Introduction
Understanding the status of marine turtle populations and anthropogenic threats that may impact their survival is important for effective conservation (Hamann et al., Reference Hamann, Godfrey, Seminoff, Arthur, Barata, Bjorndal, Bolten, Broderick, Campbell, Carreras, Casale, Chaloupka, Chan, Coyne, Crowder, Diez, Dutton, Epperly, FitzSimmons, Formia, Girondot, Hays, Cheng, Kaska, Lewison, Mortimer, Nichols, Reina, Shanker, Spotila, Tomas, Wallace, Work, Zbinden and Godley2010). These are particularly important for marine turtles due to their complex life histories and the use of a variety of spatially discrete habitats over extended lifespans (Musick & Limpus, Reference Musick, Limpus, Lutz and Musick1997). Additionally, marine turtles face a number of threats including fisheries bycatch (Casale, Reference Casale2011; Casale et al., Reference Casale, Abitsi, Aboro, Agamboue, Agbode, Allela, Angueko, Nguema, Boussamba, Cardiec and Chartrain2017), climate change (Hawkes et al., Reference Hawkes, Broderick, Godfrey and Godley2007; Fuentes et al., Reference Fuentes, Limpus and Hamann2011), direct take (Hancock et al., Reference Hancock, Furtado, Merino, Godley and Nuno2017), pollution (Lutcavage et al., Reference Lutcavage, Lutz, Bossart and Hudson1995; Duncan et al., Reference Duncan, Botterell, Broderick, Galloway, Lindeque, Nuno and Godley2017), disease (Patrício et al., Reference Patrício, Diez, van Dam and Godley2016) and coastal development (Harewood & Horrocks, Reference Harewood and Horrocks2008; Fuentes et al., Reference Fuentes, Gredzens, Bateman, Boettcher, Ceriani, Godfrey, Helmers, Ingram, Kamrowski, Pate and Pressey2016).
In the waters surrounding the UK and Ireland, six species of marine turtle have been documented, including green turtle (Chelonia mydas), hawksbill turtle (Eretmochelys imbricata), Kemp's ridley turtle (Lepidochelys kempii), leatherback turtle (Dermochelys coriacea), loggerhead turtle (Caretta caretta) and olive ridley turtle (Lepidochelys olivacea) (Brongersma, Reference Brongersma1972; Witt et al., Reference Witt, Penrose and Godley2007b; Doyle et al., Reference Doyle, Houghton, O'Suilleabhain, Hobson, Marnell, Davenport and Hays2008). Leatherback turtles, the majority adults, seasonally frequent the waters around the UK and Ireland during the boreal summer and autumn as it is a foraging ground for their gelatinous prey (Godley et al., Reference Godley, Gaywood, Law, McCarthy, McKenzie, Patterson, Penrose, Reid and Ross1998; Witt et al., Reference Witt, Broderick, Johns, Martin, Penrose, Hoogmoed and Godley2007a). This species nests on tropical and subtropical beaches, with adults undertaking extensive migrations across oceans before returning to their nesting beaches every 2–4 years (McDonald & Dutton, Reference McDonald and Dutton1996; Fossette et al., Reference Fossette, Girard, Lopez-Mendilaharsu, Miller, Domingo, Evans, Kelle, Plot, Prosdocimi, Verhage and Gaspar2010). In addition, hard-shell turtle species at the extremities of their ranges including loggerhead and Kemp's ridley turtles, the majority juveniles, are occasionally found around the UK and Ireland (Witt et al., Reference Witt, Penrose and Godley2007b). Upon hatching from nests on coastal beaches, young turtles enter the coastal waters and migrate offshore where they continue to grow and develop in oceanic habitats (Mansfield et al., Reference Mansfield, Wyneken, Porter and Luo2014; Putman & Mansfield, Reference Putman and Mansfield2015). Juvenile oceanic marine turtles are rarely observed and as such this stage of their life cycle remains the least described in terms of occurrence, distribution and abundance. These turtles are likely to originate from rookeries in the North-west Atlantic (loggerhead turtles) and the Gulf of Mexico (Kemp's ridley turtles) whereby they enter the North Atlantic Subtropical Gyre as part of their juvenile oceanic developmental stage (Carr, Reference Carr1987; Collard & Ogren, Reference Collard and Ogren1990; Bolten, Reference Bolten, Bolten and Witherington2003).
It was previously thought that juvenile turtles were passive migrants in the currents; however, a growing body of research has documented active dispersal of juvenile loggerhead, green and Kemp's ridley turtles, suggesting that currents may not necessarily be the primary driver of dispersal (e.g. Mansfield et al., Reference Mansfield, Wyneken, Porter and Luo2014; Putman & Mansfield, Reference Putman and Mansfield2015). Post-hatchling turtles, such as loggerhead and Kemp's ridley turtles, enter the North Atlantic gyre and disperse across to the east Atlantic Ocean where the Azores, Madeira and Canary Islands are developmental grounds (Carr, Reference Carr1987; Collard & Ogren, Reference Collard and Ogren1990; Bolten, Reference Bolten, Bolten and Witherington2003). From here there are several currents that could facilitate turtles reaching the European continental shelf, including the Azores current and the North Atlantic current (Witt et al., Reference Witt, Penrose and Godley2007b). The duration of this juvenile oceanic phase is highly variable. Some growth models for loggerhead turtles estimate it to last between 12 and 13 years until they return to neritic habitats in the North-west Atlantic reaching a mean straight carapace length (SCL) of 55.3 cm (Avens et al., Reference Avens, Goshe, Pajuelo, Bjorndal, MacDonald, Lemons, Bolten and Seminoff2013).
The small size, fast growth rate and migratory behaviour of juvenile marine turtles complicate efforts to monitor them in the open ocean (Mansfield et al., Reference Mansfield, Wyneken, Porter and Luo2014). The use of strandings data can provide important information on the occurrence and spatio-temporal trends of these life stages (Witt et al., Reference Witt, Penrose and Godley2007b; Casale et al., Reference Casale, Affronte, Insacco, Freggi, Vallini, D'Astore, Basso, Paolillo, Abbate and Argano2010; Leeney et al., Reference Leeney, Witt, Broderick, Buchanan, Jarvis, Richardson and Godley2012; Fleming et al., Reference Fleming, Harrod and Houghton2013; Vélez-Rubio et al., Reference Vélez-Rubio, Estrades, Fallabrino and Tomás2013; Meynecke & Meager, Reference Meynecke and Meager2016; Nicolau et al., Reference Nicolau, Ferreira, Santos, Araújo, Sequeira, Vingada, Eira and Marçalo2016). There are limitations in the use of strandings data, however, due to annual variation in recording with associated unquantifiable survey effort and oceanographic/biological factors such as ocean currents and decomposition rates that affect the probability and location of turtle strandings (Hart et al., Reference Hart, Mooreside and Crowder2006; Tomás et al., Reference Tomás, Gozalbes, Raga and Godley2008). However, strandings data represent a useful opportunity for research, informing knowledge on species distribution, aiding the assessment of difficult to access life stages and highlighting impacts of anthropogenic threats (Witt et al., Reference Witt, Penrose and Godley2007b; Chaloupka et al., Reference Chaloupka, Work, Balazs, Murakawa and Morris2008; Casale et al., Reference Casale, Affronte, Insacco, Freggi, Vallini, D'Astore, Basso, Paolillo, Abbate and Argano2010).
In this study we analyse almost 2000 records of marine turtle sightings, strandings and captures around the UK and Ireland, collected from over a century (1910–2018). We extend the research conducted by Witt et al. (Reference Witt, Penrose and Godley2007b) on the spatial and temporal trends for sightings, strandings and captures of hard-shell marine turtles and leatherback turtles around the UK and Ireland. With an additional 15 years of data we investigate how previous decadal and annual trends have either continued or changed, provide additional information regarding the spatial and temporal distribution of these species and explore contextualizing data, such as morphometric information and anthropogenic interactions, to further understand their presence and the threats they face in the temperate waters of the UK and Ireland.
Materials and methods
Turtle project database
Records of sightings, strandings and captures of marine turtles from around the UK and Ireland were obtained from the TURTLE database operated by Marine Environmental Monitoring (Penrose, Reference Penrose2019). Initiated in 2001, TURTLE is a multi-agency initiative that records marine turtle sightings, strandings and captures. Each record, where possible, includes the species, date, whether it is a sighting, stranding or capture, whether the turtle was dead or alive, geographic location, size and any additional information such as any injuries, how it was captured or found and whether a post-mortem has been conducted.
Reports are submitted to Marine Environmental Monitoring by members of the public, UK Cetacean Strandings Investigation programme (CSIP), Scottish Marine Animal Stranding Scheme (SMASS) and governmental agencies. Morphometric and relevant ancillary data are collected from stranded and captured turtles by these groups and rehabilitation, necropsy or recovery and disposal is arranged. Data are then submitted to the TURTLE coordinator, which are stringently validated to ensure records are accurate and of the highest quality and confidence, these are then added to the database.
The database also contains historic records of marine turtle sightings, strandings and captures since c. 1748. These were sourced from published literature (Brongersma, Reference Brongersma1972; Penhallurick, Reference Penhallurick1990), unpublished data (King, Reference King2005) and governmental records (e.g. from Natural England, Scottish Natural Heritage, Natural Resources Wales, Department of Agriculture, Environment and Rural Affairs (DAERA) Northern Ireland, National Parks and Wildlife Service (NPWS) Ireland and Department of Environment, Food, and Agriculture (DEFA) Isle of Man). These records were validated and subsequently added to the database. We extracted records from the TURTLE database for the period 1910–2018. Prior to 1910 records were sparse and often incomplete.
Mapping and statistical analysis
Land and coastline data were sourced from Natural Earth (http://www.naturalearthdata.com) and imported into ArcGIS 10.2.2 (ESRI, 2011). Geographic location of marine turtle sightings, strandings and captures were provided as GPS coordinates, longitude and latitude or as map references. For our mapping and statistical analysis all geographic location data were converted to longitude and latitude (WGS1984). The software R version 3.1.2 (R Development Core Team, 2014) was used for all statistical and graphical analysis.
Results
For the period 1910–2018, a total of 1997 records of marine turtle sightings (N = 1362; 93.3% live), strandings (N = 492; 23.8% live) and captures (N = 143; 69.2% live) were identified. The dataset contained 1683 leatherback turtle records (79.7% alive) and 314 hard-shell turtle records (48.7% alive). Of these hard-shell turtle records, the majority were loggerhead turtles (N = 240, 76.4% of hard-shell turtle total and 12% of total records) and Kemp's ridley turtles (N = 61, 19.4% of hard-shell turtle total and 3% of total records). Additionally, there were sighting and stranding records of 11 green turtles that occurred between 1980 and 2016. There were single live stranding events of a hawksbill turtle near Cork, Ireland in 1983 and an olive ridley turtle on Anglesey, Wales in 2016. Records reporting capture (N = 134) were of three species only (loggerhead N = 6, Kemp's ridley N = 2, leatherbacks N = 135). In these circumstances, turtles were indirectly captured as bycatch in fishing nets, lines and ropes. For the purposes of statistical analysis these capture records were combined with sightings and strandings.
Decadal and annual patterns
Decadal patterns of sightings and strandings of loggerhead, Kemp's ridley and leatherback turtles in the UK and Ireland revealed different patterns of occurrence for each species (Figure 1a–c).
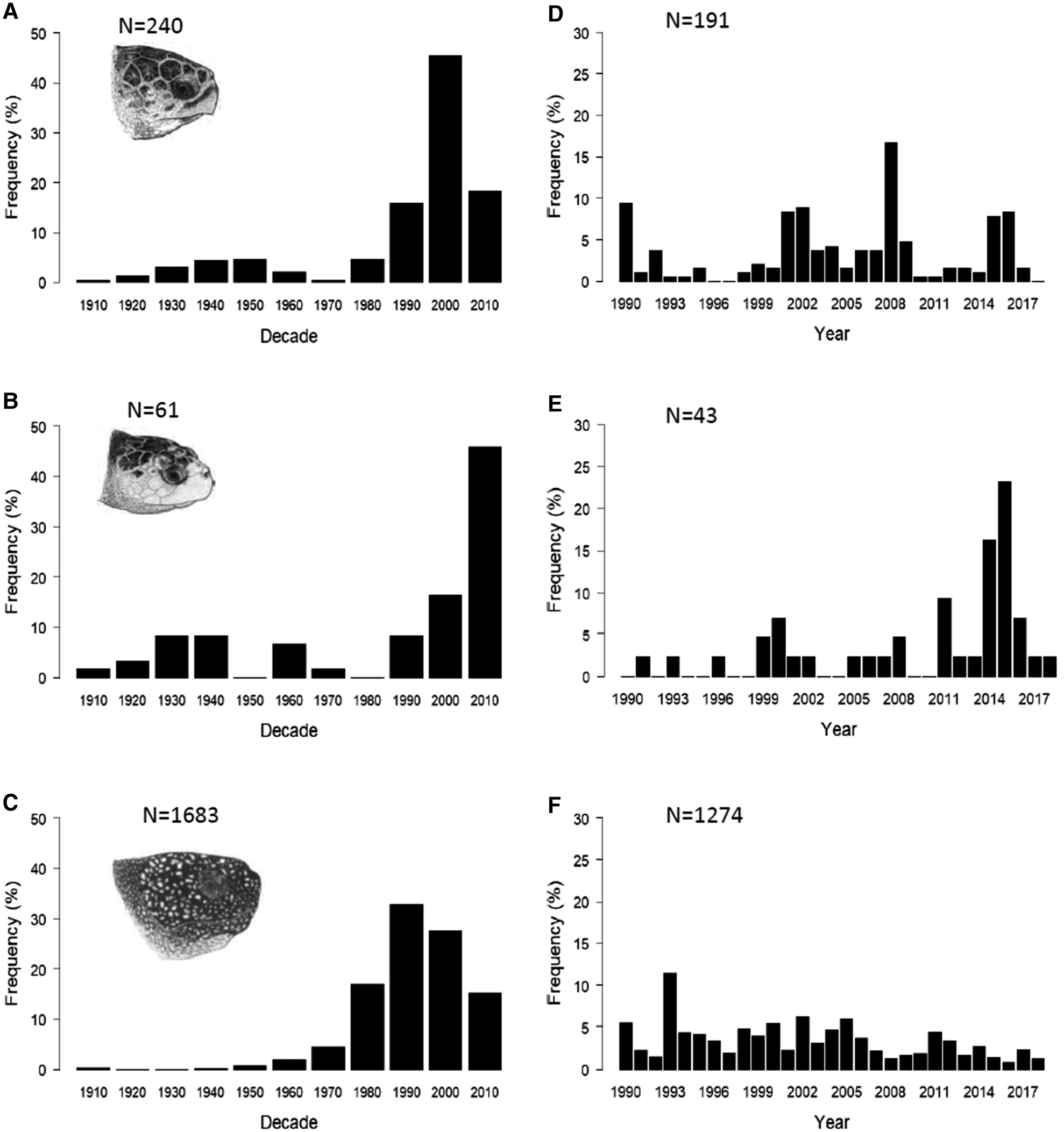
Fig. 1. Temporal distribution of loggerhead, Kemp's ridley and leatherback turtle sightings, strandings and capture records in the UK and Ireland, 1910–2018 (incomplete decade 2010–2018): (a) loggerhead turtles (N = 240), (b) Kemp's ridley turtles (n = 61), (c) leatherback turtles (N = 1683). Recent (1990–2018) annual distribution of loggerhead, Kemp's ridley and leatherback turtles in the UK and Ireland: (d) loggerhead turtles (N = 191), (e) Kemp's ridley turtles (N = 43), (f) leatherback turtles (N = 1274). Marine turtle skull figures used with permission of WIDECAST; original artwork by Tom McFarland.
Loggerhead turtle records increased gradually from the 1910s until the 1950s, decreasing through the 1960s and 1970s. Since the 1980s, records have increased significantly over time (incomplete decade 2010–2018; Spearman's rank rs = 0.8, P < 0.05) although records appear to have since decreased again in the most recent decade (Figure 1a). Kemp's ridley records (Figure 1b) increased between the 1910s and 1930s, remained stable in the 1940s and were then lower and variable until the 1990s with no sightings, strandings or captures in the 1950s or 1980s. From the 1990s, the number of records increased with the 2000–2009 decade experiencing the most records. Despite this increase, we found no significant correlation with time and the decadal number of records for Kemp's ridley turtles (incomplete decade 2010–2018; Spearman's rank rs = 0.4, P > 0.05). A comparison of decadal patterns for loggerhead and Kemp's ridley turtles revealed no correlation (Spearman's rank rs = 0.5, P > 0.05).
The decadal abundance of leatherback turtle records (Figure 1c) was relatively low from 1910–1960, with no records in the 1920s and 30s. From the 1960s, the number of records increased and peaked in the 1990s but decreased in the subsequent two decades. There was, however, a significant positive correlation in the decadal number of records for leatherback turtles over time (incomplete decade 2010–2018; Spearman's rank rs = 0.9, P < 0.05).
Since the majority (75.5%) of turtle sightings, strandings and capture records have occurred since 1990 (Figure 1a–c), we analysed the annual records for each species from 1990 to 2018 (Figure 1d–f). There was no significant correlation in the number of loggerhead turtle records over time (Figure 1d) (Spearman's rank rs = 0.1, P > 0.05) but there was a significant positive correlation with year and the annual number of Kemp's ridley turtle records (Figure 1e) (Spearman's rank rs = 0.5, P < 0.05) and a negative correlation in leatherback turtle records with year (Figure 1f) (Spearman's rank rs = −0.5, P < 0.05). A comparison of the annual records between the two hard-shell species found no significant correlation (Spearman's rank rs = 0.1, P > 0.05).
Spatial distribution
The majority of the sightings, strandings and captures of loggerhead (N = 240, 89%) and Kemp's ridley (N = 61, 88.5%) turtles occurred on the western aspect of the UK and Ireland, and along the English Channel coast (Figure 2a–f). Likewise, the majority of the sightings, strandings and captures of leatherback turtles (N = 1683, 93.4%) occurred on western facing aspects, along the English Channel but also along the entirety of Ireland's coastline, including in the Irish Sea (Figure 2g–i).
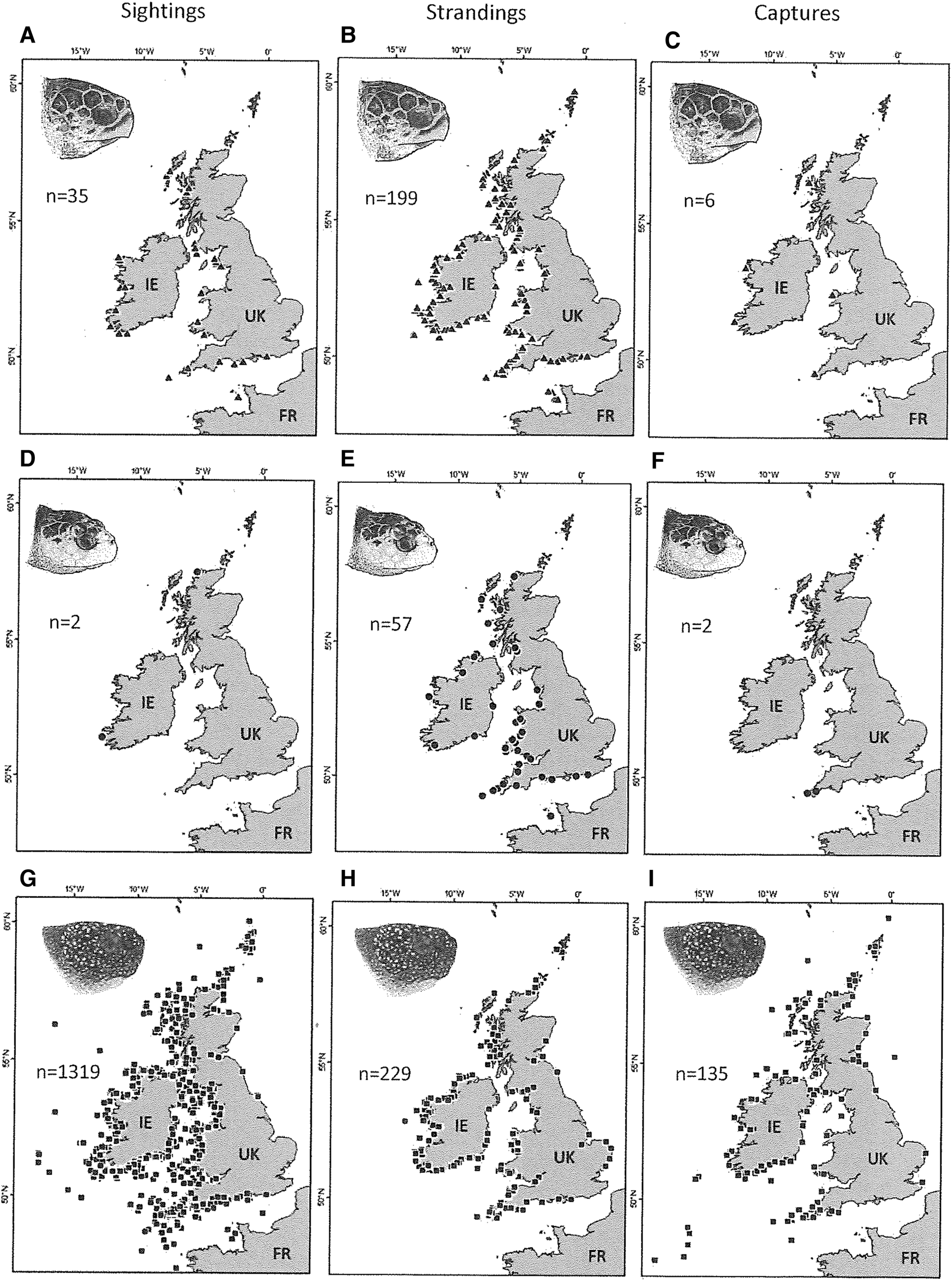
Fig. 2. The distribution of sightings, strandings and capture records for loggerhead turtles (a) sightings N = 35, (b) strandings N = 199, (c) captures N = 6), Kemp's ridley turtles (d) sightings N = 2, (e) strandings N = 59, (f) captures N = 2) and leatherback turtles (g) sightings N = 1319, (h) strandings N = 229, (i) captures N = 135) around the UK and Ireland (1910–2018). Sea turtle skull figures used with permission of WIDECAST; original artwork by Tom McFarland.
The numbers of records of all species were negatively correlated with increasing latitude; Kemp's ridley turtles (Pearson r = −0.38, P < 0.05), loggerhead turtles (Pearson r = −0.52, P < 0.05) and leatherback turtles (Pearson r = −0.19, P < 0.05) (Figure S1 in supplementary material).
There was no significant correlation between mortality (number of turtle records reported as dead) and latitude in all three turtle species; loggerhead turtles (Spearman's rank rs = 0.1, P > 0.05), Kemp's ridley (Spearman's rank rs = 0.2, P > 0.05) and leatherback turtles (Spearman's rank rs = −0.0007, P > 0.05) (Figure S2 in supplementary material).
Seasonal patterns
Loggerhead and leatherback turtle records occurred throughout the year, with the highest occurrence of loggerheads between November and March (Figure 3a), and leatherbacks between June and October (Figure 3c). Kemp's ridley records only occurred between October and February (Figure 3b).
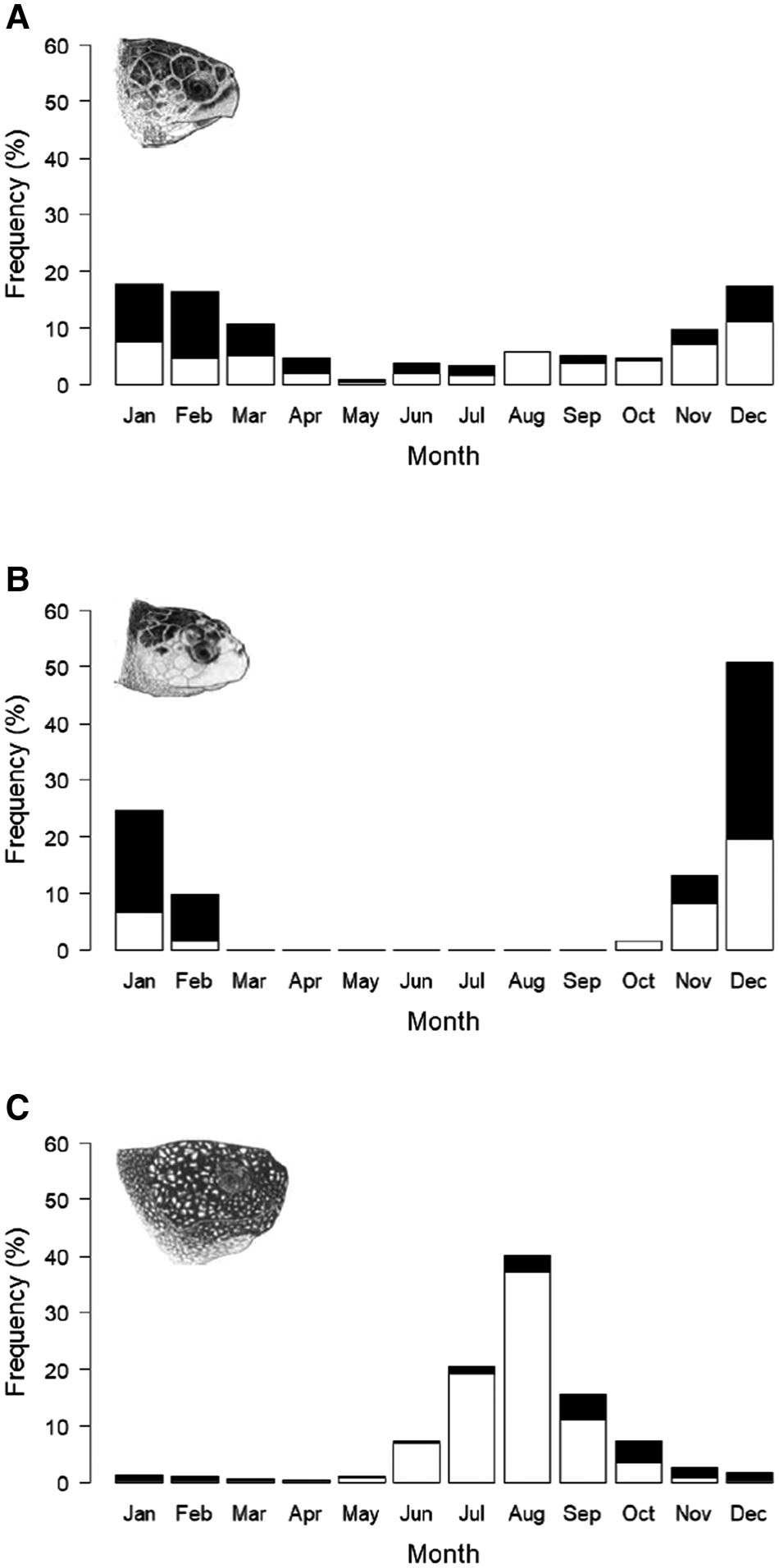
Fig. 3. Cumulative monthly frequency of sightings, stranding and capture records from the UK and Ireland 1910–2018; records of live turtles (open bars) and records of dead turtles (filled bars): (a) loggerhead turtles (N = 237), (b) Kemp's ridley turtles (N = 61), (c) leatherback turtle (N = 1646). Chi squared statistical tests show a significant seasonal pattern in each species; loggerhead turtle (χ2(109.9), P < 0.001), Kemp's ridley turtle (χ2(192.2), P < 0.001) and leatherback turtle (χ2(3087), P < 0.001). Chi squared tests also show a significant seasonal pattern in the number of dead turtles in each species; loggerhead (χ2(121.6), P < 0.001), Kemp's ridley turtles (χ2(86.5), P < 0.001) and leatherback turtles (χ2(1134.5), P < 0.001). Marine turtle skull figures used with permission of WIDECAST; original artwork by Tom McFarland.
Whilst records of dead loggerhead turtles occurred throughout the year except for August, the frequency increased during the highest occurrence of conspecific turtle records between December and April (Figure 3a). Similarly, records of dead Kemp's ridley turtles also increase during the highest occurrence of conspecific turtles records between December and February (Figure 3b). Records of living leatherback turtles were only recorded between May and November, whilst dead leatherbacks were recorded throughout the year with the highest frequency in September and October (Figure 3c).
Body size
A total of 117 hard-shell turtle stranding and capture records from the UK and Ireland had associated data on straight carapace length (SCL); of these 86 were measurements of loggerhead turtles (mean 29.6 cm ± 16.3 SD, range 11–85 cm) and 31 were measurements of Kemp's ridley turtles (mean 27.3 cm ± 10.1 SD, range 9–62 cm) (Figure 4a, b). Whilst growth rates in these two species can be influenced by environmental and genetic factors, with typically much higher rates in the first year, 5 cm increments were selected as the size increment as it is estimated to be 1–2 years growth (Bjorndal et al., Reference Bjorndal, Bolton and Martins2000; Snover et al., Reference Snover, Hohn, Crowder and Heppell2007). Typical SCL measurements estimated for adults at maturation in the North Atlantic range between 75–103 cm for loggerhead turtles (Avens et al., Reference Avens, Goshe, Coggins, Snover, Pajuelo, Bjorndal and Bolten2015) and 53–69 cm for Kemp's ridley turtles (Avens et al., Reference Avens, Goshe, Coggins, Shaver, Higgins, Landry and Bailey2017). The modal size group for loggerhead turtles was between 15.1 and 20 cm and for Kemp's ridley turtles was between 20.1 and 25 cm, indicating that the majority were almost certainly juvenile turtles. These stranding and capture records also contained the reported status of the turtle; loggerhead: 58 alive, 28 dead, and Kemp's ridley: 19 alive, 12 dead. There was no significant difference in the median SCL of animals recorded as dead or alive for loggerhead turtles (Mann–Whitney U = 782.5, P > 0.05, median: dead 21.4 cm, alive 23 cm) or for Kemp's ridley turtles (Mann–Whitney U = 121.5, P > 0.05, median: dead 24.5 cm, alive 25.2 cm).
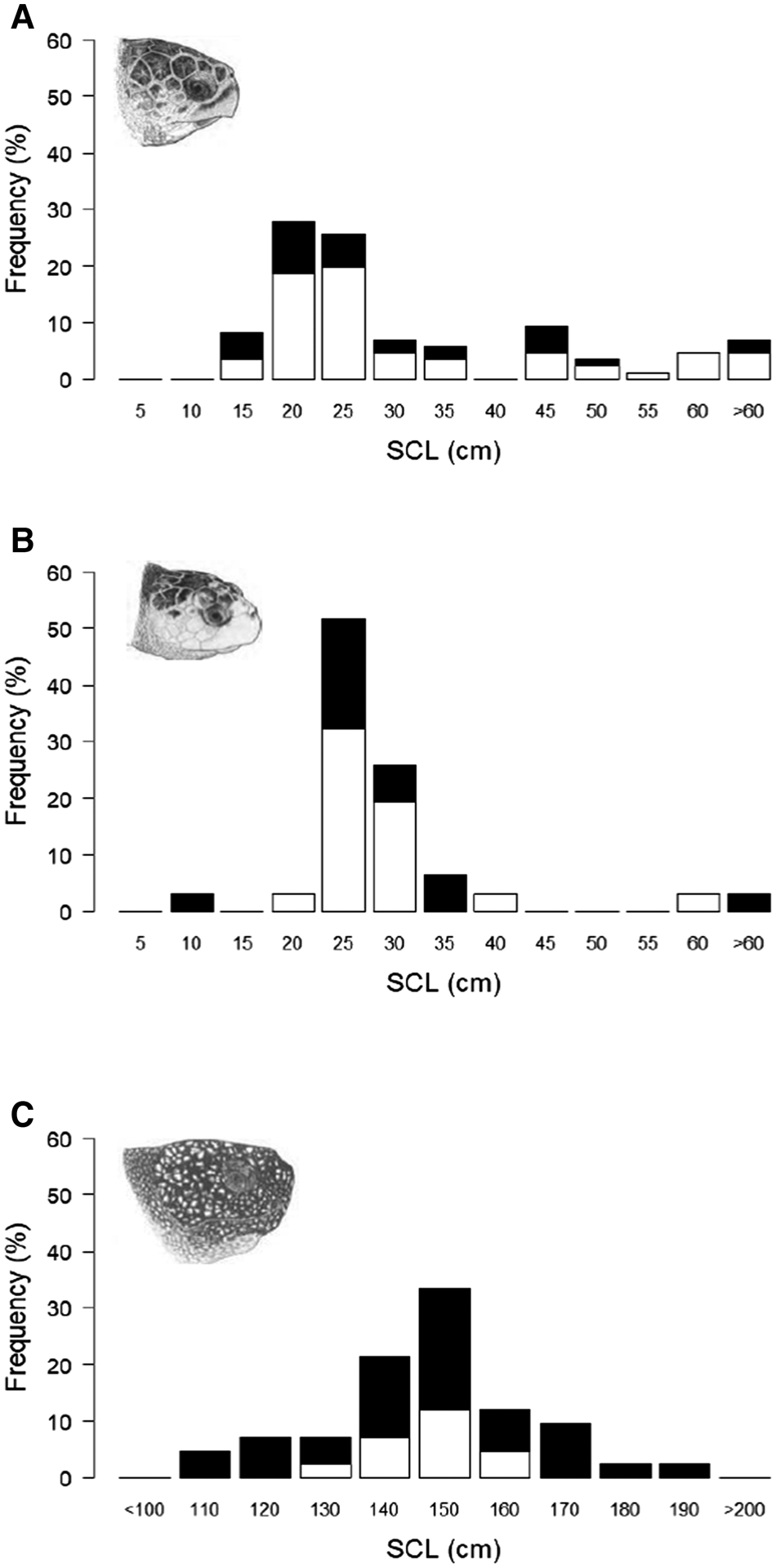
Fig. 4. Straight carapace length (SCL) distribution from loggerhead, Kemp's ridley and leatherback turtle stranding and capture records in the UK and Ireland 1910–2018: (a) loggerhead turtles (N = 86), (b) Kemps' ridley turtles (N = 31), (c) leatherback turtles (n = 48). Marine turtle skull figures used with permission of WIDECAST; original artwork by Tom McFarland.
There were 48 records that contained data on leatherback turtle body size (SCL; mean 144 cm ± 27.1 SD, range 80–229 cm) (Figure 4c). Curved carapace length (CCL) measurements estimated for adults at maturation in the North Atlantic range between 112–153 cm (108–148 cm SCL using conversion equation in Avens et al., Reference Avens, Taylor, Goshe, Jones and Hastings2009; Avens et al., Reference Avens, Goshe, Zug, Balazs, Benson and Harris2020). The modal size class was between 140.1 and 150 cm, indicating that the majority of records were probably adults. As with hard-shell turtles, growth rates can be influenced by environmental and genetic factors and since growth rates are slower in adults and records reported over a large size range, 10 cm increments were selected as the size increment (Avens et al., Reference Avens, Taylor, Goshe, Jones and Hastings2009). These stranding and capture records also contained the reported status of the leatherback turtles (13 alive, 35 dead); there was no significant difference in the median SCL of the leatherback turtles recorded as dead or alive (Mann–Whitney U = 241, P > 0.05, median: dead 143 cm, alive 147 cm).
Anthropogenic interactions
Records indicated that 143 marine turtles were indirectly captured as bycatch in fishing nets, ropes and lines (7.2% of total turtle records). This comprised six loggerhead turtles (2.5% of 240 conspecific records; all alive), two Kemp's ridley turtles (3.3% of 61 conspecific records; both alive) and 135 leatherback turtles (8% of 1683 conspecific records; 95 alive). Of the bycaught leatherback turtles, a substantial number were associated with ropes from lobster pots, crab pots and creels (49.6%, 67 of 135), and nets including trawls and drift nets (31.9%, 43 of 135). Spatial incidences of entangled turtles (bycatch) predominantly occur on the western aspect of the UK and Ireland with higher occurrence in south-west England and the southern coast of Ireland (Figure 2c, f, i). Other interactions included 16 leatherback turtles found entangled in ghost gear (six alive) and five leatherback turtles (0.3% of conspecific records, one alive) had injuries consistent with collision with boats and their propellers.
Discussion
Our analysis of nearly 2000 records of sightings, strandings and capture of marine turtles around the UK and Ireland has identified possible decadal, annual and seasonal trends that provide further insights into a poorly understood life history phase of hard-shelled marine turtles and leatherback turtles at high latitudes. Here we speculate as to the significance of some of these patterns.
For loggerhead and Kemp's ridley turtles, the patterns of decadal records potentially reflect the historical events that have impacted the number of nesting females, therefore the total number of clutches laid and subsequently the number of hatchlings recruited each year (Mazaris et al., Reference Mazaris, Schofield, Gkazinou, Almpanidou and Hays2017). For loggerhead turtles, the observed decline in records between 1960 and 1980 is prior to the classification of loggerheads as an endangered species in the USA (1978) whereupon rapid conservation action was implemented. From the 1980s onwards we observed a significant increase in the decadal totals of loggerhead turtle sightings and strandings, which could be the result of increased hatchling recruitment due to successful conservation measures. For the Kemp's ridley turtle there is a similar pattern of decline, with no records in either the 1950s or 1980s; in the following decades the number of records rapidly increases. Prior to 1966, Kemp's ridley eggs were intensively harvested from their main nesting beach, Rancho Nuevo, Mexico, where the population was in swift decline (Hildebrand, Reference Hildebrand1963). This intense harvesting would have repercussions for several decades to come, with the lowest recorded nesting year on Rancho Nuevo in 1985 where only 706 clutches were laid (Witzell et al., Reference Witzell, Burchfield and Pena2007). Since the late 1960s conservation management initiatives in Mexico and at Padre Island, Texas, USA have protected nests and hatchlings (Zwinenberg, Reference Zwinenberg1977). In combination with turtle excluder devices (TEDs) in USA shrimp nets, which reduced the number of nesting females caught, these actions are thought to have increased nesting in the 1990s (Márquez et al., Reference Márquez, Díaz, Sánchez, Burchfield, Leo, Carrasco, Peña, Jiménez and Bravo1999). This rise in the number of nests and subsequent rise in the number of juveniles is reflected in the UK and Irish data presented here up until the most recent decade, where there has been a significant increase in the annual number of Kemp's ridley strandings and sightings since 1990. We believe the decadal and annual patterns that we have observed in the Kemp's ridley turtles augments previous evidence of increased hatchling recruitment (Shaver et al., Reference Shaver, Rubio, Walker, George, Amos, Reich, Jones and Shearer2016).
The 2010 Deepwater Horizon oil spill (DWH) released an estimated 3.2 million barrels of crude oil into the waters of the northern Gulf of Mexico (DWH NRDA Trustees, 2016; Wallace et al., Reference Wallace, Brosnan, McLamb, Rowles, Ruder, Schroeder, Schwacke, Stacy, Sullivan, Takeshita and Wehner2017). The Gulf of Mexico supports a large number of marine vertebrates including five species of marine turtle (including loggerhead and Kemp's ridley) (Stacy et al., Reference Stacy, Field, Staggs, MacLean, Stacy, Keene, Cacela, Pelton, Cray, Kelley and Holmes2017; Ylitalo et al., Reference Ylitalo, Collier, Anulacion, Juaire, Boyer, da Silva, Keene and Stacy2017). Marine turtles can be exposed to contamination in both the marine environment and on nesting beaches by a number of routes including via dermal/skin, inhalation/lungs, incidental consumption and diet (Lutz & Lutcavage, Reference Lutz and Lutcavage1989; Lutcavage et al., Reference Lutcavage, Lutz, Bossart and Hudson1995; Mitchelmore et al., Reference Mitchelmore, Bishop and Collier2017; Wallace et al., Reference Wallace, Stacy, Cuevas, Holyoake, Lara, Marcondes, Miller, Nijkamp, Pilcher, Robinson and Rutherford2020). Turtle mortality directly linked to the DWH event is unknown; however, using data from direct captures during the oil spill, it has estimated that ~30% of turtles that were not heavily oiled would have perished (Mitchelmore et al., Reference Mitchelmore, Bishop and Collier2017). A recent study assessing the annual nesting of Kemp's ridley turtles in Tamaulipas, Mexico, found that the annual number of nests dropped by 35.4% during the 2010 nesting season and remained low for the 2011–2014 nesting seasons, suggesting a large decline in the number of females (Caillouet et al., Reference Caillouet, Gallaway and Putman2016). Large-scale environmental perturbations such as DWH, occurring near to breeding grounds, could influence hatchling production and subsequent stranding rates of juvenile marine turtles in the North-east Atlantic, and this may offer one explanation for the decline in records observed.
The decadal trend of records in the UK and Ireland for leatherback turtles generally increased, peaking in the 1990s from which it has since decreased. The initial observed increase in the records of leatherback sightings and strandings, as for hard-shell turtles too, could be due to increased awareness and promotion of marine vertebrate reporting schemes; however, it could in part reflect increasing numbers of leatherback turtles in the North Atlantic (McGowan et al., Reference McGowan, Broderick, Frett, Gore, Hastings, Pickering, Wheatley, White, Witt and Godley2008; Stewart et al., Reference Stewart, Sims, Meylan, Witherington, Brost and Crowder2011). It is possible that as a result of regional warming the thermal niche of leatherback turtles has expanded so that the waters surrounding the UK and Ireland are a more favourable temperature for longer periods over the boreal summer and autumn and over a larger area (Witt et al., Reference Witt, Broderick, Johns, Martin, Penrose, Hoogmoed and Godley2007a). Likewise this could be a response to changing distribution and abundance of their gelatinous prey (Mills, Reference Mills2001). However, in the last two decades, the sightings, strandings and capture of leatherbacks around the UK and Ireland have declined. The Atlantic leatherback turtle population is comprised of a number of sub-populations with variable conservation status. In comparison to the Pacific leatherback population, which are in precipitous decline (Spotila et al., Reference Spotila, Reina, Steyermark, Plotkin and Paladino2000; Tapilatu et al., Reference Tapilatu, Dutton, Tiwari, Wibbels, Ferdinandus, Iwanggin and Nugroho2013), North Atlantic populations are, for the majority, stable or increasing (Dutton et al., Reference Dutton, Dutton, Chaloupka and Boulon2005; Girondot et al., Reference Girondot, Godfrey, Ponge and Rivalan2007; McGowan et al., Reference McGowan, Broderick, Frett, Gore, Hastings, Pickering, Wheatley, White, Witt and Godley2008; Stewart et al., Reference Stewart, Sims, Meylan, Witherington, Brost and Crowder2011; Colman et al., Reference Colman, Thomé, Almeida, Baptistotte, Barata, Broderick, Ribeiro, Vila-Verde and Godley2019). The increasing intensification of the long-line fisheries in African and Latin American waters, known for substantial turtle bycatch, has been identified as a threat to particularly the South Atlantic leatherback population (Witt et al., Reference Witt, Bonguno, Broderick, Coyne, Formia, Gibudi, Mounguengui, Moussounda, NSafou, Nougessono and Parnell2011; Fossette et al., Reference Fossette, Witt, Miller, Nalovic, Albareda, Almeida, Broderick, Chacón-Chaverri, Coyne, Domingo and Eckert2014). Yet this decline in records from around the UK and Ireland could also be due to reduced reporting effort. The majority of leatherback sightings and captures were reported by fishers. Recent policy measures put in place in a bid to protect dwindling fish stocks included reducing the fishing effort (Engelhard et al., Reference Engelhard, Lynam, Garcia-Carreras, Dolder and Mackinson2015). The reduction in the number of fishing boats operating in the UK and/or a reduced number of days at sea could have therefore decreased the likelihood of people encountering turtles (Greenstreet et al., Reference Greenstreet, Holland, Fraser and Allen2009; Engelhard et al., Reference Engelhard, Lynam, Garcia-Carreras, Dolder and Mackinson2015).
Analysis of the spatial distribution of records for hard-shell turtles indicates that the majority of sightings, strandings and captures occur on the western facing aspects of the UK and Ireland (Figure 2a–f). A similar pattern was observed for leatherback turtle records, with a higher relative abundance of records also extending around the entirety of Ireland's coastline (Figure 2g–i). This highlights the importance of the western coasts of the UK and Ireland and the Irish Sea. For both hard-shell species, the number of records significantly decreased with increasing latitude. The spatial pattern is most likely driven by wind patterns, surface currents, water temperature and coastal morphology (Burke et al., Reference Burke, Standora and Morreale1991; Still et al., Reference Still, Griffin and Prescott2005; Roberts et al., Reference Roberts, Collins, Paxton, Hardy and Downs2014). These factors have also been important in predicting cold-stunning and stranding events along the east coast of the USA (Still et al., Reference Still, Griffin and Prescott2005; Roberts et al., Reference Roberts, Collins, Paxton, Hardy and Downs2014). Since the majority of these hard-shell turtles were stranded or sighted in the boreal winter and spring months, it is likely that some of them have been cold stunned. This occurs when turtles fail to migrate south before the water temperature quickly decreases below their metabolic threshold (Burke et al., Reference Burke, Standora and Morreale1991; Still et al., Reference Still, Griffin and Prescott2005). Wind patterns and surface currents will in part then determine the location of strandings (Hart et al., Reference Hart, Mooreside and Crowder2006). In contrast, leatherback turtle records in the UK and Ireland peak during the boreal summer months when their prey, jellyfish, are at their most plentiful (Houghton et al., Reference Houghton, Doyle, Wilson, Davenport and Hays2006; Pikesley et al., Reference Pikesley, Godley, Ranger, Richardson and Witt2014). Unlike the hard-shelled turtles whose distribution in the UK and Ireland is likely largely due to ocean currents, active habitat selection demonstrated by endothermic leatherbacks will also play an important role in their distribution (Houghton et al., Reference Houghton, Doyle, Wilson, Davenport and Hays2006; Witt et al., Reference Witt, Broderick, Johns, Martin, Penrose, Hoogmoed and Godley2007a).
Analysis of the hard-shell turtle SCL size class distribution indicates that the majority of both loggerhead and Kemp's ridley turtles were juveniles. In comparison, whilst records of leatherback turtle SCL data were sparse, the majority were adults. However, in accordance with previous research it is clear that the majority of the hard-shell turtles recorded around the UK and Ireland are probably ‘first passage’ turtles, having likely dispersed from the nesting beaches in the Americas and the Gulf of Mexico (Hays & Marsh, Reference Hays and Marsh1997). Additionally, there is growing evidence suggesting that the Bay of Biscay is a residency spot for loggerhead turtles (Avens & Amico, Reference Avens and Amico2018). This could explain the presence of larger individuals in the records but also the number of live sightings and strandings that are recorded throughout the year.
Records of other species of hard-shell turtles were very sparse; these included green turtles, a hawksbill turtle and an olive ridley turtle. Whilst these species are present in the North Atlantic, the apparent absence of them in the public recording schemes is probably due to several reasons: lesser physiological tolerance to cooler temperatures at lower latitudes meaning these species rarely reach European waters; inter-species variability in dispersal mechanisms where species could occupy different oceanic features (Caribbean, Sargasso Sea) and a shorter juvenile oceanic phase (Witt et al., Reference Witt, Penrose and Godley2007b).
The analysis of records from public reporting can not only infer trends in occurrence but also highlight anthropogenic threats to marine turtles (Casale et al., Reference Casale, Affronte, Insacco, Freggi, Vallini, D'Astore, Basso, Paolillo, Abbate and Argano2010). From our records an appreciable number of leatherback turtles were thought to have interacted with the fishing industry, with 8% of leatherback records due to bycatch. Other reporting schemes from Italy and Portugal also found bycatch to be the substantial cause of mortality/stranding in leatherback turtles (Casale et al., Reference Casale, Nicolosi, Freggi, Turchetto and Argano2003; Nicolau et al., Reference Nicolau, Ferreira, Santos, Araújo, Sequeira, Vingada, Eira and Marçalo2016). Injuries through collision with vessels were recorded for five leatherback turtles in the UK and Ireland and entanglement in ghost gear was recorded in 16 leatherback strandings and sightings. Post-mortem examinations were only carried out on a small number of strandings (4.5% of records; N = 89); it is likely that additional causes of stranding/mortality such as disease are present, yet are not recorded in the data.
Whilst there are limitations to interpreting data from public recording schemes, if carefully analysed they can provide important information on patterns of distribution and relative abundance and can potentially provide signals of population recoveries and declines. This is especially true as the temporal extent of the data increases. Our data analysis provides an extension to previous work conducted by Witt et al. (Reference Witt, Penrose and Godley2007b) and continues to show an increase in the decadal totals of Kemp's ridley turtle strandings and sightings which possibly indicates recovery of populations elsewhere. However, this study identifies a decrease in the number of recent annual records of sightings, strandings and captures of all three species of turtles. The underlying reasons for this decrease can only be speculated upon, although it may potentially be a result of reduced reporting effort. This TURTLE database provides an important source of information regarding distribution and abundance of marine turtles in the North Atlantic that are far from their natal beaches and at elusive life stages. Our results provide a valuable reference point to assess species range modification due to climate change, identify possible evidence of anthropogenic threats and to assess the future trajectory of marine turtle populations in the North Atlantic and underpins the utility of long-term commitment to programmes such as TURTLE and UK Cetacean Strandings Investigation Programme (CSIP) and Scottish Marine Animal Stranding Scheme (SMASS).
Supplementary material
The supplementary material for this article can be found at https://doi.org/10.1017/S0025315420000843.
Acknowledgements
We are thankful to the many members of the public for the reporting of sightings, strandings and captures of marine turtles in the UK and Ireland, and to the organizations which have supported TURTLE: Natural England (formerly English Nature), National Parks & Wildlife Service, Scottish Natural Heritage, Countryside Council for Wales (now Natural Resources Wales) and Welsh Government. In particular, we acknowledge the pivotal role of UK Cetacean Strandings Investigation Programme (CSIP) and Scottish Marine Animal Stranding Scheme (SMASS), funded by UK Governments. We are grateful to Karen Eckert of WIDECAST for granting access to turtle graphics. This work was initiated as part of a Natural Environment Research Grant (NE/J012319/1) and this work was supported by UKRI grant BB/T018461/1. ZB is supported by an EnvEast NERC DTP grant (NE/L002582/1). We thank the editor and two anonymous reviewers for their constructive and insightful feedback which improved the manuscript.