Introduction
It is known that dogs transmit over 60 zoonotic diseases that can affect humans (Macpherson et al. Reference Macpherson, Torgerson, CNL, FX and AI2013). Some of the most important helminths transmitted from dogs to human beings include roundworms Toxocara spp., hookworms (Uncinaria stenocephala and Ancylostoma caninum) and Echinococcus tapeworms (e.g., Pullola et al., Reference Pullola, Vierimaa, Saari, Virtala, Nikander and Sukura2006; Martínez-Carrasco et al., Reference Martínez-Carrasco, Berriatua, Garijo, Martínez, Alonso and Ruiz de Ybáñez2007; Laurimaa et al., Reference Laurimaa, Davison and Süld2015a; Baneth et al., Reference Baneth, Thamsborg, Otranto, Guillot, Blaga, Deplazes and Solano-Gallego2016). Humans can be final, intermediate, paratenic or accidental hosts by ingesting eggs or infective stages from the contaminated environment (plants, soil, water or scats) or by consuming raw or undercooked meat containing infective stages of parasites. Moreover, some geohelminth (Toxocara spp.) infective stages can be transmitted directly through animal–human contact and in some cases infective larvae can penetrate the skin (Ancylostomatidae). Children are among the most vulnerable to helminth infections. They may come into contact with animal scats on potential endoparasite infection hotspots such as recreational zones including parks, playgrounds and other green areas near schools and nurseries (Talvik et al., Reference Talvik, Moks, Mägi, Järvis and Miller2006; Tull et al., Reference Tull, Moks, Laurimaa, Keis and Süld2020).
Regardless of the efficient anthelmintics available and the control measures taken by owners and veterinarians, gastrointestinal parasites, especially helminth infections are still abundant in dogs (Tylkowska et al., Reference Tylkowska, Pilarczyk, Gregorczyk and Templin2010; Kostopoulou et al., Reference Kostopoulou, Claerebout, Arvanitis, Ligda, Voutzourakis, Casaert and Sotiraki2017; Roussel et al., Reference Roussel, Drake and Ariza2019; Strube et al., Reference Strube, Neubert, Springer and von Samson-Himmelstjerna2019). Free-ranging dogs in urban and rural areas can be an important source of helminth infection (Laurimaa et al., Reference Laurimaa, Davison and Süld2015a; Knapp et al., Reference Knapp, Giraudoux and Combes2018; Jarošová et al., Reference Jarošová, Antolová, Lukáč and Maďari2021). They may come into contact with carcasses or scats of red fox (Vulpes vulpes), raccoon dog (Nyctereutes procyonoides), European badger (Meles meles) and other wild animals infected with helminths of zoonotic potential, facilitating helminth transmission from wildlife to humans. Also, feeding dogs with offal or raw viscera of hunted or domesticated animals (e.g., sheep and cattle) promotes the shift from the sylvatic cycle of helminths such as Echinococcus granulosus sensu lato (s.l.) and other taeniids to the synantropic cycle (Marcinkutė et al., Reference Marcinkutė, Šarkūnas and Moks2015; Baneth et al., Reference Baneth, Thamsborg, Otranto, Guillot, Blaga, Deplazes and Solano-Gallego2016).
In Europe, helminth prevalence of dogs in rural and urban areas varies, among the studied countries being largest (63.5%) in rural areas of Spain (Regidor-Cerrillo et al., Reference Regidor-Cerrillo, Arranz-Solís, Moreno-Gonzalo, Pedraza-Díaz, Gomez-Bautista, Ortega-Mora and Collantes-Fernandez2020), whereas in rural and urban areas of the Czech Republic helminth prevalence of dogs has been estimated at 41.7% and 14.3%, respectively (Dubná et al., Reference Dubná, Langrová, Nápravník, Jankovská, Vadlejch, Pekár and Fechtner2007). In Hungary and Portugal, the helminth prevalence of dogs in rural areas is rather similar, 56.3% and 58.8%, respectively (Fok et al., Reference Fok, Szatmári, Busák and Rozgonyi2001; Cardoso et al., Reference Cardoso, Costa, Figueiredo, Castro and Conceição2014). Fok et al. (Reference Fok, Szatmári, Busák and Rozgonyi2001) demonstrated that 30.1% of rural dogs were infected with Toxocara canis causing toxocariasis in humans. The zoonotic Uncinaria sp. and Ancylostoma sp. have high prevalence in rural areas of Portugal (40.9%; Cardoso et al., Reference Cardoso, Costa, Figueiredo, Castro and Conceição2014) and Spain (35.6%; Regidor-Cerrillo et al., Reference Regidor-Cerrillo, Arranz-Solís, Moreno-Gonzalo, Pedraza-Díaz, Gomez-Bautista, Ortega-Mora and Collantes-Fernandez2020). These studies refer to major contamination of the environment with zoonotic helminths in rural areas. In Estonia, there is a large knowledge gap considering helminths of rural dogs.
As it is often difficult to reliably separate dog scats from those of other canids based on morphology, and genetic approaches are needed for correct species identification. In Estonia, the other canids are red fox, grey wolf (Canis lupus), raccoon dog and golden jackal (Canis aureus). Studies have confirmed that faecal DNA analysis provides more accurate and informative results than scat-based morphological studies (Davison et al., Reference Davison, Birks, Brookes, Braithwaite and Messenger2002; Janečka et al., Reference Janečka, Jackson, Yuquang, Diqiang, Munkhtsog, Buckley-Beason and Murphy2008; Rosellini et al., Reference Rosellini, Osorio, Ruiz-González, Piñeiro and Barja2008; Monterroso et al., Reference Monterroso, Castro, Silva, Ferreras, Godinho and Alves2013; Laurimaa et al., Reference Laurimaa, Davison and Plumer2015b; Mumma et al., Reference Mumma, Adams, Zieminski, Fuller, Mahoney and Waits2016; Oja et al., Reference Oja, Soe, Valdmann and Saarma2017; Valdmann & Saarma, Reference Valdmann and Saarma2020). Therefore, for reliable identification of dog scats, genetic analysis was applied. The aims of the present work were to determine helminth infections among dogs in rural areas of Western Estonia and to compare these findings with a previous endoparasite study focusing on dog helminths in urban areas of Estonia (Tull et al., Reference Tull, Moks, Laurimaa, Keis and Süld2020). In addition, the effect of diet on the risk of infection of rural dogs with various helminths was studied.
Materials and methods
Study area and samples
A non-probabilistic sampling was used which involved collecting available scats of canids during fieldwork. Faecal samples (N = 328) were collected from rural areas (local small roads near settlements) in Western Estonia, mainly in Matsalu National Park, in April–June 2019 (fig. 1). Samples were placed into a separate plastic bag and tagged with unique ID, including coordinates. To inactivate eggs of zoonotic parasites, for example Echinococcus multilocularis and E. granulosus s.l., which are endemic in Estonia (Moks et al., Reference Moks, Jõgisalu, Saarma, Talvik, Järvis and Valdmann2006, Reference Moks, Jõgisalu, Valdmann and Saarma2008; Laurimaa et al., Reference Laurimaa, Davison and Süld2015a, Reference Laurimaa, Davison and Plumerb), samples were kept at −80°C for a minimum of seven days. In order to compare the infection prevalence between rural and urban dogs, data of 657 urban dogs were included from a previous study carried out in five Estonian towns (Tull et al., Reference Tull, Moks, Laurimaa, Keis and Süld2020) and pooled together with rural dogs for further analyses.

Fig. 1. Sampling sites for faecal samples of rural dogs in Western Estonia, in Matsalu National Park (N = 68), Hiiumaa (N = 3) and Häädemeeste (N = 13). The buffer distance around scat samples (the average straying area of free-ranging dogs) is marked with light purple colour. Base map: Estonian Land Board, 2021.
Molecular identification of dogs
Scats of different canid species are sometimes difficult to distinguish and to avoid mixing the data of various species, we conducted a genetic analysis to identify dog samples. Genomic DNA was isolated from scats using the QIAamp Fast DNA Stool Mini Kit (Qiagen, Hilden, Germany) according to the manufacturer's protocol. A hypervariable fragment of the mitochondrial DNA (mtDNA) control region that enables to distinguish between wolves and dogs in Estonia, was polymerase chain reaction (PCR)-amplified and sequenced as described in Plumer et al. (Reference Plumer, Talvi, Männil and Saarma2018). In brief, a 351 base-pair (bp) fragment of the mtDNA control region was PCR-amplified using 0.25 pmol of primers Canis1F and Canis3R. The reaction mixture (20 μl in total), contained 2 μl of DNA, 4 μl of 5× Phusion HF buffer, 0.4 mm deoxynucleoside triphosphate (dNTP) and 0.2 μl Phusion HS II polymerase (Thermo Fisher Scientific, Waltham, USA). The following PCR cycling parameters were used: 30 s at 98°C, then 10 cycles: 10 s at 98°C, 30 s at 68°C (with touchdown of −0.8°C per cycle), 45 s at 72°C; then 35 cycles: 10 s at 98°C, 30 s at 60°C, 45 s at 72°C, and finally 2 min at 72°C. PCR products were purified with 1 U of both FastAP and ExoI (Thermo Fisher Scientific). Purified PCR products were sent for sequencing to the core laboratory of the Institute of Genomics at the University of Tartu.
Sequences of both DNA chains were aligned with CodonCode Aligner v.5.0.2 (CodonCode Corp.) to produce consensus sequences and corrected using BioEdit v.7.2.5 (Hall, Reference Hall1999). The length of the final alignment was 245 bp and the dataset was further aligned with homologous wolf and dog sequences from Estonia (Hindrikson et al., Reference Hindrikson, Männil, Ozolins, Krzywinski and Saarma2012; Plumer et al., Reference Plumer, Talvi, Männil and Saarma2018). Molecular identification of species was possible due to specific nucleotide characters that distinguish between wolves and dogs in Estonia; at three nucleotide positions: 15,598, 15,655 and 15,803 (according to reference sequence KT448278 in GenBank) nucleotides C, G and T (respectively) are specific to domestic dogs, whereas T, A and C (respectively) are specific to Estonian wolves (Plumer et al., Reference Plumer, Talvi, Männil and Saarma2018).
Molecular identification of food objects
For the identification of birds, mammals, reptiles and fish, a 303 bp fragment of mtDNA cox1 gene was PCR-amplified with primers AVS2F (CCTGTGACCTTCATCAACC) and AVS3R (GTTATTTATGCGTGGGAATGCTATGTC). PCR reactions were carried out in a total volume of 20 μl with 1× Phusion HF Buffer (Thermo Fisher Scientific), 0.2 mm dNTP, 0.25 μM of each primer and 0.4 U Phusion Hot Start II DNA Polymerase and 2 μl of purified DNA. The PCR mixture was initially denatured at 98°C for 30 s, followed by 10 touchdown cycles for 10 s at 98°C, 20 s at 60°C (reducing the temperature 1°C per cycle) and 30 s at 72°C, followed by 30 cycles of 10 s at 98°C, 20 s at 50°C and 30 s at 72°C. In case the PCR was negative due to highly degraded DNA, we performed a second analysis by PCR-amplifying a shorter, 183 bp fragment of mtDNA 12S rRNA gene, using primers Ave12 F and Ave12R, described in Oja et al. (Reference Oja, Soe, Valdmann and Saarma2017). PCR products were checked using 2% 1xTAE gel-electrophoresis and visualized under ultraviolet (UV) radiation using ethidium bromide.
The PCR products were purified, sequenced and aligned as described above for the identification of dogs. A nucleotide Basic Local Alignment Search Tool (https://blast.ncbi.nlm.nih.gov/Blast.cgi) was used to identify mammal, reptile, fish and bird taxa.
Morphological analysis of food objects
The analysis was done as described in Valdmann & Saarma (Reference Valdmann and Saarma2020). Shortly, faecal samples were processed according to standard laboratory procedures (Reynolds & Aebischer, Reference Reynolds and Aebischer1991). Non-mammal remains (e.g., birds) recovered in predator scats were identified in comparison with reference materials. Mammal remains were identified by examining the cuticular pattern and the medulla of the hairs using reference manuals (Teerink, Reference Teerink1991; Tóth, Reference Tóth2017) and hairs collected from hunted animals.
Parasite identification and prevalence
Helminth occurrence was determined using the concentration flotation technique (sodium chloride, specific gravity = 1.2 g/cm3) (Roepstorff & Nansen, Reference Roepstorff and Nansen1998), followed by helminth egg counting in McMaster chambers until 100 eggs per parasite taxa. Identification of helminth taxa was based on morphological characteristics (Bowman, Reference Bowman2013). Most of the parasite taxa were identified at the genus level, except T. canis and U. stenocephala. Although, we attempted genetically to determine the species among the isolated eggs of Taeniidae, it was not successful, possibly due to partial degradation of DNA by high-UV radiation and other environmental factors.
Helminth prevalence was defined as the proportion of all eggs in scats, and infection intensity was determined as the count of eggs up to 100 per taxa in a sample. Since the upper limit in counting the helminth taxa was set to 100, this should be considered as relative intensity.
Spatial analysis
Maps were created using the Free & Open Source QGIS (v3.18; 2021) to display infected dog scats and to describe the average distance from private houses (henceforth privates) to collected faecal samples which was considered as the buffer distance around scat samples known as the average straying area of free-ranging dogs (see fig. 1). The map layers originated from public Web Map Services (Base map: Land Board, 2021).
Statistical analysis
The dependent variables consisted of (co)infection prevalence and infection intensity. The independent variable consisted of food items (data not given; a separate manuscript on the diet of rural dogs is in preparation). Food objects were divided into five categorical variables: game; bird; dog food; plant material; and rodent.
Since multiple testing was performed between (co)infection and different food groups, it is possible to obtain false positive results (Type I error) in a set of tests. The Holm–Bonferroni method (more powerful compared to the Bonferroni procedure) was applied to prevent Type I error rates when performing multiple tests (Aickin & Gensler, Reference Aickin and Gensler1996).
Due to the availability of data from a previous study of urban dog endoparasites (Tull et al., Reference Tull, Moks, Laurimaa, Keis and Süld2020), rural dogs (N = 84) were compared to urban dogs (N = 657) to find associations between (co)infection occurrence with helminths. Proportions were compared with SAS Studio v9.04 (SAS Institute, Cary NC, 2021) software using Chi-squared tests of independence (PROC FREQ) to determine independent variables associated with overall (co)infection and single taxa prevalence. If one or more cells in the 2 × 2 contingency tables had expected values of less than 5, Fisher's exact test was used. Generalized linear models (package ‘glmmTMB’ (Brooks et al., Reference Brooks, Kristensen, Benthem, Magnusson, Berg, Nielsen, Skaug, Maechler and Bolker2017) or ‘logistf’ (Heinze & Ploner, Reference Heinze and Ploner2018; R Development Core Team 2020)) were used to evaluate consumption of various food objects associated with overall helminth prevalence, coinfection prevalence and infection intensity. It was also estimated how dog diet associates with prevalence (binomial error distribution) and intensity (negative binomial error distribution) of individual helminth taxa. Models were compared using the Akaike information criterion corrected for small samples (AICc) (Burnham & Anderson, Reference Burnham and Anderson2004). Package ‘MuMIn’ (Barton, Reference Barton2019) was used for conducting model selection and model averaging. Only models with the highest Akaike weight wi(AIC) (ΔAICc < 2) were described. Furthermore, the weights (w) of the same factors presented in one model set were summed for calculating the relative variable importance (RVI).
Results
Of the 328 collected scat samples, genetic analysis identified that 84 belonged to dogs. Coprological study revealed that 87.0% (73/84, 95% confidence limit (CL) 79.5–94.3) of the faecal samples were infected with helminths. Over half of the rural dog scats harboured eggs of Taeniidae (65.5%, CL 56.7–74.3), followed by Trichuris spp./Eucoleus spp. (15.5%, CL 8.8–22.2), U. stenocephala (14.7%, CL 8.1–21.2) and T. canis (4.3%, CL 0.6–8.1) (fig. 2).
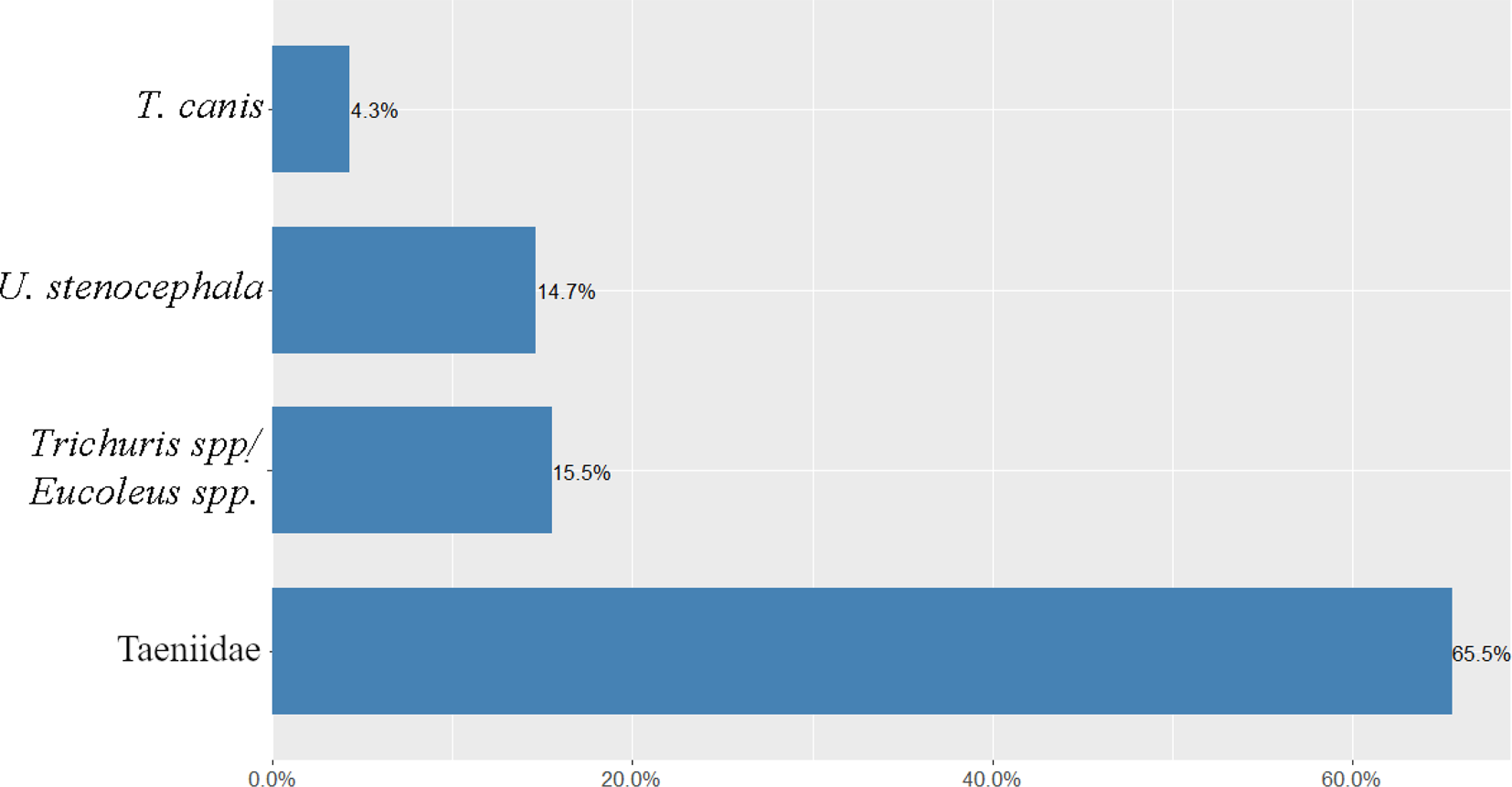
Fig. 2. Infection prevalence with different helminth taxa among rural dogs in Western Estonia.
Coinfections with more than one helminth taxa occurred in 34.5% of dogs (29/84, 95% CL 24.1–44.9). The most common coinfections were between Taeniidae and U. stenocephala (41.4%, CL 22.3–60.5), and Taeniidae and Trichuris spp./Eucoleus spp. (31.0%, CL 13.1–48.9) (fig. 3).

Fig. 3. Coinfection prevalence with helminths among rural dogs in Western Estonia.
In comparison with urban dogs, the overall helminth prevalence of urban (Tull et al., Reference Tull, Moks, Laurimaa, Keis and Süld2020) and rural dogs differed nearly nine times (9.8% and 87%, respectively) (table 1). Rural dogs (73/741, 9.9%) had significantly (P < 0.0001) higher infection prevalence than urban dogs (43/741, 5.8%). Of different helminth taxa, rural dogs (73/741, 9.9%) had significantly (P < 0.0001) higher infection prevalence with Taeniidae than urban dogs (3/741, 0.4%). However, there was no statistical difference (P = 0.5) between Toxocara sp. occurrence among rural (4/741, 0.5%) and urban dogs (22/741, 3.0%). Urban dogs were significantly more infected with U. stenocephala compared to rural dogs (P < 0.0001) (fig. 4).

Fig. 4. Comparison of parasite infections and coinfections for rural and urban dogs. Data for urban dogs are from Tull et al. (Reference Tull, Moks, Laurimaa, Keis and Süld2020). Infected faecal samples are marked with red colour and uninfected with blue.
Table 1. Helminth prevalence in dogs from rural and urban areas in European countries.
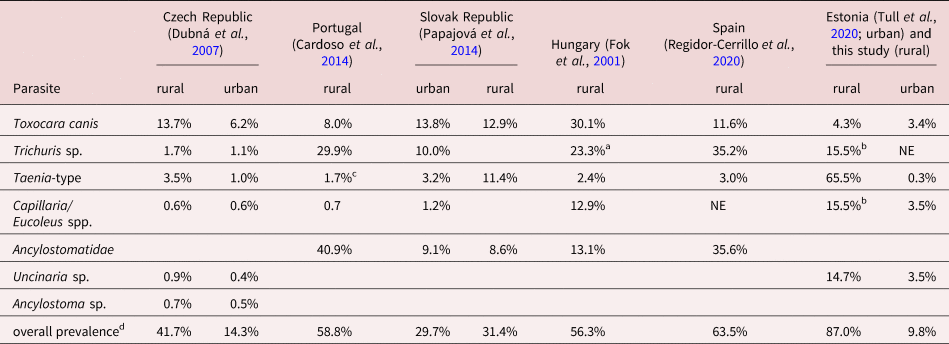
NE – not estimated; aTrichuris vulpis; bno differentiation in the study; cTaenia sp.; dincluding all parasites in the study.
Coinfections with helminths occurred more in rural (29/741, 3.9%) than in urban areas (6/741, 0.8%; P < 0.0001) (fig. 4). Rural dogs’ coinfection prevalence with helminths was predicted by two equally good models (ΔAICc < 2). The best coinfection prevalence model (wi = 0.5) with helminths showed that rural dogs preying on rodents had significantly (3.7 times) higher odds to be coinfected than rural dogs who had not preyed on rodents (βRODENT = 1.3, SE = 0.6, P = 0.02). The model also indicated a 63% reduction in rural dogs’ coinfection with helminths, if they consumed dog food (βDOGFOOD = −1.0, SE = 0.6, P = 0.1). All good models contained the factors ‘dog food’ and ‘rodent’. The RVI indicates strong effect for the factor ‘rodent’ but moderate effect towards the factor ‘dog food’.
The best infection intensity model (wi = 0.6) with Taeniidae revealed 1.8 times higher infection intensity for rural dogs preying on game (βGAME = 0.6, SE = 0.3, P = 0.04) and a 1.7 times higher infection intensity for rural dogs preying on birds (βBIRD = 0.5, SE = 0.3, P = 0.03). The RVI indicates moderate effects for the factor ‘bird’ and towards the factor ‘game’.
Rural dogs’ scats which contained rodent remains had up to four times (wi = 0.9) more Eucoleus spp./Trichuris spp. (βRODENT = 1.4, SE = 0.6, P = 0.01). However, dogs feeding on dog food more than on other prey items had significantly lower infection prevalence with Eucoleus spp./Trichuris spp. (βDOGFOOD = −2.6, SE = 1.5, P = 0.008). The RVI indicates strong effect for the factors ‘rodent’ and ‘dog food’. The intensity model (wi = 0.8) with Eucoleus spp./Trichuris spp. displayed significantly higher intensity for rural dogs preying on game than on other food items (βGAME = 2.6, SE = 0.6, P < 0.0001). The RVI indicates strong effect for the factor ‘game’ but very weak effect towards the factor ‘bird’.
The average distance between infected scats and privates was approximately 560 m. The minimum distance of an infected dog from a household was 8 m and maximum distance 1834 m. There were respectively 124 privates adjacent to the 560 m buffer zone.
Discussion
The genetic identification of scats applied in the study allowed to distinguish dog samples from other free-ranging canid species such as the red fox, raccoon dog, golden jackal and grey wolf, which guarantees that only the scats of dogs were included in further analyses.
The results revealed very high helminth occurrence (87%) among rural dogs in Western Estonia, especially near private houses (on average 560 m adjacent to privates), suggesting that the majority of dog owners do not provide anthelmintic treatment to their dogs or do it ineffectively. However, note that as it was not possible to identify individual dogs based on scat samples collected in nature (DNA degradation was too high to apply microsatellite analysis), it is difficult to tell how many dogs were involved in the sampling. Based on the relatively large study area and the abundance of dogs in this area, we can rule out that the scats belong to very few individuals. Moreover, our aim was not to analyse the parasite burden of different individuals, but the general impact of infected rural dogs contaminating the environment with parasite ova.
Although previous studies have also demonstrated extensive helminth infections among rural dogs with percentage ranging from 31% (Schurer et al., Reference Schurer, Ndao, Skinner, Irvine, Elmore, Epp and Jenkins2013) to 84.4% (Ngui et al., Reference Ngui, Lee and Yap2014), the current study has shown the highest prevalence to date. Most of the collected scats originated from the Matsalu National Park, which is also a recreational area, offering various hiking trails and outdoor sights. Humans and their pets living at or visiting the national park may come into close contact with contaminated soil, water or directly with infected scats. Therefore, considering the very high helminth prevalence in scats of rural dogs and close contacts between dogs and humans, these problematic key findings could increase transmission of zoonotic diseases and affect public health.
Over half of the examined rural dog scats contained eggs of Taeniidae (65.5%). It is known that most of taeniid species that occur in dogs have zoonotic potential (e.g., Dipylidium caninum, Dibothriocephalus latus, Taenia spp. and Echinococcus spp.). The current study revealed a higher prevalence of taeniids than most other studies (Dubná et al., Reference Dubná, Langrová, Nápravník, Jankovská, Vadlejch, Pekár and Fechtner2007; Soriano et al., Reference Soriano, Pierangeli and Roccia2010; Schurer et al., Reference Schurer, Ndao, Skinner, Irvine, Elmore, Epp and Jenkins2013; Papajová et al., Reference Papajová, Pipiková, Papaj and Čižmár2014). In some areas, the high prevalence of Taeniidae may result from coastal effect, which provides more opportunities to prey on raw fish that are host for example of D. latus. The occurrence of this parasite in black or grizzly bears and in wolves has been linked with seasonality when hosts’ diet shifts to salmon (Frechette & Rau, Reference Frechette and Rau1978; Gau et al., Reference Gau, Kutz and Elkin1999; Bryan et al., Reference Bryan, Darimont, Hill, Paquet, Thompson, Wagner and Smits2012). Fish remains can be fed to rural dogs by humans, and dogs can find these also in nature during roaming. Because the composition of taeniid species was not revealed, further studies are needed to estimate the prevalence of different taeniid species in coastal rural areas.
Although T. canis was among the least common parasites found in Western Estonian rural areas, earlier studies have shown, on the contrary, that T. canis was one of the most prevalent helminth species in rural areas of Hungary and the Slovak Republic (Antolová et al., Reference Antolová, Reiterová, Miterpáková, Stanko and Dubinský2004; Fok et al. Reference Fok, Szatmári, Busák and Rozgonyi2001). Albeit, even lower prevalence of T. canis has been found from Eastern Spain (Sanchez-Thevenet et al., Reference Sanchez-Thevenet, Carmena, Adell-Aledón, Dacal, Arias, Saugar, Rodríguez and Dea-Ayuela2019). The epidemiology of T. canis is largely affected by age of the host. The highest infection rates with T. canis were found among puppies of 4 weeks old and the infection decreased in older dogs (Barutzki & Schaper, Reference Barutzki and Schaper2013). Over the recent years, toxocariasis has gained much attention as this disease was listed as one of the five most neglected parasitic infections by the Centers for Disease Control and Prevention (CDC) in the United States. In Estonia, Remm & Remm (Reference Remm, Remm, Schærström, Jørgensen, Kistemann and Sivertun2014) showed that dog owners had a significantly higher risk of being infected with Toxocara spp. Another study, carried out by Lassen et al. (Reference Lassen, Janson, Viltrop, Neare, Hütt, Golovljova, Tummeleht and Jokelainen2016) found higher T. canis seroprevalence in animal caretakers than in the general population.
Over a third of dogs had coinfections, the most common one occurred between Taeniidae and U. stenocephala. Eggs of the zoonotic U. stenocephala are highly resistant to cold and the parasite is proliferating in areas of temperate and subarctic regions (Traversa, Reference Traversa2012; Bowman, Reference Bowman2013). According to the CDC (2020), larvae of U. stenocephala penetrate unprotected skin causing cutaneous larva migrans in humans. According to the authors’ knowledge, it is uncommon for Taeniidae and U. stenocephala to co-occur in most samples. Because scats were collected during the spring when mild weather conditions and adequate precipitation exist in temperate climate, U. stenocephala larvae rapidly develop to the infective stage, hence explaining the higher co-occurrence with Taeniidae in spring season. The higher Taeniidae coinfection rate is possibly linked to the predator–prey relationships when free-ranging rural dogs have the opportunity to prey on diverse intermediate (pike and perch) or paratenic host (rodents) species.
To date, the greatest attention has been paid to studies of the parasitological situation in urban sites of Estonia. These have included sandpits, park lawns, avenues and recreational areas, as well as public playgrounds near schools/nurseries in various towns of Estonia such as Tartu, Pärnu, Rakvere, Elva and Kunda (Talvik et al., Reference Talvik, Moks, Mägi, Järvis and Miller2006; Tull et al., Reference Tull, Moks, Laurimaa, Keis and Süld2020). Talvik et al. (Reference Talvik, Moks, Mägi, Järvis and Miller2006) reported that 2.7% of collected dog scats contained eggs of Toxocara spp. in Tartu, whereby scats adjacent to privates had higher Toxocara positivity than scats near to apartment blocks. However, more than a decade later Tull et al. (Reference Tull, Moks, Laurimaa, Keis and Süld2020) revealed higher geohelminth rates near apartment blocks than near privates. The most frequently found helminths in Estonian towns were Toxocara spp. and U. stenocephala: in Tartu 5.3%; Elva 7.8%; Kunda 12.3%; Pärnu 8.1%; and Rakvere 6.9% (Tull et al., Reference Tull, Moks, Laurimaa, Keis and Süld2020). In Iran, a higher contamination rate with Toxocara eggs (29%) was found in public parks (Maraghi et al., Reference Maraghi, Mazhab Jafari, Sadjjadi, Latifi and Zibaei2014). In Poland, after 20 years of study Mizgajska-Wiktor et al. (Reference Mizgajska-Wiktor, Jarosz, Fogt-Wyrwas and Drzewiecka2017) concluded that the level of soil contamination was highest in cities, lower in villages and the lowest in small towns. In cities, a relatively larger number of dogs in a small area can result in a high level of contamination with geohelminth eggs. Once an infected dog defecates, the eggs are passed to the environment, where they embryonate and can remain infectious for years (Blaszkowska et al., Reference Blaszkowska, Wojcik, Kurnatowski and Szwabe2013). An infected dog with T. canis can shed 10,000 eggs in each gram of scat (Ahmad et al., Reference Ahmad, Maqbool, Saeed, Ashraf and Qamar2011). Therefore, insufficient anthelmintic treatment of dogs may facilitate transmission of zoonotic parasites to humans, but also a high level of soil contamination. It should be highlighted that many available anthelmintics target only adult worms, whereas eggs are resistant to treatment. Effective methods for reducing environmental contamination and transmission to humans include: controlling the free-ranging dog population; establishing dog walking areas; enabling hygienic scat disposal by pet owners; and preventing dog access to public areas.
In a previous study (Tull et al., Reference Tull, Moks, Laurimaa, Keis and Süld2020) that focused on urban infection hotspots, it was revealed that helminth infection is higher in smaller than in larger towns in Estonia. However, the current study suggests that rural areas are in comparison with urban areas by far more contaminated with helminths and the infection risk among dogs is nine times higher in rural areas than in towns. There may be several reasons for increased infection hazard in rural areas. Rural dogs that prey on various paratenic or intermediate hosts increase their infection risk with parasites, including zoonotic. In the current study, rural dogs preying on rodents had higher coinfection risk with helminths than rural dogs consuming other food objects. In rural and suburban areas, rodents such as Arvicola terrestris, Microtus arvalis, Myodes glareolus and Apodemus agrarius can be paratenic or intermediate hosts for E. multilocularis and Toxocara spp. (Antolová et al., Reference Antolová, Reiterová, Miterpáková, Stanko and Dubinský2004; Reperant et al., Reference Reperant, Hegglin, Tanner, Fischer and Deplazes2009). Another problem highlighted by the current study is that dogs in rural areas may still have access to raw meat and offal of domestic and wild animals. It is known, for example, that dogs scavenging internal organs of wild game infected with E. granulosus s.l. can become a direct source of infection for humans and domestic animals (Baneth et al., Reference Baneth, Thamsborg, Otranto, Guillot, Blaga, Deplazes and Solano-Gallego2016). Moreover, contamination of pastures or coastal meadows with scats of infected wild carnivores also results in E. granulosus s.l. infection of domestic ruminants. The establishment of a pastoral cycle may then result from the feeding of uncooked offal from these domestic animals to dogs (Bowman, Reference Bowman2013). In Estonia E. granulosus s.l. has been found in dogs (Laurimaa et al., Reference Laurimaa, Davison and Plumer2015b), grey wolves (Moks et al., Reference Moks, Jõgisalu, Saarma, Talvik, Järvis and Valdmann2006), but also in moose (Alces alces; Moks et al., Reference Moks, Jõgisalu, Valdmann and Saarma2008) and roe deer (Capreolus capreolus; Marcinkutė et al., Reference Marcinkutė, Šarkūnas and Moks2015).
The intensity model indicated a higher helminth infection rate for rural dogs preying on rodents and preying on game. Both Eucoleus spp. and Trichuris spp. are geohelminths, maturing in the soil up to several weeks before becoming infective. However, if dog diet consisted of commercial dog food, animals had lower infection risk with helminths, especially with Eucoleus spp./Trichuris spp. Generally, dogs become infected by ingesting Eucoleus aerophilus or Trichuris vulpis infective eggs from the environment, but in rare cases infection may occur when consuming invertebrates (earthworms) or Norway rats (Rattus norvegicus) (Rothenburger et al., Reference Rothenburger, Himsworth, Lejeune, Treuting and Leighton2014; Traversa et al., Reference Traversa, Frangipane di Regalbono, Di Cesare, La Torre, Drake and Pietrobelli2014). The high helminth burden in rural dogs indicates that rural environment and customs (including no or less effective anthelmintic treatment, feeding remains of animals, etc.) are contributing significantly to the distribution of zoonotic helminths compared to urban areas.
These findings emphasize the need to implement measures such as properly disposing of dog scats, not feeding dogs with raw fish/offal and/or game meat, routine parasite screening and applying strategic parasite target-treatment to reduce helminth infections and environmental contamination in rural areas of Western Estonia. A study by Vienažindienė et al. (Reference Vienažindienė, Joekel, Schaper, Deplazes and Šarkūnas2018) found significant decreases in excretion of T. canis eggs one month after treatment with anthelmintics (Drontal Plus® (combination of pyrantel embonate and febantel) and Profender® (combination of emodepside and praziquantel)). It is also important to diagnose which (gastrointestinal) parasite species infect dogs to determine strict and specific anthelmintic treatment against cestodes, nematodes, protozoa or trematodes. The same applies also for cats (Tull et al., Reference Tull, Moks and Saarma2021). Such strategic parasite target-treatment aids to minimize anthelmintic resistance because metaphylactic use of broad spectrum anthelmintic (e.g., benzimidazoles, pyrantel and macrocyclic lactones against hookworm A. caninum) combinations could result in anthelmintic resistance (Kopp et al., Reference Kopp, Kotze, McCarthy, Traub and Coleman2008; Jimenez Castro et al., Reference Jimenez Castro, Howell, Schaefer, Avramenko, Gilleard and Kaplan2019; von Samson-Himmelstjerna et al., Reference von Samson-Himmelstjerna, Thompson, Krücken, Grant, Bowman, Schnyder and Deplazes2021).
Summary
Free-ranging dogs in rural areas can be an important source of helminth infection. We have collected and analysed 328 samples from Western Estonia. The genetic method applied in this study allowed to distinguish dog faecal samples from other free-ranging canids, identifying 84 scats belonging to dogs. Of these, 87.0% were infected with helminths, suggesting high environmental contamination with gastrointestinal parasites shed by rural dogs. In comparison with urban dogs, rural dogs have nine times higher infection risk with gastrointestinal parasites. There may be several reasons for increased infection hazard in rural areas. Rural dogs that prey on various paratenic and intermediate hosts increase their infection risk with parasites. In the current study, rural dogs preying on rodents had a higher coinfection risk with helminths than rural dogs consuming other food objects. The intensity model indicated a higher helminth infection rate for rural dogs preying on rodents and preying on game. The high helminth burden in rural dogs indicates that rural environment and customs (including none or less effective anthelmintic treatment, feeding remains of animals, etc.) are contributing significantly to the distribution of zoonotic helminths compared to urban areas.
Last but not least, educating and counselling pet owners about helminth infections and their impact on the health of pets and humans is essential, especially in rural regions, considering the very high prevalence of intestinal helminths found among rural dogs.
Acknowledgements
We thank Ants Kaasik, Agnes Kapanen and Kristjan Põldma for their valuable help.
Financial support
This work was supported by research funding (grant PRG1209) from the Estonian Ministry of Education and Research; Environmental Investment Centre (project 14338).
Conflicts of interest
The authors declare there are no conflicts of interest.
Ethical standards
All ethical requirements stipulated in Estonian law were met in this study.
Author contributions
AT, HV and US conceived and designed the study. AT, US, HV, RR, TK and ET conducted the sampling. AT performed statistical analyses. US and ET performed genetical analysis. AT wrote the first draft and US, HV, RR, TK and ET contributed.