Introduction
Microcotyle Van Beneden & Hesse, Reference Van Beneden and Hesse1863 is one of the most frequent and diverse monogenean genera, with 67 species listed in the World Register of Marine Species (2022). This diversity implies a high taxonomic complexity, and as a consequence of Microcotyle being the first microcotylid genus erected, many species were initially assigned to this taxon. Most species were subsequently reassigned to new genera (see Mamaev, Reference Mamaev1986; World Register of Marine Species, 2022), but many citations still need revision. The diversity of Microcotyle is also reflected in the host range, with the parasites infecting several species of different fish orders, mostly Eupercaria and Perciformes (formerly belonging to Perciformes and Scorpaeniformes, respectively, see World Register of Marine Species, 2022). Among various host families, species of Microcotyle (13.4%) have mostly been reported in sparids (Eupercaria), mainly in the Mediterranean (see also Lablack et al., Reference Lablack, Rima, Georgieva, Marzoug and Kostadinova2022). Within the Scorpaenoidei (Perciformes), 7.5% of Microcotyle species have been reported in sebastids and 3% in scorpaenids (Gibson et al., Reference Gibson, Bray and Harris2006; Ayadi et al., Reference Ayadi, Gey, Justine and Tazerouti2017; Ono et al., Reference Ono, Matsumoto, Nitta and Kamio2020; only including reports identified up to species level). To date, these records are mostly from the Pacific Ocean, but also from the Atlantic Ocean and the Mediterranean Sea.
The great morphological resemblance between Microcotyle species requires the exploration of new morphological characters to differentiate at species level. For example, based on their similarity, several specimens of Microcotyle spp. from numerous hosts worldwide have been identified as Microcotyle sebastis or Microcotyle erythrini, but many of these records have been considered as doubtful, often revised and reclassified in different species (e.g. Ayadi et al., Reference Ayadi, Gey, Justine and Tazerouti2017; Bouguerche et al., Reference Bouguerche, Gey, Justine and Tazerouti2019b; Víllora-Montero et al., Reference Víllora-Montero, Pérez-del-Olmo, Georgieva, Raga and Montero2020). There is an additional need to expand the molecular database and define the genome regions and distance ranges useful for differentiating species. This study provides the description of specimens found in a recent study on scorpaenoids of commercial interest (Helicolenus dactylopterus (Delaroche, 1809) and Scorpaena spp.) from the Mediterranean and north-east Atlantic. The descriptions include a new species of Microcotyle and a new host and locality records for Microcotyle algeriensis. These new findings contribute to the knowledge about the diversity of this parasite genus, which is particularly abundant in the study area, specially in scorpaenoid hosts.
Materials and methods
Sample collection
A total of 107 H. dactylopterus (Sebastidae) and 107 Scorpaena spp. (Scorpaenidae: 37 Scorpaena notata Rafinesque, 1810, 42 Scorpaena porcus L. and 28 Scorpaena scrofa L.) were examined for microcotylid infections. Fishes were collected by commercial bottom trawling vessels during the period 2015–2016, off Guardamar del Segura (Balearic, Division 37.1.1, Western Mediterranean Sea), and Galicia (Bay of Biscay-South, Division 27.8.c, north-east Atlantic) (see table 1 for the biological and geographical data of samples; fishing areas reported according to the United Nations Food and Agriculture Organization, https://www.fao.org/fishery/en/area/search). Fish were stored on ice and transferred to the laboratory (Cavanilles Institute of Biodiversity and Evolutionary Biology) where they were weighed and measured (weight provided in g and standard length in cm, expressed as the range with the mean and standard deviation in parentheses; this information is only provided for infected hosts in the parasite description sections). Fish were dissected fresh and the gills were examined for monogeneans under a stereomicroscope. All specimens were collected and washed in 0.9% saline solution. Two different protocols described in Víllora-Montero et al. (Reference Víllora-Montero, Pérez-del-Olmo, Georgieva, Raga and Montero2020) were used for the fixation and storage of mature specimens of Microcotyle spp. Firstly, with specimens selected for molecular analyses, the testes and clamps were counted in temporary saline solution preparations and then the entire worms were photographed prior to excising the specimens into three parts. The anterior and posterior parts were kept as molecular vouchers (hologenophores sensu Pleijel et al., Reference Pleijel, Jondelius, Norlinder, Nygren, Oxelman, Schander, Sundberg and Thollesson2008) and the middle part of the body was used for DNA extraction (the latter material was preserved in molecular-grade ethanol). In the second protocol, used for morphological analyses, representative specimens (not broken, contracted, stretched, wrinkled or folded) were selected, fixed in 4% formaldehyde solution for four days and preserved in 70% ethanol. The prevalence, expressed as a percentage, was calculated as the number of infected fish divided by the total number of analysed fish, and mean intensity, expressed as the mean ± standard deviation (SD), was calculated as the number of monogenean specimens collected in infected hosts divided by the number of fish infected, following Bush et al. (Reference Bush, Lafferty, Lotz and Shostak1997).
Table 1. Summary of the sampling data and biological parameters of the scorpaenoid fishes examined.

Abbreviations: N, number of individual fish examined; TL, total length (cm); SL, standard length (cm); W, weight (g).
Morphological analyses
Selected parasites were stained with iron-acetocarmine, dehydrated through an ethanol series (70–100%), cleared with dimethyl phthalate and examined as permanent mounts in Canada balsam under a Nikon Optiphot-2 light microscope (Nikon Instruments, Tokyo, Japan) with differential interference contrast at magnifications of 40–1000×.
Clamps, testes and spines were counted, and all morphometric measurements were taken from illustrations made with a drawing tube attached to the Nikon Optiphot-2 light microscope. The illustrations were digitalized to obtain 46 morphometric measurements (in micrometres, see table 2) using ImageJ v.1.48 software (Schneider et al., Reference Schneider, Rasband and Eliceiri2012). Morphometric data are reported as mean ± SD in the description, and as ranges in tables (see tables 2, 3). Clamp terminology follows Llewellyn (Reference Llewellyn1956) (see fig. 1). Following the suggestions of Víllora-Montero et al. (Reference Víllora-Montero, Pérez-del-Olmo, Georgieva, Raga and Montero2020) to differentiate Microcotyle species, the lengths of the anterior and posterior haptor lobes and the thickness of clamps presented by the ratio between ‘C’ sclerite maximum width/total clamp length are provided. A total of 59 specimens of Microcotyle spp. were selected and drawn: n = 40 ex H. dactylopterus (n = 20 from the Western Mediterranean Sea and n = 20 from the north-east Atlantic) and n = 19 ex S. scrofa from the north-east Atlantic (no microcotylids were found in Mediterranean samples). No infected S. notata and S. porcus were found. Type-specimens were deposited in the Collection of the Natural History Museum (NHMUK), London, UK.

Fig. 1. Schematic representations for measurements of microcotylid clamps and haptors: (a) clamp measurements ‘A’, ‘C’, ‘D’ and ‘E’, microcotylid sclerites according to Llewellyn (Reference Llewellyn1956); (b) and (c) unmounted specimens of Microcotyle sp., modified from fig. 1b in Víllora-Montero et al. (Reference Víllora-Montero, Pérez-del-Olmo, Georgieva, Raga and Montero2020)* in three-dimensional (3D) ventrolateral views, (b) corresponds to the most habitual Microcotyle spp. haptor morphology, ‘inverted T-shaped’ (such as Microcotyle merche n. sp.) and (c) to ‘L-shaped’ haptor (such as Microcotyle algeriensis); and (d) body outlines of ‘inverted T-shape’ and ‘L-shape’ specimens of Microcotyle spp. in two-dimensional (up) and 3D (down) lateral views. Abbreviations: ahl, anterior haptor lobe length; cl, clamp length; cw, clamp width; csw, ‘C’ sclerite width; dfr, dorsal frenulum; hl, haptor length; n-ahl, haptor length without anterior lobe; and vfr, ventral frenulum. *Note that the ‘anterior haptor lobe’ (ahl) is measured from the body–haptor junction to the anterior tip, and therefore ‘the posterior haptor lobe length’ (phl) in Víllora-Montero et al. (Reference Víllora-Montero, Pérez-del-Olmo, Georgieva, Raga and Montero2020) really corresponded to the length of the rest of the haptor (the haptor length without anterior lobe, ‘n-ahl’ in this figure).
Table 2. Metrical ranges for Microcotyle merche n. sp. from Helicolenus dactylopterus and Microcotyle algeriensis from Scorpaena spp. Records from present and other studies.

Table 3. Metrical data from descriptions of Microcotyle spp. similar to Microcotyle merche n. sp. from scorpenoid fishes.

a Diameter.
b Length of the longest spine as indicated Bonham & Guberlet, Reference Bonham and Guberlet1937.
Molecular methods
Genomic DNA isolation from the middle part of the body of the specimens was performed with Chelex™100 Resin (BIO-RAD, Hercules, USA) following the protocol described in Lablack et al. (Reference Lablack, Rima, Georgieva, Marzoug and Kostadinova2022). Partial fragments of the mitochondrial cytochrome c oxidase subunit 1 gene (cox1) were amplified using the primers JB3 (=COI-ASmit1) (forward: 5′ -TTT TTT GGG CAT CCT GAG GTT TAT-3′) and JB4.5 (= ASmit2) (reverse: 5′-AAA GAA AGA ACA TAA TGA AAA TG-3′) (Bowles et al., Reference Bowles, Blair and McManus1995). The thermocycling profile consisted of an initial denaturation step at 94°C for 5 min, followed by 40 cycles of amplification (92°C for 30 s, 45.5°C for 45 s, and 72°C for 90 s) and a final extension step at 72°C for 10 min. Polymerase chain reaction (PCR) amplicons were purified using the QIAquick TM PCR Purification Kit (Qiagen Ltd., Hilden, Germany) following the manufacturer's instructions and both strands were sequenced directly using the PCR primers. Sequencing was carried out at Macrogen Europe Inc. (Amsterdam, the Netherlands).
Contiguous sequences were assembled using MEGA v.6 (Tamura et al., Reference Tamura, Stecher, Peterson, Filipski and Kumar2013) and alignments were constructed using MAFFT v.7 (Katoh & Standley, Reference Katoh and Standley2013) under default gap parameters on the European Molecular Biology Laboratory–European Bioinformatics Institute bioinformatics web platform (http://www.ebi.ac.uk/Tools/msa/mafft) together with the currently available sequences for the Microcotyle species in the GenBank database (accessed on 25 June 2022; see table 4). The cox1 alignment (396 nt) included a total of seven newly generated sequences and 17 published sequences from the ten species available on GenBank. Bivagina pagrosomi (Murray, 1931) Dillon & Hargis, 1965 ex Sparus aurata L. was used as an outgroup in analyses (Z83003) based on previous phylogenies of the group using this species as the outgroup (Ayadi et al., Reference Ayadi, Gey, Justine and Tazerouti2017; Nam et al., Reference Nam, Whang and Kim2020; Víllora-Montero et al., Reference Víllora-Montero, Pérez-del-Olmo, Georgieva, Raga and Montero2020; Lablack et al., Reference Lablack, Rima, Georgieva, Marzoug and Kostadinova2022).
Table 4. Summary data for the isolates of Microcotyle spp. used in the phylogenetic analyses.

Abbreviations: NEA, north-east Atlantic; NWP, north-west Pacific; SA, southern Australia; SWP, south-west Pacific; WM, Western Mediterranean.
Note: The newly generated sequences are indicated in boldface type.
a As Sparus auratus in Littlewood et al. (Reference Littlewood, Rohde and Clough1997).
Model-based Bayesian inference (BI) and maximum likelihood (ML) analyses were carried out using MrBayes v.3.2.6 on XSEDE at the CIPRES Science Gateway v. 3.3 (http://www.phylo.org/sub_sections/portal) (Miller et al., Reference Miller, Pfeiffer and Schwartz2010) and PhyML v.3.0 (Guindon et al., Reference Guindon, Dufayard, Lefort, Anisimova, Hordijk and Gascuel2010) on the ATGC bioinformatics platform (http://www.atgc-montpelier.fr/). BI analyses were run using Markov chain Monte Carlo chain searches on two simultaneous runs of four chains for 107 generations, with trees sampled every 103 generations. The ‘burn-in’ was set for the first 25% of the trees sampled and consensus topology and nodal support was estimated from the remaining 75% of the trees. Posterior probabilities for the ML analyses were estimated with a nonparametric bootstrap validation of 1000 pseudoreplicates. Prior to both analyses the best-fitting models of nucleotide substitution were estimated under the Akaike information criterion using MEGA v 6. This was the Tamura–Nei model (Tamura et al., Reference Tamura, Stecher, Peterson, Filipski and Kumar2013) with gamma distributed among-site rate variation (TN93+ Г) for the ML, and the general time reversible model with gamma distributed among-site rate variation (GTR+ Г) for the BI analysis. FigTree v.1.4.3 (Rambaut & Drummond, Reference Rambaut and Drummond2012) was used to visualize tree topologies. Posterior probabilities (PP) and bootstrap support (BS) values are summarized on the BI trees (as PP/BS). Distance matrices (uncorrected p-distance model) were calculated in MEGA v.6. Distance-based neighbour-joining analyses based on Kimura 2-parameter distances were also performed in MEGA v.6 with nodal support estimated using 1000 bootstrap replicates.
Results
Molecular identification
Partial cox1 sequences were generated from specimens recovered in the two fish hosts collected from the Western Mediterranean and north-east Atlantic coasts of Spain. Partial cox1 (402–417 nt) sequences were generated for a total of seven isolates, that is, three M. algeriensis Ayadi, Gey, Justine & Tazerouti, 2016 ex S. scrofa collected from the north-east Atlantic and four M. merche n. sp. ex H. dactylopterus (two from the north-east Atlantic and two from the Western Mediterranean, see table 4). The newly generated cox1 sequences were analysed together with 16 published sequences for Microcotyle spp. using Bivagina pagrosomi (GenBank: Z83003; Littlewood et al., Reference Littlewood, Rohde and Clough1997) as an outgroup (table 4). The tree resulting from the BI analysis is provided in fig. 2 together with the statistical support from the ML analysis. The seven newly generated isolates clustered together with high support with the isolates of M. algeriensis ex S. notata (GenBank: KX926443; Ayadi et al., Reference Ayadi, Gey, Justine and Tazerouti2017) and Microcotyle sp. ex H. dactylopterus (GenBank: KX926447; Ayadi et al., Reference Ayadi, Gey, Justine and Tazerouti2017), reported from the southern coast of the Western Mediterranean off Algeria. The three isolates ex S. scrofa clustered together with the isolate of M. algeriensis ex S. notata (Ayadi et al., Reference Ayadi, Gey, Justine and Tazerouti2017), while the sequences for the four isolates of M. merche n. sp. ex H. dactylopterus from the north-east Atlantic and the Western Mediterranean clustered together with the published sequences for Microcotyle sp. from the same host species (Ayadi et al., Reference Ayadi, Gey, Justine and Tazerouti2017), albeit with poor support for both groupings. Overall, the cox1 phylogeny (fig. 2) recovered a well-supported group of species of Microcotyle collected from hosts of the family Sebastidae: Microcotyle caudata Goto, 1894 ex Sebastes inermis Cuvier, 1829; Microcotyle kasago Ono et al., Reference Ono, Matsumoto, Nitta and Kamio2020 ex Sebastiscus marmoratus (Cuvier, 1829); and M. sebastis Goto, 1894 ex Se. schlegelii Hilgendorf, 1880. Although with poor support, this group was also clustered together with the group formed by the newly generated sequences of M. algeriensis ex S. notata and Microcotyle sp. ex H. dactylopterus.

Fig. 2. Bayesian inference (BI) phylogram based on the mitochondrial cytochrome c oxidase subunit 1 gene dataset for Microcotyle spp. Bivagina pagrosomi was used as an outgroup. Posterior probabilities and bootstrap support values are shown as nodal support; only values > 0.90 (BI) and 75% (maximum likelihood). The scale-bar indicates the expected number of substitutions per site. Sequence identification is as in GenBank, followed by a letter: A, Ayadi et al. (Reference Ayadi, Gey, Justine and Tazerouti2017); B, Bouguerche et al. (Reference Bouguerche, Gey, Justine and Tazerouti2019a); K, Ono et al. (Reference Ono, Matsumoto, Nitta and Kamio2020); L, Littlewood et al. (Reference Littlewood, Rohde and Clough1997); N, Nam et al. (Reference Nam, Whang and Kim2020); O, Oliva et al. (Reference Oliva, Sepulveda and González2014); S, Song et al. (Reference Song, Kim and Choi2021); and V, Víllora-Montero et al. (Reference Víllora-Montero, Pérez-del-Olmo, Georgieva, Raga and Montero2020). Geographical abbreviations: NEA, north-east Atlantic; and WM, Western Mediterranean.
The intraspecific sequence divergence (see OnlineSupplementary Table S1) between the newly generated cox1 sequences for M. algeriensis (ex S. scrofa) was 0.2% (1 nt difference), while the two sequences for M. merche n. sp. from the north-east Atlantic and the Western Mediterranean were identical. The newly generated sequences for the isolates of M. algeriensis ex S. scrofa from the north-east Atlantic differed by 3.8–4.0% (15–16 nt) from M. algeriensis ex S. notata from off of Algeria; the sequences for M. merche n. sp. ex H. dactylopterus differed by 4.5–5.3% (18–21 nt) from the published isolate of M. algeriensis ex S. notata from off of Algeria. The newly generated isolates of M. merche n. sp. ex H. dactylopterus differed from Microcotyle sp. ex H. dactylopterus from off of Algeria by 0.8–2.5% (3–10 nt) and by 2.8–3.8% (11–16 nt) from M. algeriensis ex S. scrofa from the north-east Atlantic. The newly generated isolates of M. merche n. sp. ex H. dactylopterus from the north-east Atlantic differed from the Western Mediterranean by 2.3% (9 nt difference). The overall interspecific sequence divergence for the genus Microcotyle ranged between 8.1–16.4% (31–65 nt difference).
Taxonomic summary
Family: Microcotylidae Taschenberg, 1879
Subfamily: Microcotylinae Taschenberg, 1879
Microcotyle merche n. sp. ex Helicolenus dactylopterus
Type host: Blackbelly rosefish, H. dactylopterus (Teleostei: Sebastidae) [weight: 27.7–338.9 (94.2 ± 72.5); standard length: 10.5–19.5 (14.0 ± 2.6)] off Guardamar del Segura, Spain; [weight: 327.0–507.5 (403.6 ± 52.8); standard length: 22.4–26.5 (25.0 ± 1.0)] in Bay of Biscay, Spain.
Type locality: Off Guardamar del Segura, Western Mediterranean off Spain. Other localities with valid records: Bay of Biscay off Spain; off Bouharoun, Algeria; and off Montenegro.
Site of infection: Gill filaments.
Infection parameters: Off Guardamar del Segura, Spain (n = 74): prevalence, 24% (18 out of 74); mean intensity, 2.17 ± 1.95; Bay of Biscay off Spain (n = 33): prevalence, 52% (17 out of 33); mean intensity, 1.76 ± 1.20.
Deposition of specimens: Holotype (2023.2.2.1) and three paratypes (2023.2.2.2–2023.2.2.4) (from Guardamar del Segura, and from Guardamar del Segura and the Bay of Biscay, respectively) deposited at the NHMUK; remaining material from Guardamar del Segura and Bay of Biscay deposited in the parasitological collection of the Cavanilles Institute of Biodiversity and Evolutionary Biology, University of Valencia, Spain.
Molecular sequence data: GenBank accession numbers: OQ243284–OQ243287 (cox1).
ZooBank registration: urn:lsid:zoobank.org:act:40CFA7F5-4465-4FAD-993F-C39FC2A95649
Comparative specimens examined: Five vouchers ex H. dactylopterus from Bouharoun, Algeria, deposited at the Muséum National d'Histoire Naturelle, Paris (MNHN HEL579 A–E).
Etymology: The new species is named in apposition, in honour of the Dr Mercedes Fernández (‘Merche’), from the University of Valencia, an eminent biologist, and an expert in aquatic animal parasitology. Mercedes Fernández has taught and inspired several generations of biologists and parasitologists thanks to her talent and selfless dedication.
Description
Based on 40 mature adults, (20 ex H. dactylopterus from the Western Mediterranean and 20 from the north-east Atlantic), except when otherwise indicated. Morphometric data refer to specimens from both localities together; ranges (when more than one specimen was measured) are provided in table 2, including measurements from specimens from each locality separately; see fig. 3.

Fig. 3. Microcotyle merche n. sp. from Helicolenus dactylopterus (Delaroche, 1809) from Guardamar del Segura, Spain. All drawings from the holotype: (a) whole mount; (b) anterior body end; (c) clamp; (d) genital atrium, including copulatory organ; (e) germarium; and (f) egg. Scale bars: (a) 500 μm; (b), (d)–(f) 100 μm; (c) 50 μm.
Body fusiform, elongate, occasionally slender, 3963.4 ± 740.3 long and 173.0 ± 39.4 wide at level of suckers, 314.3 ± 68.5 wide at level of genital atrium, 612.8 ± 159.4 wide at level of germarium (maximum width), and 449.4 ± 125.0 wide at level of testes.
Haptor elongated, spatulate, pointed at anterior and posterior extremities, well differentiated from body (haptor length/total length ratio 20–37% (30%)), peduncle inconspicuous; laterally symmetrical, divided into an anterior (ventral) lobe and a longer posterior (dorsal) lobe (anterior/posterior haptoral lobe length ratio 10–38% (19%) (n = 30)) (see figs 1b and 3). A ventral frenulum connects the anterior haptor lobe with the body ventral surface and a small dorsal frenulum connects the posterior lobe with the body dorsal surface (fig. 1b). Haptoral clamps in two laterals frills, sessile or slightly protruded, in two rows (42–60 in total number; 8–14 in the anterior haptor projection). Clamps ‘microcotylid’ type, relative robust (‘C’ sclerite maximum width 5.3 ± 1.0 and 0.105 ± 0.021 corrected by clamp length (n = 54)); trident-shaped accessory sclerite (‘E’) with long thick central bar reaching to distal tips of the antero-lateral sclerites ‘C’ and two delicate lateral branches originating at the base of ‘E’. Clamps at the anterior and posterior ends of the haptor slightly smaller.
Mouth subventral within subconical vestibule with a pair of septate buccal suckers ventrally oriented. Pharynx muscular, subspherical; oesophagus short, connected to intestinal bifurcation at the end or posterior to the genital atrium. Caeca mostly pre-haptoral, ramified with the vitellaria in inner and external lateral branches (external more profuse).
Testes flattened, irregular, numerous (13–22 in number), arranged in clusters of 1–2 rows, and some testes piled up dorsally or ventrally; testicular field at 2092.2 ± 503.5 from anterior extremity of the body, post-germarial and pre-haptoral. Testes area ending up to 549.5 ± 150.1 from the insertion of the haptor. Vas deferens wide, straight, dorsal to the uterus, ending in a short muscular copulatory organ (55.8 ± 13.5 × 50.7 ± 13.0 (n = 11)) posterior to genital atrium. Genital atrium at 262.5 ± 108.8 from anterior end, formed by a wide medial muscular chamber, armed with small conical spines (146–221 in number), followed by two posterior small lateral chambers (‘pockets’ sensu Mamaev, Reference Mamaev and Lebedev1989) one on each side of the copulatory organ (41.2–85.3, n = 10). Pockets armed with conical spines (23–40 in number, 24 in holotype), longer than the ones in the main chamber (see table 2).
Germarium at 1770.8 ± 320.4 (n = 34) from anterior end; 884.3 ± 239.7 long (n = 25), question mark-shaped; proximal globular germinal area 134.1 ± 45.8 × 113.7 ± 32.6 (n = 31) followed by a straight section 351.5 ± 113.4 (n = 31), widening in a long distal globular region 532.8 ± 147.4 long (n = 25) with a proximal arched dextro-sinistral section, connected to a wider distal arch directed sinistro-dextrally, with a maximum distal width of 88.5 ± 24.0 (n = 27). Oviduct slightly sinuous, including an elongated seminal receptacle 244.7 ± 178.4 × 211.6 ± 187.0 (n = 9) directed postero-sinistrally; ending in an oötype, with Mehlis’ gland. Uterus ascending straight with curving walls, ending in genital atrium.
Vaginal pore unarmed, mid-dorsal, often unobserved, at 1087.7 ± 426.6 from anterior end (n = 8). Vitelline follicles disperse, posterior or overlapping with genital atrium, starting at 481.4 ± 127.0 from anterior body extremity, extended in lateral fields surrounding caecal ramifications and surrounding the testes, usually pre-haptoral (n = 33). Posterior fields of vitellaria mostly joined (n = 38), with one single specimen with different field lengths (204.8). Vitelline ducts Y-shaped, with two unjoined ducts 300.6 ± 174.0 and 366.3 ± 145.4 long (right and left, respectively) (n = 26), joining ventral to the germarium in a slightly sinuous deferent duct, 240.8 ± 78.3 long (n = 26).
Egg fusiform; opercular filament long and thin and abopercular filament short and thin. Operculum with hollow tip, abruptly narrowed into a tubular section continuing into a solid opercular filament. Filaments’ tips solid and either not capitated or slightly capitated.
Remarks
Five species of Microcotyle are known to parasitize sebastids (M. caudata (syn. Microcotyle sebastisci Yamaguti, 1958), M. kasago, Microcotyle neozealanicus Dillon & Hargis, 1965, M. sebastis and Microcotyle victoriae Woolcock, 1936), and all of them have been described from Pacific fishes, for example, M. caudata, M. neozealanicus and M. victoriae in Helicolenus spp. Additionally, Balboa & Nascimento (Reference Balboa and George-Nascimento1998) reported Microcotyle sp. ex Helicolenus lengerichi Norman, 1937 (and Sebastes capensis (Gmelin, 1789)) off of Chile, although no morphological information was provided. According to the numbers of clamps and testes, M. merche n. sp. mostly resembles M. kasago from Seb. marmoratus and M. neozealanicus and M. victoriae, both from Helicolenus percoides (Richardson & Solander, 1842) (see table 3). These traits marginally overlap in M. merche n. sp. and M. kasago and overlap with M. neozealanicus and M. victoriae. Other diagnostic traits such as the number of spines of the genital atrium are not reported in these species’ descriptions; however, this region is graphically represented in all these descriptions. Counts conducted from original drawings reveal differences in the number of spines of the lateral small chambers of M. merche n. sp. (24–39), higher than those of M. kasago (14), M. neozealanicus (21) and M. victoriae (22).
Two records of Microcotyle sp. have been reported in Mediterranean H. dactylopterus: off of Montenegro (Radujković & Euzet, Reference Radujković and Euzet1989, as M. sebastis) and Algeria (Ayadi et al., Reference Ayadi, Gey, Justine and Tazerouti2017, as Microcotyle sp.). These exemplars must be considered as M. merche n. sp. as their traits fit with the present description (see table 2), therefore expanding the distribution of the new species to the north-central and south-western Mediterranean. The re-examination of the vouchers of Microcotyle sp. from Algerian H. dactylopterus also revealed no incongruence with the description of M. merche n. sp. Another species, M. algeriensis has been reported in other Mediterranean scorpaenoid fishes (Scorpaenidae): S. notata off Algeria (Ayadi et al., Reference Ayadi, Gey, Justine and Tazerouti2017); and S. scrofa from the Bay of Biscay (present study). Microcotyle algeriensis can be differentiated from the new species by the lower number of clamps (22–39 vs. 42−60 in M. merche n. sp.). Moreover, the haptor shape of M. algeriensis is characterized by its uniquely short anterior lobe (or absent, see remarks on M. algeriensis below and fig. 1).
The new species can also be distinguished from other Mediterranean species, many of them parasites of sparids (Microcotyle erythrini Van Beneden & Hesse, Reference Van Beneden and Hesse1863, Microcotyle isyebi Bouguerche, Gey, Justine & Tazerouti, 2019, Microcotyle visa Bouguerche, Gey, Justine & Tazerouti, 2019 and Microcotyle whittingtoni Víllora-Montero, Pérez-del-Olmo, Georgieva, Raga & Montero, 2020, see Online supplementary Table S3 in Víllora-Montero et al., Reference Víllora-Montero, Pérez-del-Olmo, Georgieva, Raga and Montero2020), but also in fishes from other families (Microcotyle donavini Van Beneden & Hesse, Reference Van Beneden and Hesse1863, Microcotyle lichiae Ariola, 1899 and Microcotyle pomatomi Goto, 1899, see supplementary table S4 in Víllora-Montero et al., Reference Víllora-Montero, Pérez-del-Olmo, Georgieva, Raga and Montero2020). The number of clamps of M. merche n. sp. (42–60) is smaller than those of these species except for M. lichiae (52); however, the body length of this latter species (8000) is much greater than the body length of this new species (2517–5706). Microcotyle isyebi and M. visa are similar to M. merche n. sp., and the number of clamps slightly overlaps (54–102 and 59–126, respectively). The ranges of number of clamps of these species required re-examination, as they are particularly wide, especially regarding the lower values; in fact, Víllora-Montero et al. (Reference Víllora-Montero, Pérez-del-Olmo, Georgieva, Raga and Montero2020) reported a much narrower range of clamps number in their description of M. isyebi from Spanish specimens (80–100), which does not overlap with that of the new species.
Taxonomic summary
M. algeriensis ex S. scrofa
Host: Red scorpionfish, S. scrofa (Teleostei: Scorpaenidae) (weight: 292.5–745.1 (542.6 ± 123.7); standard length: 23.5–29.3 (26.2 ± 2.0)) (new host), other hosts with valid records S. notata (Teleostei: Scorpaenidae) (type-host).
Locality: Bay of Biscay off Spain. Other localities with valid records: off Bouharoun, Algeria (type-locality).
Site of infection: Gill filaments.
Infection parameters: n = 19; prevalence, 58% (11 out of 19); mean intensity, 4.09 ± 2.61.
Deposition of specimens: Two specimens from Bay of Biscay off Spain are deposited in the NHMUK (2023.2.2.5–2023.2.2.6); remaining material from Bay of Biscay in the parasitological collection of the Cavanilles Institute of Biodiversity and Evolutionary Biology, University of Valencia, Spain.
Molecular sequence data: GenBank accession numbers: OQ243288–OQ243290 (cox1).
Description
Based on 19 mature adults; ranges (when more than one specimen was measured) are provided in table 2; fig. 4.
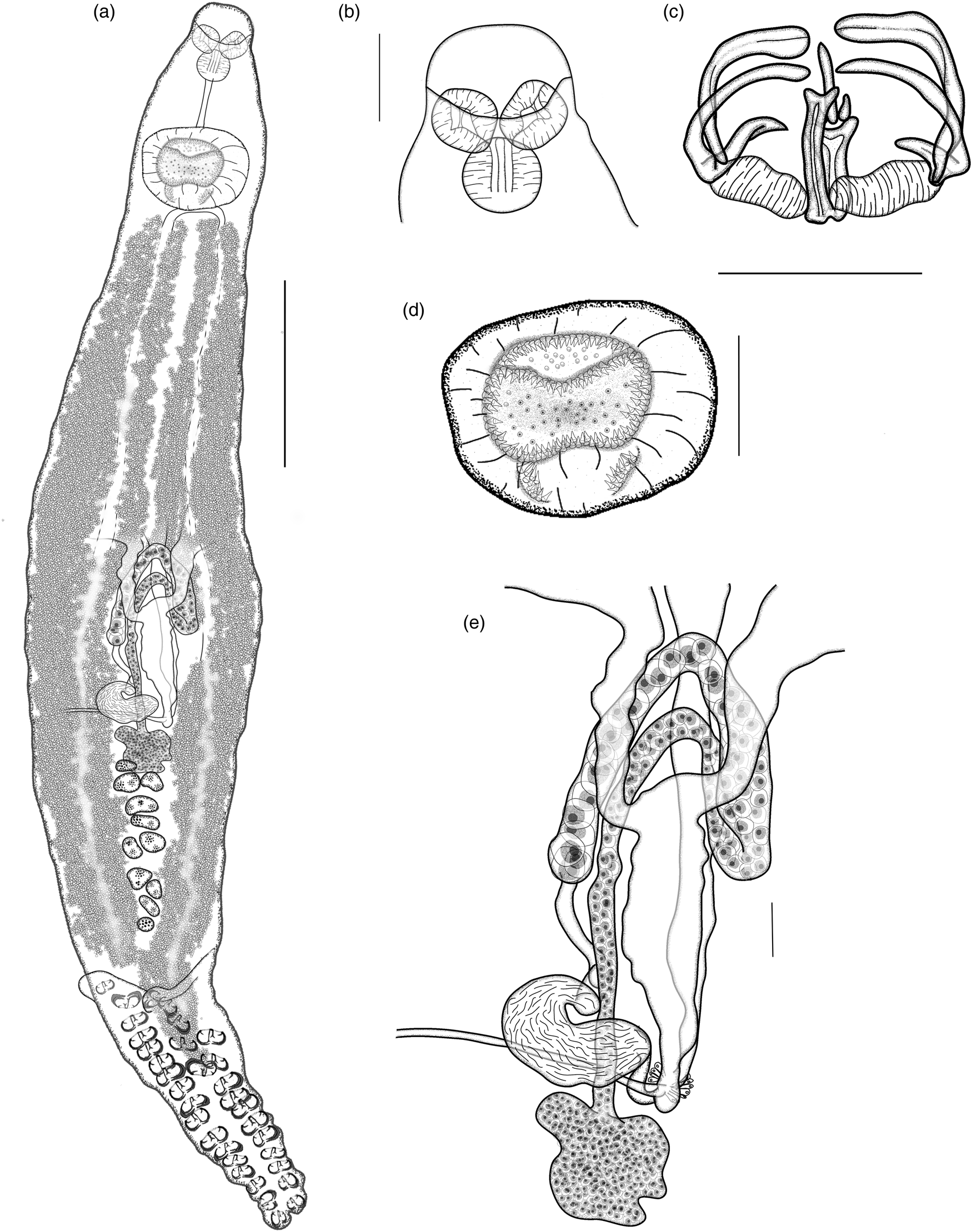
Fig. 4. Microcotyle algeriensis Ayadi, Gey, Justine & Tazerouti, 2016, from Scorpaena scrofa L. from Guardamar del Segura, Spain. All drawings from the same voucher specimen: (a) whole mount; (b) anterior body end; (c) clamp; (d) genital atrium; and (e) germarium. Scale bars: (a) 500 μm; (b), (d)–(f) 100 μm; (c) 50 μm.
Body fusiform and elongate, often slender, 3173.9 ± 633.2 long and 164.0 ± 15.9 wide at level of suckers, 292.8 ± 29.9 wide at level of genital atrium, 548.2 ± 99.1 wide at level of germarium (maximum width), and 410.2 ± 81.5 wide at level of testes.
Haptor flat and spindle-shaped, well differentiated from the body (haptor length/total length ratio 15–59% (27%)), peduncle not visible; laterally symmetrical, projected in a very short anterior (ventral) lobe (often not distinguishable) and a relatively longer posterior (dorsal) lobe (anterior/posterior haptoral lobe length ratio 0–9% (5%) (n = 16)). Two small frenula connect anterior and posterior haptor lobes with ventral and dorsal body surfaces, respectively (see fig. 1c). Haptor with two lateral frills armed each with one row of sessile or slightly protruded clamps (22–34 in total number; 0–4 in the anterior projection). Clamps ‘microcotylid’ type, robust (‘C’ sclerite maximum width 3.9 ± 0.9 and 0.092 ± 0.020 corrected by clamp length (n = 29)); trident-shaped accessory sclerite ‘E’ formed by long thick central bar reaching to distal tips of the antero-lateral sclerites ‘C’ and two lateral slender and short bars originating at the base of ‘E’. Clamps at the anterior and posterior ends of the haptor smaller.
Mouth ventro-subterminal, within a funnel-shaped vestibule including a pair of septate buccal suckers. Muscular pharynx subspherical, followed by a short oesophagus; intestine bifurcation at the end of the genital atrium. Caeca usually not extending within the haptor, with inner and external lateral ramifications accompanying vitellaria; external branches more profuse.
Testes flattened and irregular (7–10 in number), arranged in 1–2 rows, with some testes piled up; testicular field at 1872.9 ± 192.6 (n = 16) from anterior extremity of the body; testes post-germarial and pre-haptoral. Testicular area ends up to 525.3 ± 254.6 (n = 17) from the insertion of the haptor. Vas deferens wide, unwinding, dorsal to uterus; muscular copulatory organ short (45.8 ± 8.1 × 52.9 ± 5.1 (n = 3)), protruded at the posterior part of the genital atrium. Genital atrium at 300.1 ± 28.9 from anterior extremity of the body, formed by a main wide medial armed muscular chamber, armed with small conical spines (189–234 in number), connected to two small postero-lateral chambers (pockets), at either side of the copulatory organ (39.6–55.0, n = 3). Pockets with conical spines (20–38 in number), longer than the main chamber spines (see table 2).
Germarium 743.1 ± 119.8 long (n = 15), at 1795.4 ± 179.6 (n = 15) from anterior end; question mark-shaped with proximal globular germinal area 113.9 ± 22.4 × 110.2 ± 38.0 (n = 14) connected to a straight and narrow section 306.6 ± 77.0 (n = 31), widening into a tubular region 436.5 ± 72.7 long (n = 14) with a proximal arched dextro-sinistral section, connected to a wider distal arch directed sinistro-dextrally, with a maximum distal width of 67.6 ± 15.0 (n = 14). Oviduct, directed postero-sinistrally, with an elongated oviducal seminal receptacle 76.3 ± 30.7 × 110.1 ± 47.6 (n = 5) joined to the oötype by a sinuous narrow section. Oötype with Mehlis’ gland well developed. Uterus wide, ascending straight up to the genital atrium.
Vaginal pore unarmed, medial, dorsal, observed in one specimen at 238.6 from anterior extremity of the body. Vitelline follicles disperse, posterior or overlapping with genital atrium, spread from 495.3 ± 41.8 from anterior extremity of the body, extended in lateral fields together with the caeca and surrounding the testes, on occasion pre-haptoral (n = 17). Posterior fields of vitellaria always joined (n = 17). Vitelline ducts Y-shaped, with two unjoined ducts 327.5 ± 118.0 and 345.2 ± 121.6 long (right and left, respectively) (n = 11), joining ventral to germarium in a slightly sinuous deferent duct, 227.0 ± 39.8 long (n = 11). No eggs observed.
Remarks
The specimens of M. algeriensis ex S. scrofa from the Spanish Atlantic share the same morphological and molecular traits of the specimens originally described ex S. notata off Algeria (Ayadi et al., Reference Ayadi, Gey, Justine and Tazerouti2017). This species has not been found in S. notata from the Spanish Mediterranean (neither in S. porcus from Spain). Based on the new specimens analysed in this study, some comments on relevant diagnostic characters regarding the haptor of M. algeriensis must be pointed out. According to the original description the haptor is ‘continuous with body’, however, as in other Microcotyle species, the body is inserted perpendicularly to a haptor projected into two lobes, one ventral and one dorsal (see fig. 1c). A distinctive trait of M. algeriensis, previously unreported in other Microcotyle species, is the short or almost absent ventral lobe (this trait also differentiates M. merche n. sp. from M. algeriensis, see schematic comparative drawings in fig. 1b, c). Subsequently the haptor is slightly short and the number of clamps of this species is inferior to other Microcotyle spp. (see tables 2 and 3). Another minor comment regards the number of spines of the main chamber of the genital atrium, slightly higher in the specimens’ ex S. scrofa than in the species description. Unfortunately, type specimens are not available, and a recount of the genital atrium spines was not possible.
Discussion
This study increases the knowledge about the diversity of species of Microcotyle within the Scorpaenidei, specifically in scorpaenids and sebastids. Interestingly, M. algeriensis and M. merche n. sp. are not only geographically, but also phylogenetically close. This finding is particularly interesting as several authors consider that sebastids should be included in a subfamily (Sebastinae) within the Scorpaenidae (Jia et al., Reference Jia, Zhang, Xu, Yang, Yanagimoto and Gao2020). In fact, the genetic distance between M. algeriensis and M. merche n. sp. is very narrow, although the prominently distinct morphology (including key diagnostic traits as clamp number, haptor dimensions or genital atrium spines) and the phylogenetic tree clearly differentiate both species. In contrast, other more genetically distant species, such as M. caudata, are highly difficult to differentiate morphologically from these species (particularly from M. merche n. sp.).
The description of M. merche n. sp. contributes to the delimitation of the host and geographical distribution of M. sebastis, a species which has mostly reported in sebastids (in up to 40 species of Sebastes), but there are also several doubtful reports worldwide in species of non-sebastid fishes such as aulorhynchids, paralichthyids and syngnathids (Gibson et al., Reference Gibson, Bray and Harris2006). According to the present study the specimens of M. sebastis reported by Radujković & Euzet (Reference Radujković and Euzet1989) in Mediterranean H. dactylopterus are in fact M. merche n. sp., thus meaning that M. sebastis is not present in this sea. Furthermore, the high number of historical reports indicate that M. sebastis is probably specific to species of Sebastes Cuvier, 1829 from the Pacific Ocean, and that other host and locality reports should be re-examined (Gibson et al., Reference Gibson, Bray and Harris2006). This work also expands the reported host range and geographical distribution of M. algeriensis. Interestingly, neither this species nor any other microcotylid species has been found in the other Scorpaena species analysed in this study (the type host S. notata and S. porcus), despite the fact that these fishes are sympatric and the localities are relatively close to Algeria. These local differences can be explained by the high sedentarism of the species of the Scorpaena genus (Hureau & Litvinenko, Reference Hureau, Litvinenko, Whitehead, Bauchot, Hureau, Nielsen and Tortonese1986). In S. porcus from Italy, Ulmer & James (Reference Ulmer and James1981) found M. cf. sebastisci, which according to Ono et al. (Reference Ono, Matsumoto, Nitta and Kamio2020) was a possible synonym of M. caudata due to misidentification; however, based on the similarities in host genus, distribution and morphology, these specimens could also be M. algeriensis.
As indicated by Víllora-Montero et al. (Reference Víllora-Montero, Pérez-del-Olmo, Georgieva, Raga and Montero2020) the haptors of the species of Microcotyle are arranged in a three-dimensional pattern, usually undescribed or wrongly interpreted based on the two-dimensional way of mounting the specimens. Figure 1 in Víllora-Montero et al. (Reference Víllora-Montero, Pérez-del-Olmo, Georgieva, Raga and Montero2020) describes the haptor morphology in three-dimensions, including the parts to be measured in order to compare species. With the aim of standardizing the way of measuring the haptor lobes and avoiding the overlap of the body and haptor in mounted specimens, a modified version of the figure is herein included (fig. 1b, c); both dorsal and ventral lobes of the haptor are herein measured from their tips to their connection to the body, not from their tips to the centre of the insertion of the body, as this point is often difficult to identify. These standardized measures are useful to describe objectively (numerically) the short ventral lobe of M. algeriensis (table 2). The arrangement of the haptor of this species is unique (previously undescribed so far), as the ventral lobe is almost indistinct or absent, giving the worm an ‘L-shape’ in lateral view, instead of an ‘inverted T-shape’ (fig. 1d), with anterior and posterior projections perpendicular to the body. This trait could exist in other Microcotyle species, but it could have gone unseen, especially in mounted individuals, where the anterior part of the haptor usually overlaps with the body. Microcotyle merche n. sp. shows the habitual arrangement of the haptor with distinct ventral and dorsal projection (‘inverted T-shape’, fig. 1d) as seen in other Microcotyle species (see also Víllora-Montero et al., Reference Víllora-Montero, Pérez-del-Olmo, Georgieva, Raga and Montero2020). Haptor shape is not only relevant taxonomically, but also functionally, as the ‘L-shape’ haptor lacks the extra ventral clamps of the anterior projection lobe. These anterior clamps would contribute to parasite attachment, not only increasing the number of anchoring points, but also improving the antero-posterior balance, something crucial in this type of gill monogeneans, ectoparasites that can stretch their elongated bodies to reach distant parts of the branchial region (Buchmann & Bresciani, Reference Buchmann, Bresciani and Woo2006).
Eleven species of Scorpaenidae and Sebastidae exist in the Mediterranean ( World Register of Marine Species, 2022), six of them Scorpaena spp., and specimens of four of them have been analysed to date (Radujković & Euzet, Reference Radujković and Euzet1989; Ayadi et al., Reference Ayadi, Gey, Justine and Tazerouti2017; present study), showing very interesting differences in the diversity of Microcotyle spp. of hosts from relatively close geographical areas of this sea. Knowing more about the diversity of Microcotyle spp. in the Mediterranean ecosystem can provide not only relevant information on the taxonomy and phylogeny of Microcotyle spp. within scorpaenoids, but also on these hosts’ distribution and biology.
Supplementary material
To view supplementary material for this article, please visit http://doi.org/10.1017/S0022149X23000019.
Acknowledgements
The authors thank Dr David I. Gibson (Natural History Museum, UK) for his generous advice on taxonomy and nomenclature and Dr Jean-Lou Justine (Muséum National d'Histoire Naturelle, France) for his generous comments and the loan of specimens. We are indebted to Rachel V. Pool (University of Valencia) for revising the English and to the anonymous reviewers for their helpful comments and suggestions.
Financial support
This work was supported by the Spanish Government [project MINECO/FEDER PID2019-110730RB-I00] co-funded by the Spanish Ministry for Science and Innovation (MCIN) MCIN/AEI/10.13039/501100011033 by ‘ERDF A way of making Europe’ by the European Union (EU), the Valencian Regional Government [project AICO/2021/279] and the ThinkInAzul programme supported by the MCIN with funding from the EU NextGenerationEU (PRTR-C17.I1), and by Generalitat Valenciana (THINKINAZUL/2021/029).
Conflicts of interest
None.
Ethical standards
Parasitological samples used in this study were collected from dead fish caught in the wild, that the fish species analyzed are not included in Royal Decree 139/2011 (BOE 2011), nor in the BOE 2020 of “Wild Species under Protection Regime Special and of the Spanish Catalog of Threatened Species”, and that no additional permits are required in Spain to collect these organisms as they were originally destined for human consumption.