeutic agents or with paclitaxel itself( Reference Ghoreishi, Esfahani and Djazayeri 154 ), but not as a DHA conjugate. It should be noticed, however, that the reason for covalently binding DHA to paclitaxel in the conjugate was not for taking advantage of the antineoplastic properties of DHA, but just for enhancing the cellular uptake of paclitaxel, thanks to the lipid carrier function of DHA, and for making slower the paclitaxel release inside the cells. For this reason, in the trials performed with the DHA–paclitaxel conjugate, the treatment scheme was quite unusual for a nutritional compound such as DHA. As a matter of fact, in these studies DHA was always administered at concentrations much lower and at intervals between administrations much longer than those shown to be effective in the other clinical trials where n-3 PUFA supplementations were combined to concomitant, but separate, treatments with a variety of antineoplastic agents.
Conclusions
Overall, all the data analysed here concur to support the hypothesis that n-3 PUFA used in combination with various conventional antineoplastic agents may exert beneficial effects, either by increasing the efficacy of the concomitant therapies, and/or by reducing their deleterious side-effects. The potentiating action of n-3 PUFA has been widely demonstrated both in in vitro and animal studies, thus attracting considerable interest. Several human studies have recently confirmed their ability to sensitise cancer cells to the action of chemical and radiation therapy, even though their number appears still too small to draw definitive conclusions. Moreover, the number of patients enrolled in the clinical trials is often too limited. This highlights the need to undertake further studies evaluating potential molecular targets for each type of cancer and anti-cancer agent studied in combination with n-3 PUFA. Finally, it could also be hypothesised a therapy combining these FA, which is safe, quite inexpensive, and provided with pleiotropic effects and multi-targeted action at molecular levels, with the innovative single-targeted cancer therapies, which, instead, are very expensive and, as recently observed, not completely devoid of dangerous side-effects( Reference Thornton, Howard and Jagannathan 169 , Reference Ederhy, Izzedine and Massard 170 ).
Acknowledgements
The present review was supported in part by grants D.1 2013 and D.1 2014, and D.3.2 2013 to G. C. from ‘Università Cattolica del S. Cuore’, Rome, Italy, within its programme of promotion and diffusion of scientific research.