Introduction
Hair lichens constitute a dominant floristic element in boreal forests across the Northern Hemisphere (Ahti Reference Ahti and Seaward1977; Esseen et al. Reference Esseen, Renhorn and Pettersson1996, Reference Esseen, Ekström, Westerlund, Palmqvist, Jonsson, Grafström and Ståhl2016), extending southward to temperate latitudes along the Western Cordillera of North America (Brodo & Hawksworth Reference Brodo and Hawksworth1977). Here we use the term hair lichen as a growth form category characterized by threadlike or narrowly cylindrical branches, the presence of a trebouxioid photobiont and, at boreal latitudes, a strong preference for conifers (Goward & Arsenault Reference Goward and Arsenault2003). Throughout their range, hair lichens perform important ecosystem functions from nutrient and water cycling (Van Stan & Pypker Reference Van Stan and Pypker2015; Pypker et al. Reference Pypker, Unsworth, Van Stan and Bond2017) to habitat and/or food for various animals including invertebrates, birds and mammals (Pettersson et al. Reference Pettersson, Ball, Renhorn, Esseen and Sjöberg1995; Rominger et al. Reference Rominger, Robbins and Evans1996, Reference Rominger, Robbins, Evans and Pierce2000). These ecosystem services are now compromised in many regions by industrial forestry (Esseen et al. Reference Esseen, Ekström, Grafström, Jonsson, Palmqvist, Westerlund and Ståhl2022). Interestingly, hair lichens can be sorted into two groups based on their colour. One group produces cortical melanic pigments which give the thallus a brownish or blackish colour, as in Bryoria and Nodobryoria, while another group is pale yellow-green owing to the presence of usnic acid in the cortex, as in Alectoria, Ramalina thrausta (Ach.) Nyl. and Usnea. All genera belong to the Parmeliaceae, apart from Ramalina (thrausta) (Ramalinaceae).
The two hair lichen morphogroups differ in their response to spatial gradients (Ahlner Reference Ahlner1948). Usnic hair lichens are more dominant at southern latitudes than farther north, while melanic lichens show the opposite trend (Esseen et al. Reference Esseen, Ekström, Westerlund, Palmqvist, Jonsson, Grafström and Ståhl2016). At the same time, usnic species are more common in the lower canopy of forest trees, while melanic species tend to dominate in the middle and upper canopy (Benson & Coxson Reference Benson and Coxson2002; Coxson & Coyle Reference Coxson and Coyle2003; Goward Reference Goward2003; Gauslaa et al. Reference Gauslaa, Lie and Ohlson2008). Finally, the annual growth rates of the usnic hair lichens Alectoria sarmentosa (Ach.) Ach. and Usnea dasopoga (Ach.) Nyl. have been shown to increase strongly with increasing rainfall, whereas growth in the melanic species Bryoria fuscescens (Gyelnik) Brodo & D. Hawksw. remained unchanged (Phinney et al. Reference Phinney, Gauslaa, Palmqvist and Esseen2021). These observations are consistent with the importance of canopy ventilation in the distributional ecology of water-retentive species in the genus Bryoria (Goward Reference Goward1998; Coxson & Coyle Reference Coxson and Coyle2003). Ventilation facilitates rapid desiccation and may thus counter the damaging effects of much externally stored water (Esseen et al. Reference Esseen, Rönnqvist, Gauslaa and Coxson2017). By contrast, usnic hair lichens are less water-retentive (Eriksson et al. Reference Eriksson, Gauslaa, Palmqvist, Ekström and Esseen2018) and should therefore be less dependent on ventilation. At the same time, an instantaneous photosynthetic activation of thin hair lichens upon exposure to humid air (Phinney et al. Reference Phinney, Solhaug and Gauslaa2018) may explain their ability to inhabit sheltered canopies (Gauslaa et al. Reference Gauslaa, Ohlson and Rolstad1998) where rain is shed centrifugally (Beier et al. Reference Beier, Hansen and Gundersen1993).
Our purpose is to describe the horizontal distribution of melanic versus usnic hair lichens on the lower branches of trees in even-aged coniferous stands formed over a small volcanic cone (Fig. 1). More specifically, we aim to test the potential of the canopy openness parameter, Angle-To-Canopy-Skyline measure (ATCS; see Goward et al. Reference Goward, Gauslaa, Björk, Woods and Wright2022), to predict spatial variation in the percent cover of usnic versus melanic hair lichens. Consistent with the ‘similar gradient hypothesis’ of McCune (Reference McCune1993), we predict that earlier findings concerning the vertical distributions of these two groups in the forest canopy (Benson & Coxson Reference Benson and Coxson2002) will be mirrored in their respective horizontal positions relative to canopy openness.

Fig. 1. The upper portions of Pyramid Mountain (British Columbia, Canada) as viewed from the east. The southern slope on the left had an open pine-dominated forest, whereas the northern slope on the right had denser forest with few pines. Note that dead and grey pine trees in this photograph were killed by the Mountain Pine Beetle (Dendroctonus ponderosae) a few years after fieldwork for this study was completed. In colour online.
Material and Methods
Study area
Fieldwork was performed in 2004 on Pyramid Mountain (Fig. 1), a 250 m conical volcano rising from the Murtle Plateau (800 m; 51°N, 120°W) in the Clearwater Valley of south-central British Columbia (BC). Soils were shallow and well drained, and derived from peralkaline basalts laid down in the volcanic eruptions that formed this mountain c. 12 000 years ago (Hickson Reference Hickson1986). This area of BC has cool, moist summers and cold, snowy winters (Goward & Ahti Reference Goward and Ahti1992). Forest cover was mostly c. 60 years old, dating from a wildfire that swept the area c. 1940. Common tree species were Abies lasiocarpa, Betula papyrifera, Picea glauca × engelmannii, Pinus contorta, Populus tremuloides and Pseudotsuga menziesii, with some Populus trichocarpa, Thuja plicata and Tsuga heterophylla in moister sites at its base. Henceforth these trees are referred to by genus name only. A detailed description of our study area appears in Goward et al. (Reference Goward, Gauslaa, Björk, Woods and Wright2022).
Field investigations
To capture hair lichen cover on lower conifer branches on all aspects of Pyramid Mountain, we established five linear east-west transects running in parallel at a distance of 200 m. Each transect consisted of 30 plots spaced at 50 m intervals. The transects thus created a slope-corrected grid over the mountain and the adjacent plateau (see Goward et al. Reference Goward, Gauslaa, Björk, Woods and Wright2022). Candidate trees were situated within 25 m of the plot centre and measured ≥ 10 m tall. Only living or recently dead branches with intact bark were examined. Four branches per tree (1.5–2.5 m above the ground) were studied in each cardinal direction (N, E, S and W), though only 1–3 suitable branches were available in some cases. In total, 523 branches were examined on 145 trees.
Total hair lichen cover (in percent) was assessed over a 1 m length of the upper side of the main branch axis centered midway between the trunk of the tree and the tip of the branch. The percent cover of each hair lichen species was also visually estimated on this branch segment. Lichen cover was calculated as the sum of individual species for melanic genera (Bryoria and Nodobryoria) versus usnic genera (Alectoria sarmentosa, Ramalina thrausta and Usnea spp.).
Relative degree of canopy ventilation is influenced by three separate variables, namely canopy height, stand spacing, and Angle-To-Canopy-Skyline (ATCS). Average canopy height was assessed within a 20-m radius of the plot centre. For stand spacing, we recorded the number of trees taller than 3 m growing within 10 m of the focal tree. ATCS is defined as the lowest angle at which foliar cover in the forest canopy obscures > 50% of the sky as viewed through a 4.5 cm circular viewfinder held 30 cm from the observer's eye (Goward et al. Reference Goward, Gauslaa, Björk, Woods and Wright2022). Each branch was accorded a separate ATCS reading across a horizontal span of 180°. Also measured was the diameter of the focal tree at breast height (DBH). Slope and aspect were estimated within 20 m of each focal tree. Finally, macroslope position was recorded as plateau surface, lower slope, middle slope and upper slope.
Our fieldwork preceded important revisionary work on Bryoria and Usnea by Myllys et al. (Reference Myllys, Velmala, Holien, Halonen, Wang and Goward2011) and Clerc (Reference Clerc2016), respectively. Taxonomic innovations advanced in these studies bring into question some of the species concepts applied by us in the field. Accordingly, and for the purposes of this paper, we prefer to unite morphologically similar species under a single ‘form name’. Hence the name Bryoria pseudofuscescens (Gyelnik) Brodo & D. Hawksw., as applied here, includes B. pikei Brodo & D. Hawksw. and B. inactiva Goward et al. (erroneously recorded in the field as B. friabilis), while B. fuscescens (Gyelnik) Brodo & D. Hawksw. includes B. glabra (Motyka) Brodo & D. Hawksw. and B. lanestris (Ach.) Brodo & D. Hawksw. For Usnea, we recognize two morphogroups: 1) ‘shrubby Usnea species’ in the case of U. glabrescens (Nyl. ex Vain.) Vain., U. perplexans Stirt. and U. substerilis Motyka; 2) ‘pendent Usnea species’ in the case of U. barbata (L.) F. H. Wigg and U. dasopoga (Ach.) Nyl.
Statistical analyses
All data recorded per branch were averaged for each tree. Normality was tested and data were treated in ways appropriate to their distributions and log-transformed when required. One-way ANOVAs for lichen functional group variables across host tree species were applied with level effects tested using the post-hoc Tukey-Kramer test. Significance cut-off values were P = 0.05 throughout. Means were reported ± one standard error. These statistics were analyzed using Jamovi 2.2.5 (https://www.jamovi.org/).
Best subsets multiple linear regression models for the cover of 1) usnic and 2) melanic hair lichens were searched for by including measured stand-related parameters. Regression models and correlation matrices were made in Minitab®, v. 21.1.1.
Results
Stand characteristics
Study plots had slopes between 0–37°, canopy height ranked 8–30 m, DBH was 10–65 cm, and ATCS varied from close to zero to 90° (see Supplementary Material Fig. S1, available online). Pinus was the most frequent tree recorded, followed by Pseudotsuga, Picea, Thuja and Tsuga (Table 1). Pinus was mostly situated on the steepest slopes (22 ± 1°) near the top and on open south-facing slopes, and had the lowest ATCS (11 ± 1°); Pseudotsuga (ATCS = 37 ± 2°) occurred on less steep slopes (10 ± 2°), whereas Thuja situated on level ground had the highest ATCS (60 ± 7°), consistent with its association with closed canopies.
Table 1. Functional lichen groups recorded on trees on Pyramid Mountain (British Columbia, Canada).

Means ± 1 standard error of functional groups also include trees without the presence of a given functional group.
*: log-transformation required for the ANOVA. Data for trees sharing the same superscript letter do not significantly differ from each other.
Hair lichen species distribution patterns
Nine hair lichen species (s. lat.) were recorded (Table 2). Among these, Alectoria sarmentosa, Ramalina thrausta, and shrubby and pendent Usnea morphogroups comprised the usnic species, whereas Bryoria fremontii (Tuck.) Brodo & D. Hawksw., B. fuscescens s. lat., B. pseudofuscescens s. lat., Nodobryoria abbreviata (Müll. Arg.) R. Howe and N. oregana (Tuck.) Common & Brodo made up the melanic species. Mean total hair lichen cover was 31.9 ± 2.4% but varied considerably according to tree (Table 1) and lichen species (Table 2). The most frequent hair lichens (growing on > 80% of the trees) were B. fuscescens s. lat., B. fremontii and A. sarmentosa (Table 2). The best host tree species for hair lichens in these young stands was Pinus (58.5% hair lichen cover), followed by Pseudotsuga (15.3% hair lichen cover).
Table 2. Summary statistics given as means ± 1 standard error (SE), minimum (Min) and maximum (Max) value, median with lower (Q1) and upper quartiles (Q3), for hair lichen species frequency and percent cover on lower branches where these lichens are present on studied trees (n = 145) on Pyramid Mountain (British Columbia, Canada). Species are ranked by decreasing frequency in each pigment group.

Shrubby Usnea species include U. glabrescens, U. perplexans (formerly U. lapponica Vain.) and U. substerilis; pendent Usnea species include U. barbata (formerly U. scabrata Nyl.) and U. dasopoga (formerly U. filipendula Stirt.); Bryoria fuscescens includes B. glabra and B. lanestris; B. pseudofuscescens includes B. pikei and B. inactiva.
Taken as a whole, the distribution of percent cover of usnic hair lichens on Pyramid Mountain was broadly mutually exclusive with that of the melanic hair lichens (Fig. 2), although the cover of the latter was c. 10 times higher (Table 2). Thus, while the melanic species (Fig. 2A) dominated on the south flanks of the mountain, usnic lichens (Fig. 2B) had their highest cover on its east, west, and especially north flanks. Concerning the spatial distribution of percent cover of individual genera, Alectoria (Fig. 3A) and Ramalina (R. thrausta; Fig. 3B) had similar patterns and, as the two dominant genera, shaped the spatial distribution of the cover of usnic hair lichens as a group (Fig. 2B). Alectoria was present on most trees (Fig. 3D), excepting a small number of trees on the south flank of the mountain as well as at its base. Its cover was low, except on the north flank and again at the base (Fig. 3A). By comparison, R. thrausta occurred on the north, east and west flanks (Fig. 3E). The distribution of the genus Usnea (Fig. 3F), mainly represented by the shrubby Usnea morphogroup, resembled that of the melanic hair lichens (Fig. 2A), although Usnea contributed little to usnic hair lichen cover (Fig. 3C).

Fig. 2. The distribution of total cover (relative scale) of melanic (A) and usnic (B) hair lichens in the lower canopy on Pyramid Mountain (British Columbia, Canada). The symbol size for melanic hair lichens has been reduced to 1/10 compared to usnic hair lichens due to the former's much greater abundance.

Fig. 3. The distribution of total cover (relative scale; A–C) and occurrence (D–F) of the usnic hair lichen genera Alectoria (A & D), Ramalina (B & E) and Usnea (C & F) in the lower canopy on Pyramid Mountain (British Columbia, Canada). In colour online.
Regarding the two melanic hair lichen genera, Bryoria (Fig. 4A) was the more abundant genus, while Nodobryoria (Fig. 4B) was comparatively sparse. At the same time, both genera had their highest cover on the south flank of the mountain, and their lowest cover on the north flank and at the base. Only on south-facing slopes near the summit did Nodobryoria achieve moderate cover values.

Fig. 4. The distribution of total cover (relative scale; A & B) and occurrence (C & D) of the melanic hair lichen genera Bryoria (A & C) and Nodobryoria (B & D) in the lower canopy on Pyramid Mountain (British Columbia, Canada). The symbol size for Bryoria has been reduced to 1/10 compared to Nodobryoria due to the former's much greater abundance. In colour online.
Melanic hair lichens were more strongly correlated with ATCS (r = −0.833) than with any other measure including canopy height (r = −0.638), slope (r = 0.596), tree spacing (r = −0.492), and DBH (r = −0.027) (see Supplementary Material Table S1, available online). ATCS alone accounted for 69.2% of the variation in melanic hair lichen cover (Fig. 5A). The best subset regression model for melanic hair lichens (Supplementary Material Table S1), which included ATCS (P < 0.001) and canopy height (P = 0.001), accounted for slightly more variation ($R_{adj .}^2 $ = 0.712; Variation Inflation Factor, VIF < 1.65) than ATCS alone (
$R_{adj .}^2 $ = 0.692). By contrast, usnic hair lichens did not correlate with ATCS (Fig. 5B) and increased significantly but weakly with canopy height (r = 0.207, P = 0.012) and DBH (r = 0.195, P = 0.019; Supplementary Material Table S2, available online). The best subset regression model for usnic hair lichens (Supplementary Material Table S2), which included canopy height and DBH, accounted for only a small part of the variation (
$R_{adj .}^2 $ = 0.073, P = 0.003, VIF < 1.1).

Fig. 5. The relationship between cover of melanic (A) and usnic (B) hair lichens versus angle to canopy skyline (solid line). Symbol shape and colour code for four macroslope positions: red upright triangles = upper slope and summit; yellow circles = middle slope; green down-facing triangles = lower slope; blue squares = base (plateau surface). The dotted lines show 95% confidence interval. In colour online.
Discussion
Distribution patterns of functional hair lichen groups
In this study we recorded two measures of hair lichen occurrence in the lower canopy of conifer forests on Pyramid Mountain. The first measure was presence/absence, which shows that melanic and usnic hair lichens were both widely distributed in these forests only six decades after stand initiation, a finding consistent with the inference that cortical chemistry per se has little relevance to the dispersal and establishment of Alectoria, Bryoria, Nodobryoria, Ramalina and Usnea. Even A. sarmentosa was well established here, though elsewhere it is often considered an associate of old-growth forests (e.g. Esseen et al. Reference Esseen, Ekström, Grafström, Jonsson, Palmqvist, Westerlund and Ståhl2022). However, our second measure, percent cover, revealed a very different picture, in which the south flanks of Pyramid Mountain were dominated by melanic hair lichens while usnic hair lichens were most abundant on its north flanks, a strikingly complementary distributional pattern that probably has its basis in different pigment-specific responses (Färber et al. Reference Färber, Solhaug, Esseen, Bilger and Gauslaa2014).
This aspect-mediated trade-off in the horizontal distribution of melanic and usnic hair lichens corresponds well with earlier reports of their respective vertical distribution in the forest canopy (i.e. with the first group increasing in abundance with increasing height and the second group decreasing) (Campbell & Coxson Reference Campbell and Coxson2001; Coxson & Coyle Reference Coxson and Coyle2003; Gauslaa et al. Reference Gauslaa, Lie and Ohlson2008). Importantly, this pattern recalls the ‘similar gradient hypothesis’ (McCune Reference McCune1993), which posits that the horizontal and vertical distributions of epiphytic lichens run in parallel, as also concluded by Esseen (Reference Esseen2019). In the present case, melanic hair lichens would therefore be expected to attain their optimum development in open, sun-exposed sites, while usnic hair lichens should be more common in more sun-sheltered sites, consistent with our results. We suggest that this occurrence justifies the separation of pendent hair lichens into two pigment-based functional groups, here referred to as usnic hair lichens and melanic hair lichens.
Light screening of cortical pigments
The dominance of dark-pigmented hair lichens in sun-exposed situations and pale-pigmented hair lichens in more shaded sites can be attributed to quantified differences in their respective tolerance for direct sunlight, with light tolerance in the melanic group being markedly higher than that in the usnic group (Färber et al. Reference Färber, Solhaug, Esseen, Bilger and Gauslaa2014). This is true especially for lichens in the desiccated state, which less efficiently handle excess excitation and lack an active repair mechanism (Beckett et al. Reference Beckett, Minibayeva, Solhaug and Roach2021). An interesting corollary of this is that frequent hydration allows usnic hair lichens to thrive in light-exposed upper canopies in wet climates (Benson & Coxson Reference Benson and Coxson2002; Antoine & McCune Reference Antoine and McCune2004) and on scattered trees in humid open forests, a consequence of hydration-dependent improved repair of photoinhibitory damage formed during dry periods.
The light-screening function of cortical pigments optimizes lichen performance along gradients from shade to sun (Gauslaa & Solhaug Reference Gauslaa and Solhaug2001; Solhaug & Gauslaa Reference Solhaug and Gauslaa2012; Gauslaa & Goward Reference Gauslaa and Goward2020). Effects of hydration on light screening in hair lichens are schematically summarized in Fig. 6. Usnic hair lichens (Fig. 6A) have pale cortices that are slightly transparent even when dry, thereby exposing the desiccated underlying chlorophylls to some light and thus to oxidative stress. Their distinct yellowish green colour is attributable partly to chlorophyll and partly to the faintly yellow usnic acid. Such pale pigments screen solar radiation by reflectance (Solhaug et al. Reference Solhaug, Larsson and Gauslaa2010), thereby reducing solar radiation-induced warming (Kershaw Reference Kershaw1975) and prolonging photosynthesis after hydration (Phinney et al. Reference Phinney, Asplund and Gauslaa2022). By contrast, the dark cortex of the melanic genus Bryoria (Fig. 6B) absorbs most incident solar radiation with hardly any visible green reflectance. Thereby, photoinhibitory damage to the underlying photosynthetic algae is prevented (Färber et al. Reference Färber, Solhaug, Esseen, Bilger and Gauslaa2014). At the same time, desiccated Bryoria also tolerates solar heating up to 70 °C (Lange Reference Lange1953), probably a necessary adaptation for blackish lichens growing in sun-exposed sites. During wetting, photobiont cells become turgid and thus larger, while water absorbed into the expanding cortical hyphae dilutes their pigments and fills empty air spaces. Particularly in usnic hair lichens, this results in greater cortical transparency, seen as a green tinge caused by reflectance from the underlying algal chlorophylls. Crucially, a transparent cortical window optimizes photosynthesis in thalli growing in shaded sites.
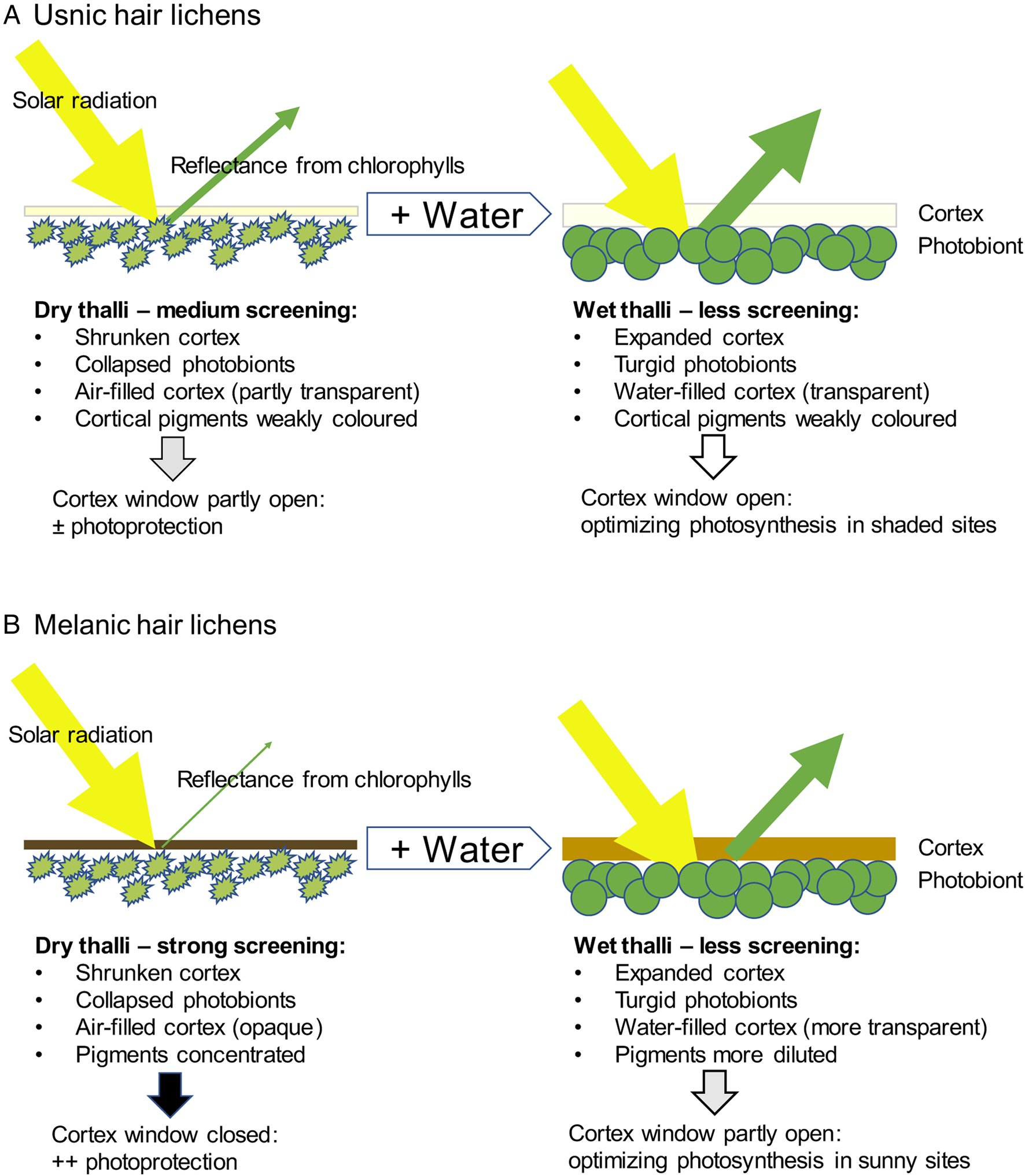
Fig. 6. Schematic interpretations of effects of hydration on light screening in hair lichen functional groups. A, usnic hair lichens (usnic acid in the cortex). B, melanic hair lichens (with cortical melanic pigments). The thickness of the green/darker shade arrows depicts the greenness in desiccated (left) and hydrated thalli (right) as seen from outside through the cortical window. The greenness is caused by reflectance from the algal chlorophylls and is thus a measure of cortical transmittance; see text for further explanation. Some lichen characteristics are summarized in the text below, emphasizing ways in which hydration state and pigments affect photoprotection in terms of cortical light screening and photosynthesis. In colour online.
An interesting finding of our study is the strong association of short, shrubby species (Usnea glabrescens, U. perplexans, U. substerilis) with south-facing, sun-exposed slopes (Fig. 3) versus the almost exclusive occurrence of long, pendent species such as A. sarmentosa, Ramalina thrausta, U. barbata and U. dasopoga (data for the two pendent Usnea species not shown) on more sun-sheltered aspects. It is worth noting that shrubby Usnea species have thick branches and high water storage capacity compared to the thin branches and low water storage of pendent species (Eriksson et al. Reference Eriksson, Gauslaa, Palmqvist, Ekström and Esseen2018). Thallus morphology thus influences hydration traits in this genus. In addition, the thicker branches probably improve light screening efficiency, as suggested by their predominance on the south flanks of Pyramid Mountain. It is well known that thick foliose lichens containing usnic acid, such as Arctoparmelia and Xanthoparmelia, are well suited to fully sun-exposed arid environments, even in hot climates (e.g. Elix Reference Elix and Orchard1994; Hauck et al. Reference Hauck, Dulamsuren and Mühlenberg2007). By contrast, dry pendent usnic hair lichens have poor cortical screening (Färber et al. Reference Färber, Solhaug, Esseen, Bilger and Gauslaa2014), thereby hindering successful colonization in dry, sun-exposed habitats, a feature apparently unique among usnic lichens. We predict that usnic hair lichens will increase in the lower canopy of trees on Pyramid Mountain as the forests age and become shadier.
Conclusion
Considering the data summarized in this paper, the occurrence in pendent hair lichens of cortical melanin versus usnic acid justifies the recognition of two functional groups, consistent with our hypothesis. Investment in light-absorbing melanic pigments can be considered an extreme form of specialization for high light (one present in only a small number of hair lichen genera) that favours persistence in dry, sun-exposed canopies of otherwise cool forests. By contrast, an ability to synthesize light-reflecting usnic acid yields a more flexible solar radiation screen that has evolved across a wide array of lichen genera worldwide. Only in pendent usnic hair lichens is the cortex sufficiently thin to facilitate optimum light transmission in shaded sites, though this comes at the cost of high sensitivity to light in open habitats, especially in rather dry regions. By contrast, shrubby Usnea species characterized by thicker branches are less light-sensitive and hence better adapted to sun-exposed localities. Cortical pigments thus play important roles in shaping the ecological niches occupied by epiphytic hair lichens.
Acknowledgements
We thank Wes Bieber, former Planning Forester for Weyerhaeuser Company, for generous assistance in the early stages of this study, while Glenn Thiem, of Forsite Consultants, is thanked for his efforts in coordinating the project. We also thank Curtis Björk, Derek Woods and Ken Wright for field work during this project. Funding was provided through the British Columbia Forest Science Council.
Author ORCIDs
Yngvar Gauslaa, 0000-0003-2630-9682; Trevor Goward, 0000-0003-2655-9956.
Competing Interests
The authors declare none.
Supplementary Material
To view Supplementary Material for this article, please visit https://doi.org/10.1017/S0024282923000075.