Introduction
Adequate protein intake is required for the maintenance of whole-body protein mass. The protein mass in a 70 kg adult is about 11 kg. Whole-body protein mass maintenance relies on equal rates of protein synthesis and breakdown, resulting in a zero net balance. The constant kinetics of protein synthesis and breakdown of body proteins were originally demonstrated by Schoenheimer and colleagues in 1939(Reference Schoenheimer, Ratner and Ritten-Berg1) and were termed protein turnover, which is a modifiable and adaptable process. In adult humans, the daily whole-body protein turnover rate is 5·7 g/kg body weight(Reference Waterlow2), meaning that approximately 400 g mixed proteins are turned over every day for a 70 kg adult individual. Behind that number, a large proportion of amino acids are recycled and reutilised for protein synthesis(Reference Wolfe3–Reference Nishimura, Musa and Holm5), whereas some are lost via oxidation for energy production and the formation of urea to scavenge nitrogen(Reference Wolfe3). Further, nitrogen-containing substances, such as skin, hair, sweat, urine and faeces, are lost from the body. To maintain whole-body protein mass, irreversible loss of amino acids and nitrogen needs to be reconstituted via dietary protein, which founds the basis for the recommended dietary protein intake.
It is well established how much dietary protein should be ingested to account for obligatory nitrogen loss in healthy adults using nitrogen balance methodology(6,Reference Rand, Pellett and Young7) . However, it has continuously been debated(Reference Traylor, Gorissen and Phillips8–Reference Phillips, Paddon-Jones and Layman14) whether increasing dietary protein intake in older age is an effective strategy to counteract the age-dependent loss of muscle mass(Reference Gallagher, Visser and De Meersman15,Reference Janssen, Heymsfield and Wang16) and strength(Reference Goodpaster, Park and Harris17,Reference Delmonico, Harris and Visser18) , a phenomenon termed sarcopenia(Reference Rosenberg19–Reference Bülow, Ulijaszek and Holm21). However, an increase in the dietary protein recommendations for older adults would categorise numerous people as being protein malnourished and thereby increase the incidence of protein malnutrition worldwide. Such a change would challenge nutritional guidelines and nutritional societies and force governments to act with enormous socio-economic consequences. Thus, it is of utmost importance that we adopt a critical approach to the evidence underlying the dietary protein recommendations.
Independent authorities and expert groups have adopted different criteria and evidence from distinct methodological approaches, resulting in differences in published recommended values of protein recommendations. Consequently, there is currently confusion about the requirements of dietary protein for healthy older adults, both in the scientific community and amongst the general older population, who wish to follow the recommendations. Thus, the purpose of this narrative review is to critically discuss the existing evidence for protein requirements founding the basis for protein recommendations for older adults.
Methodology
This narrative review was undertaken by: (1) searching PubMed and Google Scholar using keywords related to each topic; (2) screening reference lists for relevant papers; and (3) searching nutritional societies’ and authorities’ guidelines and references. We specifically included studies conducted in older individuals and only included studies on younger adults when studies in older adults were not available. All articles had undergone peer review and were available in English.
The first part of this review is a summary of the historical development of the protein recommendation with a brief mention of the approaches used and methodologies applied. In the second part, we present and describe in more detail the methodological approaches that found the evidence for determining protein requirements and present the key studies providing data for older adults. Finally, we discuss major factors influencing the requirement for dietary protein and that thus need to be considered in future study designs striving to determine protein requirements. With such consensus, we can accelerate international agreement on protein recommendations for healthy older adults.
Historical development of determining protein requirements and recommendations
The first official recommendation for protein intake of 1·0 g/kg/d, published in 1936 by the League of Nations(22), was set based on observations from practice rather than relying on data from a strict scientific approach. Several joint Food and Agriculture Organization (FAO)/World Health Organization (WHO) expert committees have faced challenges in defining ‘protein requirements’(23). In 1957, FAO/WHO(23) reported their first official protein recommendation, and the latest was reported in 2007(6), which thereafter has been challenged by other expert bodies. Table 1 provides an extensive overview of the WHO/FAO/United Nations University (UNU) recommendations over the years. While the 2007 recommendation defined the protein requirement as being ‘the lowest level of dietary protein intake that will balance the losses of nitrogen from the body, and thus maintain the body protein mass, in persons at energy balance with modest levels of physical activity…’, it is uncertain whether this amount is sufficient to counteract protein loss in catabolic conditions(Reference Wolfe24). Even though the Expert Committee rigorously reassessed all available data on protein balance in older people, it was concluded that no studies unequivocally demonstrate that the protein requirement would be higher in older adults when expressed as protein requirement per kg body weight when the purpose was to maintain whole-body protein balance.
Table 1. Successive protein requirements and recommendations by international groups to ensure nitrogen balance in adults
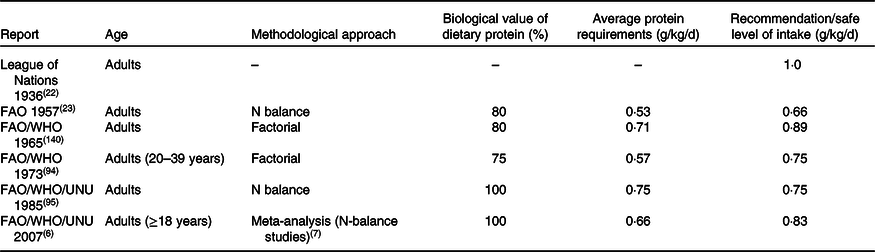
Adopted from NS Scrimshaw(Reference Scrimshaw141) and updated.
Recently, this has led to some expert bodies challenging the protein recommendations for older adults. Table 2 summarises major guidelines from external expert bodies, which, however, are not official recommendations. Although our understanding of protein requirements and recommendations has improved over these 60 years, the outcome measurements, and the methodologies on which the outcome measurement is founded, have not evolved. The discrepancy in protein recommendations is partly due to the lack of a definitive criterion for ‘adequate protein’, as well as differences in outcome measurements will be discussed. Then, important factors that can impact protein requirements will be reviewed.
Table 2. Protein recommendations by expert bodies to maintain muscle mass and strength in healthy older adults

Generation of evidence for protein recommendations for older adults
Protein/nitrogen balance studies
The nitrogen balance methodology has been used most widely and is considered ‘gold standard methodology’ to establish the protein requirement(6,Reference Rand, Pellett and Young7) . Whole-body nitrogen balance is determined by measuring or estimating all nitrogen intake (protein intake) and all excretion and loss (skin, hair, sweat, urine and faeces). Positive balance means excess nitrogen intake relative to losses (i.e. protein accretion within the body). Two crucial prerequisites for a valid measurement are: (1) metabolic adaptation to any given amount of daily protein intake before conducting the measurements(Reference Millward, Price and Pacy25–Reference Price, Halliday and Pacy27); and (2) the achievement of energy balance during the period of measurement(Reference Rand, Pellett and Young7). However, the nitrogen balance methodology has inherent shortcomings: it has been criticised for insufficient sensitivity (i.e. inability to detect small differences between nitrogen intake and excretion)(Reference Young28). Further, the overestimation of nitrogen intake through food and underestimation of nitrogen loss can lead to erroneous balance measurements.
While nitrogen balance data provide limited mechanistic insight(Reference Young28,Reference Young, Yu and Fukagawa29) , Waterlow and colleagues proposed in 1977(Reference Waterlow, Golden and Picou30) that a relationship between nitrogen balance and protein balance could be expressed at steady state as: flux (Q) = protein synthesis (S) + nitrogen oxidations/excretion (O) = protein breakdown (B) + nitrogen input (I) → Q = I − O = S − B.
Protein balance calculated as the difference between protein synthesis and breakdown rates provides information about the underlying dynamics of protein kinetics. Protein balance is the rate of either gain or loss of protein per unit time and indicates whether protein mass in the body will quantitatively change over time. Therefore, the prolonged readout of the protein balance data is whole-body protein mass and/or changes herein. Whole-body protein mass, often determined by the surrogate measure of muscle mass, is determined in another set of scientific studies, namely epidemiological studies.
Epidemiological studies of protein intake
Epidemiological studies, such as cross-sectional and prospective cohort studies, can be used for assessing the association between habitual dietary intake patterns and whole-body/muscle mass(Reference Asp, Richardson and Collene31–Reference Sahni, Mangano and Hannan42). Epidemiological studies have the strength that adaptation to a given protein intake level would be inherent, yet they often suffer from several confounding factors, limiting the translation into other settings and making it impossible to use the results for assessing protein requirements. Longitudinal intervention studies can be applied to examine the cause–effect relationship and/or dose–response relationship between protein intake and most often physiological and functional outcomes, such as muscle mass, muscle strength and physical function, which is particularly relevant to older adults(Reference Kerstetter, Bihuniak and Brindisi43–Reference Mertz, Reitelseder and Bechshoeft48).
Cross-sectional studies
The role of protein intake in the maintenance of muscle mass has been investigated across the lifespan in numerous studies (Table 3). A cross-sectional study from the Framingham Offspring Cohort showed a positive association between the total daily and total animal protein intake and muscle mass after adjustment of physical activity and energy intake(Reference Sahni, Mangano and Hannan42). The association between protein intake and muscle mass became apparent when dividing the cohort into quartiles of protein intake. In men, the difference appeared between the highest (101·1 g/d) and the lowest quartile (64·9 g/d) for total protein intake. In women, muscle mass differed between the highest and the second-lowest quartile for total protein and animal protein intake (93·4 g/d versus 63·1 g/d)(Reference Sahni, Mangano and Hannan42). Mangano et al.(Reference Mangano, Sahni and Kiel39) examined the third-generation offspring of the original Framingham Heart Study. They divided the cohort into four quartiles based on their protein intake, from the lowest intake (quartile 1) 59 g/d (0·8 g/kg/d) to the highest intake (quartile 4) 129 g/d (1·8 g/kg/d). After adjustment for various confounders, including physical activity and energy intake, they found a positive association between protein intake and appendicular lean mass index. A difference was found between the first and the second quartile (80 g/d or 1·1 g/kg/d). However, there was no further difference between the first quartile and the third (99 g/d or 1·3 g/kg/d) and the fourth quartile (129 g/d or 1·8 g/kg/d)(Reference Mangano, Sahni and Kiel39). Collectively, cross-sectional observational studies suggest that protein intake around the international recommendation (0·8 g/kg/d, 60–65 g/d in average-sized individuals, 75–80 kg) is sub-optimal for the maintenance of muscle mass throughout adult life. They also suggest that muscle mass may be better maintained when protein intake is higher (80 g/d or 1·1 g/kg/d) than the international recommendation (0·83 g/kg/d), and that no further beneficial effects on muscle mass are observed beyond this amount, suggesting a non-linear association above this level of intake.
Table 3. Selected cross-sectional observational studies assessing the association between protein intake and muscle mass
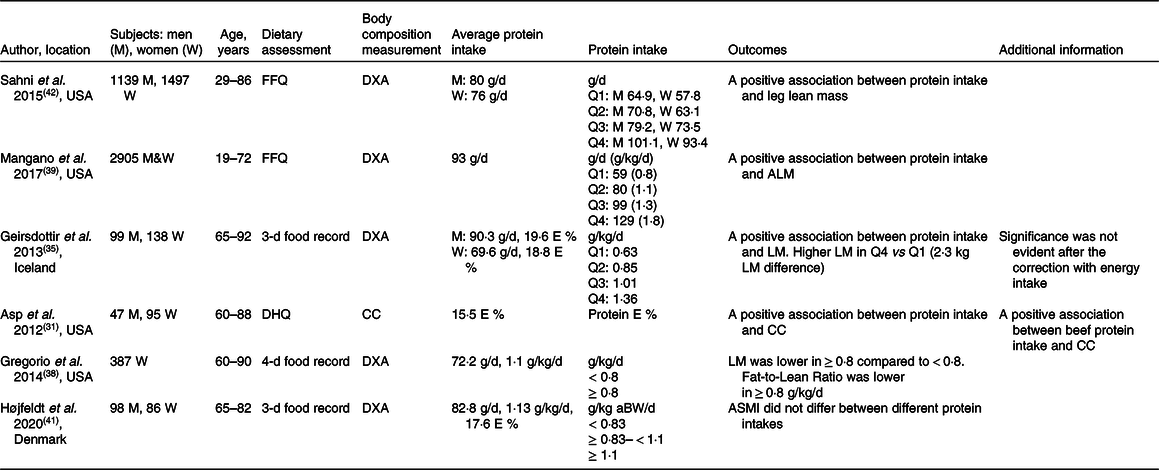
FFQ, food-frequency questionnaire; E, energy; DXA, Dual-energy X-ray absorptiometry; Q, quartile; DHQ, diet history questionnaire; CC, calf circumference; aBW, adjusted body weight; LM, lean body mass; ALM, appendicular lean body mass; ASMI, appendicular skeletal muscle index.
Cross-sectional observational studies including healthy older adults have provided insight into the role of dietary protein intake in the maintenance of muscle mass(Reference Asp, Richardson and Collene31,Reference Geirsdottir, Arnarson and Ramel35,Reference Gregorio, Brindisi and Kleppinger38,Reference Højfeldt, Nishimura and Mertz41) (Table 3). A positive association was observed between protein intake and muscle mass(Reference Asp, Richardson and Collene31,Reference Geirsdottir, Arnarson and Ramel35) . In contrast, it was recently reported that muscle mass did not differ between total daily protein intakes < 0·83, ≥ 0·83 < 1·1, and ≥ 1·1 g/kg adjusted body weight in healthy Danish older individuals (≥65 years.), but this might be due to the small sample size (n = 25) in protein intake below 0·83 g/kg/d(Reference Højfeldt, Nishimura and Mertz41). Geirsdottir et al.(Reference Geirsdottir, Arnarson and Ramel35) reported data adjusted for sex, BMI, age and physical activity level and found higher lean body mass in the quartile with the highest protein intake (1·15–1·92 g/kg/d, the fourth quartile) compared with only the first quartile (0·41–0·75 g protein/kg/d, P = 0·04) and the second quartile (0·76–0·92 g/kg/d, P = 0·05), but not the third quartile. Interestingly, the differences disappeared when correcting for total energy intake, which emphasises the crucial importance of considering energy intake when evaluating the impact of protein intake on the regulation of body composition and especially lean body mass in older adults. Similarly, data by Asp et al.(Reference Asp, Richardson and Collene31) emphasise the dependency between protein and energy intake on muscle and body size as they found that protein intake correlated positively with nutritional status and BMI even when accounting for age, sex and activity level. In support of protein intake as a determining factor for a lean body composition, Gregorio et al.(Reference Gregorio, Brindisi and Kleppinger38) divided a total of 387 older women into those ingesting ≥ 0·8 g/kg/d (n = 290) and those ingesting < 0·8 g/kg/d (n = 97) and found that, although the women in the ≥ 0·8 g/kg/d group had lower lean mass than those in the < 0·8 g/kg/d group, they also had a lower fat mass, resulting in a lower ratio of fat to fat-free mass in the high-protein group. Collectively, some cross-sectional observational studies have reported positive associations between protein intake and muscle mass in older adults. However, it seems that, when accounting for nutritional status, energy intake and physical activity, there is limited evidence to recommend that protein intake greater than the international recommendation is beneficial for muscle mass preservation.
Observational studies
Association between protein intake and muscle mass was investigated in an observational study design in healthy older adults(Reference Houston, Nicklas and Ding32–Reference Scott, Blizzard and Fell34,Reference McDonald, Ankarfeldt and Capra36,Reference Chan, Leung and Woo37,Reference Verreijen, Engberink and Houston40) (Table 4). A positive association between protein intake and muscle mass was observed in some studies(Reference Houston, Nicklas and Ding32–Reference Scott, Blizzard and Fell34,Reference McDonald, Ankarfeldt and Capra36) , and these studies concluded that protein intake above the current international recommendation is beneficial for preserving muscle mass in older adults. In all of these studies, baseline average protein intake was higher (∼1·2 g/kg/d) than the current international recommendation (0·83 g/kg/d)(Reference Houston, Nicklas and Ding32–Reference Scott, Blizzard and Fell34,Reference McDonald, Ankarfeldt and Capra36) . In contrast, other studies found that protein intake higher than the current international recommendation does not preserve muscle mass in older adults(Reference Chan, Leung and Woo37,Reference Verreijen, Engberink and Houston40) . Chan et al.(Reference Chan, Leung and Woo37) discussed that the null association between protein intake and muscle mass was due in part to the relatively higher protein intake in this cohort (1·3 and 1·1 g/kg/d in men and women, respectively). More recently, Verreijen et al.(Reference Verreijen, Engberink and Houston40) reported that protein intake was not associated with 5-year changes in muscle mass. The contradicting results from Houston et al.(Reference Houston, Nicklas and Ding32) were explained by the methodological approaches used to assess muscle mass. Whilst Houston et al.(Reference Houston, Nicklas and Ding32) employed dual-energy X-ray absorptiometry (DXA), Verreijen et al.(Reference Verreijen, Engberink and Houston40) used computed tomography (CT), which is regarded as a more accurate methodology to examine muscle mass. In summary, inconsistent results have been reported in observational studies regarding the association between protein intake above the international recommendation (0·83 g/kg/d)(6). Thus, the newly suggested protein recommendations for healthy older adults of 0·94–1·3 g/kg/d from different authorities and expert groups(Reference Bauer, Biolo and Cederholm49–51,Reference Capra142) can still be questioned.
Table 4. Selected observational studies assessing the association between protein intake and muscle mass in healthy older adults

FFQ, food-frequency questionnaire; E, energy; DXA, dual-energy X-ray absorptiometry; Q, quartile; CT, computed tomography; RDI, recommended dietary intake; BW, body weight; LM, lean body mass; ASM, appendicular skeletal muscle mass; aLM, appendicular lean body mass.
Intervention studies
Several randomised controlled intervention studies have investigated whether higher protein intake increases muscle mass in older adults(Reference Kerstetter, Bihuniak and Brindisi43–Reference Mertz, Reitelseder and Bechshoeft48) (Table 5). Some studies were unable to show a beneficial effect of increasing dietary protein intake above their average intake of ∼1·2 g/kg/d on muscle accretion or maintenance in older adults(Reference Kerstetter, Bihuniak and Brindisi43,Reference Zhu, Kerr and Meng44,Reference Mertz, Reitelseder and Bechshoeft48) . Some studies showing no effect of higher protein intake (≥0·8 g/kg/d) tended to have a longer study period (≥1 year) compared with studies in which an increase in muscle mass was demonstrated (∼24 weeks)(Reference Kerstetter, Bihuniak and Brindisi43–Reference Ten Haaf, Eijsvogels and Bongers47), suggesting a possible adaptation effect. Mitchell et al. (Reference Mitchell, Milan and Mitchell46) showed that protein intake at 2RDA increased muscle mass compared with RDA in a group of men aged >70 years. over a period of 10 weeks. However, the individuals in this study consumed a habitual protein intake of 1·1–1·2 g/kg/d on average, and the individuals in the RDA group consumed less protein than the habitual intake during the intervention, which may explain the loss of appendicular lean mass and caused a group difference between RDA and 2RDA. For this study, several limitations can be listed, including that energy balance was not maintained in the RDA group; that lack of a steady metabolic state condition as adaptation was not present for the entire intervention period of 10 weeks; and finally, that no habitual protein intake group was included, which therefore does not allow us to conclude on changes of lean mass without altered protein intake. In summary, null effects of longer-term intervention of elevated dietary protein intake may indicate that metabolic adaptation may level out acute benefits, which was exemplified by a recent study by Højfeldt et al.(Reference Højfeldt, Bülow and Agergaard52).
Table 5. Selected randomised controlled trials investigating the impact of protein intake on muscle mass in healthy older adults

DXA, dual-energy X-ray absorptiometry; E, energy; BW, body weight; LM, lean body mass; RDA, recommended dietary allowance; MRI, magnetic resonance imaging; BW, body weight; CSA, cross-sectional area.
Protein turnover kinetic measurement
Protein turnover kinetic studies are used as more exploratory and mechanistic measurements of the underlying protein turnover kinetic rates. Since the 1970s(Reference Halliday and McKeran53), experimental settings in which the results of muscle protein synthesis (MPS) based on the stable isotope tracer direct incorporation measurement using precursor–product methods(Reference Wolfe and Chinkes54,Reference Kim, Suh and Lee55) , have established an important basis of knowledge in protein nutrition. In particular, stable isotope tracer and mass spectrometer methodology have advanced our knowledge on MPS in response to amino acids or protein intake(Reference Wilkinson56–Reference Wilkinson, Brook and Smith58). Experiments with infusion of stable isotope amino acid tracers are usually conducted for <24 h, and responses to ingestion of amino acids/proteins or mixed meals are measured in a controlled laboratory setting. Experiments are usually designed to measure post-prandial MPS (∼6 h) in response to protein intake while participants are kept in artificial conditions such as fasting and bedridden, often with standardised prior dietary intake. The tissue of interest is often skeletal muscle, with an emphasis on MPS rate. As muscle protein turnover contributes only approximately 25–35 % of whole-body protein turnover in humans(Reference Nair, Halliday and Griggs59,Reference Deutz, Wagenmakers and Soeters60) , it is important to take this into consideration if the results from these studies are to be used for estimating protein requirement.
Skeletal muscle protein
Amino acid availability in the circulation is a determinant of MPS stimulation(Reference Bohe, Low and Wolfe61), a response that is dose-dependent and saturable even in the presence of sustained elevated circulating amino acids(Reference Moore, Churchward-Venne and Witard62–Reference Bechshoeft, Dideriksen and Reitelseder68). Although originally hypothesised(Reference Balagopal, Rooyackers and Adey69–Reference Welle, Thornton and Jozefowicz71), recent accumulated evidence has concluded that post-absorptive MPS rates do not differ between older and younger individuals(Reference Volpi, Sheffield-Moore and Rasmussen72,Reference Hirsch, Church and Kim73) . However, older individuals exhibit a blunted post-prandial MPS response to amino acid/protein intake when compared with younger individuals(Reference Cuthbertson, Smith and Babraj63,Reference Katsanos, Kobayashi and Sheffield-Moore74–Reference Wall, Gorissen and Pennings76) , which has been termed age-related ‘anabolic resistance’. Moore and colleagues synthesised post-prandial MPS data generated in the lab previously(Reference Moore, Churchward-Venne and Witard62) and suggested that younger and older individuals are required to consume 0·24 g/kg/meal and 0·4 g/kg/meal, respectively, to maximally stimulate MPS, meaning that approximately 70 % more protein is required to maximally stimulate MPS in older individuals compared with younger individuals.
A blunted MPS and, hence, sub-optimal net balance in response to protein feeding and meals could be a plausible driver in the development of sarcopenia. This indicates that older adults need more dietary protein. However, it is important to note that this evidence is derived from acute post-prandial MPS studies in response to high-quality, rapidly digested, mostly animal-based proteins (e.g. egg, whey or casein protein)(Reference Moore, Churchward-Venne and Witard62,Reference Wall, Gorissen and Pennings76) .
In addition to the limitations involved in this experimental design, the potential beneficial effects of higher protein intake for muscle and/or whole-body net protein balance or anabolic response are unknown without the simultaneous measurement of muscle or whole-body protein breakdown. Further, the protein recommendation refers to whole-body protein balance(6,Reference Rand, Pellett and Young7) , which is not easily comparable with the responsiveness of skeletal muscle. Hence, we argue that data originating from experimental settings on MPS responsiveness to single dose of protein may lead to misinterpretation and erroneous conclusions on dietary protein requirements in humans at the whole-body level, and therefore cannot be extrapolated to whole-body protein requirements in daily living.
In summary, studies measuring the acute post-prandial response to protein feeding with the stable isotope tracer technique have led to the hypothesis of age-related muscle anabolic resistance. It is suggested as one of the underlying mechanisms of the development of sarcopenia(Reference Moore, Churchward-Venne and Witard62,Reference Cuthbertson, Smith and Babraj63,Reference Wall, Gorissen and Pennings76) . Such quantitative data suggest that protein intake of 0·4–0·6 g/kg/meal at three main meals and a snack per day is required to preserve muscle mass in healthy older individuals (Table 6), which equates to a protein intake of <1·2–1·8 g/kg/d(Reference Phillips and Martinson77).
Table 6. Protein recommendations derived from acute stable isotope tracer incorporation studies in younger and older adults

LBM, lean body mass.
* An individual with 75 kg of body mass (BM).
Values were adopted from Moore et al.(Reference Moore, Churchward-Venne and Witard62) determined by Biphasic Linear Regression Model from six independent studies(Reference Yang, Breen and Burd64,Reference Witard, Jackman and Breen65,Reference Moore, Tang and Burd146–Reference Churchward-Venne, Burd and Mitchell149) .
Whole-body protein
More recent investigations have shifted from the use of isolated protein sources to whole foods, the form of protein consumption in everyday life. This shift is due to the interactive effects of protein contained in whole foods with other nutrients and bioactive compounds on muscle and whole-body protein turnover. Studies have primarily focused on MPS in response to beef(Reference Symons, Schutzler and Cocke78,Reference Burd, Gorissen and van Vliet79) , eggs(Reference van Vliet, Shy and Abou Sawan80,Reference Kim, Shin and Schutzler81) and mixed macronutrient meals(Reference Kim, Schutzler and Schrader82,Reference Park, Jang and Choi83) . These studies highlighted the importance of considering complex food matrices in the regulation of muscle and whole-body protein turnover.
Previous studies investigating whole-body protein turnover in the context of mixed macronutrient meal intake demonstrated that anabolic response is not limited by protein synthesis(Reference Kim, Schutzler and Schrader82–Reference Kim, Schutzler and Schrader84). Deutz and Wolfe(Reference Deutz and Wolfe85) advocated that there is no limit to in vivo whole-body protein anabolism when protein is consumed as a part of a mixed macronutrient meal(Reference Beattie, Herbert and Bell97,Reference Pasiakos, Margolis and Orr101) . More recently, Park et al.(Reference Park, Jang and Choi83) showed in healthy older adults (69·3 ± 1·8 years) that there is a higher MPS response following the consumption of a higher protein intake (70 g) compared with moderate (35 g) as part of a mixed macronutrient meal, and a greater whole-body net protein balance in the higher protein intake group due to the suppression of protein breakdown as well as increased protein synthesis (Fig. 1). Importantly, the doses of protein used in this study (35 or 70 g) were beyond the amount suggested to maximally stimulate MPS in healthy older men(Reference Moore, Churchward-Venne and Witard62) (Table 6). Similar findings were also found by the same research group in healthy younger adults(Reference Kim, Schutzler and Schrader82).
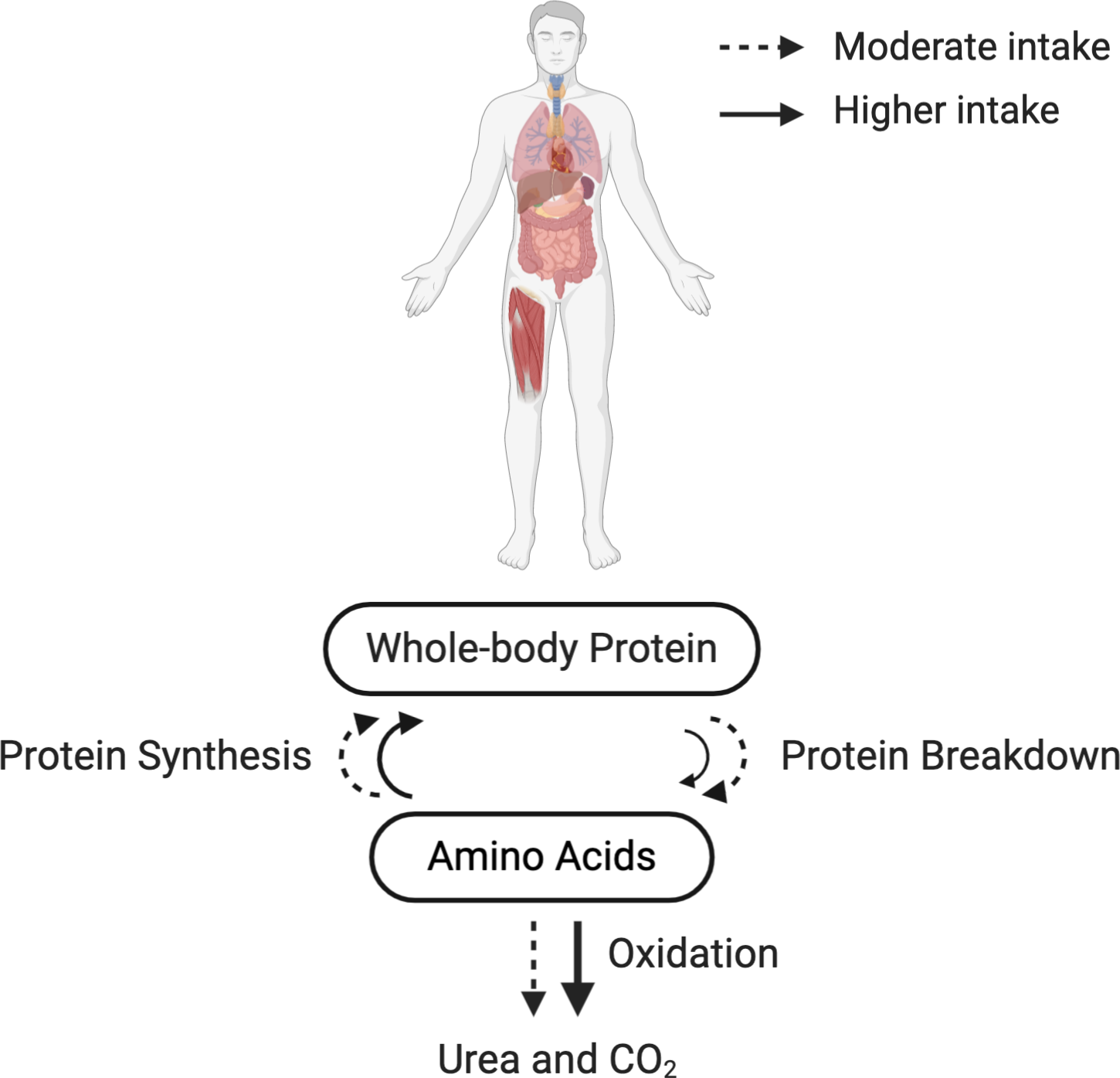
Fig. 1. Whole-body protein turnover in response to the recommended or higher protein intake in a mixed macronutrient meal. Dashed (- -) and solid (–) lines indicate a moderate (∼35 g/meal) and a higher protein intake (∼70 g/meal) in a mixed macronutrient meal, respectively. Protein synthesis is saturable at the given amounts of protein intake(Reference Kim, Schutzler and Schrader82), illustrated by the similar-sized arrows for protein synthesis between the moderate and higher protein intake. Protein breakdown is suppressed by the higher protein intake in a mixed macronutrient meal (illustrated by a smaller solid arrow), resulting in a greater whole-body net protein balance(Reference Kim, Schutzler and Schrader82,Reference Park, Jang and Choi83,Reference Deutz and Wolfe85,Reference Kim, Deutz and Wolfe150) . Be aware that a higher protein intake (i.e. surplus of amino acids) also inevitably increases amino acid oxidation and urea excretion(Reference Højfeldt, Bülow and Agergaard52,Reference Walrand, Short and Bigelow117) . The results depicted in this figure originate from collective data based on stable isotope tracer studies.
The suppression of protein breakdown may also be explained by insulin secretion from non-protein energy sources(Reference Gelfand and Barrett86,Reference Tessari, Inchiostro and Biolo87) . Higher insulin concentration in circulation is observed when protein is consumed in a mixed macronutrient meal(Reference Reitelseder, Tranberg and Agergaard88). A blunted protein breakdown suppression(Reference Wilkes, Selby and Atherton89) and a failure to achieve positive phenylalanine whole-body net balance(Reference Rasmussen, Fujita and Wolfe90,Reference Groen, Horstman and Hamer91) in response to insulin have been reported in older individuals, which may suggest a difficulty in achieving net positive whole-body protein balance in older individuals in response to mixed macronutrient meal intake. A systematic review and meta-analysis concluded that insulin has a permissive role in MPS, whereas insulin attenuates muscle protein breakdown independently of amino acid availability(Reference Abdulla, Smith and Atherton92,Reference Trommelen, Groen and Hamer93) . These studies highlight the importance of protein breakdown measurement in the context of a mixed meal intake owing to the suppression of protein breakdown in response to higher protein intake and insulin from non-protein energy sources, resulting in increased whole-body protein anabolism. Moving forward to the justification of the necessity to increase the current international safe level of intake for protein in healthy older adults, the upper limit of protein intake that suppresses protein breakdown, and thus better preserves whole-body protein, should be defined, as highlighted recently by Phillips et al.(Reference Phillips, Paddon-Jones and Layman14). We argue that whole-body protein turnover data and real-life interventions are more appropriate to extrapolate to protein requirements.
Future directions of protein requirements and recommendations for healthy older adults
Energy balance
The importance of energy intake in relation to the determination of protein requirement was raised already by the Joint FAO/WHO Ad hoc Expert Committee on Energy and Protein Requirements in 1971(94), and later in 1981(95). Notably, Young(Reference Young, Yu and Fukagawa29) and Millward(Reference Millward96) explored the important concept of protein and energy inter-relation to determine protein requirement. The interaction between energy and nitrogen intake on the maintenance of body nitrogen was well documented by studies on the role of non-protein energy sources (carbohydrate and fat)(Reference Beattie, Herbert and Bell97–Reference Munro99) using nitrogen balance methodology. For example, Colloway et al.(Reference Calloway and Spector98) showed back in 1954 that exogenous nitrogen-bearing sources contribute to energy production rather than deposition in the body until adequate energy is consumed. Further, increased non-protein energy intake can also mitigate nitrogen loss in a dose-dependent manner(Reference Calloway and Spector98). However, the provision of energy attenuates the recruitment of amino acid metabolism for energy turnover and hence retains nitrogen, leading to improvement in nitrogen balance(Reference Calloway and Spector98). Accordingly, the achievement of energy balance is an underlying assumption for the current internationally recommended protein intake(6).
In recent years, protein metabolism and turnover rate have been studied under a condition of negative energy balance(Reference Carbone, McClung and Pasiakos100–Reference Carbone, McClung and Pasiakos102) in healthy adults and with an attempt to preserve muscle mass or attenuate muscle loss under negative energy balance by increasing protein intake(Reference Carbone, Margolis and McClung103–Reference Mojtahedi, Thorpe and Karampinos108). The effect of protein intake above the current recommended safe level of intake (0·83 g/kg/d) on muscle mass maintenance under negative energy balance has been well documented(Reference Carbone, McClung and Pasiakos100,Reference Carbone, McClung and Pasiakos102,Reference Wycherley, Moran and Clifton109) . Evidence indicates that, whilst amino acids contribute more to energy production at negative energy balance(Reference Pasiakos, Margolis and McClung105,Reference Berryman, Young and Karl110) , they are utilised more for de novo protein synthesis when energy balance is achieved. However, evidence is scarce in healthy older individuals, and it is unclear whether increased protein intake during negative energy balance is sufficient to maintain whole-body and muscle protein mass in healthy older adults.
In contrast, limited knowledge is currently available on the impact of positive energy balance on the regulation of protein turnover rates and muscle mass in healthy older adults. Nonetheless, based on knowledge from energy deficit studies(Reference Pasiakos, Margolis and McClung105,Reference Berryman, Young and Karl110) as well as studies by Woolfson(Reference Woolfson111) and Calloway and Spector(Reference Calloway and Spector98), it can be assumed that positive energy balance reduces amino acid oxidation. Accordingly, exogenous amino acids (dietary protein) under such conditions are more efficiently utilised to achieve net positive protein balance. The impact of protein content relative to total energy intake on body composition in younger healthy individuals during overfeeding (18–35 years) was investigated by Bray et al.(Reference Bray, Smith and de Jonge112). Participants were divided into 5 % (low protein), 15 % (normal protein) and 25 % (high protein) of total energy intake from protein after a weight-stabilising diet period. Following an 8-week intervention, fat mass was similarly increased in all groups because of overfeeding (40 % excess energy from a weight-stabilising diet). However, lean body mass was significantly increased with normal and high protein groups, along with a concomitant increase in resting energy expenditure. These results suggest that energy intake from protein is a sole determinant to increase lean body mass, but not the accretion of fat mass during overfeeding in healthy younger individuals. In this cohort, additional protein may not be required when energy balance is maintained since no impact on lean body mass changes was detected between normal and high protein intake groups(Reference Bray, Smith and de Jonge112). Since these findings are limited to healthy younger individuals, further studies are required in healthy older adults. The interaction between energy balance and protein intake in amino acid oxidation, urea excretion and whole-body net protein and nitrogen balance at steady state is illustrated in Fig. 2.

Fig. 2. Interaction between energy and protein intake on amino acid oxidation, urea excretion, and whole-body net nitrogen and protein balance during the condition of adaptation. In each column, relative energy balance, protein intake, amino acid oxidation and urea excretion, and nitrogen/protein balance are expressed. For energy balance and nitrogen/protein balance, 0 (dashed line) indicates that a balance is maintained. Safe intake (dashed line) in protein intake shows the protein intake recommended by WHO/FAO/UNU (0·83 g/kg/d). (a) The column indicates zero whole-body net nitrogen and protein balance at the safe level of intake for protein recommended by WHO/FAO/UNU under energy balance condition(6). (b) and (c) indicate a negative energy balance condition. (b) The column shows the protein intake at the safe level of intake, but amino acid oxidation and urea excretion are increased under a negative energy balance condition, leading to negative whole-body net protein and nitrogen balance during negative energy balance(Reference Pasiakos, Margolis and McClung105,Reference Berryman, Young and Karl110) . (c) The column demonstrates that an increased protein intake (>0·83 g/kg/d) preserves whole-body net protein and nitrogen balance whilst increasing amino acid oxidation and urea excretion under a negative energy balance condition(Reference Pasiakos, Cao and Margolis104,Reference Leidy, Carnell and Mattes107,Reference Mojtahedi, Thorpe and Karampinos108) . (d) The column denotes that amino acid oxidation and urea excretion are reduced under a positive energy balance condition with an increased protein intake (>0·83 g/kg/d), resulting in positive whole-body net protein and nitrogen balance(Reference Bray, Smith and de Jonge112).
In summary, energy balance in addition to protein intake is a key determinant of protein turnover rates and net protein balance at the whole-body level. However, energy surplus itself does not seem to increase muscle mass, although it reduces amino acid oxidation. Thus, as repeatedly stated in the international reports from WHO/FAO/UNU, energy balance needs to be considered when determining protein requirements. In addition, it is important to highlight that evidence of the interaction between energy balance and protein intake is currently limited to younger individuals, and further evidence is required in healthy older individuals.
Metabolic adaptation to dietary protein intake
The consideration of metabolic adaptation to any given amount of protein intake is required for a valid measure of protein requirement. Protein turnover in the splanchnic area as well as in the periphery is adaptable to a given amount of protein intake(Reference Millward, Price and Pacy25–Reference Price, Halliday and Pacy27). Metabolic adaptation covers processes affecting the utilisation and fate of amino acids, primarily in the splanchnic area. A prolonged exposure to a given amount of protein intake forces enzyme and transporter levels to change accordingly to handle the amino acid availabilities. Hence, metabolic adaptation to a given protein intake level is a fundamental prerequisite when estimating protein requirements. In physiology, adaptation covers conditions where achievement of a steady state can be obtained after adjustments of metabolism and physiological function(Reference Waterlow113,Reference Waterlow114) . In contrast, when conditions are too extreme for metabolic pathways to adjust sufficiently, but rather continuously lag behind and the changes are beyond the range of adaptation, the condition is defined as accommodation (Reference Young115).
Recent emerging evidence highlights the mechanisms of adaptation to a protein intake higher than the current safe level of dietary protein intake. For example, Gorissen et al.(Reference Gorissen, Horstman and Franssen116) measured the availability of dietary protein using intrinsically labelled whey protein. In this study, older individuals (62 ± 1 years) were habituated to a protein intake of either 0·7 g/kg/d (LOW) or 1·5 g/kg/d (HIGH) for 2 weeks from a habitual intake of protein at 1·0 g/kg/d. Interestingly, no group differences were noted in either post-absorptive or post-prandial MPS, and more intrinsically labelled whey protein was available in the circulation in LOW (61 %) in comparison with HIGH (56 %). This was in agreement with our recent study where responses in amino acid and protein metabolism were investigated after habituation to a protein intake of >2·1 g/kg lean body mass (LBM)/d (0·82 g/kg/d) and a protein intake of 1·1 g/kg LBM/d (1·76 g/kg/d) for 20 d in older men (65–70 years)(Reference Højfeldt, Bülow and Agergaard52). These findings suggest that exogenous amino acids are directed less effectively into the circulation when habituated to a high protein intake. Habituation to a high protein intake also resulted in a diminished post-prandial synthesis rate of plasma proteins and a more negative overnight fasted whole-body net protein balance, suggesting a less effective utilisation of exogenous amino acids for protein synthesis. In accordance, Walrand et al.(Reference Walrand, Short and Bigelow117) demonstrated that higher protein intake showed a post-absorptive catabolic state, as demonstrated by higher amino acid oxidation and whole-body protein turnover, without changing MPS in both younger (24 ± 1 years) and older individuals (70 ± 2 years) when higher protein intake (3·0 g/kg fat-free mass) was compared with ‘usual’ protein intake (1·5 g/kg fat-free mass) over 10 d. However, nitrogen balance was improved in the higher protein intake group, which may suggest insufficient time to adapt to the new protein intake level in this study. Evidence emphasises the necessity of allowing time for metabolism to adapt to increased protein intake. Further evidence adopting study designs and methodological approaches that can account for metabolic adaptation is required to obtain a meaningful value for protein requirement, which can then be translated into recommendations.
Metabolic adaptation to changes in protein intake may explain the null findings when an intervention was performed long enough to achieve adaptation(Reference Millward26,Reference Højfeldt, Bülow and Agergaard52,Reference Millward118,Reference Millward and Smith119) . In other words, sustained increases in muscle mass would not be achieved when individuals chronically consume dietary protein levels higher than habitual protein intake level. Baseline protein intake level might be a key determinant of the beneficial effect of dietary protein intervention on muscle mass gain in healthy older adults in a randomised controlled intervention study. This notion was highlighted previously(Reference Holm and Nordsborg120) and is supported by a meta-analysis conducted by Ten Haaf et al.(Reference Ten Haaf, Nuijten and Maessen121), where they assessed the impact of protein supplementation on lean body mass, muscle strength and physical performance in community-dwelling older individuals. Protein supplementation is not beneficial for those outcome measures when sufficient habitual protein intakes are already consumed in non-frail older individuals.
A recent meta-analysis including a total of 8107 community-dwelling older individuals from cohorts in the Netherlands, the UK, Canada and the United States showed that the prevalence of protein intake lower than the currently recommended level of 0·8 g/kg adjusted body weight/d is 14–30 %(Reference Hengeveld, Boer and Gaudreau122). Thus, this low habitual protein intake group may be a more relevant target group for protein supplementation intervention with a concomitant focus on ensuring energy requirements. However, when the purpose is to determine whether a higher than currently recommended protein intake is favourable for muscle and whole-body protein mass, healthy older individuals habitually consuming protein around the current safe level of intake should be the target group and not the low-habitual intake group. Collectively, more randomised controlled intervention studies are required to conclude whether the newly suggested higher protein intakes from several expert groups above the safe level of intake at 0·83 g/kg/d have a favourable impact on muscle mass in healthy older adults consuming protein at the current international recommendation.
Protein quality
Protein quality is an overall measure of the ability of a protein source to meet the metabolic demand and is defined in terms of biological value (i.e. the fraction of amino acids absorbed by the gut from a food that is subsequently retained by the body). Biological value is expressed in terms of indispensable amino acid patterns relative to the requirement(6).
The concept of protein quality can be learned from the Ideal Protein Concept developed in the late 1950s by Mitchell(Reference Glista, Mitchell and Scott123) and Scott(Reference Fisher and Scott124). The Ideal Protein Concept is defined as the exact amounts of amino acids needed for optimal growth, meaning that it causes neither amino acid deficiency nor surplus availability. Thus, the Ideal Protein Concept is an effective way to define minimum protein in the diet to meet amino acid requirements for metabolic demands in animals. For example, the Ideal Protein Concept initially attempted to provide diets containing the exact balance of essential amino acids based on the composition of eggs and casein for the maximal growth and production performance of chickens. However, non-essential amino acids were not considered in the concept. Re-evaluations in the area of optimal animal feed for growth have found that the provision of non-essential amino acids is also required for development, growth, survival, reproduction and health(Reference Wu125,Reference Eggum126) . Consequently, the provision of non-essential amino acids will lower the required amounts of some essential amino acids as they will not be needed as precursors for de novo synthesis of non-essential amino acids. Therefore, protein quality must also be considered when discussing protein requirements and should be reflected in the overall recommendations(Reference Wolfe, Rutherfurd and Kim127), including the consideration of non-essential amino acids(Reference Tessari128).
Digestibility of amino acids is an integrated part of the protein quality measure. FAO developed the Protein Digestibility Corrected Amino Acid Score (PDCAAS) as a measure of overall nitrogen digestibility considering loss in faecal matter. This concept had some inherent limitations(Reference Lee, Weisell and Albert129) that were later attempted to be overcome by replacing it with the new term Digestible Indispensable Amino Acid Score (DIAAS), which includes: (1) the adoption of ileal amino acid digestibility; (2) abrogation of truncation of scores; (3) taking the influence of food processing into account; and (4) the use of individual amino acid digestibility.
The earlier protein requirements and recommendations by the WHO/FAO/UNU specified protein quality, whereas the current recommendations refer to ‘high-quality protein’ with a biological value of 100, usually an animal source protein (Table 1). Animal-based proteins are generally accepted as high-quality protein owing to better essential amino acid profile and higher digestibility (approximately 100) as compared with plant-based proteins (ranging from 80 to 85)(Reference Wolfe, Rutherfurd and Kim127,Reference Marinangeli and House130) . Plant-based proteins are less digestible due to existence of dietary fibre and compounds that inhibit enzymatic protein digestion.
Despite the accumulated evidence that supports muscle protein anabolic response by the ingestion of animal-based proteins, plant-based proteins have attracted more attention due to their environmental sustainability and population health benefits(Reference Tilman and Clark131). Recently, Burd et al.(Reference Burd, McKenna and Salvador132) discussed that consideration of protein quality is critical when protein recommendations are determined in relation to environmental considerations (e.g. managing greenhouse gas emissions, land and water use, and loss of biodiversity). Evidence shows that greenhouse gas emission is lower in plant-based proteins as compared with animal-based proteins(Reference Tilman and Clark131). Growing evidence has shown health benefits of vegetarian and vegan diets, including lower incidence and mortality from ischemic heart disease and lower incidence of cancer(Reference Dinu, Abbate and Gensini133). However, the effects of vegetarian and vegan diets on overall mortality rates are currently unclear(Reference Norman and Klaus134).
The impact of vegan and vegetarian diets on muscle mass maintenance in healthy older adults is an important consideration. Cross-sectional studies have shown that total protein and animal protein intakes, but not plant protein intake, are positively associated with muscle mass index in older women(Reference Lord, Chaput and Aubertin-Leheudre135). Results from a longitudinal observational study show that higher intakes of total protein and animal-based protein are associated with a reduced loss of lean mass over 3 years of follow-up, whereas plant-based protein intake is not associated with lean mass with the fully adjusted models in older adults(Reference Houston, Nicklas and Ding32). These data suggest that plant-based diet may not be favourable for muscle mass maintenance in older adults. However, we argue that more studies are required to fully elucidate this association.
Several strategies have been proposed by Gorissen and Witard(Reference Gorissen and Witard136) to overcome the perceived inferior anabolic properties of plant-based proteins. Firstly, the doses of plant-based protein intake can be increased; secondly, several plant-based protein sources can be mixed in a meal and in the whole diet to overcome any deficiency of a single essential amino acid; thirdly, co-ingestion of leucine can be added as an anabolic stimulant; and fourthly, muscle anabolic sensitivity can be enhanced by physical activity or by other means (e.g. by providing fish oil-derived n-3 polyunsaturated fatty acids in the meal or diet).
Considering potential metabolic roles of individual amino acids is also important. This notion has been exemplified by a study demonstrating that increasing the proportion of leucine to mixed amino acids without altering total amino acids content overcomes anabolic resistance in older individuals(Reference Katsanos, Kobayashi and Sheffield-Moore137). Furthermore, addition of leucine to a sub-optimal amount of protein is hypothesised to optimise anabolic response. For example, Wall et al.(Reference Wall, Hamer and de Lange138) demonstrated a greater post-prandial MPS following the consumption of 20 g casein protein with 2·5 g of crystalline leucine compared with no leucine in older men (74 ± 1 years), indicating that modifying and/or supplementing specific amino acid content might be an effective and practical strategy to improve anabolic response. This is particularly relevant to older individuals, whose energy requirement and appetite are decreasing with advancing age(Reference Roberts and Dallal139). Protein requirement that maintains whole-body and muscle protein mass can be achieved with lower total protein intake by optimising protein quality, which is also an important consideration for environmental issues. However, evidence is required on whether the modification of protein quality is a feasible approach to maintain whole-body protein balance in healthy older adults.
Conclusions
Considering the historical development of protein requirements and the use of various research methodologies to obtain scientific evidence for assessing protein requirements to create recommendations, we found that the previously used whole-body nitrogen balance methodology is challenged by methods evaluating whole-body and/or muscle mass and whole-body and/or muscle protein turnover rate as criteria. The newly suggested protein recommendations (0·94–1·3 g/kg/d) formulated by some authorities and expert groups target muscle mass maintenance instead of whole-body protein mass as their primary outcome criterion.
To close the knowledge gap between protein requirements for maintaining both muscle mass and whole-body mass, we identified that future research should assess the degree of agreement between these different, though related, outcome measures based on distinct methodologies. There is a strong need for prospective longitudinal studies with frequent monitoring of reliable dietary intake and concurrent measurements of whole-body protein mass and muscle mass with multiple methodologies. These would include short-term measurements (e.g. muscle and whole-body protein balance, kinetic rates, and nitrogen fluxes) and longer-term measurements (ensuring metabolic adaptation and energy balance) with consideration of protein quality. Also evaluating clinically relevant outcomes, such as muscle strength and function, physical function, body composition and metabolic health parameters, would be preferable. Such a holistic experimental approach would support establishing an agreement between muscle and whole-body protein mass maintenance, and thereby reveal the ‘true’ and healthy dietary protein requirements and recommendations for healthy older adults.
Acknowledgements
Y.N. is supported by the Postgraduate Research Scholarship Fund at the University of Birmingham.
Y.N.: Conceptualisation, Visualisation, Writing – original draft, Writing – review and editing. G.H.: Writing – review and editing. L.B.: Writing – review and editing. I.T.: Conceptualisation, Writing – review and editing. L.H.: Conceptualisation, Writing – review and editing. All authors read and approved the final manuscript.
The authors have no conflicts of interest.