Introduction
Rudist bivalves were ecologically important in marine ecosystems of the late Mesozoic where they occupied multiple benthic niches (Kauffman and Johnson, Reference Kauffman and Johnson1988; Ross and Skelton, Reference Ross, Skelton and Wright1993; Hernández, Reference Hernández2011; Steuber et al., Reference Steuber, Scott, Mitchell and Skelton2016). During the hothouse conditions of the middle to Late Cretaceous (Norris et al., Reference Norris, Bice, Magno and Wilson2002; Hay, Reference Hay2017), rudist morphology diversified to include elevator, recumbent, and encruster forms in relation to the substrate (Skelton and Gili, Reference Skelton and Gili2002). Rudists exhibited a cosmopolitan distribution in warm, shallow epeiric seas, including the Mediterranean (Masse and Maresca, Reference Masse and Maresca1997; Masse and Fenerci-Masse, Reference Masse and Fenerci-Masse2008) and Middle East in the Eastern Hemisphere (Fenerci, Reference Fenerci1999; Çaglar and Önal, Reference Çaglar and Önal2009), and Caribbean (Chubb, Reference Chubb1971; Kauffman and Sohl, Reference Kauffman and Sohl1974; Kauffman and Johnson, Reference Kauffman and Johnson1988; Johnson and Kauffman, Reference Johnson, Kauffman, Kauffman and Otto1990; Ross and Skelton, Reference Ross, Skelton and Wright1993; Mitchell, Reference Mitchell2003, Reference Mitchell2010, Reference Mitchell2020, Reference Mitchell2022), Central America (Scott, Reference Scott1996; Pons et al., Reference Pons, Vicens and Schmidt-Effing2016), Mexico (Pons et al., Reference Pons, Vicens, Oviedo, Aguilar, Garcia-Barrera and Alencaster2013, Reference Pons, Vicens and García-Barrera2019, Reference Pons, García Barrera, Oviedo and Vicens2021), and the Western Interior Seaway (WIS) (Caldwell and Evans, Reference Caldwell and Evans1963; Hook and Cobban, Reference Hook and Cobban2013; Everhart, Reference Everhart2018) in the Western Hemisphere.
During the middle to Late Cretaceous, rudists became prominent within the carbonate platforms of the Tethys Sea (Kauffman and Johnson, Reference Kauffman and Johnson1988; Jablonski, Reference Jablonski1996; Hernández, Reference Hernández2011). In addition to constructing large biostromes in tropical and subtropical waters, many rudists lived in solitary or small groups at temperate latitudes (Kauffman, Reference Kauffman and Hallam1973; Kauffman and Sohl, Reference Kauffman and Sohl1974; Brownlow, Reference Brownlow1992; Ross and Skelton, Reference Ross, Skelton and Wright1993; Rojas et al., Reference Rojas, Iturralde-Vinent and Skelton1996). Throughout the Cretaceous, various families exhibited diversification, culminating in a radiation of rudist diversity in the Campanian and Maastrichtian, followed by extinction of the entire group at or near the end of the Maastrichtian (Kauffman and Johnson, Reference Kauffman and Johnson1988; Jablonski, Reference Jablonski1996; Steuber et al., Reference Steuber, Mitchell, Buhl, Gunter and Kasper2002).
Biostratigraphic and paleobiogeographic analyses indicate rudist extinction may have occurred at varying times in different regions (Johnson and Kauffman, Reference Johnson, Kauffman, MacLeod and Keller1996; Philip, Reference Philip1998; Steuber et al., Reference Steuber, Mitchell, Buhl, Gunter and Kasper2002). For the Eastern Hemisphere, stratigraphic analyses in western Europe and the Adriatic Sea indicate rudists went extinct ca. 0.5 million years before the Cretaceous-Paleogene boundary, possibly due to sea level fall (Philip, Reference Philip1998). Carbon isotope analyses in central Europe suggest rudist extinction was contemporaneous with the Cretaceous-Paleogene Extinction (Drobne et al., Reference Drobne, O, Dolenec, Marton and Palinkas1996), and other work in the Middle East suggests rudist extinction occurred in the middle Maastrichtian (Roger et al., Reference Roger, Bourdillon, Razin, Le Callonnec, Renard, Aubry, Philip, Platel, Wyns and Bonnemaison1998). For the Western Hemisphere, macro-biostratigraphic analyses indicate rudists disappeared in the Caribbean 1.5 million years before the Cretaceous-Paleogene boundary, while microbiostratigraphic analyses suggest extinction there occurred at 2.5–3.0 million years before the boundary (Johnson and Kauffman, Reference Johnson, Kauffman, MacLeod and Keller1996). Furthermore, rudist demise was possibly driven by general cooling of tropical temperatures and loss of habitat due to sea level regression (Johnson et al., Reference Johnson, Barron, Kauffman, Arthur, Fawcett and Yasuda1996). Other findings based on strontium isotope ratios in rudist shells suggest Caribbean rudists persisted into the latest Maastrichtian, with extinction occurring as part of the Cretaceous-Paleogene Extinction (Steuber et al., Reference Steuber, Mitchell, Buhl, Gunter and Kasper2002). Overall, interpretations of extinction timings highlight the value of analyzing rudist occurrences and paleobiogeography across multiple regions in the Late Cretaceous.
It is important to describe and analyze new specimens, particularly from understudied areas, to better understand rudist paleobiogeography progressing into the group's extinction in the Maastrichtian. Undescribed rudists from the Gulf Coastal Plain (GCP) and Flor de Alba Limestone Member of the Pozas Formation in Puerto Rico (PR) in the Caribbean (Fig. 1) provide such opportunities. The GCP possesses a rich and well-studied Cretaceous marine vertebrate and invertebrate diversity (Stephenson and Monroe, Reference Stephenson and Monroe1940; Manning and Dockery, Reference Manning and Dockery1992; Dockery and Thompson, Reference Dockery and Thompson2016), as well as established biostratigraphic models of thick sequences of neritic and marginal marine sediments (Stephenson and Monroe, Reference Stephenson and Monroe1938; Pessagno, Reference Pessagno1969; Mancini et al., Reference Mancini, Puckett, Tew and Smith1995; Puckett, Reference Puckett2005; Liu, Reference Liu2007; Larina et al., Reference Larina, Garb, Landman, Dastas, Thibault, Edwards, Phillips, Rovelli, Myers and Naujokaityte2016). The stratigraphic components provide an excellent framework to interpret and relate rudist paleobiogeography of the GCP to other regions in the Western Hemisphere (Fig. 1). In comparison, the Flor de Alba Limestone Member of the Pozas Formation has fewer biostratigraphic studies. Nelson and Monroe (Reference Nelson and Monroe1966) examined non-rudist bivalves from the Revés, Botijas, and Flor de Alba limestone members throughout the Pozas Formation and estimated the age of the entire formation as Campanian or Maastrichtian. Mitchell (Reference Mitchell2022) examined species of the rudist genus Barrettia from the same members and resolved member ages to upper Santionian for the Revés Limestone Member, lower Campanian for the Botijas Limestone Member, and middle Campanian for the Flor de Alba Limestone Member. Analyzing novel rudist specimens from the Flor de Alba Limestone Member would help reinforce Mitchell's (Reference Mitchell2022) newly determined age for that member.

Figure 1. Outline of modern Gulf Coastal Plain (GCP) and northern Caribbean, including Puerto Rico (PR), with bold line indicating extent of the sea during the Campanian and Maastrichtian. GCP outline synthesized from Murray (Reference Murray1961), Wood and Walper (Reference Wood and Walper1974), and Mancini et al. (Reference Mancini, Puckett, Tew and Smith1995). Western Interior Seaway (WIS) outline synthesized from Kauffman (Reference Kauffman1977). Base country outline from QGIS (2021).
There are two primary objectives of this research. The first is a systematic treatment of novel rudists from the GCP and Puerto Rico. The second objective is to analyze rudist occurrences within the published integrated lithostratigraphic and micro- and macro-biostratigraphic frameworks for these regions.
Resolving the stratigraphic placement and paleobiogeography of GCP rudists will test sea surface-current reconstructions in the Western Hemisphere for the Late Cretaceous. Previous rudist paleobiogeographic analyses in the Western Hemisphere indicate that Campanian and Maastrichtian sea surface currents dispersed fauna from the Caribbean in the south to more northerly locales (Johnson, Reference Johnson1999). The new specimens examined in this study will reveal if Caribbean taxa are present in the GCP, and, if so, the temporal ranges of each taxon can be evaluated to assess age differences between the Caribbean and GCP. If the same species occur in younger strata in the GCP and older strata in the Caribbean Sea then surface current reconstructions (Johnson, Reference Johnson1999) will be further supported.
Overall, incorporating descriptions and occurrence data from the previously unstudied GCP region and new specimens from Puerto Rico in the Caribbean fill a substantial spatial gap in Western Hemisphere rudist literature. This work contributes additional taxonomic and biostratigraphic data to aid in correlating rudist temporal and spatial ranges prior to the group's extinction. Ultimately, augmenting the paleobiogeography of this ecologically important and diverse order of bivalves may provide a valuable resource for better understanding the fate of modern shallow-water tropical reefs during present-day oceanic warming.
Materials and methods
Over 200 Upper Cretaceous rudists from the GCP were available for study—142 specimens from the Alabama Museum of Natural History Paleontology Collections (ALMNH:Paleo:), University of Alabama, Tuscaloosa, Alabama, and 63 specimens from the Mississippi Museum of Natural Science (MMNS), Mississippi Department of Wildlife, Fisheries, and Parks, Jackson, Mississippi. Specimens are housed in Alabama and Mississippi, yet they were collected from a broad geographic area across the east and west provinces of the GCP, including Alabama, Mississippi, Arkansas, and Texas (see Supplemental Data 1 for list of specimen identification numbers; information on precise collecting localities are available on request from respective museums). Formations of the Selma Group, which cover the East GCP, yielded the majority of rudist specimens at 196, including 147 specimens from the Mooreville, 17 from the Demopolis, four from the Ripley, 27 from the Prairie Bluff, and one from the Owl Creek (Table 1, left column). Formations of the Taylor Group, which span the West GCP and are equivalent in age to the lower part of the Selma Group, yielded nine specimens, including four from the Ozan, four from the Annona, and one from the Marlbrook (Table 1, center column).
Table 1. Total number of rudist specimens from each formation examined in this study. GCP = Gulf Coastal Plain.

Many of the GCP specimens were donated by avocational collectors or not found exactly in situ, although 75% of specimens are well-resolved stratigraphically. Regarding ALMNH:Paleo: material, 64% of specimens were sourced from the Harrell Station Paleontological Site (HSPS). HSPS has a precise stratigraphic placement based on foraminifera, ostracodes, and nannoplankton biostratigraphy, as recently reviewed by Ehret and Harrell (Reference Ehret and Harrell2018). Located in central Alabama, HSPS encompasses over 140 acres of exposed chalk gullies and yielded the most rudists (94 specimens) of any single site. Regarding MMNS material, if not from in situ, specimens were stratigraphically traceable to within a meter or so of source points. In addition, localities yielding specimens contributed by hobbyists were field checked.
Puerto Rico material consisted of 50 samples from the Flor de Alba Limestone Member of the Pozas Formation (Table 1). Specimens were collected from one locality near Flor de Alba Hacienda, which is southwest of Ciales, Puerto Rico. The rock exposure consisted of a limestone layer in a cave ceiling that was nearly 1 m thick and followed along an adjacent valley. Flor de Alba figured specimens, reference fossil and rock materials, and locality data are housed in the Indiana University Paleontology Collections (IUPC), Department of Earth and Atmospheric Sciences, Indiana University, Bloomington, Indiana, USA. Specimens are assigned IUPC numbers 101110–101113 for figured specimens and 101114–101159 for reference specimens.
Specimens were photographed and described in terms of taxonomy and taphonomy. Each specimen was identified to the lowest taxonomic rank using presence of identifiable morphologic characters, including height and width of radial bands, presence or absence of a ligamental ridge, shape of shell costae, outer layer shell thickness, and shell geometry. When possible, specimens were measured to determine posterior-anterior diameter, dorsal-ventral length, costae width, and taxa-specific traits such as beading and rays in Barrettia specimens or radial band width in Radiolitidae genera (Supplemental Data 2). The preservation type of each specimen was documented as original shell material, permineralized, or preserved as a mold/cast. The relative completeness of each shell was assessed qualitatively and shell sections that were not present were also noted. The degree of shell breakage was assessed qualitatively and noted in terms such as the most ventral section of the right valve. If epibionts were preserved with the shell, the number, shell location, and size were described. If a specimen possessed more than one preserved rudist, then the total number was counted and the shell orientations were documented. Finally, the determined taxonomic designations were placed within the lithostratigraphic and biostratigraphic framework provided in the literature of the GCP and Caribbean (Fig. 2). GCP specimens were not cut or sectioned because high-quality rudist specimens from that region are rare. Furthermore, these specimens are primarily found as weathered lower valves that are not encased in matrix, and the myophores, upper valves, and teeth usually are not preserved. Thus, they do not require transverse sections to identify the shell shape, whereas many rudist specimens from the Caribbean, Central America, or Mexico are encased in dense limestone matrix and need to be cut to view shell morphology. Also, given that most GCP specimens are missing upper valves, teeth, and myophores, there is far less usefulness in cutting transverse sections and permanently damaging the specimens.

Figure 2. Summary chart compiled from previous biostratigraphic and sequence stratigraphic work from the Late Cretaceous of the Gulf Coastal Plain. (1) Chronostratigraphy based on Cohen et al. (Reference Cohen, Finney, Gibbard and Fan2018); (2, 3) lithostratigraphy for the West Gulf of Mexico Coastal Plain (West GCP) from the Arkansas Geological Survey (McFarland, Reference McFarland2004); lithostratigraphy for the East Gulf Coastal Plain (East GCP) from Mancini et al. (Reference Mancini, Puckett, Tew and Smith1995), Mancini and Puckett (Reference Mancini and Puckett2005), and Naujokaitytė et al. (Reference Naujokaitytė, Garb, Thibault, Brophy, Landman, Witts, Cochran, Larina, Phillips and Myers2021); (4) foraminifera biozonations from Caron (Reference Caron, Bolli, Saunders and Perch-Nielsen1985), Puckett (Reference Puckett1994, Reference Puckett2005), and Mancini et al. (Reference Mancini, Puckett and Tew1996); (5) ostracode biozonations from Hazel and Browers (Reference Hazel, Browers and Maddocks1982), Dowsett (Reference Dowsett1989), and Puckett (Reference Puckett1994, Reference Puckett2005); (6) calcareous nannoplankton biozonations from Sissing (Reference Sissing1977) and Perch-Nielsen (Reference Perch-Nielsen, Bolli, Saunders and Perch-Nielsen1985); (7) ammonite biozonations from Cobban and Kennedy (Reference Cobban and Kennedy1995), Kennedy et al. (Reference Kennedy, Cobban and Landman1997), Cobban et al. (Reference Cobban, Walaszczk, Obradovich and McKinney2006), and Larina et al. (Reference Larina, Garb, Landman, Dastas, Thibault, Edwards, Phillips, Rovelli, Myers and Naujokaityte2016); (8) bivalve biozonations Stephenson and Monroe (Reference Stephenson and Monroe1938) and Copeland (Reference Copeland1968); (9) transgressive-regressive cycles based on Mancini et al. (Reference Mancini, Puckett and Tew1996), Puckett and Mancini (Reference Puckett and Mancini2000), and Liu (Reference Liu2007). Fm = formation; Grp = group; Mbr = member; Lst = limestone; T-R = transgressive-regressive; sinusoidal lines = hiatus; hash marks = well-defined hiatus.
Repositories and institutional abbreviations
Figured and reference specimens examined in this study are deposited in the following institutions: Alabama Museum of Natural History (ALMNH:Paleo:), University of Alabama, Tuscaloosa, Alabama, USA; Mississippi Museum of Natural Science (MMNS), Mississippi Department of Wildlife, Fisheries, and Parks, Jackson, Mississippi, USA; and Indiana University Paleontology Collections (IUPC), Department of Earth and Atmospheric Sciences, Indiana University, Bloomington, Indiana, USA.
Systematic paleontology
Of the total 205 specimens from the GCP, 76 were identified to genus within the families of Monopleuridae! Munier-Chalmas, Reference Munier-Chalmas1873, including Gyropleura Douvillé, Reference Douvillé1887, and Radiolitidae d'Orbigny, Reference d'Orbigny1847, including Biradiolites d'Orbigny, Reference d'Orbigny1850, Durania Douvillé, Reference Douvillé1908, Guanacastea Pons et al., Reference Pons, Vicens and Schmidt-Effing2016, Radiolites Lamarck, Reference Lamarck1801, and Sauvagesia Choffat, Reference Choffat1886. Of the 50 Puerto Rico specimens from the Flor de Alba Limestone Member of the Pozas Formation, 12 were identified to the genus Barrettia Woodward, Reference Woodward1862, in the family Hippuritidae Gray, Reference Gray1848.
Taxonomy and nomenclature follow Skelton (Reference Skelton2013), including that ‘!’ designates a paraphyletic group.
Order Hippuritida Newell, Reference Newell1965 (Syn. Rudistæ Lamarck, Reference Lamarck1819 [Dechaseaux, Reference Dechaseaux and Piveteau1952])
Family Hippuritidae Gray, Reference Gray1848
Genus Barrettia Woodward, Reference Woodward1862
Type species
Barrettia monilifera Woodward, Reference Woodward1862, by monotypy, from upper middle Campanian, Back Rio Grande Limestone, Back Rio Grande, Portland, Jamaica.
Diagnosis
Hippuritid genus where right valve has multiple folds and a coarse vesicular structure in the inner layer. Left valve has multiple reticulate pores over each bead and pallial canals in the inner layer. Angle between second pillar and posterior myophore ranges from 65–147°, angle between first pillar and posterior tooth ranges from 103–196°, and angle between posterior myophore and anterior tooth ranges from 132–171° (Mitchell, Reference Mitchell2010).
Occurrence
Middle Santonian to middle Maastrichtian (Steuber et al., Reference Steuber, Scott, Mitchell and Skelton2016).
Barrettia monilifera Woodward, Reference Woodward1862
Figure 3
- Reference Woodward1862
Barrettia monilifera Woodward, p. 372, pl. 20, figs 1–4, pl. 21, fig 5.
- Reference Douvillé1894
Barrettia monilifera; Douvillé, p. 110, pl. 17, fig. 6.
- Reference Whitfield1897
Barrettia monilifera; Whitfield, pl. 27, fig. 1, pl. 28, fig. 1 (reproduction of Woodward, 1862, pl. 20, 21).
- Reference Toucas1903
Orbignya monilifera Woodward; Toucas, p. 47, text-fig. 83.
- Reference Boissevane and Mac Gillavry1932
Barrettia monilifera; Boissevane and Mac Gillavry, fig. 3c.
- Reference Chubb1971
Barrettia monilifera; Chubb, p. 208, pl. 51, figs. 1–3, pl. 52, figs. 2, 3, text-fig. 8.
- Reference van Dommelen1971
Barrettia monilifera; van Dommelen, text-figs. 6E, 7C, 9A.
- Reference Grubić2004
Barrettia monilifera; Grubić, p. 160, pl. 5, fig. 2.
- Reference Mitchell2010
Barrettia monilifera; Mitchell, p. 10, text-figs. 3D, 4, 6A–D, 8A, 9 (text-fig 4 = photograph of Woodward's [Reference Woodward1862] specimen in pl. 20, fig. 2, pl. 21, fig. 5).
- Reference Pons, Vicens and Schmidt-Effing2016
Barrettia monilifera; Pons et al., fig. 3.

Figure 3. Barrettia monilifera Woodward, Reference Woodward1862, specimens from the Flor de Alba Limestone Member of the Pozas Formation in Ciales Municipio, Puerto Rico. (1) Top view of right (lower) valve showing distinctive radial bead morphology and characteristic pillar shapes (IUPC 101111); (2, 3) top and side view of specimen (IUPC 101112); (4, 5) top view of right valves showing taphonomic variation, which partially obscures view of pillars and beading (IUPC 101110 and 101113). P1 = first pillar and P2 = second pillar.
Holotype
Barrettia monilifera Woodward, Reference Woodward1862 (Natural History Museum, London, BMNH 42861), from upper middle Campanian, Back Rio Grande Limestone, Back Rio Grande, Portland, Jamaica.
Diagnosis
Barrettia species where right valve shows a short ligamental ray, a moderate ray density, moderately large round beads, thin, irregular inter-ray partitions, and an advanced myocardinal pillar arrangement that consists of a large angle (commonly ~150°) between the first pillar, the posterior myophore, and the posterior tooth, and a smaller angle (commonly ~90°) between the second pillar, first pillar, and the posterior myophore. Note that the angles listed are the angles between two lines that connect these three points. The first line is drawn from the first point to the middle point and the second line is drawn from the middle point to the last point. Termination of first pillar is rounded, and termination of second pillar is elongated and oval. Left valve has pores with raised domes over each bead (Woodward, Reference Woodward1862; Chubb, Reference Chubb1971; Mitchell, Reference Mitchell2010).
Occurrence
Middle Campanian (Mitchell and Ramsook, Reference Mitchell and Ramsook2009; Mitchell, Reference Mitchell2010, Reference Mitchell2022; Pons et al., Reference Pons, Vicens and Schmidt-Effing2016).
Description
Right valves are cylindro-conical, 102–174 mm in posterior-anterior diameter, and at least 241–421 mm in dorsal-ventral length. Outer shell shows regularly spaced costae that are 6–8 mm in width and are separated by grooves that correspond to rays visible in cross section. Rays are moniliform, because the beads are generally elongated circles and show well-developed separations. When countable, number of rays ranges between 34–42. Diameter of beads is 1–2 mm. Separation distance between beads is irregular and varies across individual specimens, from <1–3 mm on average. Termination of first pillar is rounded and 6–10 mm in diameter. Termination of the second pillar is elongated, oval shaped, 5–8 mm in width, and 20–26 mm in length. Left valves are low cone shaped and are partially preserved in three specimens, where they are attached to right valves. Dorsal-ventral length is 23–28 mm. Pore indentations in left valve follow the same radial pattern as the beads and rays within right valves.
Specimens are preserved primarily as recrystallized single individuals in packstones. Two specimens are preserved as a pair of individuals that are aligned in the same orientation. Beads, rays, and the central body cavities are completely infilled with packstones in most specimens. Two specimens show a central body cavity partially infilled with recrystallized calcite. Most specimens lack preservation of the most ventral section of the right valve, which restricts measuring full dorsal-ventral length.
Material
Twelve specimens, Flor de Alba Member of the Pozas Formation in Puerto Rico: IU 101110–101113, 101142, 101144, 101148, 101149, 101151–101154.
Remarks
The density and angle of rays, density of beads, rounded termination of the first pillar, elongated oval termination of second pillar, and the angles support assigning the valves to specimens of Barrettia monilifera described by Mitchell (Reference Mitchell2010). Mitchell (Reference Mitchell2010) concluded that the species is found in Jamacia, and that specimens from Cuba, Puerto Rico, and St. Croix that were identified as Barrettia monilifera belong to other species of Barrettia. Subsequently, Pons et al. (Reference Pons, Vicens and Schmidt-Effing2016) identified specimens of Barrettia monilifera from Playa Panamá, Santa Rosa National Park, and Bolsón, Costa Rica. Most recently, Mitchell (Reference Mitchell2022) identified Barrettia monilifera from the Flor de Alba Limestone Member of the Pozas Formation in Puerto Rico.
Family Monopleuridae! Munier-Chalmas, Reference Munier-Chalmas1873
Genus Gyropleura Douvillé, Reference Douvillé1887
Type species
Requienia cenomanensis d'Orbigny, Reference d'Orbigny1850 by original designation.
Diagnosis
Monopleuridae! genus with invaginated ligament. Right value is gyropleuriform and contains posterior myophoral plate. Left valve is arched with the umbo overhanging the commissural plane (Dechaseaux et al., Reference Dechaseaux, Cox, Coogan, Perkins, Moore and Teichert1969).
Occurrence
Early Aptian to late Maastrichtian (Chubb, Reference Chubb1971; Steuber et al., Reference Steuber, Scott, Mitchell and Skelton2016).
Gyropleura sp. indet.
Figure 4
Description
Right valves are gyropleuriform, 8–12 mm in posterior-anterior diameter, and 4–6 mm in dorsal-ventral length. Fine, sub-mm growth lines are visible across outer shell. Commissure line is flat to slightly curved. Rounded umbo is prominent and overhangs commissural plane. Left valves are very low cone shaped, 5–11 mm in posterior-anterior diameter, and 2–4 mm in dorsal-ventral length. Left valves are preserved attached to right valves in all specimens except two, in those specimens the left valves are preserved without the right valves.

Figure 4. Gyropleura sp. indet. specimens from GCP. (1, 2) Bottom views of right (lower) valves showing borings into shell (MMNS 4163, Prairie Bluff Formation, Union Co., MS and MMNS 4875, Prairie Bluff Formation, Pontotoc Co., MS); (3) top view of left (upper) valve showing commissure with right (lower) valve (MMNS 4875); (4–7) a steinkern representing an articulated valve pair showing how both valves attach (MMNS 5427, Prairie Bluff Formation, Wilcox Co., AL); (8) transverse section cut near commissure showing myocardinal arrangement (MMNS 4365, Ripley Formation, Union Co., MS). Gyropleura sp. indet. specimens were the only taxon that showed preserved left (upper) valves in all the GCP material.
Specimens are preserved as individuals, either as steinkerns or recrystallized with calcite precipitation filling shell cavities. Sub-mm round borings are preserved in many specimens and range in number from 6–18. Shell cavities are completely infilled by marl sediment. Many specimens are preserved mostly intact, with both right and left valves still attached and nearly complete.
Material
Six specimens, GCP. Prairie Bluff Formation, four: MMNS 4163, 4658, 4875, and 5427; Ripley Formation, two: MMNS 3173 and 4365. Not preserved with adequate morphological features for species identification.
Remarks
Detailed morphology of the myocardinal arrangement, which is used for species designations, is not well preserved in these specimens, which prevents species identification.
Family Radiolitidae d'Orbigny, Reference d'Orbigny1847
Genus Biradiolites d'Orbigny, Reference d'Orbigny1850
Type species
Biradiolites canaliculatus d'Orbigny, Reference d'Orbigny1850, by subsequent designation of Toucas, Reference Toucas1909.
Diagnosis
Radiolitidae genus with no ligamental ridge. Right valve has radial bands situated in furrows, and the outer layer is composed of cellular and compact wall structures (Dechaseaux et al., Reference Dechaseaux, Cox, Coogan, Perkins, Moore and Teichert1969).
Occurrence
Early Turonian to late Maastrichtian (Steuber et al., Reference Steuber, Scott, Mitchell and Skelton2016).
Biradiolites cardenasensis Böse, Reference Böse1906
Figure 5
- Reference Böse1906
Biradiolites cardenasensis Böse, p. 59, pl. 11, fig. 3, pl. 12, fig. 3.
- Reference Böse1906
Biradiolites potosianus Böse, p. 60, pl. 5, figs. 2, 3, pl. 11, fig. 4, pl. 12, fig 5.
- Reference Trechmann1924
Bournonia barretti Trechmann, p. 405, pl. 26, figs. 2, 2a.
- Reference Myers1968
Biradiolites cardenasensis; Myers, p. 45, pl. 4, figs. 1–4.
- Reference Alencaster1971
Biradiolites cardenasensis; Alencaster, p. 43, pl. 7, figs. 5–7, pl. 19, figs. 2–4.
- Reference Chubb1971
Bournonia barretti; Chubb, p. 194, pl. 40, figs. 5.
- Reference Alencaster1990
Bournonia cardenasensis; Alencaster, p. 64, pl. 2, fig. 4.
- Reference Scott1996
Bournonia cardenasensis; Scott, p. 303, text-fig. 6E, pl. 2, figs. 5, 6.
- Reference Mitchell2003
Bournonia barretti; Mitchell, p. 152, pl. 3, figs. a–c.
- Reference Mitchell2003
Bournonia cancellata; Mitchell, p. 151, pl. 3, figs. d–f, pl. 4, figs. 4a, c, d.
- Reference Oviedo2005
Bournonia cardenasensis; Oviedo, p. 23, fig. 14.
- Reference Pons, Vicens, Oviedo, Aguilar, Garcia-Barrera and Alencaster2013
Biradiolites cardenasensis; Pons et al., p. 730, figs. 4.1–4.5, 5.1–5.10, 6.1–6.9.
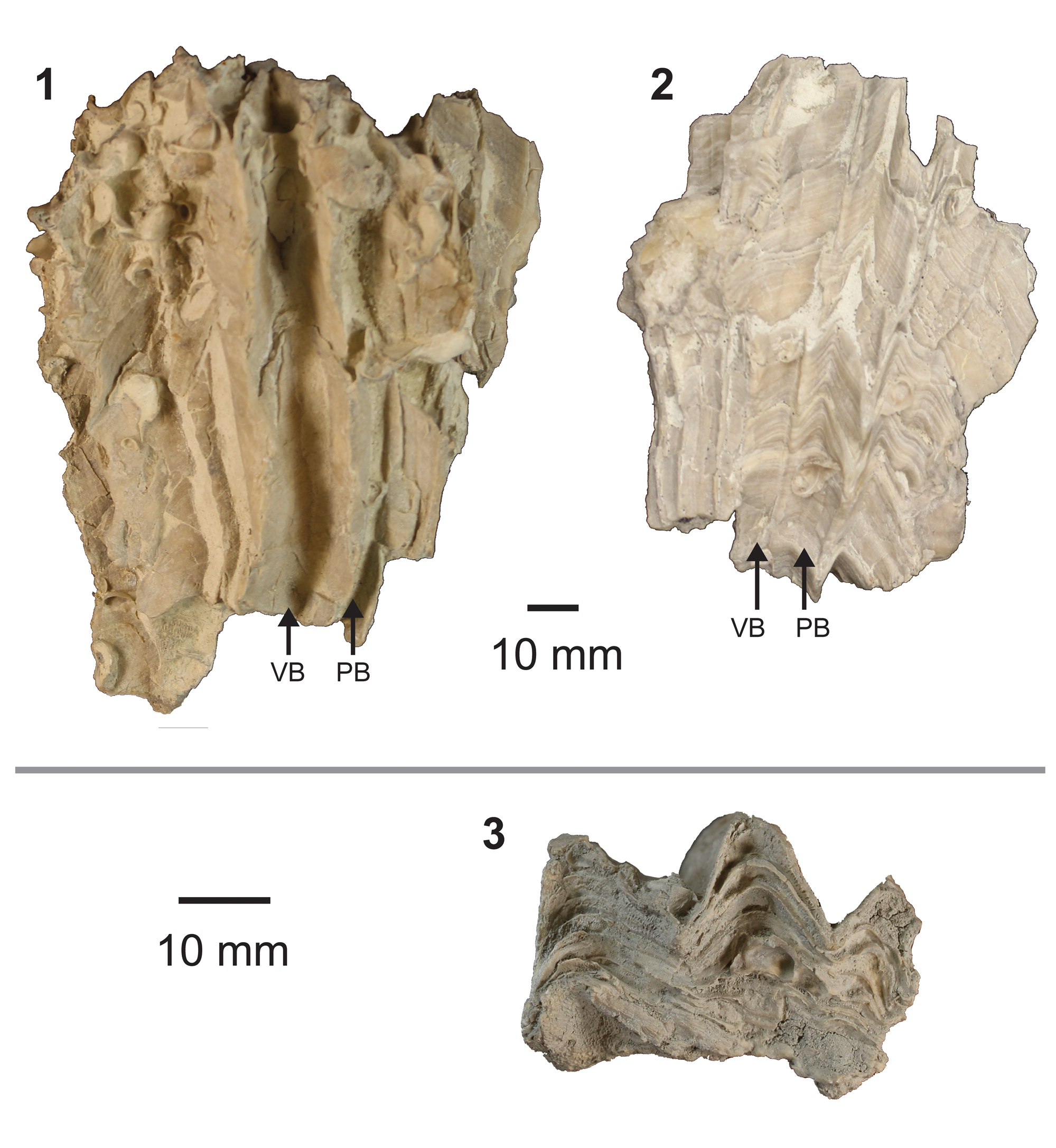
Figure 5. Biradiolites specimens from GCP. (1, 2) Side views of right (lower) valve of B. cardenasensis Böse, Reference Böse1906, showing radial bands and distinctive very pronounced costae and sinuses with flat radial bands (ALMNH:Paleo: 13212 and 13208, respectively, both Mooreville Formation, Dallas Co., AL); (3) top view of right valve fragment of Biradiolites sp. aff. B. cardenasensis associated with larger specimen showing detail of layers of shell which make up the valve (ALMNH:Paleo: 12659, Mooreville Formation, Dallas Co., AL). VB = ventral radial band; PB = posterior radial band. Gray line separates specimens at different scales.
Holotype
Biradiolites cardenasensis Böse, Reference Böse1906, by original designation, Cardenas Formation, Cardenas San Luis Potosi, Mexico.
Diagnosis
Biradiolites species where right valve has very pronounced costae and sinuses at the ventral, posterior, and dorsal side, radial groove is commonly developed on the anterior side, and radial bands appear as two flat costae with an acute costa between them (Pons et al., Reference Pons, Vicens, Oviedo, Aguilar, Garcia-Barrera and Alencaster2013).
Occurrence
Middle Campanian to late Maastrichtian (Alencaster, Reference Alencaster1971; Scott, Reference Scott1996; Pons et al., Reference Pons, Vicens, Oviedo, Aguilar, Garcia-Barrera and Alencaster2013).
Description
Right valves are cylindro-conical, 46–94 mm in posterior-anterior diameter, and at least 62–137 mm in dorsal-ventral length. Outer shell shows very pronounced angular costae that are 6–8 mm in width. Deep sinuses separate the costae and are 7–12 mm in width. Radial bands are wide and flat, 14–20 mm in width, and are separated by an inter-band that is 3–8 mm in width and contains one small costa. The thickness of the right valve remains relatively consistent across the shell.
Specimens are preserved as recrystallized individuals that exhibit moderate calcite precipitation in the shell's outer layer. Evidence of small, 3–5 mm, encrusters preserved on the exterior of four shells, although the fragmentary preservation prevents taxonomic identification. All specimens lack preservation of the most ventral section of the right valve, which restricts measuring full dorsal-ventral length. No left valves are preserved.
Material
Three specimens, Mooreville Formation, GCP. Two Biradiolites sp. aff. B. cardenasensis: ALMNH:Paleo: 12659 and 13208; one B. cardenasensis: ALMNH:Paleo: 13212.
Remarks
The species assignment is supported by the very pronounced costae and sinuses and the presence of two radial bands with flat costae separated by an acute costa. The species has been assigned to Biradiolites or Bournonia. Pons et al. (Reference Pons, Vicens, Oviedo, Aguilar, Garcia-Barrera and Alencaster2013) noted the pattern of radial structure and rib in the outer surface between the radial bands as characteristic of Biradiolites and not Bournonia. This paper follows that discussion and assigns these specimens as Biradiolites. Pons et al. (Reference Pons, Vicens, Oviedo, Aguilar, Garcia-Barrera and Alencaster2013) also recognized that specimens attributed to Bournonia cancellata (Whitfield, Reference Whitfield1897) by Chubb (Reference Chubb1971) and Mitchell (Reference Mitchell2003) show an acute costa between the two radial bands while the original specimens designated as Radiolites cancellata (Whitfield, Reference Whitfield1897) do not show this key-species trait. Pons et al. (Reference Pons, Vicens, Oviedo, Aguilar, Garcia-Barrera and Alencaster2013) concluded that the original specimens of Whitfield's Radiolites cancellata should not be attributed to Biradiolites cardenasensis Böse, Reference Böse1906.
Genus Durania Douvillé, Reference Douvillé1908
Type species
Hippurites cornupastoris Des Moulins, Reference Des Moulins1826, designated by Douvillé, Reference Douvillé1908.
Diagnosis
Radiolitidae genus with no ligamental ridge. Right valve is cylindrical and can be short or elongate, radial bands can be concave, smooth, or ribbed, and bifurcating radial furrows are present on the upper surface of the outer wall of right valve in many species (Dechaseaux et al., Reference Dechaseaux, Cox, Coogan, Perkins, Moore and Teichert1969).
Occurrence
Early Albian to middle Maastrichtian (Chubb, Reference Chubb1971; Steuber et al., Reference Steuber, Scott, Mitchell and Skelton2016).
Durania maxima (Logan, Reference Logan1898)
Figure 6.1, 6.2, 6.4–6.7
- Reference Logan1898
Radiolites maximus Logan, p. 494, pls. 115, 119, fig. 1.
- Reference Miller1968
Durania maxima; Miller, p. 37, pl. 4, figs. 6–8.
- Reference Hattin1982
Durania maxima; Hattin, 1982, p. 40, pl. 7, figs. 2–5.
- Reference Hattin1986
Durania maxima; Hattin, 1986, p. 361, text-figs. 35, 36 (text-fig. 36 = photograph of specimen from Hattin, 1982, pl. 7, fig. 5).
- Reference Everhart2018
Durania maxima; Everhart, 2018, p. 113, text-figs. 4–7.

Figure 6. Durania specimens from GCP. (1, 2) Top and side view of right (lower) valve of D. maxima (Logan, Reference Logan1898) showing encruster growth on the exterior and interior of the shell (MMNS 4542, Ozan Formation, Sevier Co., AR); (3) top view of right valve fragment of Durania sp. aff. D. maxima showing distinctive bifurcating radial furrows; many specimens are preserved as fragments similar to this (ALMNH:Paleo: 12629, Demopolis Formation, Perry Co., AL); (4) top view of four right valves of D. maxima preserved together (MMNS 42, Mooreville Formation, Lowndes Co., MS); (5) top view of D. maxima right valve, showing encrusters growing on the exterior and interior of the shell (MMNS 44, Mooreville Formation, Lowndes Co., MS); (6, 7) top and side views of right valve of D. maxima; note smaller additional D. maxima specimens are preserved and growing next to larger specimen (ALMNH:Paleo: 13458, Mooreville Formation, Dallas Co., AL). VB = ventral radial band; PB = posterior radial band. Gray line separates specimens at different scales.
Holotype
Radiolites maximus, Logan, Reference Logan1898, designated by Miller, Reference Miller1968.
Diagnosis
Durania species where right valve has outer shell surface that is regularly ribbed, commonly very large in size, with radial bands that are wide, finely ribbed, concave, and separated by a regularly ribbed inter-band. Bifurcating radial furrows are present on the upper surface of the outer wall of right valve (Miller, Reference Miller1968; Hattin, Reference Hattin1982).
Occurrence
Late Coniacian to early Campanian (Hattin, Reference Hattin1982; Everhart, Reference Everhart2018).
Description
Right valves are conical to cylindro-conical, 42–346 mm in posterior-anterior diameter, and at least 34–267 mm in dorsal-ventral length. Outer shell shows rounded costae that have a width of 1–2 mm and are evenly spaced across the shell. Radial bands are concave, 8–56 mm in width, and finely ribbed. The separating inter-band is 28–48 mm in width and contains costa similar to the rest of the shell exterior. Thickness of the right valve decreases toward the radial bands and reaches a minimum thickness where the radial bands occur (e.g., ranging from 98 mm in width at the thickest section to 18 mm in width at the thinnest section). The outer wall of right valves shows bifurcating radial furrows that are ~1 mm in width.
Specimens are preserved primarily as recrystallized individuals with calcite precipitation in the cellular structure of the shell's outer layer. There are only two specimens that have more than one individual—one specimen preserved with four individuals, and one specimen with two individuals. In each case, shells are aligned in an overall similar orientation. Another specimen preserved a pair of individuals. Central body cavities range from completely infilled with marl sediment to no infilling. All specimens lack preservation of the most ventral section of the right valve, which restricts measuring full dorsal-ventral length. No left valves are preserved. Evidence of many small, 4–6 mm, encrusters preserved on the exterior and interior of three shells, although the fragmentary preservation prevents taxonomic identification.
Material
Fourteen specimens, GCP. Six Durania sp. aff. D. maxima, Demopolis Formation, two: ALMNH:Paleo: 12626 and 12629; Mooreville Formation, four: MMNS 14, 4401, 654, and 6783. Eight Durania maxima, Ozan Formation, one: MMNS 4542; Demopolis Formation, one: MMNS 6958; Mooreville Formation, six: ALMNH:Paleo: 12696, 13458, MMNS 288, 42, 43, and 44.
Remarks
Pons et al. (Reference Pons, Vicens, Oviedo, Aguilar, Garcia-Barrera and Alencaster2013) proposed a new genus, Huasteca, with type specimen Durania ojanchalensis Myers, Reference Myers1968, by monotypy. Pons et al. (Reference Pons, Vicens, Oviedo, Aguilar, Garcia-Barrera and Alencaster2013) summarized that both Durania and Huasteca are Radiolitidae with ribbed thick outer shells, cellular structure, and no ligamental ridge. The primary difference between Huasteca and Durania is the radial structures. In Huasteca, Pons et al. (Reference Pons, Vicens, Oviedo, Aguilar, Garcia-Barrera and Alencaster2013) stated that the radial structures consist of wide up-and-inward radial folds that result in smooth radial bands at the outer shell. In Durania, Pons et al. (Reference Pons, Vicens, Oviedo, Aguilar, Garcia-Barrera and Alencaster2013) summarized that radial structures at the outer shell consist of finely ribbed radial bands. The species assignment of these GCP specimens to Durania maxima, instead of Huasteca ojanchalensis, is supported by the regularly ribbed, very large right valve that exhibits wide, finely ribbed radial bands that are concave and separated by a regularly ribbed inter-band.
Durania sp. indet.
Figure 6.3
Description
Right valves are conical to cylindro-conical, estimated 45–263 mm in posterior-anterior diameter, and at least 34–146 mm in dorsal-ventral length. Outer shell shows rounded costae that are evenly spaced and have a width of <1–2 mm. In many specimens, the outer wall of the right valve shows bifurcating radial furrows that are ~1 mm in width.
Specimens are preserved as recrystallized right valve fragments with calcite precipitation in the cellular structure of the shell's outer layer. All specimens lack preservation of the most ventral section of the right valve, which restricts measuring full dorsal-ventral length. No left valves are preserved. Evidence of multiple small, 3–5 mm, encrusters preserved on the exterior and interior of many shells, although the fragmentary preservation prevents taxonomic identification.
Material
Thirty-five specimens, GCP. Annona Formation, one: MMNS 1935; Ozan Formation, two: MMNS 4540 and 8902; Prairie Bluff Formation, three: MMNS 1120, 41, and 8147; Demopolis Formation, two: ALMNH:Paleo: 12631 and MMNS 6964; Mooreville Formation, 27: ALMNH:Paleo: 12623, 12642, 12652, 12654, 12656–12658, 12668, 12669, 12675, 12681, 12682, 12687, 12698, 12809, 12889, 12890, 12891, 12937, 12940, 12944, 13207, 13210, 13524, MMNS 5327, 62, and 876. Not preserved with adequate morphological features intact for species identification.
Remarks
Morphology of both radial bands, which is used for species designations, is not well preserved in these specimens and prevents species identification.
Genus Guanacastea Pons et al., Reference Pons, Vicens and Schmidt-Effing2016
Type species
Biradiolites jamaicensis Trenchmen, Reference Trechmann1924, by subsequent designation of Pons et al., Reference Pons, Vicens and Schmidt-Effing2016.
Diagnosis
Radiolitidae genus with no ligamental ridge. Right value is conical and elongated and possesses well-developed acute or swollen costae limited by deep furrows, with radial bands appearing as two wide costae separated by only a deep furrow. The posterior radial band is usually slightly longer while the ventral radial band is commonly shorter and wider. The outer shell layer shows a cellular structure and compact outer margin. The left valve has a concave margin, a generally convex center, and fits with the folds of the right valve (Pons et al., Reference Pons, Vicens and Schmidt-Effing2016).
Occurrence
Late Campanian to late Maastrichtian (Pons et al., Reference Pons, Vicens and Schmidt-Effing2016).
Guanacastea jamaicensis (Trechmann, Reference Trechmann1924)
Figure 7
- Reference Trechmann1924
Biradiolites jamaicensis Trechmann, p. 404, pl. 24, figs. 5, 5a, 6, 6a, 7.
- Reference Chubb1971
Biradiolites robinsoni Chubb, p. 187, pl. 36, figs. 1–3.
- Reference Mitchell2003
Biradiolites jamaicensis; Mitchell, p. 164, pls. 5a, d, e, 6a, b, e.
- Reference Pons, Vicens and Schmidt-Effing2016
Guanacastea jamaicensis; Pons et al., p. 232, fig. 15.

Figure 7. Guanacastea jamaicensis (Trechmann, Reference Trechmann1924) specimens from GCP. (1, 2) Top views of clusters; clustering is characteristic of the species (ALMNH:Paleo: 12685, Demopolis Formation, Perry Co., AL and MMNS 8791, Marlbrook Formation, Clark Co., AR); (3, 4) side views showing cylindrical conical shape of the right valves (MMNS 7394, Demopolis Formation, Oktibbeha Co., MS). VB = ventral radial band; PB = posterior radial band.
Holotype
Biradiolites jamaicensis Trechmann, Reference Trechmann1924, designated by Pons et al., Reference Pons, Vicens and Schmidt-Effing2016.
Diagnosis
Guanacastea species with costae that are acute, long, and smooth, and that show a minor development of compact structure in the outer layer of the shell (Pons et al., Reference Pons, Vicens and Schmidt-Effing2016).
Occurrence
Late Campanian to late Maastrichtian (Scott, Reference Scott1996; Mitchell, Reference Mitchell2003; Pons et al., Reference Pons, Vicens and Schmidt-Effing2016).
Description
Right valves are cylindrical, 4–14 mm in posterior-anterior diameter, and at least 28–46 mm in dorsal-ventral length. Outer shell shows angular costae that are sub-mm in width and evenly spaced across the shell. Radial bands are flat, sub-mm in width, and separated by an inter-band that is <1–1 mm in width. The thickness of the right valve remains relatively consistent across the shell.
Specimens are preserved as recrystallized aggregates ranging in number from 3–40 individuals. In all aggregates, specimens are aligned in an overall similar orientation. Within larger aggregates, the right valves of many specimens are slightly twisted, and sizes of individuals vary from <1–14 mm. Central body cavities are completely infilled with marl sediment, and specimen aggregates are preserved in marl. All specimens lack preservation of the most ventral section of the right valve, which restricts measuring full dorsal-ventral length. No left valves are preserved.
Material
Four specimens, GCP. Marlbrook Formation, one: MMNS 8791; Demopolis Formation, two: ALMNH:Paleo: 12685 and MMNS 7394; Mooreville Formation, one: MMNS 6829.
Remarks
The species assignment is supported by the acute, long, and smooth costae, along with the thin compact structure in the margin of the outer shell layer. Pons et al. (Reference Pons, Vicens and Schmidt-Effing2016) proposed the new genus Guanacastea with type species Biradiolites jamaicensis Trechmann, Reference Trechmann1924, from the Titanosarcolites limestones of Jamaica. The primary difference between Guanacastea and Biradiolites is the radial band structure. In Biradiolites, Pons et al. (Reference Pons, Vicens and Schmidt-Effing2016) noted that radial structures consist of two flat bands separated by an inter-band that contains one or more prominent costae. For Guanacastea, Pons et al. (Reference Pons, Vicens and Schmidt-Effing2016) stated the radial structures consist of two folds of the growth lamellae in the ventral and posterior position that are different from all others in the shell, the bands disturb the main concave curvature of the inner margin of the shell, and there are no costae in the inter-band. This paper follows that discussion and assigns these specimens to the updated designation of Guanacastea jamaicensis.
Genus Radiolites Lamarck, Reference Lamarck1801
Type species
Ostracites angeiodes Lapeirouse, Reference Lapeirouse1781, designated by Lamarck, Reference Lamarck1801.
Diagnosis
Radiolitidae genus possessing ligamental ridge. Right valve has outer layer composed of cellular wall structures, costae are commonly prominent, and radial bands are present, commonly smooth, and separated by an inter-band that has costae similar to those of the rest of the shell surface. Left valve is low conical with undulated margin, right valve is conical to conical-cylindrical (Dechaseaux et al., Reference Dechaseaux, Cox, Coogan, Perkins, Moore and Teichert1969).
Occurrence
Early Aptian to middle Maastrichtian (Chubb, Reference Chubb1971; Steuber et al., Reference Steuber, Scott, Mitchell and Skelton2016).
Radiolites acutocostata (Adkins, Reference Adkins1930)
Figure 8.1, 8.2
- Reference Adkins1930
Sauvagesia acutocostata Adkins, p. 99, pl. 7, figs. 3, 4.
- Reference Oviedo2005
Radiolites acutocostata; Oviedo, p. 42, text-figs. 31.1–31.9, 32.
- Reference Oviedo, Pons, Pichardo-Barrón, Aguilar and Alencaster2007
Radiolites acutocostata; Oviedo et al., p. 310.
- Reference Pichardo Barrón2008
Radiolites acutocostata; Pichardo Barrón, p. 95, text-fig. 34A–D.
- Reference Pons, Vicens, Pichardo, Aguilar, Oviedo, Alencaster and Garcia-Barrera2010
Radiolites acutocostata; Pons et al., p. 983, text-figs. 8.1–8.3, 9.1–9.3.

Figure 8. Radiolites specimens from GCP. (1, 2) Top and side views of right (lower) valve of R. acutocostata (Adkins, Reference Adkins1930); the prominent and distinctive ligamental ridge (LR) is highlighted with the white arrow, also note encrusters growing on the exterior of the shell (MMNS 6896.1, Demopolis Formation, Kemper Co., MS); (3, 4) top and side views of right valve fragment of Radiolites sp. aff. R. acutocostata showing the ligamental ridge; many specimens were preserved as fragments similar to this one (ALMNH:Paleo: 13235, Mooreville Formation, Dallas Co., AL); (5–8) top and side views of right valve of Radiolites sp. indet. specimens showing the positioning of the ligamental ridge and the ventral and posterior radial bands, (5, 6) ALMNH:Paleo: 13456, (7, 8) ALMNH:Paleo: 12997, both Mooreville Formation, Dallas Co., AL). LR = ligamental ridge; VB = ventral radial band; PB = posterior radial band; RB = radial band. Gray line separates specimens at different scales.
Holotype
Sauvagesia acutocostata Adkins, Reference Adkins1930, designated by Oviedo, Reference Oviedo2005.
Diagnosis
Radiolites species where right valve is conical, ventral radial band is smooth and typically larger than posterior radial band that can have one to two fine costae, ligamental ridge is prominent and rounded at edge (Oviedo, Reference Oviedo2005; Pons et al., Reference Pons, Vicens, Pichardo, Aguilar, Oviedo, Alencaster and Garcia-Barrera2010).
Occurrence
Early to middle Campanian (Scott, Reference Scott2005; Pons et al., Reference Pons, Vicens, Pichardo, Aguilar, Oviedo, Alencaster and Garcia-Barrera2010).
Description
Right valves are cylindrical to cylindro-conical, 61–110 mm in posterior-anterior diameter, and at least 116–168 mm in dorsal-ventral length. Outer shell shows angular costae that have a width of ~1 mm and are evenly spaced across the shell. Radial bands shape and size vary between ventral and posterior bands. Ventral bands are flat, smooth, and 8–13 mm in width. Posterior bands are slightly concave, have one very fine costa, and 4–5 mm in width. The separating inter-band is 8–9 mm in width and contains costa similar to the rest of the shell exterior. The thickness of the right valve slightly decreases near the radial bands, ranging from 15 mm in width at the thickest section to 6 mm in width at the thinnest section. Ligamental ridge is elongated and narrow, and where fully preserved it is rounded at edge and reaches length of 5 mm.
Specimens are preserved as recrystallized individuals with calcite precipitation in the cellular structure of the shell's outer layer. Central body cavities range from completely infilled with marl sediment to no infilling. All specimens lack preservation of the most ventral section of the right valve, which restricts measuring full dorsal-ventral length. Ligamental ridge is fully preserved in only one specimen. No left valves are preserved. Evidence of many small, 3–5 mm, encrusters preserved on the exterior and interior of two shells, although the fragmentary preservation prevents taxonomic identification.
Material
Four specimens, GCP. Three Radiolites sp. aff. R. acutocostata, Demopolis Formation, one: ALMNH:Paleo: 12888; Mooreville Formation, two: ALMNH:Paleo: 13235 and MMNS 638. One R. acutocostata, Demopolis Formation: MMNS 6896.1.
Remarks
The species assignment is supported by the prominent ligamental ridge rounded at the end along with the conical right valve and ventral radial band that is more smooth and larger than posterior radial band.
Radiolites sp. indet.
Figure 8.3–8.8
Description
Right valves are conical to cylindro-conical, 16–203 mm in posterior-anterior diameter, and at least 28–386 mm in dorsal-ventral length. Outer shell shows angular costae that are 3–8 mm in width and are evenly spaced across the shell. Radial bands flat to slightly concave, smooth to finely ribbed, and 7–22 mm in width. The separating inter-band is 12–37 mm in width and contains costa similar to the rest of the shell exterior. The thickness of the right valve remains relatively consistent across the shell. Ligamental ridge is angular and narrow, reaching lengths of at least 3–6 mm.
Specimens are preserved as recrystallized individuals with calcite precipitation in the cellular structure of the shell's outer layer. Central body cavities range from completely infilled with marl sediment to no infilling. All specimens except one lack preservation of the most ventral section of the right valve, which restricts measuring full dorsal-ventral length. No left valves are preserved. Evidence of many small, 3–5 mm, encrusters preserved on the exterior and interior of two shells and evidence of one large, 11 mm encruster preserved on the upper surface of the outer wall of one additional specimen. The fragmentary preservation of the encrusters prevents taxonomic identification.
Material
Ten specimens, GCP. Prairie Bluff Formation, three: MMNS 1154, 337, and 40; Ripley Formation, one: MMNS 3237; Mooreville Formation, six: ALMNH:Paleo: 12679, 12997, 13000, 13234, 13456, and 13471. Not preserved with adequate morphological features intact for species identification.
Remarks
Morphology of myocardinal arrangement and detailed radial bands, which are used for species designations, are not well preserved in these specimens and prevent species identification.
Genus Sauvagesia Choffat, Reference Choffat1886
Type species
Sphaerulites sharpei Bayle, Reference Bayle1857, designated by Choffat, Reference Choffat1886.
Diagnosis
Radiolitidae genus possessing ligamental ridge. Right valve is conical to cylindrical and commonly elongate, radial bands are concave and smooth or finely ribbed, and costae are evenly spaced and rounded (Dechaseaux et al., Reference Dechaseaux, Cox, Coogan, Perkins, Moore and Teichert1969).
Occurrence
Early Cenomanian to late Maastrichtian (Chubb, Reference Chubb1971; Steuber et al., Reference Steuber, Scott, Mitchell and Skelton2016).
Sauvagesia sp. indet.
Figure 9
Description
Right valves are cylindrical to cylindro-conical, 18–103 mm in posterior-anterior diameter, and at least 48–160 mm in dorsal-ventral length. Outer shell shows angular to sub-angular costae that are 2–7 mm in width and are evenly spaced across the shell. Radial bands concave, finely ribbed, and 4–12 mm in width. The separating inter-band is 10–16 mm in width and contains costa similar to the rest of the shell exterior. Thickness of the right valve is largest near the ligamental ridge (e.g., in one specimen ranging from 24 mm in width at the thickest section to 10 mm in width at the thinnest section). Ligamental ridge is angular and narrow, reaching lengths of at least 3–6 mm.

Figure 9. Sauvagesia sp. indet. specimens from GCP. (1, 2) Top and side views of right (lower) valve showing placement of ligamental ridge in relation to the ventral and posterior radial bands (ALMNH:Paleo: 13211, Mooreville Formation, Dallas Co., AL); (3) side view of less complete right valve (ALMNH:Paleo: 12960, Mooreville Formation, Dallas Co., AL); (4) side view of right valves of two individuals; note one indeterminate radial band is visible (MMNS 6130, Prairie Bluff Formation, Sumter Co., AL); (5) side view of right valve showing substantial taphonomic effects and infilling of the valve with sediment (MMNS 2710, Prairie Bluff Formation, Oktibbeha Co., MS). LR = ligamental ridge; VB = ventral radial band; PB = posterior radial band; RB = radial band.
Specimens are preserved primarily as recrystallized individuals with calcite precipitation in the cellular structure of the shell's outer layer. One specimen is preserved as a pair of individuals that are aligned in the same orientation. Central body cavities range from completely infilled with marl sediment to no infilling. All specimens lack preservation of the most ventral section of the right valve, which restricts measuring full dorsal-ventral length. No left valves are preserved.
Material
Ten specimens, GCP. Prairie Bluff Formation, four: MMNS 1103, 1346, 2710, and 6130; Mooreville Formation, six: ALMNH:Paleo: 12693, 12948, 12960, 12999, 13211, and MMNS 6962. Not preserved with adequate morphological features intact for species identification.
Remarks
Morphology of left valve and myocardinal arrangement, which are used for species designations, are not well preserved in these specimens and prevent species identification.
Discussion
This study provides the first investigation of Campanian and Maastrichtian rudists from the GCP and novel specimens from Puerto Rico. Identified rudists from the GCP comprise Monopleuridae Munier-Chalmas, Reference Munier-Chalmas1873, including Gyropleura Douvillé, Reference Douvillé1887, as well as Radiolitidae d'Orbigny, Reference d'Orbigny1847, including Biradiolites cardenasensis Böse, Reference Böse1906, Durania Douvillé, Reference Douvillé1908, Durania maxima (Logan, Reference Logan1898), Guanacastea jamaicensis (Trechmann, Reference Trechmann1924), Radiolites Lamarck, Reference Lamarck1801, Radiolites acutocostata (Adkins, Reference Adkins1930), and Sauvagesia Choffat, Reference Choffat1886. Identified rudists from Puerto Rico comprise Hippuritidae Gray, Reference Gray1848, and include Barrettia monilifera Woodward, Reference Woodward1862. Rudists are preserved dominantly as partial lower (attached) valves; the only upper valves are four Gyropleura specimens from the GCP and three Barrettia monilifera specimens from Puerto Rico. Taxa preserved as aggregates include Guanacastea jamaicensis, ranging from 3–40 individuals, and Durania maxima, commonly with 2–4 individuals. Ultimately, these new data provide the basis for integrating rudist occurrences into the micro- and macro-biostratigraphy of the GCP and rudist biostratigraphy of the Caribbean.
Placing the temporal occurrences of Puerto Rico rudist species within documented biostratigraphic ranges reinforces the age of the Flor de Alba Limestone Member of the Pozas Formation to the middle Campanian. Previous biostratigraphic interpretations of non-rudist bivalves indicated the age of the member as Campanian or Maastrichtian (Nelson and Monroe, Reference Nelson and Monroe1966), while more recent rudist work based on Barrettia monilifera narrowed the age to the middle Campanian (Mitchell, Reference Mitchell2022). Results herein document twelve Barrettia monilifera specimens from the Flor de Alba Limestone Member of the Pozas Formation, which supports the middle Campanian age assigned by Mitchell (Reference Mitchell2022).
Comparing occurrences of GCP rudist species to literature ranges extends the stratigraphic ranges of four species (Fig. 10). The upper stratigraphic range of Durania maxima was previously noted as early Campanian (Hattin, Reference Hattin1982; Everhart, Reference Everhart2018), although the presence of D. maxima from the Ozan Formation extends that range into the middle Campanian in the East GCP. In addition, the presence of D. maxima from the Mooreville and Demopolis formations expands the range into the late Campanian in the West GCP. The upper stratigraphic range of Radiolites acutocostata was previously the middle Campanian (Scott, Reference Scott2005; Pons et al., Reference Pons, Vicens, Pichardo, Aguilar, Oviedo, Alencaster and Garcia-Barrera2010), and the presence of R. acutocostata from the Mooreville and Demopolis formations expands the range into the late Campanian in the West GCP. The lower stratigraphic range of Biradiolites cardenasensis was previously the middle Campanian (Scott, Reference Scott2005; Pons et al., Reference Pons, Vicens, Pichardo, Aguilar, Oviedo, Alencaster and Garcia-Barrera2010), and the presence of B. cardenasensis from the Mooreville Formation expands the range into the early Campanian in the West GCP. The lower stratigraphic range of Guanacastea jamaicensis was previously the late Campanian (Scott, Reference Scott1996; Mitchell, Reference Mitchell2003; Pons et al., Reference Pons, Vicens and Schmidt-Effing2016), and the presence of G. jamaicensis from the Marlbrook Formation aligns with that range in the East GCP. However, the presence of G. jamaicensis from the Mooreville and Demopolis formations expands the range into the early Campanian in the West GCP.
Comparing occurrences of GCP rudist genera to ranges reported in the literature aligns with the stratigraphic ranges of two genera and slightly extends two others (Fig. 10). The upper stratigraphic range of Durania and Radiolites was previously the middle Maastrichtian (Chubb, Reference Chubb1971; Steuber et al., Reference Steuber, Scott, Mitchell and Skelton2016), although the presence of Durania and Radiolites from the Prairie Bluff Formation extends their range into the late Maastrichtian in the East GCP. The upper stratigraphic range of Gyropleura and Sauvagesia was previously the late Maastrichtian (Chubb, Reference Chubb1971; Steuber et al., Reference Steuber, Scott, Mitchell and Skelton2016), and the presence of both genera from the Prairie Bluff Formation aligns with that range.
The temporal placements of GCP rudists allow for correlation to the south, into the Caribbean and Central America (Fig. 11). The two most biostratigraphically useful species for southward correlation are Biradiolites cardenasensis and Guanacastea jamaicensis. Biradiolites cardenasensis is preserved in the GCP from the lower Campanian, but it is also preserved in Guatemala in the middle Campanian in the Campur/Angostura Formation (Scott, Reference Scott1996), the upper Maastrichtian in the Cardenas Formation in central Mexico (Pons et al., Reference Pons, Vicens, Oviedo, Aguilar, Garcia-Barrera and Alencaster2013), and in the upper Maastrichtian in the Guinea Corn Formation in Jamaica (Chubb, Reference Chubb1971; Mitchell, Reference Mitchell2003). Similarly, G. jamaicensis is preserved in the GCP from the lower to upper Campanian, but then only preserved in the upper Campanian in the Campur/Angostura Formation in Guatemala (Scott, Reference Scott1996), and the upper Maastrichtian in the Guinea Corn Formation in Jamaica (Mitchell, Reference Mitchell2003).

Figure 10. Biostratigraphic placement of rudists documented in this study and in the literature. Names of taxa are on the top row, with numbers in parentheses indicating the number of occurrences in the West GCP and the East GCP, respectively. The gray bar represents literature ranges in time units for each taxon listed. This range is based on data collected from Caribbean and WIS and is not based on the stratigraphic units shown here. The vertical black lines indicate occurrences from this study and are placed based on the unit in which rudist taxon was found. If a specimen was found in a stratigraphic unit without precise stratigraphic information, then the black line was drawn throughout the entire stratigraphic unit. For each taxon, the left black line represents occurrences from West GCP units, and the right black line represents occurrences from East GCP units. Dotted lines represent inferred occurrences. (1–3) Chronostratigraphy and lithostratigraphy of GCP; see Figure 2 for references; (4–12) previously documented ranges of taxa: (4) Durania maxima (Hattin, Reference Hattin1982; Everhart, Reference Everhart2018); (5) Radiolites acutocostata (Scott, Reference Scott2005; Pons et al., Reference Pons, Vicens, Pichardo, Aguilar, Oviedo, Alencaster and Garcia-Barrera2010); (6) Biradiolites cardenasensis (Alencaster, Reference Alencaster1971; Scott, Reference Scott1996; Pons et al., Reference Pons, Vicens, Oviedo, Aguilar, Garcia-Barrera and Alencaster2013); (7) Guanacastea jamaicensis (Scott, Reference Scott1996; Mitchell, Reference Mitchell2003; Pons et al., Reference Pons, Vicens and Schmidt-Effing2016); (8) Durania sp. (Dane, Reference Dane1929; Stephenson and Monroe, Reference Stephenson and Monroe1938; Stephenson, Reference Stephenson1941; Chubb, Reference Chubb1971; Steuber et al., Reference Steuber, Scott, Mitchell and Skelton2016); (9) Radiolites sp. (Chubb, Reference Chubb1971; Steuber et al., Reference Steuber, Scott, Mitchell and Skelton2016); (10) Sauvagesia sp. (Dane, Reference Dane1929; Stephenson and Monroe, Reference Stephenson and Monroe1938; Stephenson, Reference Stephenson1941; Chubb, Reference Chubb1971; Steuber et al., Reference Steuber, Scott, Mitchell and Skelton2016); (11) Gyropleura (Chubb, Reference Chubb1971; Steuber et al., Reference Steuber, Scott, Mitchell and Skelton2016).
Temporally placing GCP rudists also allows for correlation into the Western Interior Seaway (WIS) (Fig. 12). The substantial work on nannoplankton and ammonite biostratigraphy in both the GCP and WIS provides an excellent correlative framework (Sissing, Reference Sissing1977; Perch-Nielsen, Reference Perch-Nielsen, Bolli, Saunders and Perch-Nielsen1985; Cobban and Kennedy, Reference Cobban and Kennedy1995; Cobban et al., Reference Cobban, Walaszczk, Obradovich and McKinney2006; Larina et al., Reference Larina, Garb, Landman, Dastas, Thibault, Edwards, Phillips, Rovelli, Myers and Naujokaityte2016; Kita et al., Reference Kita, Watkins and Sageman2017). The Niobrara Formation can be correlated precisely via nannoplankton Zones CC16–CC19 (Sissing, Reference Sissing1977; Perch-Nielsen, Reference Perch-Nielsen, Bolli, Saunders and Perch-Nielsen1985; Kita et al., Reference Kita, Watkins and Sageman2017), and the upper section of the Hell Creek Formation can be correlated using two ammonite zones, which also contain the species Discoscaphites conradi (Morton, Reference Morton1834) found in the GCP (Cobban, Reference Cobban1993; Larina et al., Reference Larina, Garb, Landman, Dastas, Thibault, Edwards, Phillips, Rovelli, Myers and Naujokaityte2016). One species that is common between the WIS and GCP is Durania maxima. In the WIS, D. maxima is preserved from the lower Santonian to the lower Maastrichtian. In the GCP, D. maxima is preserved from the lower to upper Campanian, which is much younger than the range recorded in the WIS rocks of the Niobrara Formation.
The combined taxonomic and biostratigraphic analyses of the GCP and novel Puerto Rico rudists herein provide unique opportunities to augment rudist research in the Western Hemisphere, which is best studied from the Caribbean (Chubb, Reference Chubb1971; Kauffman and Johnson, Reference Kauffman and Johnson1988; Johnson and Kauffman, Reference Johnson, Kauffman, Kauffman and Otto1990; Ross and Skelton, Reference Ross, Skelton and Wright1993; Mitchell, Reference Mitchell2003, Reference Mitchell2010, Reference Mitchell2020, Reference Mitchell2022), Central America (Scott, Reference Scott1996; Pons et al., Reference Pons, Vicens and Schmidt-Effing2016), Mexico (Pons et al., Reference Pons, Vicens, Oviedo, Aguilar, Garcia-Barrera and Alencaster2013, Reference Pons, Vicens and García-Barrera2019, Reference Pons, García Barrera, Oviedo and Vicens2021), and the Western Interior Seaway (WIS) (Hattin, Reference Hattin1982; Kauffman, Reference Kauffman1984; Cobban et al., Reference Cobban, Skelton and Kennedy1991; Kauffman et al., Reference Kauffman, Sageman, Kirkland, Elder, Harries and Villamil1993; Hook and Cobban, Reference Hook and Cobban2013). Caribbean rudists exhibited a high biodiversity during the Campanian and Maastrichtian, with >25 genera reported (Chubb, Reference Chubb1971; Kauffman and Johnson, Reference Kauffman and Johnson1988; Johnson and Kauffman, Reference Johnson, Kauffman, MacLeod and Keller1996; Steuber et al., Reference Steuber, Mitchell, Buhl, Gunter and Kasper2002; Mitchell, Reference Mitchell2010, Reference Mitchell2022), while to the west, 18 genera have been reported from Mexico (Pons et al., Reference Pons, Vicens, Pichardo, Aguilar, Oviedo, Alencaster and Garcia-Barrera2010, Reference Pons, Vicens, Oviedo, Aguilar, Garcia-Barrera and Alencaster2013, Reference Pons, Vicens and García-Barrera2019, Reference Pons, García Barrera, Oviedo and Vicens2021). Rudists in both regions commonly constructed large biostromes in the tropical shallow carbonate platforms (Kauffman and Johnson, Reference Kauffman and Johnson1988; Johnson and Kauffman, Reference Johnson, Kauffman, MacLeod and Keller1996; Mitchell, Reference Mitchell2003, Reference Mitchell2010; Pons et al., Reference Pons, Vicens, Pichardo, Aguilar, Oviedo, Alencaster and Garcia-Barrera2010, Reference Pons, Vicens, Oviedo, Aguilar, Garcia-Barrera and Alencaster2013, Reference Pons, Vicens and Schmidt-Effing2016). The large Barrettia specimens and the supporting packstone matrix in the Flor de Alba Limestone Member documented herein provide additional examples of large biostrome growth.
In contrast to the Caribbean, rudists in the temperate WIS exhibited lower biodiversity, with only two genera reported, Radiolites and Durania, during the Campanian and Maastrichtian (Hattin, Reference Hattin1982; Everhart, Reference Everhart2018). Rudists in the WIS usually lived as solitary individuals or in small clusters within the sand- and chalk-based depositional environments (Kauffman and Sohl, Reference Kauffman and Sohl1974; Hattin, Reference Hattin1982; Kauffman, Reference Kauffman1984; Cobban et al., Reference Cobban, Skelton and Kennedy1991; Kauffman et al., Reference Kauffman, Sageman, Kirkland, Elder, Harries and Villamil1993; Hook and Cobban, Reference Hook and Cobban2013). Within the temperate and passive margin of the GCP dominated by chalk, marl, and sand deposition (Mancini et al., Reference Mancini, Puckett, Tew and Smith1995; McFarland, Reference McFarland2004; Mancini and Puckett, Reference Mancini and Puckett2005), conditions were unfavorable for construction of rudist biostromes or high biodiversity. Results herein show GCP rudists were preserved primarily as individuals and there was no fossil evidence of large biostromal growth in GCP formations. This work also reports six genera from the GCP during the Campanian and Maastrichtian: Biradiolites, Durania, Guanacastea, Gyropleura, Radiolites, and Sauvagesia. Overall, this comparison shows that rudist biodiversity in the GCP was lower than in the Caribbean but higher than in the WIS.
The current project expands the paleobiogeographic range of many rudist taxa. Durania and Guanacastea are found in both the West and East GCP, while Biradiolites, Gyropleura, Radiolites, and Sauvagesia are only found in the East GCP. This difference is likely controlled by specimen availability, because only nine rudist specimens were available to study from the West GCP, compared to 196 specimens from formations in the East GCP (Table 1). Biradiolites, Radiolites, Guanacastea, Gyropleura, and Sauvagesia were previously found only in the Caribbean, Central America, and Mexico (Alencaster, Reference Alencaster1971; Chubb, Reference Chubb1971; Scott, Reference Scott1996; Steuber et al., Reference Steuber, Mitchell, Buhl, Gunter and Kasper2002; Pons et al., Reference Pons, Vicens, Oviedo, Aguilar, Garcia-Barrera and Alencaster2013, Reference Pons, Vicens and Schmidt-Effing2016, Reference Pons, García Barrera, Oviedo and Vicens2021). This work expands their paleobiogeographic range northward to include the GCP. Similarly, Durania was found in the Caribbean, Central Mexico, and the WIS (Chubb, Reference Chubb1971; Steuber et al., Reference Steuber, Scott, Mitchell and Skelton2016), and results herein expand that range to the northeast into the GCP. Overall, this paleobiogeographic comparison shows that the GCP represents the northeastern Gulf of Mexico paleobiogeographic range of several genera and that there were no rudist taxa endemic to the GCP. Thus, rudist communities of the GCP were likely not isolated, and dispersal current paths were probably open between the GCP and regions to the south, such as Central Mexico and the Caribbean.
Combining the stratigraphic and paleogeographic occurrences of GCP rudists provides new data to reevaluate sea surface-current reconstructions in the Western Hemisphere of the Late Cretaceous (Fig. 13). Previous surface current reconstructions indicate currents flowed westward over the Caribbean (as they do today), turned northwest and diverged, with one path traveling north toward the WIS and the other traveling east towards the GCP (Johnson, Reference Johnson1999). Thus, it would be expected that B. cardenasensis and G. jamaicensis would occur in younger or synchronous strata in the GCP compared to the Caribbean and Central America. This work shows the species may have occurred in older strata in the GCP (Fig. 11). Furthermore, it may be expected then that D. maxima would occur in similarly aged strata in the GCP and WIS, although results of this work show the species occurred in younger strata in the GCP (Fig. 12). Given these temporal incongruencies, this study supplies chronostratigraphic and paleobiogeographic data to help inform future updates for reconstructions of sea surface currents in the Western Hemisphere during the Late Cretaceous.

Figure 11. Stratigraphy and biostratigraphic correlation of the Gulf Coastal Plain, the Caribbean, Central America, and Mexico. Occurrences of Biradiolites and Guanacastea species documented in this study are shown in solid black vertical lines, with occurrences from the West GCP on the left of the vertical gray bars and occurrences from the east GCP on the right (see legend description from Fig. 10). Each taxon name is followed in parentheses by the number of occurrences in the West GCP and the East GCP, respectively. (1–3) Chronostratigraphy and lithostratigraphy of GCP (see Fig. 2 for references); (4) previously documented range of B. cardenasensis (Alencaster, Reference Alencaster1971; Scott, Reference Scott1996; Pons et al., Reference Pons, Vicens, Oviedo, Aguilar, Garcia-Barrera and Alencaster2013) shown as vertical gray bar; (5) previously documented range of G. jamaicensis (Scott, Reference Scott1996; Mitchell, Reference Mitchell2003; Pons et al., Reference Pons, Vicens and Schmidt-Effing2016) shown as vertical gray bar; (6) relevant lithostratigraphy of Jamaica from Coates (Reference Coates1965) and Kauffman and Sohl (Reference Kauffman and Sohl1974); (7) relevant lithostratigraphy of Central Mexico from Myers (Reference Myers1968); (8) relevant lithostratigraphy of Guatemala from Bishop (Reference Bishop1980) and Fourcade et al. (Reference Fourcade, Piccioni, Escribá and Rossela1999); (9) B. cardenasensis range in Guinea Corn Formation from Chubb (Reference Chubb1971) and Mitchell (Reference Mitchell2003); (10) G. jamaicensis range in Guinea Corn Formation from Mitchell (Reference Mitchell2003) and Pons et al. (Reference Pons, Vicens and Schmidt-Effing2016); (11) B. cardenasensis range in Cardenas Formation from Pons et al. (Reference Pons, Vicens, Oviedo, Aguilar, Garcia-Barrera and Alencaster2013); (12) B. cardenasensis and G. jamaicensis range in the Campur/Angostura Formation from Scott (Reference Scott1996).

Figure 12. Stratigraphy and biostratigraphic correlation between the Gulf Coastal Plain and central Western Interior Seaway. Occurrences of D. maxima documented in this study are shown in solid black vertical lines, with occurrence from the West GCP on the left of the gray bar and occurrences from the east GCP on the right (see legend description from Fig. 10). Taxon name is followed in parentheses by the number of occurrences in the West GCP and the East GCP, respectively. (1–3) Chronostratigraphy and lithostratigraphy of GCP, see Figure 2 for references; (4) calcareous nannoplankton biozonations from Sissing (Reference Sissing1977) and Perch-Nielsen (Reference Perch-Nielsen, Bolli, Saunders and Perch-Nielsen1985); (5) ammonite biozonations from Cobban and Kennedy (Reference Cobban and Kennedy1995), Kennedy et al. (Reference Kennedy, Cobban and Landman1997), Cobban et al. (Reference Cobban, Walaszczk, Obradovich and McKinney2006), and Larina et al. (Reference Larina, Garb, Landman, Dastas, Thibault, Edwards, Phillips, Rovelli, Myers and Naujokaityte2016); (6) previously documented range of Durania maxima (Hattin, Reference Hattin1982; Everhart, Reference Everhart2018) shown both as vertical gray bar and vertical black line on far right of figure; (7) lithostratigraphy for the central Western Interior Seaway from Scott and Cobban (Reference Scott and Cobban1964), Gill and Cobban (Reference Gill and Cobban1973), Kauffman (Reference Kauffman1977), and Martin et al. (Reference Martin, Bertog and Parris2007); (8) ammonite zones from Cobban (Reference Cobban1993) and correlation to the GCP from Larina et al. (Reference Larina, Garb, Landman, Dastas, Thibault, Edwards, Phillips, Rovelli, Myers and Naujokaityte2016); (9) calcareous nannoplankton biozonation from Kita et al. (Reference Kita, Watkins and Sageman2017) using framework of Sissing (Reference Sissing1977) and Perch-Nielsen (Reference Perch-Nielsen, Bolli, Saunders and Perch-Nielsen1985).

Figure 13. Approximate locations of rudist material for this study (lettered solid dark gray triangles) and for referenced literature material (numbered hollow dark gray circles), along with surface current reconstructions (bold dashed light gray lines with arrows) from Johnson (Reference Johnson1999) during the Campanian and Maastrichtian. (A) Northeast Texas yielded no identifiable genera; (B) southwest Arkansas yielded Durania and Guanacastea; (C–E) north and east Mississippi and west Alabama yielded Biradiolites, Durania, Guanacastea, Gyropleura, Radiolites, and Sauvagesia; (F) central Puerto Rico yielded Barrettia. Stratigraphic and biostratigraphic correlations for (1) the WIS are shown and referenced in Figure 12 and correlations for (2) Central Mexico, (3) Guatemala, and (4) Jamacia are shown and referenced in Figure 11. Base map is same as Figure 1. Gulf Coastal Plain (GCP) outline synthesized from Murray (Reference Murray1961), Wood and Walper (Reference Wood and Walper1974), and Mancini et al. (Reference Mancini, Puckett, Tew and Smith1995). Western Interior Seaway outline synthesized from Kauffman (Reference Kauffman1977). Base country outline from QGIS (2021). WIS = Western Interior Seaway, GCP = Gulf Coastal Plain, PR = Puerto Rico.
The Prairie Bluff and Owl Creek formations are the youngest stratigraphic units to yield rudists in the GCP, although the preservation is quite poor in the latter and these new specimens do not resolve extinction timings of Western Hemisphere rudists. The top of both formations show an unconformable contact with the overlying Paleogene units (Cushing et al., Reference Cushing, Boswell and Hosman1964; Davis, Reference Davis1987). The presence of the planktonic foraminifera Racemiguembelina fructicosa (Egger, Reference Egger1899) indicates the age of the upper Prairie Bluff Formation to be at least 67 Ma (Caron, Reference Caron, Bolli, Saunders and Perch-Nielsen1985; Mancini et al., Reference Mancini, Puckett and Tew1996; Puckett, Reference Puckett2005). Thus, that is the youngest possible age for GCP rudists. Several GCP specimens are confined to the upper section of the Prairie Bluff in Mississippi and Alabama, although the formation thickness varies substantially, from 23–27 meters in Mississippi and 3–4 meters in Alabama (Mancini et al., Reference Mancini, Puckett, Tew and Smith1995; Mancini and Puckett, Reference Mancini and Puckett2005). These specimens show rudists at least persisted into the middle Maastrichtian in the GCP. Ultimately, the debate regarding whether Western Hemisphere rudist extinction occurred 1.5 or 2.5–3.0 million years before the Cretaceous-Paleogene Extinction (Johnson and Kauffman, Reference Johnson, Kauffman, MacLeod and Keller1996) or as part of the Cretaceous-Paleogene Extinction (Steuber et al., Reference Steuber, Mitchell, Buhl, Gunter and Kasper2002) will require additional specimens and study.
Overall, comparing stratigraphic and paleobiogeographic ranges of GCP rudists to the Caribbean and WIS reveals new temporal gaps and challenges, which highlight the importance of additional data. There are likely many factors that lead to these gaps, including strong effects of taphonomy, because many GCP specimens do not have species level traits preserved. There also are persistent challenges of collecting large numbers of specimens from multiple intervals. Collection issues are also present in the Caribbean and Central America, where the tropical terranes and highly faulted units result in only select exposures being readily available for study (Berryhill, Reference Berryhill1965; Nelson and Monroe, Reference Nelson and Monroe1966; Briggs, Reference Briggs1969; Schellekens, Reference Schellekens1998). Systematics also poses a challenge, because rudist taxonomy is regularly revised and many species are based on morphologic variation from localized outcrops (Skelton, Reference Skelton2013; Steuber, Reference Steuber2017). For example, Everhart (Reference Everhart2018) suggested that the systematics of all Durania species would benefit substantially from revision. In addition, the previously discussed paleocurrent reconstructions were developed using rudist occurrence data from before the year 2000 (Johnson, Reference Johnson1999). New occurrence data, including the GCP results of this study, can integrate with and complement the original reconstructions. Additional specimens and new data would help mitigate these ongoing challenges. Avocational contributions play a critical role in data accumulation as long as the provenance of the specimens can be satisfactorily vetted. All of these research challenges are potentially manageable for the production of useful data.
Acknowledgments
Researchers, staff, and volunteers of the Alabama Museum of Natural History, University of Alabama, Tuscaloosa, Alabama and the Indiana University Paleontological Collections at Indiana University, especially J. Miller-Camp, are acknowledged for their contributions in facilitating the loan of specimens and specimen curation. Avocational collectors and museum volunteers made this research possible, and thanks are extended to the many people who collected, donated, and/or assisted in the acquisition of specimens for the Mississippi Department of Wildlife, Fisheries, and Parks’ Museum of Natural Science in Jackson, Mississippi. This includes K. Irwin, Arkansas Game and Fish Commission, and C. Sloan, Arkansas Department of Transportation, for their assistance with field work, stratigraphic consultation, and acquisition of specimens. Landowners who assisted in the collecting of specimens include the J. Wynne Family. Additional specimen donors and collections include A. Armstrong, R. Wiygul, J. Robinson, J. Elliott, N. Roberts, R. Rolke, A. Smith, A. Talluto, and D. Woehr. Additionally, special thanks are extended to H. Santos and students, University of Puerto Rico, Mayaguez, and the late E.G. Kauffman, Indiana University, for field assistance with the Flor de Alba Limestone Member in Puerto Rico. This research was supported by the Galloway-Perry-Horowitz Fellowship of Indiana University and a Student Grant from the Geological Society of America to A.N. Zimmerman. This work also benefitted from the valuable and thorough suggestions of two anonymous reviewers.
Data availability statement
Supplemental Material 1 and Supplemental Material 2 are hosted at Dryad at: https://doi.org/10.5061/dryad.b5mkkwhh2.