Introduction
The Magelonidae is a small family of annelids found worldwide, with distinctly flattened, spade-like prostomia. They possess a pair of ventrally inserted, papillated palps unique to the family. The majority of Magelona species occupy sandy-muddy habitats (Uebelacker & Jones, Reference Uebelacker, Jones, Uebelacker and Johnson1984) at shallow depths (Rouse, Reference Rouse, Rouse and Pleijel2001a; Blake, Reference Blake, Rouse and Pleijel2006), although deep-water species have been described (Hartman, Reference Hartman1971; Aguirrezabalaga et al., Reference Aguirrezabalaga, Ceberio and Fiege2001). The family is predominately distributed in coastal areas and on continental shelves (Hernández-Alcántara & Solís-Weiss, Reference Hernández-Alcántara, Solís-Weiss, De León-González, Bastida-Zavala, Carrera-Parra, García-Garza, Peña-Rivera, Salazar-Vallejo and Solís-Weiss2009).
Magelonids have been primarily described as surface deposit feeders (Jones, Reference Jones1968; Uebelacker & Jones, Reference Uebelacker, Jones, Uebelacker and Johnson1984). Suspension feeding has been reported for Magelona johnstoni Fiege, Licher & Mackie, Reference Fiege, Licher and Mackie2000 (Mortimer & Mackie, Reference Mortimer and Mackie2014) and additionally suggested for other magelonid species by Hartmann-Schröder (Reference Hartmann-Schröder1971), Wolff (Reference Wolff1973) and Rouse (Reference Rouse, Rouse and Pleijel2001a). Conversely, Jumars et al. (Reference Jumars, Dorgan and Lindsay2015) indicated that subsurface feeding (primarily based on observations of Magelona pitelkai Hartman, Reference Hartman1944) and carnivory may be more common within the family than previously indicated. Fauchald & Jumars (Reference Fauchald and Jumars1979) considered feeding to be selective, with selectivity increasing in nutrient-poor conditions.
Diets of a number of magelonid species have been described to include crustaceans, crustacean larvae, silt, detritus, sandy detritus, sand, diatoms, algal cysts, spores, tintinnids, molluscs, worms and other small animals (McIntosh, Reference McIntosh1911; Hunt, Reference Hunt1925; Mare, Reference Mare1942; Jones, Reference Jones1968; Hartmann-Schröder, Reference Hartmann-Schröder1971; Wolff, Reference Wolff1973; Kühl, Reference Kühl1974). Despite these records, knowledge of species-specific diets is lacking. Additionally, Mortimer & Mackie (Reference Mortimer and Mackie2014) suggested the likelihood of interspecific variation in diets of co-existing European magelonids.
In general, magelonids have been reported to be relatively motile, burrowing more or less continually through sediments (Jones, Reference Jones1968; Fauchald & Jumars, Reference Fauchald and Jumars1979; Mortimer & Mackie, Reference Mortimer and Mackie2014). Although some species such as Magelona polydentata Jones, Reference Jones1963, Magelona variolamellata Bolívar & Lana, Reference Bolívar and Lana1986, Magelona longicornis Johnson, Reference Johnson1901 (Jones, Reference Jones1971), Magelona cincta Ehlers, Reference Ehlers1908, Magelona falcifera Mortimer & Mackie, Reference Mortimer and Mackie2003, Magelona symmetrica Mortimer & Mackie, Reference Mortimer and Mackie2006 and Magelona alleni Wilson, Reference Wilson1958 have previously been reported to construct tubes (Mortimer et al., Reference Mortimer, Cassa, Martin and Gil2012; Mortimer, Reference Mortimer, Purschke and Westheide2017), no information on the permanency of tubes has been recorded.
Currently, nine European magelonids are described (Fiege et al., Reference Fiege, Licher and Mackie2000; Aguirrezabalaga et al., Reference Aguirrezabalaga, Ceberio and Fiege2001; Mortimer et al., Reference Mortimer, Gil and Fiege2011): Magelona filiformis Wilson, Reference Wilson1959, Magelona wilsoni Glémarec, Reference Glémarec1966, Magelona minuta Eliason, Reference Eliason1962, Magelona mirabilis (Johnston, Reference Johnston1865), M. johnstoni, Magelona lusitanica Mortimer, Gil & Fiege, Reference Mortimer, Gil and Fiege2011, Octomagelona bizkaiensis Aguirrezabalaga, Ceberio & Fiege, Reference Aguirrezabalaga, Ceberio and Fiege2001, Magelona equilamellae Harmelin, Reference Harmelin1964 and M. alleni. The latter species is readily distinguished from all other European Magelona in having sub-equal abdominal lamellae (Fiege et al., Reference Fiege, Licher and Mackie2000). It possesses a wider-than-long prostomium and a conspicuous reddish pigment band on posterior thoracic chaetigers, as described by Wilson (Reference Wilson1958) (Figure 1B).

Fig. 1. (A) Aquarium tank and cooling system, with time-lapse camera set up: camera, flash and interval timer; (B) anterior end of a live Magelona alleni (dorsal view) from Jennycliffe Bay, Plymouth Sound, relaxed (MgCl2), showing thoracic pigment band on posterior thorax (photo: Andrew Mackie).
Defecation in magelonids has not been previously described, and due to their fragility upon collection, many magelonid species descriptions do not contain figures or information of the posterior region. Consequently, there has been minimal attention directed at the morphology of the pygidium. At present, the pygidia of 16 magelonid species have been illustrated (Mortimer, Reference Mortimer, Purschke and Westheide2017). Rouse (Reference Rouse, Rouse and Pleijel2001a), primarily based on the type description and illustration of Magelona sacculata Hartman, Reference Hartman1961, stated that the magelonid anus is terminal. However, early illustrations from McIntosh (Reference McIntosh1878) of M. mirabilis (possibly M. johnstoni, see Fiege et al., Reference Fiege, Licher and Mackie2000) clearly show the anus in a distinctly ventral position. Unfortunately, the majority of subsequent illustrations are from a dorsal view, and the position of the anus is not clearly visible.
In order to add clarity, posterior regions of several European species and M. sacculata have been investigated in comparison to M. alleni. Furthermore, the present paper aims to increase current understanding of the behaviour and diet of magelonids, including the first record of defecation for the family. The tubicolous lifestyle of M. alleni is discussed in relation to all observed behaviours.
Materials and methods
Animal collection and processing
Benthic samples were collected from Jennycliffe Bay, Plymouth Sound (50°20.91′N 4°07.71′W, 8 m, muddy sand) from RV ‘Sepia’ on 27 March 2017 using a short-armed, chain-rigged Van Veen grab. Samples were gently washed with copious amounts of seawater in large vats, gradually breaking-up the sediment and suspending animals. These washings (containing the majority of animals) were collected on a 0.5 mm mesh and placed into labelled containers. They were taken back to laboratories at the Marine Biological Association (MBA) for further processing and removal of M. alleni individuals. Additional sediment from the sampling site was sieved at the MBA through a 0.5 mm sieve to remove macrofauna. Some of the sieved sediment was placed into a small glass tank and individuals of M. alleni were added on the sediment surface. Further sediment was placed on top of the animals and allowed to settle in a fridge before the tank was placed into a larger aquarium holding additional tanks containing other magelonid species.
Experimental design
The experimental set up was as described by Mortimer & Mackie (Reference Mortimer and Mackie2014) for observations of M. johnstoni. An aquarium tank (45L × 20W × 20D cm), holding ~11 litres of artificial seawater (salinity ~35 ppt), housed a separate, smaller, cylindrical observation tank (3.8 cm in diameter and 8.5 cm deep, ~95 cm3). During initial observations, the cylindrical tank was filled to ~75% with sediment (Figure 1A). However, in later observations, the sediment was filled to the top of the tank to ensure water flow across the sediment surface, and that sediment depth was ~3 times animal length (~2.8 cm, allowing space for burrowing both vertically and horizontally). An aquarium cooler (D-D DC300) regulated water temperatures and corresponded to average sea surface temperatures for Plymouth (10–17 °C). An aquarium pump and large air stone were employed to aerate the water and create a current and continuous flow within the tank. Observations were carried out under ambient photoperiods.
Tank observations
Initially, three individuals were observed for a 6-month period (July 2017–January 2018) through time-lapse photography (see Figure 1A, Canon EOS 6D 20.2 MP DSLR camera, Canon EF 100 mm f/2.8 L Macro IS USM lens), with a further three individuals observed during 2018. At first, captures were carried out at differing intervals, ranging from 30 s to 5 min, either throughout the day or night, to ascertain the best times for undertaking observations. It was then determined that 24 h capture periods at 3 min intervals, 6 days a week, would provide comprehensive results. However, further captures at shorter intervals were carried out in order to investigate particular behaviours. Observations were additionally made throughout using a microscope held horizontally as described by Mortimer & Mackie (Reference Mortimer and Mackie2014).
In situ experiments
Worms were initially offered a variety of foods, the choice of which was based on information from previous magelonid observations and what is known about magelonid diets (e.g. Jones, Reference Jones1968; Taghon et al., Reference Taghon, Nowell and Jumars1980; Forbes et al., Reference Forbes, Forbes and Holmer1996; Williams, Reference Williams2002). Based on initial trials it was decided that two food suspensions (SeAquariums Invertfood and NTLABS Invertebrate Food, mixed equally), both containing plankton and essential nutrients, would be gently administered into the water column above the tank every 7 d using a plastic pipette. Observations through the microscope were made directly afterwards to determine reactions and corresponding behaviours, in addition to ongoing time-lapse photography.
Approximate palp length (overall length, ratio at surface) was calculated using a line gauge from time-lapse photographs for comparison to other European Magelona species. Sand expulsion events during each 6-day interval for a period of 12 weeks were recorded for all individuals. The variables, time of day and time elapsed since food was last administered to the tank were recorded to test relationships between them and frequency of expulsion. Time taken to turn within the burrow, resurface, and return to feeding were additionally recorded. Burrow locations for each individual were observed and mapped, in addition to residency time and changes in burrow structure.
Palp and papillae measurements
Palp lengths for preserved specimens of M. alleni, M. johnstoni and M. mirabilis from Amgueddfa Cymru – National Museum Wales (NMW) collections were measured using a Leica MZ9.5 zoom microscope, fitted with an eyepiece graticule. The length of the non-papillated region, width of palp (at base) and the length of selected distal and medial papillae were also measured. Average numbers of papillae in each row were calculated at distal, medial and proximal positions for five adult specimens of each species. Total palp length was measured both in millimetres and the approximate chaetiger it reached from its attachment site.
Scanning electron microscopy (SEM)
Further material from NMW collections (initially fixed in ~6–8% formaldehyde and transferred to 80% ethanol) was prepared for SEM. Specimens were taken through an alcohol series to 100% ethanol to remove any water before critical-point drying (Quorum K850 critical-point dryer). Samples were then sputter coated with gold (Agar auto sputter coater, 40 mA for 120 s) and imaged with a Jeol Neoscope JCM-5000 SEM.
Drawings
Drawings were made either using a camera lucida attachment on a Leica MZ9.5 zoom microscope or Leica DM2000 compound microscope, or by the tracing of time-lapse photographs.
Image stacking
Images were taken using a Canon 70D DSLR camera attached to a Leica Z6 macroscope. Individual source images were then stacked using HeliconFocus v6.22 (HeliconSoft Ltd) extended depth of field software, with calibrated scale bars added using Syncroscopy Automontage v.5.4. Material used was as described above, plus the type specimens of M. alleni borrowed from the Natural History Museum, London (NHM: BMNH 1958.5.2.1–10).
Comparative material
The holotype of M. sacculata was borrowed from the Natural History Museum of Los Angeles (NHMLA: LACM-AHF POLY 596).
Results
Feeding and palp movement
Palps were deployed into the water column through sand mounds situated around burrow entrances. Each palp independently scanned the substrate surface to collect particles using papillae at palp tips. Collected particles were passed between neighbouring papillae on a portion of the palp held in a C shape (Figure 2) that progressed swiftly down the length of the palp in a conveyor-belt-like fashion, until reaching the burrow. Many particles could be moved along the length of the palp (Figure 2D, LH palp) and/or along both palps simultaneously (Figure 2A). Within the burrow, particles passed towards the mouth between tightly aligned palps, from papillae to papillae in coordinated movements. At the non-papillated region particles were seemingly ‘dropped’ into the mouth. Collected food consisted of sand, with some foraminiferans and particles from commercial suspensions. Although sand grains predominated, M. alleni appeared relatively non-selective in particles collected.

Fig. 2. Various time-lapse photographs of cupping movements utilized for the passing of collected particles along the palps of Magelona alleni, either by both palps simultaneously (A), or just one palp (B, C); (D) showing several cupping movements on the same palp.
Suspension feeding was also exhibited, although only directly after the commercial suspensions were first added to the tank and for limited periods. C shaped portions of the palps captured particles within the water column, and the greenish hue from the newly added food was seen descending proximally down the palps.
Palp ‘knotting’ (where a palp appeared as though tied; Figure 3E, F) occurred sporadically and usually involved the collection of primarily larger particles of sand. No direct observations of particles being transferred to the mouth were made for this behaviour, however.

Fig. 3. Various time-lapse photographs of palp coiling (A–D) and palp knotting behaviours (left palps) (E, F), observed for Magelona alleni.

Fig. 4. ‘Pitchfork’ burrow network of one individual of Magelona alleni, showing two branches open at the sediment-water interface. Approximately 20 chaetigers of the animal's posterior drawn in central branch of network, pygidium at the junction of the three tubes. Anterior anchored deep down towards the base of the tank.
Palps (distal third) were predominately held within the water column regardless of time of day or food availability. In between periods of feeding, some palp movement was always visible. Palps were slightly parted and either loosely coiled, or relatively straight and gently moving laterally. During coiling, loose helices deployed at a slight angle, moved slowly up and down (Figure 3A–D). Throughout these periods (whether coiled or straight), no contact was made with the substrate surface, and sediment particles were never observed to be associated with either behaviour.
Palp morphology
Magelona alleni (5.5–8 mm, reaching ~10–13 chaetigers) palps were shorter than both M. johnstoni (10–13 mm, reaching ~23–28 chaetigers) and M. mirabilis (5–14 mm, reaching ~14–20 chaetigers) (Table 1), measured in preserved specimens (N.B. total palp length greater in live, relaxed animals, particularly M. alleni, see Figure 1B). Palps were wider, with a proportionally smaller non-papillated region for the former species (Table 2). However, the palps of M. johnstoni and M. mirabilis were more sparsely papillated, with shorter papillae (compare Figures 1B, 9E and 10A with figure 5B of Mortimer & Mackie, Reference Mortimer and Mackie2014) and with a more conspicuous longitudinal non-ciliated region (previously termed median non-papillated groove).

Fig. 5. Various time-lapse photographs of posterior positioning at differing stages of sand expulsion, observed for Magelona alleni: (A, B) straight posture upon emergence from the burrow; (C, D) relaxed posture towards the end of an expulsion event.
Table 1. Showing variation in total palp length, taken for five adult specimens of each species measured. The approximate chaetiger that the palp reached from attachment site on ventral side of the prostomium is additionally recorded.
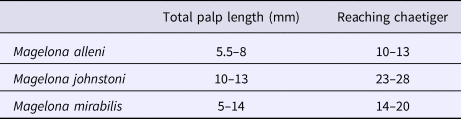
Table 2. Showing average palp and papillae measurements recorded for five adult specimens. Approximate number of rows of papillae recorded in a distal, medial and proximal location of the palps.

Defecation
Before defecating, M. alleni turned from an anterior-upwards to an anterior-downwards position, so that the prostomium faced almost vertically towards the bottom of the tank. The body appeared greatly stretched and the tail rose above the surface, whilst the head remained far down in the burrow. Turning took on average 6 min. The straightened tail of individuals emerged from the burrow (for ~15–20 chaetigers, Figure 5A, B) and moved in slow, controlled lateral movements from side to side. Sand was then excreted from the anus (Figure 6) and pygidial cirri were distinctly parted. Sand was dispersed slowly around the tank and some grains glided upwards, as if attached in a mucus string (Figure 6F), before becoming stuck to the glass, or landing back onto the sediment surface. Occasionally the expelled sand and mucus would attach to the glass for several hours, steadily sliding to the substrate (Figure 6B). As an expulsion event came to an end, the posterior became progressively flaccid and the pygidium relaxed towards the substrate surface (Figure 5C, D). Sand expulsion then ceased, and the tail was retracted back into the burrow. The length of time that the posterior region was visible above the surface ranged between 3–7 min. Animals took a further 3–90 min to reappear to an anterior-upwards posture, with palps back in the water column. Frequency of sand expulsion showed no significant variation with time of day and time elapsed since food was last administered.

Fig. 6. Various time-lapse photographs of sand expulsion, observed for Magelona alleni: (A, D, E) sand expelled downwards; (B) sand adhering to glass after expulsion event; (C) sand expelled upwards from pygidium; (F) sand ascending after release from anus, with associated mucus string (C–F, palps of another individual visible).
Burrows and tubes
Individuals formed branched burrow networks that were primarily permanent, and minimal change occurred in position and structure (August–January). However, burrowing movements were made occasionally, extending or rebuilding branches within the network. Burrowing was achieved by repeated cycles of eversion and retraction of the burrowing organ; everted for anchorage to pull the body forwards, then retracted to allow the prostomium to edge forwards, as has been observed in other magelonids.
Burrows were lined with a purple/pink papery membranous tube of several layers. The burrow network of each individual could be clearly seen, and each animal always used the same set of branched tubes. However, the tube of one individual was attached directly to the glass surface, ensuring the animal was visible within its network. This animal moved within the constructed tube with palps held stiffly and straight out in front of the prostomium.
All burrow networks were pitchfork-shaped, consisting of: one long vertical tube in the centre, bisecting a U-shaped tube (Figure 4), with either two or three openings at the sediment surface (clearly visible as distinct holes when viewed from above). Individuals tended to utilize one of the openings to access the surface, however, if disturbed, animals often used a different opening thereafter. Based on observations of one individual, the central tube was always an anchor point for either the animal's posterior, or anterior during defecation or feeding. Generally, the animal was elongated allowing it to withdraw quickly back into the burrow if required, and when turning, the tail was squeezed past the body within the tube.
Pygidia
The anuses of M. johnstoni, M. mirabilis, M. filiformis and M. minuta are all ventrally located; see Figures 8A–D and 10D–F. The pygidia of the first three species (Figures 8A, B, D and 10D, E) are rounded to sub-triangular, with two laterally placed digitiform cirri, those of M. filiformis being proportionally longer. The pygidium of M. johnstoni in the majority of specimens is more distinct and protruding in comparison with the other species. The anus of M. minuta is located closer to the triangular posterior margin, with the pygidium being somewhat constricted and pygidial cirri more slender (Figures 8C and 10F). The anus of the holotype of M. sacculata is ventrally located and most similar in appearance to M. mirabilis (Figure 8B); the posterior margin is rounded to sub-triangular with two lateral digitiform cirri.

Fig. 7. Magelona alleni Irish Sea; Rame, Plymouth respectively (A, B, C: NMW.Z.1969.104.1094; D, E: Paratype BMNH 1958.5.2.1): (A) posterior region and pygidium, showing terminal anus (dorso-lateral view); (B) prostomium, palps (regenerating) and thorax in papery tube with some sand grain coverings (dorsal view); (C) ~25 chaetigers of posterior end, eggs visible from the 25th to 17th chaetiger from the pygidium, and balls of sediment with some foraminiferans visible in the last 17 chaetigers (dorsal view, tube evident on several chaetigers); (D) ~12 chaetigers of posterior end and pygidium (dorsal view); (E) ~6 chaetigers of posterior region and pygidium, showing terminal anus (ventro-lateral view) (A–C rose bengal stained).

Fig. 8. Variation in the posterior regions and pygidia of European Magelona species (A: NMW.Z 2013.037.0008; B: NMW.Z.1999.027.0001; C: NMW.Z.1991.075.1583; D: NMW.Z.2003.047.5939 E: paratyp|e BMNH 1958.5.2.1): (A) Magelona johnstoni Berwick-upon-Tweed (ventral view); (B) Magelona mirabilis East Sands, Fife, Scotland (ventral view); (C) Magelona minuta Irish Sea (ventro-lateral view); (D) Magelona filiformis Outer Bristol Channel (ventro-lateral view); (E) Magelona alleni paratype (ventro-lateral view). All methyl green stained.
In stark contrast, the anus of M. alleni is terminally placed (Figures 7A, E, 8E, 9A C, 10C), with a conspicuous, large opening. The lateral edges of the pygidium are expanded as wide-based, robust triangular projections (Figure 8E), but the projections of the paratype (Figure 7D, E) are somewhat less distinct. Although not entirely clear from Wilson's original drawing, it appears the projections of the paratype were originally longer, and now broken.
Discussion
Observations herein suggest that M. alleni is predominately a surface deposit feeder. This is in line with observations of M. johnstoni by Mortimer & Mackie (Reference Mortimer and Mackie2014), and similarly Fauchald & Jumars (Reference Fauchald and Jumars1979) regarded magelonids as motile surface deposit-feeders. However, Jumars et al. (Reference Jumars, Dorgan and Lindsay2015) suggested that whilst surface deposit feeding may occur in constrained laboratory settings, subsurface feeding is likely to be more common within the family. These conclusions were primarily based on observations of Magelona pitelkai Hartman, Reference Hartman1944 (Dorgan, pers. obs), which deployed and undulated palps in voids within the sediment. The authors noted that the rarity of observations of magelonid palps above the sediment-water interface by divers and bottom cameras added support for subsurface feeding. However, given the size of palps (which are difficult to observe without microscopes or macro photography) and quick responses elicited to vibrational stimuli (Jones, Reference Jones1968; Mortimer & Mackie, Reference Mortimer and Mackie2014), it is unlikely they would be observed without specialist equipment in these environments. Jumars et al. (Reference Jumars, Dorgan and Lindsay2015) additionally proposed that the low uptake of 13C-labelled phytoplankton by M. mirabilis (Kamp & Witte, Reference Kamp and Witte2005) is evidence for subsurface feeding. The lack of surface deposit feeding in the same species observed by Mortimer & Mackie (Reference Mortimer and Mackie2014) is perhaps consistent with these ideas for M. mirabilis. Suspension feeding has also been suggested to occur within the Magelonidae, although Fauchald & Jumars (Reference Fauchald and Jumars1979) stipulated this was unlikely given observations by McMahon & Jones (Reference McMahon and Jones1967) and Jones (Reference Jones1968). However, neither publication directly stated where particles were captured, whether on the sediment surface or within the water column. Current observations of M. alleni and those of M. johnstoni by Mortimer & Mackie (Reference Mortimer and Mackie2014) lend support for suspension feeding at least occurring sporadically within the family.
The environmental conditions in which observations are made may impact which feeding mode is utilized by a given species. For example, Jumars et al. (Reference Jumars, Dorgan and Lindsay2015) postulated that surface deposit feeding might be undertaken if insufficient room is available for subsurface feeding, and the relationship between the two feeding modes may be linked to flow rate and concentration of suspended particles. Taghon et al. (Reference Taghon, Nowell and Jumars1980), based on observations of spionids in varying water velocities, concluded that in dynamically variable environments, organisms capable of switching feeding behaviours might be common. Thus laboratory conditions may influence the feeding mode utilized by a particular species and must therefore be considered when observations are made. However, concurrent observations of four magelonid species (M. alleni, M. filiformis, M. johnstoni and M. mirabilis) under the same conditions by the current authors have revealed that varying feeding modes predominate in different species. For instance, M. johnstoni and M. alleni were observed to primarily surface deposit feed, and to a lesser extent suspension feed. While neither feeding modes were observed in M. mirabilis (Mortimer & Mackie, Reference Mortimer and Mackie2014), despite morphological similarities to the former species.
The current paper adds credence to the idea that multiple feeding modes exist within the family. Subsurface deposit feeding is perhaps less likely for tubicolous magelonid species such as M. alleni, with tubes reducing access to surrounding sediment. However, some arenicolids are known to use pumping actions to bring food down within their burrows (Wethey et al., Reference Wethey, Woodin, Volkenborn and Reise2008), although these are species without palps, and at present this has not been suggested for magelonids. Subsurface feeding may be more prevalent for magelonid species that do not construct permanent tubes, such as M. pitelkai and M. mirabilis, as suggested by Jumars et al. (Reference Jumars, Dorgan and Lindsay2015). Additional studies are warranted to further investigate the relationship between species’ motilities and feeding mode.
The ingestion of sediment by M. alleni concurs with the diets recorded for other magelonid species: M. mirabilis (Mortimer & Mackie, Reference Mortimer and Mackie2014), Magelona variolamellata Bolívar & Lana, Reference Bolívar and Lana1986 (Magalhães & Barros, Reference Magalhães and Barros2011) and Magelona pettiboneae Jones, Reference Jones1963 (Dauer, Reference Dauer1980). Earlier records of sediment ingestion in European Magelona (McIntosh, Reference McIntosh1911; Hunt, Reference Hunt1925) also exist, although a degree of uncertainty remains as to which species were observed (see Fauchald & Jumars, Reference Fauchald and Jumars1979; Fiege et al., Reference Fiege, Licher and Mackie2000). Mare's (Reference Mare1942) description of the gut contents of M. alleni (as Magelona sp., later referred to M. alleni by Wilson, Reference Wilson1958: 617) as silt alongside small crustacean fragments, at least in part concurs with current observations. Other records have noted the magelonid diet as containing detritus and debris (Jones, Reference Jones1968; Hartmann-Schröder, Reference Hartmann-Schröder1971; Wolff, Reference Wolff1973; Kühl, Reference Kühl1974), and both Fauchald & Jumars (Reference Fauchald and Jumars1979) and Jumars et al. (Reference Jumars, Dorgan and Lindsay2015) proposed a greater tendency towards carnivory. Magelonids have been suggested to be highly selective (Fauchald & Jumars, Reference Fauchald and Jumars1979; Mortimer & Mackie, Reference Mortimer and Mackie2014; Jumars et al., Reference Jumars, Dorgan and Lindsay2015), although this was not observed for M. alleni. While knowledge of species-specific diets for magelonids are lacking, the current authors have noted stark differences between M. johnstoni and M. alleni (pers. obs) observed in the same experimental set up. Further studies are warranted with an increased number of diets and species. However, these observations add credence to the idea that variation in diets within the family exists.
Particle transfer along the palps of M. alleni was similar to that described for both M. johnstoni (Mortimer & Mackie, Reference Mortimer and Mackie2014) and Magelona sp. (Jones, Reference Jones1968), yet, the following differences in palp behaviours were noted. The palps of the former species stayed fairly consistently within the water column, whilst those of the latter two species only emerged in direct response to administered food. This may be explained by variation in burrowing activities between them, and tube habitation for the former species. It has been suggested that magelonid palps have a secondary respiratory function (McIntosh, Reference McIntosh1911; Jones, Reference Jones1968). Thus the placement of palps continually above the sediment-water interface may aid respiration in a tubicolous species, such as M. alleni. In contrast, the palps of the motile M. johnstoni and M. mirabilis trail behind the prostomium during almost continual burrowing (Mortimer & Mackie, Reference Mortimer and Mackie2014). Secondly, the anterior of M. alleni sat closer to the sediment-water interface than M. johnstoni and M. mirabilis during feeding, but this is most likely explained by the relative shortness of the palps of the former species. Lastly, the ‘knotting’ of palps in M. alleni, associated with collecting larger particles, is a behaviour previously undescribed. This may relate to the consumption of sediment, and may explain the lack of observations in other species known to consume mostly detritus and debris. The morphological differences between the palps of M. alleni and other European species, as highlighted within (i.e. shorter, wider, more densely papillated, with longer papillae, and with a shorter non-papillated region), may be linked to differences in both diet and feeding mode. Future studies looking at the relationship between the two are necessary to shed light on this.
The coiling of palps has been commonly documented in some polychaete families as a form of passive suspension feeding (Jumars et al., Reference Jumars, Dorgan and Lindsay2015). Whilst this was shown to accelerate particle transfer in M. johnstoni (Mortimer & Mackie, Reference Mortimer and Mackie2014), no particles were observed in relation to this behaviour in M. alleni. However, this may be due to the flow velocity and concentration of suspended matter within the tank environment, as has been noted for spionids by Taghon et al. (Reference Taghon, Nowell and Jumars1980). Previous studies have noted the involvement of a mucus string (McMahon & Jones, Reference McMahon and Jones1967; Jones, Reference Jones1968) in the transport of particles across the non-papillated region; this was undetected for M. alleni in the current study.
Observations of M. alleni remaining within a branched burrow network for at least three months is in stark contrast to the continual burrowing seen in other magelonids in laboratory aquariums (Mortimer & Mackie, Reference Mortimer and Mackie2014 and current authors pers. obs), and what has commonly been described for the group (e.g. Fauchald & Jumars, Reference Fauchald and Jumars1979; Jumars et al., Reference Jumars, Dorgan and Lindsay2015). Personal communications from Andrew Mackie (NMW) of branched tubes for M. alleni collected off Plymouth add credence to these networks being a natural occurrence for the species. Magelona alleni belongs to the ‘Magelona cincta’ group of species, all of which are reported to construct distinct tubes, tend to possess a fewer number of chaetigers, be stout (Figure 9B, D) and carry pigment in the posterior thorax. The permanency of burrow networks is perhaps not surprising given the tubicolous nature of the species, and the assignment of some magelonid species to the discrete motility guild by Jumars et al. (Reference Jumars, Dorgan and Lindsay2015) (see their figure 1) is in partial agreement with this. Although the tanks utilized in the current study were relatively small, other magelonid species observed in the same sized tanks under the same conditions were shown to burrow more or less continually, thus suggesting that the lack of burrowing observed for M. alleni is related to its tube-dwelling lifestyle.

Fig. 9. Magelona alleni (A, C, paratype, BMNH.1958.2.2; B, E, paratype, BMNH.1958.2.3; D: holotype, BMNH 1958.5.2.1): (A) posterior region and pygidium (dorsal view); (B) prostomium and chaetigers 1–14 (dorsal view, N.B. Lateral edges of prostomium laterally expanded); (C) posterior region and pygidium (dorso-lateral view); (D) prostomium and chaetigers 1–3 (dorsal view, left-hand palp retained but damaged); (E) distal portion of palp (papillae long and numerous, facing upwards).

Fig. 10. European Magelona species (A, B: NMW.Z.2012.022.0001; C: NMW.Z.1969.104.1094; D: NMW.Z.2002.017.0133; E: NMW.Z.2013.037.0023; F: NMW.Z.1991.075.1584): (A) distal region of a Magelona alleni palp, Swansea Bay; (B) prostomium and first two chaetigers (dorsal view); (C) pygidium and terminal anus of Magelona alleni, Irish Sea (posterio-lateral view); (D) posterior region of Magelona johnstoni, Berwick-upon-Tweed (ventral view); (E) posterior region of Magelona mirabilis, Berwick-upon-Tweed (ventral view); (F) posterior region of Magelona minuta, Irish Sea (ventro-lateral view). Position of the anus marked in E, F.
Defecation has not been previously recorded for any magelonid, despite extensive observations of several British species over a four-year period by the second author. However, species that burrow continuously are more likely to excrete waste as they move, while tubicolous annelids may employ a variety of mechanisms to remove waste products from their tubes. Sabellids for example, utilize a faecal groove to pass faeces from the anus to the thorax, which is then ejected using cilia (Rouse, Reference Rouse, Rouse and Pleijel2001b). The lugworm Arenicola marina (Linnaeus, Reference Linnaeus1758), by contrast, moves backwards until the posterior region is just outside the burrow, ejecting faecal cylinders (Darbyshire, Reference Darbyshire, Purschke and Westheide2017) in a similar way to M. alleni, thus suggesting that the method of defecation in the latter species may also be linked to its tubicolous lifestyle.
Mucus involvement in excretion has been reported in other non-magelonid species. For example, Harris (Reference Harris1991) suggested that with high rates of sand ingestion in Ophelia bicornis Savigny, Reference Savigny1822, mucus might aid rapid removal, avoiding compaction in the gut. Therefore, mucus utilized during sand expulsion in M. alleni may be linked to the high rates of sediment ingestion observed.
Perhaps due to their relative uniformity (Rouse, Reference Rouse, Rouse and Pleijel2001a), characterizations of feeding and motility for magelonids have been generalized for the family based on a low number of species. However, multiple feeding modes, differences in motility and variation in defecation methods between species are a distinct possibility for the group. Additionally, no real attempts have been made to link behavioural observations to species’ morphology. The variation in the pygidia of M. alleni in comparison to all other British species may be linked to differences in diets. The large, terminally located anus in M. alleni would seem advantageous for a high excretion rate of mostly large sand grains, in contrast to the small ventrally located anus of M. johnstoni, a species observed to consume primarily detritus (Mortimer & Mackie, Reference Mortimer and Mackie2014). Although Rouse (Reference Rouse, Rouse and Pleijel2001a) stated that the anus of magelonids is terminal, this is not a view shared by the authors for the majority of magelonid species. Rouse's description was primarily based on Hartman's (Reference Hartman1961) drawing of M. sacculata, whose anal position has now been confirmed to be distinctly ventral. At present M. alleni is the only magelonid known to have a terminal anus. While additional investigations corroborating the link between pygidal morphology and species-specific diets are needed, it is clearly evident that a generalized mode of life for magelonids cannot be made.
In summary:
1. Surface deposit feeding, and to a lesser extent suspension feeding, have been observed to predominate in M. alleni.
2. Magelona alleni consumed primarily sand grains and did not appear to be highly selective.
3. Palps remained above the sediment-water interface almost continuously, and this may be linked to respiratory needs.
4. The expulsion of sand is achieved by protrusion of the posterior region from the burrow.
5. Posterior morphology of M. alleni differs to all known British magelonid species, and is currently the only species known to have a terminal anus.
6. Further studies are needed to investigate whether laboratory conditions reflect natural behaviours.
7. The tubicolous lifestyle and permanency of burrows in M. alleni is a probable explanation for variation in behaviours observed in comparison to other magelonid species.
Acknowledgements
We would like to acknowledge the following people for their help in preparation of this paper. Andrew Mackie (Honorary Research Fellow, Amgueddfa Cymru – National Museum Wales) for help with collecting animals and provision of images. James Turner and Jana Horák (Amgueddfa Cymru) for help with time-lapse photography, and help with Kimberley Mills’ Professional Training Year Placement at the Museum respectively. Leslie Harris (Los Angeles Museum of Natural History) and Emma Sherlock (Natural History Museum, London) for the loan of the type material of M. sacculata and M. alleni respectively. The crew of the MBA's RV ‘Sepia’ for help in collection of animals for observation. The authors would like to thank the reviewers whose comments undoubtedly improved the manuscript.