Introduction
The Heteroptera, or sucking bugs, have a long fossil record, potentially spanning back to the Permian. The first putative heteropteran from this period is Paraknightia magnifica Evans, Reference Evans1943 from New South Wales (Evans, Reference Evans1950). However, the first definitive heteropteran, Arlecoris louisi Shcherbakov, Reference Shcherbakov2010, was recently described from the earliest Middle Triassic (early Anisian) of the northern Vosges Mountains of France. This species is also the earliest member of the infraorder Nepomorpha Popov, Reference Popov1968, a group containing the majority of truly aquatic heteropterans (Belostomatidae Leach, Reference Leach1815; Nepidae Latreille, Reference Latreille1802; Naucoridae Leach, Reference Leach1815; Corixidae Leach, Reference Leach1815; and Notonectidae Leach, Reference Leach1815). The Nepomorpha have the best fossil record of all Heteroptera (Grimaldi and Engel, Reference Grimaldi and Engel2005), no doubt because of their aquatic habits, but the fossil record of belostomatids is not yet well studied. Modern Belostomatidae are medium- (9 mm) to very large-sized (110 mm adult body length) swimming bugs that are efficient predators, grabbing prey with raptorial forelegs, injecting potent salivary secretions, and siphoning out the liquefied internal tissues of their prey with a sharp beak.
The earliest described belostomatid fossils are from the Jurassic, when the family first diversified. The oldest of these is Odrowazicoris polonicus Popov, Reference Popov1996, an isolated wing in Hettangian-age beds of the Holy Cross Mountains of Poland. A number of other Jurassic species have been described from Europe and the United States, including: Tarsabedus menkei Popov, Dolling, and Whalley, Reference Popov, Dolling and Whalley1994; Aenictobelostoma primitivum Polhemus, Reference Polhemus2000; Stygeonepa foersteri Popov, Reference Popov1971; and Nettelstedtia breitkreutzi Popov, Rust, and Brauckmann, Reference Popov, Rust and Brauckmann2000. The Early Cretaceous also harbored diverse species and genera, including three taxa from the Crato Formation Plattenkalk of Brazil, and a species possessing unique paddle-shaped metathoracic legs, Iberonepa romerali Martínez-Delclòs, Nel, and Popov, Reference Martínez-Delclòs, Nel and Popov1995, from Las Hoyas, Spain.
Though belostomatids are widespread in Cenozoic sediments, many are still undescribed, including a Paleocene specimen from Alberta, Canada (Mitchell and Wighton, Reference Mitchell and Wighton1979), two unnamed early Eocene specimens from Denmark (Larsson, Reference Larsson1975; Rust and Ansorge, Reference Rust and Ansorge1996), and a late Oligocene specimen from Germany (Wedmann, Reference Wedmann2000). The extant genus Lethocerus Mayr, Reference Mayr1853 became fairly diverse during the Miocene, with two species (L. sulcifemoralis Říha and Kukalová, 1967, and L. turgaicus Popov, Reference Popov1971) found in the Oligocene–Miocene of the Czech Republic and the Miocene of Russia, respectively. Additionally, two modern species, Lethocerus americanus Leidy, Reference Leidy1847 and Belostoma bakeri Montandon, Reference Montandon1913, are reported to occur in Late Pleistocene asphalt deposits of California (Miller, Reference Miller1983). See Table 1 for a complete list of fossil belostomatid species.
Table 1 List of fossil belostomatid species.

Today, belostomatids have a worldwide distribution, although the majority of species are found in the tropics. They are represented by three subfamilies consisting of nine genera and ~146 species (Schuh and Slater, Reference Schuh and Slater1995). The subfamily Belostomatinae Lauck and Menke, Reference Lauck and Menke1961, is by far the largest group within Belostomatidae and contains six genera: Abedus Stål, Reference Stål1862; Appasus Amyot and Serville, Reference Amyot and Serville1843 (Polhemus, Reference Polhemus1995); Belostoma Latreille, Reference Latreille1807; Diplonychus Laporte, Reference Laporte1833; Hydrocyrius Spinola, Reference Spinola1850; and Limnogeton Mayr, Reference Mayr1853. The subfamily Horvathiniinae Lauck and Menke, Reference Lauck and Menke1961, is monogeneric, consisting of nine species in the genus Horvathinia Montadon, Reference Montandon1911. The third subfamily, Lethocerinae Lauck and Menke, Reference Lauck and Menke1961, consists of three genera: Lethocerus Mayr, Reference Mayr1853; Benacus Stål, Reference Stål1861 (Goodwyn, Reference Goodwyn2006); and Kirkaldyia Montandon, Reference Montandon1909 (Goodwyn, Reference Goodwyn2006).
The current belostomatid phylogeny is based on morphology and reproductive behaviors: (Lethocerinae, (Horvathiniinae (Belostomatinae))) (Lauck and Menke, Reference Lauck and Menke1961; Mahner, Reference Mahner1993; Smith, Reference Smith1997). Lethocerinae is the most basal taxon because it retains many characters of Nepidae (‘water scorpions’), the sister group to Belostomatidae. In addition, all species of Lethocerinae are emergent-brooders, meaning that their eggs are deposited on emergent vegetation and attended to by the males. In contrast, the more derived members of Belostomatinae are back-brooders, in which eggs are deposited on the backs of their male mates. Horvathiniinae is placed between Lethocerinae and Belostomatinae because of its more intermediate morphological characteristics. Brooding behavior has not been observed in this group and its phylogenetic position is less certain (Lauck and Menke, Reference Lauck and Menke1961).
The relationships within subfamily Belostomatinae are mostly resolved based on morphology, with the exception of the genus Limnogeton. Its position has been questioned due to its lack of raptorial forelegs and natatorial mid and hind legs. Lauck and Menke (Reference Lauck and Menke1961) noted the possibility that these characters may indicate a more basal position within Belostomatidae. However, it has been placed within subfamily Belostomatinae because it exhibits back-brooding behavior (Voelker, Reference Voelker1968). This position was supported in a recent phylogenetic analysis of Nepomorpha (Brożek, Reference Brożek2014).
This paper describes the earliest known species of family Belostomatidae, Triassonepa solensis n. gen. n. sp., from the Late Triassic Cow Branch Formation of southern Virginia and northern North Carolina. Based on both the species assemblage within the formation and the known habitats of modern belostomatids, probable chemical and environmental conditions of the deposit are discussed.
Materials and methods
Locality and material
The fossils described in this study are from the Cow Branch Formation of southern Virginia and northern North Carolina, a Late Triassic (Carnian/early Norian, 230–220 Ma) deposit outcropping in the former Solite Quarry where >30 Van Houten cycles are preserved. Three cycles have yielded insect fossils, but one cycle has produced the majority of the insects in this formation (Olsen et al., Reference Olsen, Remington, Cornet and Thomson1978; Fraser et al., Reference Fraser, Grimaldi, Olsen and Axsmith1996). The Cow Branch Formation is significant because it preserves the oldest fauna of freshwater insects, which are preserved as thin, two-dimensional, silvery films in a matrix of very fine-grained, black shale. Preservation is often excellent, with many specimens fully articulated and microscopic details visible. In addition to 11 orders of insects (Fraser and Grimaldi, Reference Fraser and Grimaldi2003; Grimaldi et al., Reference Grimaldi, Junfeng, Fraser and Rasnitsyn2005), the Solite Quarry has produced numerous amphibious reptiles (Tanytrachelos ahynis Olsen, Reference Olsen1979), a gliding reptile (Mecistotrachelos apeoros Fraser et al., Reference Fraser, Olsen, Dooley and Ryan2007), fish fossils, dinosaur footprints, and many plant species (Olsen et al., Reference Olsen, Remington, Cornet and Thomson1978).
Methods
Specimens were viewed using a Nikon SMZ1500 microscope, fitted with a fiber optic ring light. This non-directional, diffuse light source was necessary to illuminate the silvery, carbonized film by which the insects are preserved. In order to examine the minute details, specimens were wetted with 70% ethanol to increase contrast between the fossil and the black shale matrix. Photographs were taken using two separate setups. Specimens viewed at the American Museum of Natural History (AMNH) were photographed with a Nikon 16MP camera and Nikon Elements NIS software on a Nikon SMZ1500 stereomicroscope. Specimens housed at the Virginia Museum of Natural History (VMNH) were photographed with a Canon 6D DSLR camera using Canon Utility 2 software. All specimen measurements were taken using ImageJ software. Total lengths of adults were measured from the anterior margin of head to the distal margin of the 7th abdominal segment, in order to exclude the 8th abdominal segment, which protrudes to varying degrees in different specimens.
Repositories and institutional abbreviations
Most of the material examined in this study is housed at the Virginia Museum of Natural History (VMNH), Martinsville, Virginia, USA; some specimens are deposited in the American Museum of Natural History (AMNH), New York, USA.
Systematic paleontology
Suborder Heteroptera Latreille, Reference Latreille1810
Infraorder Nepomorpha Popov, Reference Popov1968
Superfamily Nepoidea Latreille, Reference Latreille1802
Family Belostomatidae Leach, Reference Leach1815
Genus Triassonepa new genus
Type species
Triassonepa solensis n. gen. n. sp. by present designation.
Diagnosis
As for type species, by monotypy.
Etymology
The genus name is a combination of the prefix Triasso-, for the Triassic Period from which the genus is derived, and -nepa, a standard suffix used for the superfamily Nepoidea.
Occurrence
Former Solite Quarry C, Eden, Rockingham County, North Carolina, USA (36°32'29.6556''N, 79°40'12.8424''W); Carnian/Norian, Late Triassic, Cow Branch Formation.
Remarks
Triassonepa n. gen. differs from all other known extinct and extant genera of Belostomatidae by the structure of the foreleg, in which the tarsus is elongate and opposed to the tibia + femur.
Triassonepa solensis new genus new species
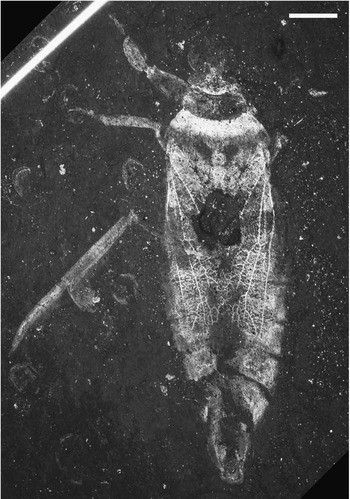
Figure 1 Triassonepa solensis n. gen. n. sp., habitus of holotype, VMNH 94671. Scale bar is 2 mm.
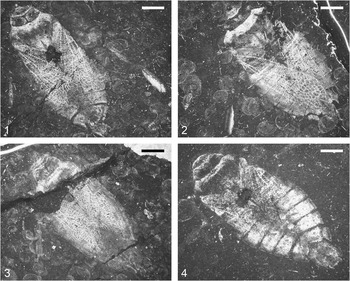
Figure 2 Photographs of adult specimens of Triassonepa solensis n. gen. n. sp.: (1) VMNH 49641; (2) VMNH 53881; (3) VMNH 53880; (4) VMNH 94672. Scale bars are 2 mm.
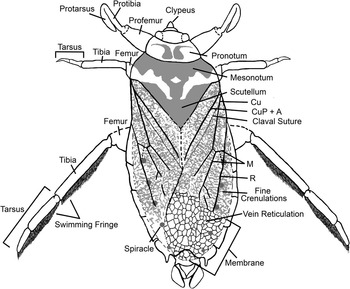
Figure 3 Reconstruction of Triassonepa solensis n. gen. n. sp. showing major morphological characters.

Figure 4 Morphological comparison of the forelegs, hemelytra, metathoracic legs, and terminalia of adult Triassonepa solensis n. gen. n. sp. and extant belostomatid species: (1) foreleg, VMNH 94671, holotype; (2) foreleg, Belostoma flumineum Say, Reference Say1832; (3) foreleg, Benacus griseus (Say, Reference Say1832) (formerly genus Lethocerus); (4) hemelytra, VMNH 53881; (5) hemelytra, Diplonychus urinator sudanensis Linnavuori, Reference Linnavuori1971; (6) setal fringe on metathoracic leg, VMNH 50230; (7) setal fringe on metathoracic leg, Belostoma elongatum Montandon, Reference Montandon1908; (8) double setal fringe on metathoracic leg, VMNH 90281; (9) double setal fringe on metathoracic leg, VMNH 90279; (10) metathoracic leg, VMNH 94671, holotype; (11) female terminalia, VMNH 90281; (12) male terminalia, VMNH 94671, holotype; (13) terminalia, Diplonychus urinator sudanensis Linnavuori, Reference Linnavuori1971. Scale bars are 1 mm.
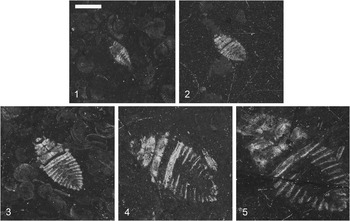
Figure 5 Triassonepa solensis n. gen. n. sp. growth series: (1) Instar I, VMNH 94575a; (2) Instar II, VMNH 50512; (3) Instar III, VMNH 53871; (4) Instar IV, VMNH 54155; (5) Instar V, VMNH 52498. Scale bar is 2 mm, located in photo 1.
Holotype
VMNH 94671, male, Fig. 1.
Diagnosis
Body elongate. Head small, largely hidden beneath pronotum dorsally, with triangular clypeus. Pronotum small with concave anterior and posterior margins. Hemelytra with prominent claval suture; dense reticulations on apical third. Prothoracic legs raptorial, with elongate tarsus opposed to tibia. Metathoracic tibia and tarsus with dense setal fringe on inner (mesal) margin. Abdomen with seven visible segments (segments 2–8) and five visible pairs of spiracles.
Occurrence
Former Solite Quarry C, Eden, Rockingham County, North Carolina, USA (36°32'29.6556''N, 79°40'12.8424''W); Carnian/Norian, Late Triassic, Cow Branch Formation.
Description of Adults
The description is based on the ~36 adult specimens collected to date (Fig. 2). Body elongate; total length (anterior margin of head, excluding clypeus, to apex of 7th abdominal segment) 10.6–14.1 mm (mean 12.1 mm).
Head
Small, largely hidden beneath pronotum dorsally, with triangular clypeus. Eyes, antennae, and mouthparts not visible. Ocelli, if present, not visible.
Thorax
Pronotum small, 2.5–3.5 mm wide, 0.8–1.1 mm long, with concave anterior and posterior margins. Mesonotum width ~1.6x pronotum width; possible pigmentation preserved on pronotum and mesonotum. Scutellum about as wide as pronotum, but with indistinct margins. Hemelytra with fine crenulations on proximal two-thirds, reticulations on distal third; clavus and claval suture distinct; venation as shown in Figure 3 (also Fig. 4.4). Hindwing, if present, not preserved. Prothoracic legs raptorial (Fig. 4.1); femur wide at base (~1 mm), narrowed distally, partially obscured by body; tarsus elongate and opposed to tibia, tibia and tarsus of similar width, tibia length ~1.1x length of tarsus; tarsal claw not visible. Mesothoracic legs slender, with no setae or fringe apparent; tibia ~1.3x length of entire tarsus. Metathoracic legs slender, with dense setal fringe on inner (mesal) margin of tibia and tarsus (Fig. 4.6); few specimens with double setal fringe on tarsus (Fig. 4.8, 4.9); femur partially obscured by body; tibia length ~1.2x tarsus length; tarsus with two segments.
Abdomen
Broad, often obscured by hemelytra; length (7.4–8.6 mm) and width (5.4–6.3 mm) vary, likely due to diagenetic alteration; abdominal segments 2–8 visible; dorsum with five pairs of spiracles visible on segments 3–7. Spiracle diameter ranges from 0.26 to 0.40 mm (mean 0.34 mm). Terminalia variable, but with lateral paddle-like lobes; pair of short processes between the lobes that resemble respiratory tubes; two specimens (VMNH 94671, Fig. 4.12, holotype; VMNH 94672, Fig. 2.4) with more elongate terminalia and two distal articulated appendages, possibly claspers.
Description of nymphs
The description is based on 51 nymph specimens. Body oval, narrowed at anterior and posterior ends. Head small, often not preserved, no features discernable. Thorax roughly trapezoidal with dividing line between lateral halves; wing pads not visible. Prothoracic and mesothoracic legs not preserved. Few specimens with metathoracic tarsus preserved; setal fringe present, as in adults; two specimens (e.g., VMNH 54155, Fig. 5.4) with double setal fringe; claws, if present, not visible. Abdomen roughly triangular; six segments visible (segments 3–8).
Etymology
The specific epithet is named after the former Virginia Solite Corporation quarries, from which the specimens were recovered.
Remarks
Specimen VMNH 94671 was chosen as the holotype because of the excellent preservation of its legs (Fig. 1). It is the only specimen yet found with a preserved foreleg, and one of only two specimens with a preserved mesothoracic leg. Though this specimen has a narrower abdomen and lacks the stoutness of the other specimens, this difference in overall shape can likely be attributed to diagenesis due to its morphologic similarity to the other specimens. Additionally, it is the largest specimen (14.10 mm), but it is of a similar size to that of the only other male specimen (VMNH 94672, Fig. 2.4, 13.49 mm). This may indicate that males of this species were larger than the females, which is unusual in the Heteroptera.
Triassonepa solensis n. gen n. sp. is the earliest known member of the family Belostomatidae. Unfortunately, all adult specimens are preserved in dorsal aspect, concealing many morphological characters. This is interesting because preservation of the ventral surface is quite common in other belostomatid fossils (e.g., Sinobelostoma liui, Chou and Hong, Reference Chou and Hong1989; Lethocerus vetus, Nel and Waller, Reference Nel and Waller2006). Triassonepa solensis n. gen. n. sp. ranges in length from ~10.6–14.1 mm, placing it on the small side of the range of modern belostomatids (9–110 mm; Schuh and Slater, Reference Schuh and Slater1995).
The head of Triassonepa solensis n. gen. n. sp. is poorly preserved in almost all specimens, preventing a detailed description at this time. The only feature often visible is the triangular clypeus. Neither compound eyes nor ocelli are preserved, although the absence of ocelli may be expected since modern belostomatids do not possess them (Lauck and Menke, Reference Lauck and Menke1961). The absence of preserved antennae is also expected given that the antennae of modern belostomatids are small and concealed within grooves beneath their heads (Schuh and Slater, Reference Schuh and Slater1995). In addition, due to the exclusive preservation of the dorsal surface, no mouthparts are visible.
The legs of Triassonepa solensis n. gen. n. sp. provide some interesting characters for comparison with modern members of the Belostomatidae. One unique character of this species is the structure of its prothoracic legs (Fig. 4.1). Though only preserved in a single specimen, the foreleg of T. solensis has an elongate tarsus that opposes the tibia. In contrast, modern belostomatids have a thin tibia + tarsus opposed to the femur (Fig. 4.2, 4.3). This raptorial foreleg appears to be less specialized than those of modern belostomatids. Another difference between T. solensis and modern belostomatids is the possession of setae on the legs. Modern belostomatids have setae on both the mesothoracic and metathoracic legs, but it appears that the mesothoracic legs of T. solensis lack these setae. This might suggest that instead of using these legs for swimming, they may have used them in conjunction with the forelegs to capture and hold prey. However, only three mesothoracic legs on two specimens have been recovered to date, indicating that the absence of these setae is not yet definitive. Triassonepa solensis n. gen. n. sp. does, however, possess a setal fringe on the metathoracic legs (Fig. 4.6, 4.8–4.10), indicating that they were specialized swimmers. It is interesting to note that a few specimens (including two nymphs) appear to have a double setal-fringe (Fig. 4.8, 4.9). It is unclear at this time whether this double-fringe is a result of the position in which they were preserved, or if these specimens are a distinct species. Measurements of body length, abdomen width, and tarsal length have shown that these two specimens have similar proportions to specimens with a single fringe, and therefore may be the same species.
The hemelytra of Triassonepa solensis n. gen. n. sp. also provide good features for comparison to modern taxa. The hemelytra do not appear to cover the entire abdomen of any specimen, but this is likely an artifact of preservation (Fig. 4.4). Because the apical parts of modern heteropteran hemelytra are thin and membranous, it is unlikely that these regions would have been preserved. In addition, the abdominal spiracles of T. solensis are located on its dorsal surface, which is typical of insects that breathe underwater via a plastron (a thin film of air held beneath the wings and used as a physical gill). In order for T. solensis to use a plastron, as is done by many modern aquatic insects including belostomatids (e.g., Abedus herberti Hildago, Reference Hildago1935; see Goforth and Smith, Reference Goforth and Smith2012), its hemelytra would need to fully cover its spiracles (i.e., to the edges of the abdomen).
The 8th abdominal segment of Triassonepa solensis n. gen. n. sp. contains two lateral, paddle-shaped lobes and two medial processes resembling respiratory tubes (Fig. 4.11). It is morphologically quite similar to the eighth abdominal segment of female naucorids (particularly Ilyocoris exclamationis Scott, Reference Scott1874 as illustrated by Lee, Reference Lee1991). This suggests that: (1) T. solensis occupies a very basal position within Belostomatidae, and (2) the majority of the preserved specimens were likely female. However, two specimens (VMNH 94671, Fig. 4.12, holotype; VMNH 94672, Fig. 2.4) possess terminalia with a slightly different structure. The 8th abdominal segments of these specimens are more elongate and possess two apical, articulated appendages that resemble claspers. It is therefore likely that these two specimens are males.
Immature Triassonepa solensis n. gen. n. sp. were separated into instars based on total body lengths (Fig. 5). Because the head was not often preserved, there is a minor amount of uncertainty in some of the measurements. A total of 51 nymphs were measured from the anterior margin of the head to the apex of the abdomen, yielding five size classes (Fig. 6): Instar I, 1.6–2.3 mm (mean 2.0 mm); Instar II, 2.6–3.4 mm (mean 3.0 mm); Instar III, 4.0–5.2 mm (mean 4.6 mm); Instar IV, 6.2–7.7 mm (mean 6.9 mm); Instar V, 9.5–10.3 mm (mean 9.9 mm). Each instar was ~1.5x larger than the preceding one. Modern belostomatids also have five instars and show a similar growth ratio of 1.2–1.5 with each successive instar (Tables 2, 3). These ratios correspond to Dyar’s Rule, which states that an insect instar is ~1.4x the size of its previous instar.

Figure 6 Lengths of Triassonepa solensis n. gen. n. sp. instars and adults: 70 specimens (51 nymphs, 19 adults) were measured. The two largest adult specimens are males.
Table 2 Mean lengths (in mm) of the five instars of Triassonepa solensis n. gen. n. sp. (this study), Lethocerus maximus (Cullen, Reference Cullen1969), L. mazzai (De Carlo, Reference De Carlo1962), Hydrocyrius columbiae (Miller, Reference Miller1961), Belostoma flumineum (Flosi, Reference Flosi1980), B. malkini (Cullen, Reference Cullen1969), and Abedus breviceps (Keffer and McPherson, Reference Keffer and McPherson1988).

Table 3 Growth ratios Triassonepa solensis n. gen. n. sp. (this study), Lethocerus maximus (Cullen, Reference Cullen1969), L. mazzai (De Carlo, Reference De Carlo1962), Hydrocyrius columbiae (Miller, Reference Miller1961), Belostoma flumineum (Flosi, Reference Flosi1980), B. malkini (Cullen, Reference Cullen1969), and Abedus breviceps (Keffer and McPherson, Reference Keffer and McPherson1988) (the same species as in Table 2), calculated by dividing instar length by length of the previous instar.

Discussion
Belostomatid habitats
Due to the presence of swimming fringes on the hind legs of Triassonepa solensis n. gen. n. sp., it is reasonable to assume that this species had similar physiology and behaviors to modern belostomatids. Understanding the habitats of these modern belostomatids has implications for determining the nature and chemistry of ancient ‘Lake Solite.’
Belostomatids live in a wide variety of habitats, but are most commonly found in shallow bodies of water with marginal vegetation. Kashian and Burton (Reference Kashian and Burton2000) reported Lethocerus sp. from the wetlands of northern Lake Huron in areas dominated by sedges. Belostomatids also occur in many of India’s small freshwater lakes. Majumder et al. (Reference Majumder, Das, Majumder, Ghosh and Agarwala2013), for example, found two genera (Lethocerus and Diplonychus) living in the marginal vegetation of manmade, urban lakes in Tripura, northeastern India. Diplonychus rusticus Fabricius, Reference Fabricius1781 was collected from both Pocharam Lake in southeastern India (Deepa and Rao, Reference Deepa and Rao2007), and Loktak Lake of northeastern India (Takhelmayum and Gupta, Reference Takhelmayum and Gupta2011). Loktak Lake is unique in its possession of phumdis, which are floating islands composed of vegetation, organic matter, and soil, among which belostomatids live. Belostomatids have also been collected from arid wetlands such as Bañado Carilauquen in west-central Argentina (Scheibler and Ciocco, Reference Scheibler and Ciocco2013). These semi-permanent wetlands are located near a shallow, saline lake, although the wetlands themselves have negligible salinity.
In addition to shallow lakes and wetland environments, belostomatids inhabit the marginal areas of deep lakes, such as Lake Victoria in Kenya (Muli and Mavuti, Reference Muli and Mavuti2001; Orwa et al., Reference Orwa, Omondi, Ojwang and Mwanchi2015). Three species were identified there: Diplonychus sp. (formerly genus Sphaerodema), Hydrocyrius sp., and Lethocerus niloticus Stål, Reference Stål1885. Similarly to Loktak Lake, Diplonychus sp. and Hydrocyrius sp. were found to inhabit the lake’s floating hyacinth mats (Orwa et al., Reference Orwa, Omondi, Ojwang and Mwanchi2015).
Belostomatids are one of the few groups of aquatic insects that can tolerate agriculture-affected and polluted water bodies. Belostomatids inhabit the length of the Enfranz River in Ethiopia, from the clean headwaters to the agriculture-dominated mouth (Mehari et al., Reference Mehari, Wondie, Mingist and Vijverberg2014). Other nepomorphs, such as naucorids and nepids, were only found in the unaffected, upstream areas. Additionally, Belostoma sp. has been collected in the eutrophic Kipkaren River of Kenya (Aura et al., Reference Aura, Raburu and Herrmann2011) and Diplonychus sp. was found to inhabit the margins of a number of polluted Bangalore lakes in southern India (Balachandran and Ramachandra, Reference Balachandran and Ramachandra2010). Perhaps the most extreme case is the collection of Belostoma sp. from hydrogen sulfide-rich Cueva del Azufre in Tabasco, Mexico (Tobler et al., Reference Tobler, Schlupp and Plath2007).
Belostomatids are also known to inhabit temporary environments such as rain pools (Fontanarrosa et al., Reference Fontanarrosa, Collantes and Bachmann2009), agricultural fields (Das and Gupta, Reference Das and Gupta2010), rice paddies (Hendawy et al., Reference Hendawy, Sherif, Abada and El-Habashy2005), and sinkholes (Blinn and Sanderson, Reference Blinn and Sanderson1989), and are common inhabitants of ephemeral playa lakes in arid environments (Haukos and Smith, Reference Haukos and Smith1992). Belostomatids have been collected in the stagnant ‘buffalo-wading pools’ of Tarangire National Park on the savanna of northern Tanzania (D.G., personal observation). Merickel and Wangberg (Reference Merickel and Wangberg1981) collected Belostoma flumineum along the shores of two playas near Lubbock, Texas, and Richardson et al. (Reference Richardson, Ward and Huddleston1972) found one juvenile belostomatid in the Jornada Playa of New Mexico. Adult belostomatids disperse to these ephemeral environments via flight, and as a result, are often found at bright lights during the night.
A few studies have even reported belostomatids inhabiting brackish waters. Angelin et al. (Reference Angelin, Jehamalar, Das and Kumar2010) collected Diplonychus sp. and Belostoma sp. from an estuary in southern India with a salinity of between 4‰ and 8‰ (ppt). Siddiqi (Reference Siddiqi2008) reported belostomatids in the marginal areas of India’s Lake Lonar, which is a hyperalkaline, saline, crater lake with a pH of ~10.5 (Siddiqi, Reference Siddiqi2008) and a salinity of up to ~6‰ (Yannawar and Bhosle, Reference Yannawar and Bhosle2013). However, Badve et al. (Reference Badve, Kumaran and Rajshekhar1993) report that the marginal areas of the lake near the inflow of the freshwater springs have a pH closer to 7.5. It is in these areas that the marshes exist, and it is likely that the belostomatids inhabit these more suitable areas.
Environmental interpretation of ‘Lake Solite’
Like their modern counterparts, many fossil belostomatids are reported from shallow, lacustrine paleoenvironments (e.g., Grimaldi and Maisey, Reference Grimaldi and Maisey1990; Martínez-Delclòs et al., Reference Martínez-Delclòs, Nel and Popov1995; Prokop and Nel, Reference Prokop and Nel2000). However, this contrasts with both interpretations of the paleoenvironment of the Cow Branch Formation. Olsen et al. (Reference Olsen, Remington, Cornet and Thomson1978) first described the environment as a large, deep, chemically stratified lake. This stratification would have produced anoxic bottom waters that prevented bioturbation and therefore allowed for exquisite fossil preservation of delicate insects such as midges and tiny hemipterans. Although unusual for a modern belostomatid habitat, other deep lacustrine paleoenvironments have been reported to contain belostomatid fossils. One such deposit, the late Oligocene Enspel Formation of Germany (Poschmann et al., Reference Poschmann, Schindler and Uhl2010), has produced ten belostomatid fossils, four of which are adult specimens (Wedmann, Reference Wedmann2000).
Recent research by Liutkus et al. (Reference Liutkus, Beard, Fraser and Ragland2010) proposed that the Cow Branch Formation was a shallow, alkaline, saline, rift valley lake. They presented a number of reasons for this interpretation: (1) dominance of terrestrial and nearshore-dwelling insects and terrestrial vascular plants, (2) exquisite fossil preservation, and (3) presence of dolomite and absence of quartz and zirconium throughout the deposit.
Based on the environmental preferences of modern belostomatids, their abundance in the Cow Branch Formation would indicate a shallow, nearshore paleoenvironment. The tolerance of modern belostomatids for polluted and harsh water conditions suggests they may have also been tolerant to extreme environments such as saline, alkaline, rift valley lakes. Because belostomatids breathe air, they would be unaffected by poor water quality. However, harsh water conditions would affect organisms possessing gill respiration (i.e., Ephemeroptera, Plecoptera, Odonata, Trichoptera, etc.), so the lack of gilled insect nymphs of these orders within the deposit is good evidence for poor water quality. The only gilled insect order reported from the Cow Branch Formation is Diptera (Liutkus et al., Reference Liutkus, Beard, Fraser and Ragland2010). However, this appears to be a misidentification of the enigmatic, gilled, larva-like arthropod, which may actually be a crustacean.
In addition to belostomatids, the insects preserved in the Cow Branch Formation are mostly terrestrial adults from the orders Hemiptera (Sternorrhyncha), Diptera, and Coleoptera. A few other taxa have been found to date, including adult members of Blattodea, Odonata, Orthoptera, Plecoptera (Fraser and Grimaldi, Reference Fraser and Grimaldi2003), Thysanoptera (Grimaldi et al., Reference Grimaldi, Shmakov and Fraser2004), Mecopterida (Grimaldi et al., Reference Grimaldi, Junfeng, Fraser and Rasnitsyn2005), Amphiesmenoptera, and Neuroptera. This unique assemblage of terrestrial insects further suggests that the water was toxic to gill-possessing, aquatic larvae and other sensitive groups. Moreover, Fraser and Grimaldi (Reference Fraser and Grimaldi1999) noted the abundance of conchostracans within the insect bed. Modern members of this group are most commonly found in ephemeral, alkaline water bodies (Tasch, Reference Tasch1969).
Liutkus et al. (Reference Liutkus, Beard, Fraser and Ragland2010) discussed the exquisite preservation of the insect fossils as evidence for a shallow lake. Most insects are completely articulated, which is quite rare for Triassic fossils (cf., Riek, Reference Riek1974; Brauckmann and Schlüter, Reference Brauckmann and Schlüter1993; Shcherbakov et al., Reference Shcherbakov, Lukashevich and Blagoderov1995; Martins-Neto et al., Reference Martins-Neto, Gallego and Zavattieri2008). The lack of disarticulation suggests limited postmortem movement. If the lake had been as deep as originally suggested, the insects would likely have decayed, disarticulated, or have been eaten before settling to the benthic zone. Moreover, there are no fossil fish found in the insect layers, further evidence that the insects were buried in very shallow water.
The geochemistry of the deposit also supports the interpretation of a saline, alkaline lake. Liutkus et al. (Reference Liutkus, Beard, Fraser and Ragland2010) reported dolomitic claystone throughout the insect bed, and in modern lakes primary precipitation of dolomite occurs most often in waters with elevated salinity, alkalinity, and with abundant magnesium and calcium (DeDeckker and Last, Reference De Deckker and Last1989). Furthermore, the surrounding basin is rich in quartz, making its absence in the Cow Branch deposit significant. In addition, albite is abundant in the deposit (Liutkus et al., Reference Liutkus, Beard, Fraser and Ragland2010), which is proposed to have formed by the reaction of clay, quartz, and sodium under alkaline conditions (van de Kamp and Leake, Reference van de Kamp and Leake1996). The deposit is also depleted in zirconium. Ayers and Zhang (Reference Ayers and Zhang2005) have demonstrated that this element dissolves in alkaline conditions. These three geochemical conditions support a saline, alkaline environment during the time of deposition.
Although most fossil belostomatids have been reported from shallow, non-saline, lacustrine environments, Polhemus (Reference Polhemus2000) reported fossils from a saline environment, the Jurassic Todilto Formation of New Mexico. This formation is interpreted as a paralic, saline playa due to its interfingered marine and continental sediments (Lucas et al., Reference Lucas, Rinehart and Estep2000). Although this interpretation is somewhat controversial, the water chemistry in this type of environment may have been similar to that proposed by Liutkus et al. (Reference Liutkus, Beard, Fraser and Ragland2010) for the Cow Branch Formation. Vega et al. (Reference Vega, García-Barrera, Perrilliat, Coutiño and Mariño-Pérez2006) gave a second example of a potentially saline belostomatid habitat from the Early Cretaceous Sierra Madre Formation of southeastern Mexico, which has been interpreted as a brackish marginal lagoon or estuary. Due to the wide environmental tolerances of both fossil and modern belostomatids, the dominance of terrestrial adult insects and lack of aquatic nymphs, the abundance of conchostracans, the exquisite preservation of the Solite fossils, and the geochemistry of the Cow Branch Formation, it is very likely that ‘Lake Solite’ was a shallow, saline, alkaline rift valley lake.
Acknowledgments
J.C. is grateful to the American Museum of Natural History Grants Program for the Theodore Roosevelt Memorial Grant, which provided the funding necessary to visit and collect at the Solite deposit. J.C. is especially grateful to C. Byrd for hosting her visits to the Virginia Museum of Natural History and to R. Vodden (VMNH) for allowing her to participate in the fossil excavation at the Solite deposit. We are grateful for the thoughtful commentary on this manuscript provided by B.W. Smith and an anonymous reviewer. Financial research support for this project came from a Graduate Teaching Assistantship from the Department of Earth and Planetary Sciences, Rutgers University.