Introduction
Golvan erected Sphaerirostris Golvan, 1956 as a subgenus of Centrorhynchus Lühe, 1911 and included 21 species with short spindle-shaped trunk, polydendritic lacunar system, three or four tubular cement glands and short globular anterior proboscis. Centrorhynchus, on the other hand, has long and cylindrical trunk with anterior dilation, transverse anastomoses of secondary lacunar vessels, 3–4 very long cement glands and truncated cylindrical anterior proboscis with slight posterior dilation. There are over 98 valid species of Centrorhynchus Lühe, 1911 known from birds throughout the world (Amin, Reference Amin2013). Much of the early descriptions of Centrorhynchus globocaudatus (Zeder, 1800) Lühe, 1911 (Centrorhynchidae) by Zeder (Reference Yamaguti1800) and Rudolphi (Reference Rubtsova, Heckmann, Smit, Luus-Powell, Halajian and Roux1802) were repeated by subsequent observers. The distribution of C. globocaudatus appears to extend throughout Europe, Asia and Africa in many species of birds of prey of the genera Anthus Bechstein (Pipits), Aquila Brisson (true eagles), Asio (Linnaeus) (typical owls); Athene Boie (owls), Buteo Lacépède (buzzards), Circus Lacépède (harrier-hawks), Falco Linnaeus (falcons and kestrel), Glaucidium Boie (pigmy owls), Milvus Lacépède (kites), Ottus Pennant (Eurasian, Old World, scops owls), Strix Linnaeus (wood owls) and Tyto Billberg (barn owls) (see Petrochenko, Reference Nelson and Ward1950, Reference Petrochenko1958; Florescu & Ienistea, Reference Florescu and Ienistea1984; Hoklova, Reference Heckmann, Amin and El-Naggar1986; Lisitsyna, Reference Lee2019). Insects serve as intermediate hosts for species of Centrorhynchus, while amphibians, reptiles and occasionally insectivorous mammals serve as second intermediate or paratenic hosts. Nelson & Ward (Reference Muhammad, Suleman, Saleem Ahmad, Li, Zhao, Ullah, Zhu and Ma1966) collected juvenile specimens of C. globocaudatus from the long-eared hedgehog, Hemiechinus auritus Gmelin, in the Egyptian El Tahreer Province, and Torres & Puga (Reference Torres and Puga1966) collected cystacanths of specimens of Centrorhynchus sp. from two species of frogs in Chile – Eupsophus calcaratus Günther and Eupsophus roseus Dumeril.
Reasonably adequate versions of the description of C. globocaudatus by these authors from Ukraine, Kyrgyzstan and former Soviet states, respectively, among others listed in table 1, were used for comparison with our Italian specimens. Other brief descriptions usually based on one or two male or female specimens were also reported from other localities in Bulgaria (Dimitrova et al., Reference Dimitrova, Gerogiev and Genov1997), Hungary (Dimitrova et al., Reference Dimitrova, Murai and Gerogiev1995), India (Gupta & Gupta, Reference Gazi, Kim, García-Varela, Park, Littlewood and Park1972), Slovakia (Komorová et al., Reference Kang and Li2015) and West Africa (Dimitrova & Gibson, Reference Dimitrova and Gibson2005). The brief descriptions in Meyer (Reference Lisitsyna and Greben1932) and Yamaguti (Reference Wright and Lumsden1963) are worth noting. Two reports on synanthropic birds and their parasites in southern Italy by Dipineto et al. (Reference Dipineto, Borelli, Pepe, Fioretti, Caputo, Cringoli and Rinaldi2013) and Santoro et al. (Reference Rudolphi2010) made reference to C. globocaudatus from the common kestrel, Falco tinnunculus Linnaeus, but no descriptive accounts are known for this acanthocephalan from Italy. Our descriptive account is based on collections from northern Italy at Ferrara. Our findings on C. globocaudatus recognize a new morphological variant and add new descriptive information that expands our understanding of this interesting acanthocephalan. For instance, the anterior five sub-apical hook roots were each found to be unique in shape and size rather than appearing identical, as has been noted in previous reports using only line drawings.
Table 1. Collections of Centrorhynchus globocaudatus from Falco tinnunculus and Buteo buteo upon death at the Experimental Zooprophylactic Institute of Ferrara, Italy, in 2018 and 2019.

a Number of birds infected/number examined (acanthocephalans collected).
b These may be specimens of C. globocaudatus, but their identity could not be verified because of extreme contraction.
As usual in most acanthocephalan families, few sequences are available for centrorhynchids. For C. globocaudatus, only two sequences, corresponding to 18S and 28S ribosomal DNA (rDNA) genes, have been published to date (García-Varela et al., Reference García-Varela, Pérez-Ponce de León, de la Torre, Cummings, Sarma and Laclette2020a). If we consider published sequences of the genus, the number can be only expanded to six for the 18S gene, and even fewer sequences (4) are available for cytochrome c oxidase 1 (cox1).
In the present study, new scanning electron microscopy (SEM) and microscopic images, energy dispersive X-ray analysis (EDXA), micropore and related studies, and DNA analysis expand the body of knowledge about C. globocaudatus in particular and the genus Centrorhynchus in general.
Materials and methods
Collections
The birds examined for this study were collected between June 2018 and August 2019 from the countryside or villages around Ferrara (44°50′N, 11°37′E). Moribund birds injured in car accidents or those unable to fly are first brought to Centro Recupero Fauna Selvatica (Center for Recovery of Wild Fauna) (CRAS), often with police (Section of Wild Fauna) intervention, for first aid, and are hospitalized with a unique CRAS number. If the birds do not recover or die, they are sent to the Experimental Zooprophylactic Institute of Ferrara at St Modena for necropsy, recovery of parasites and identification of possible infectious agencies such as West Nile Virus, then incinerated.
A total of 110 clearly identifiable specimens of C. globocaudatus were collected from 13 infected common kestrels (falcons), F. tinnunculus, and 32 from four infected common buzzard, Buteo buteo (table 1). Specimens from both host species were examined as follows: 16 specimens for SEM and EDXA, six for molecular analysis (three from each host species) and 12 males and 13 females (nine and ten from F. tinnunculus, and three and three from B. buteo, respectively) for microscopical study. Other specimens remain in O.M.A.'s personal collection. Freshly collected specimens were extended in water until proboscides everted then fixed in 70% ethanol for transport to our Arizona, USA laboratory for processing and further studies.
Methods for microscopical studies
Worms were punctured with a fine needle and subsequently stained in Mayer's acid carmine, destained in 4% hydrochloric acid in 70% ethanol, dehydrated in ascending concentrations of ethanol (24 h each) and cleared in 100% xylene then in 50% Canada balsam and 50% xylene (24 h each). Whole worms were then mounted in Canada balsam. Measurements are in micrometres, unless otherwise noted; the range is followed by the mean values between parentheses. Width measurements represent maximum width. Trunk length does not include proboscis, neck or bursa.
Microscope images were created using 10× and 40× objective lenses of a BH2 light Olympus microscope (Olympus Optical Co., Osachi-shibamiya, Okaya, Nagano, Japan) attached to an AmScope 1000 video camera (United Scope LLC, dba AmScope, Irvine, California, USA), linked to an ASUS laptop equipped with a high-definition multimedia interface system (Fremont, California, USA). Images from the microscope are transferred from the laptop to a USB and stored for subsequent processing on a computer.
Specimens were deposited in the University of Nebraska State Museum's Harold W. Manter Laboratory (HWML) under collection number 139,404 (voucher specimens on one slide), Lincoln, Nebraska, USA.
SEM
Specimens that had been fixed and stored in 70% ethanol were processed for SEM following standard methods (Lee, Reference Kumar and Filipski1992). These included critical-point drying in sample baskets and mounting on SEM sample mounts (stubs) using conductive double-sided carbon tape. Samples were coated with gold and palladium for 3 min using a Polaron #3500 sputter coater (Q150 TES, Quorum: www.quorumtech.com) establishing an approximate thickness of 20 nm. Samples were placed and observed in an FEI Helios Dual Beam Nanolab 600 (FEI, Hillsboro, Oregon, USA) scanning electron microscope, with digital images obtained in the Nanolab software system (FEI, Hillsboro, Oregon, USA) and then transferred to a USB for future reference. Samples were received under low vacuum conditions using 10 KV, spot size 2, 0.7 torr using a gaseous secondary electron detector.
EDXA
Standard methods were used for preparation similar to the SEM procedure. Specimens were examined and positioned with the aforementioned SEM instrument, which was equipped with a Phoenix energy-dispersive X-ray analyser (FEI, Hillsboro, Oregon, USA). X-ray spot analysis and live scan analysis were performed at 16 Kv with a spot size of 5, and results were recorded on charts and stored with digital imaging software attached to a computer. The TEAM (Texture and Elemental Analytical Microscopy) software system (FEI, Hillsboro, Oregon, USA) was used. Data were stored in a USB for future analysis. The data included weight percent and atom percent of the detected elements following correction factors.
Ion sectioning of hooks
A dual-beam SEM with a gallium (Ga) ion source (GIS) was used for the liquid metal ion source (LMIS) part of the process. The hooks of the acanthocephalans were centred on the SEM stage and cross-sectioned using a probe current of between 0.2 nA and 2.1 nA according to the rate at which the area was cut. The time of cutting was based on the nature and sensitivity of the tissue. Following the initial cut, the sample also underwent a milling process to obtain a smooth surface. The cut was then analysed with X-ray at the tip, middle and base of hooks for chemical ions with an electron beam (Tungsten) to obtain an X-ray spectrum. Results were stored with the attached imaging software. The intensity of the GIS was variable according to the nature of the material being cut.
Molecular methods
Total genomic DNA (gDNA) was extracted from four specimens of C. globocaudatus (two ex. B. buteo and two ex. F. tinnunculus) preserved in ethanol 70% using a Qiagen™ (Valencia, California, USA) DNeasy® Tissue Kit and following the manufacturer's instructions. Partial nuclear small subunit (SSU) rDNA (18S rDNA) and partial fragments of mitochondrial cox1 gene were amplified (50 μl total volume) using ExcelTaqTM SMOBIO® PCR Master Mix (Taiwan) containing 5× concentrated master mix – that is, a mixture of recombinant Taq DNA polymerase, reaction buffer, magnesium chloride (2 mM), deoxynucleotide triphosphates (dNTPs) (0.2 mM) and enzyme stabilizer; 0.25 μM of each polymerase chain reaction (PCR) primer and 2 μl of extracted gDNA. Primer pairs and amplification conditions used for both genes were as described in Amin et al. (Reference Amin, Heckmann, Dallarés, Constenla and Ha2019a). In every PCR run, one negative and one positive control were included. PCR amplicons were sequenced directly for both strands using the same PCR primers.
Sequences were assembled and edited using ContigExpress implemented in the software Vector NTI Advance® version 10.3.0 (Thermo Fisher Scientific, Massachusetts, U.S.) and submitted to GenBank under accession numbers MT993836–MT993837 (18S rDNA) and MT992255–MT992256 (COI). Sequences were aligned using Muscle implemented in MEGA version 6 (Tamura et al., Reference Tamura, Stecher and Peterson2013), together with all published 18S and cox1 sequences of species within the family Centrorhynchidae available in GenBank. Detailed data on the sequences used on both alignments and phylogenetic reconstructions can be found in table 2. Echinorhynchus truttae Schrank, 1788 (Echinorhynchidae) was used as outgroup in both datasets (AY830156 and FR856883 in 18S and cox1 datasets, respectively). Both alignments (18S: 764 nt positions, of which four were excluded prior to analysis; cox1: 668 nt positions, of which 29 were excluded prior to analysis) were used for comparative sequence analysis.
Table 2. Data for Centrorhynchidae sequences used in molecular alignments and phylogenetic reconstructions. All sequences were retrieved from GenBank (except those from the present study).
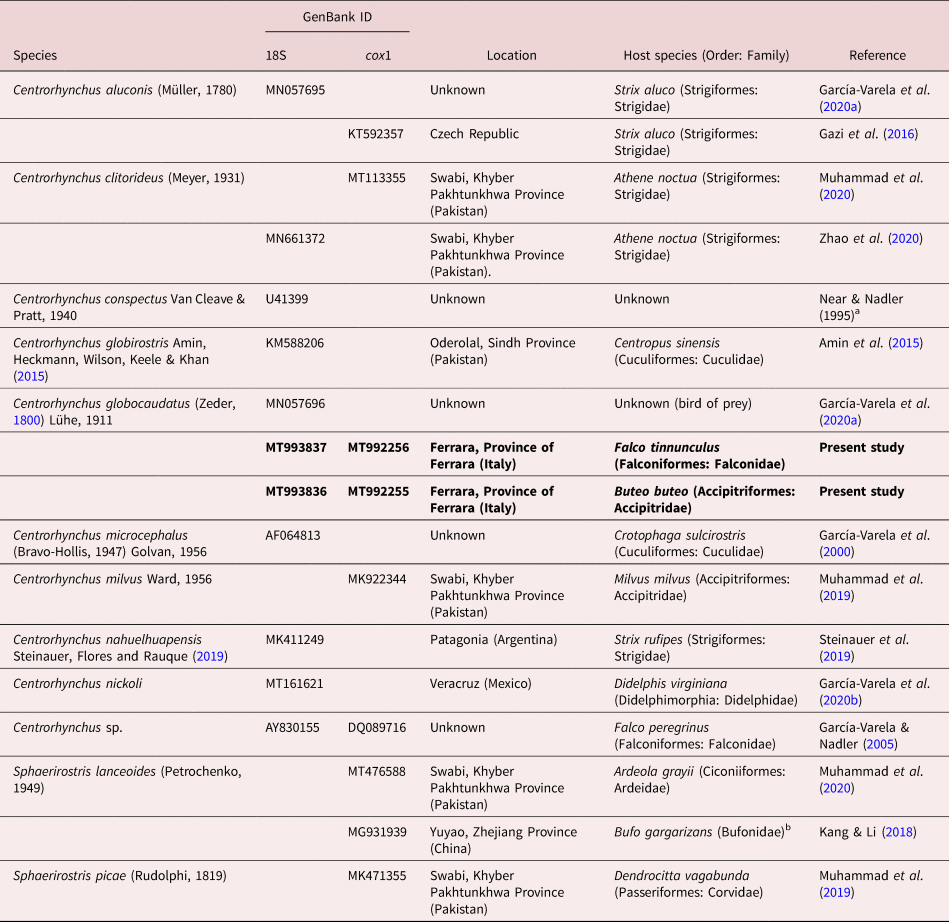
a Direct submission in GenBank.
b Acanthocephalans retrieved from their amphibian intermediate/paratenic host.
Gblocks 0.91b implemented on the Phylogeny.fr site (Dereeper et al., Reference Dereeper, Guignon and Blanc2008) was used to select blocks of evolutionarily conserved sites. Maximum likelihood (ML) and Bayesian inference (BI) algorithms were used for phylogenetic tree reconstruction after determination of the best-fit model of nucleotide substitution with jModelTest version 2.1.4 (Darriba et al., Reference Darriba, Taboada, Doallo and Posada2012) using the Akaike Information Criterion and the Bayesian Information Criterion, respectively. For the 18S dataset, the best-fitting model selected for the ML algorithm was the GTR + G model (nst = 6, rates = gamma, ngammacat = 4), while for BI it was K80 + G (nst = 2, rates = gamma, ngammacat = 4). For the cox1 dataset, the best-fitting model selected for ML algorithm was the GTR + G model (nst = 6, rates = gamma, ngammacat = 4), while for BI it was HKY + G (nst = 2, rates = gamma, ngammacat = 4). ML analyses were performed in PhyML version 3.0 (Guindon et al., Reference Guindon, Dufayard, Lefort, Anisimova, Hordijk and Gascuel2010) with a non-parametric bootstrap of 100 replicates. BI analyses were carried out with MrBayes version 3.2.6 (Ronquist et al., Reference Ronquist, Teslenko, Van Dermark, Ayres, Darling, Höhna, Larget, Liu, Suchard and Huelsenbeck2012) on the CIPRES Science Gateway version 3.3 (Miller et al., Reference Meyer2010). Log likelihoods were estimated over 10,000,000 generations using Markov chain Monte Carlo searches on two simultaneous runs of four chains, sampling trees every 1000 generations. The first 25% of the sampled trees were discarded as ‘burn-in’, and a consensus topology and nodal support estimated as posterior probability values (Huelsenbeck et al., Reference Hoklova2001) were calculated from the remaining trees. Pairwise genetic distance matrices were calculated using the ‘uncorrected p-distance’ model implemented in MEGA version 6.
Results
The distribution of C. globocaudatus is known from at least ten genera of birds of prey in Europe, Asia and Africa (table 3). Our Ferrara, Italy, population of C. globocaudatus became available as a result of the regional monitoring of birds of prey to assess the health of wild fauna and to identify the risk of parasitic and infectious agents, especially West Nile virus, for domestic animals, farm animals and humans. Our collection (table 1) shows a markedly higher intensity of infection in F. tinnunculus than in B. buteo, but rather similar prevalence rates. The comparable feeding modality of both bird species, mostly on young mammals like voles, less frequently on game and passerine birds, lizards, snakes, frogs, toads and invertebrates like arthropods, may be at play (Bergman, Reference Bergman1961; Cramp & Brooks, Reference Cramp and Brooks1992; Viitala et al., Reference Torres and Puga1995).
Table 3. Morphometric comparisons of key taxonomic characters between populations of Centrorhynchus globocaudatus from various bird hosts in different geographical locations in Asia, Europe and Africa.

L: length, W: width, H: hook.
a Except for size of trunk, Ward (Reference Viitala, Korpimäki, Palokangas and Koivula1964) provided only one set of measurements and counts for both male and female specimens.
b Range (mean) in micrometres unless otherwise noted.
c Largest dorsal hook length, largest ventral hook length. Longest hook length is used as an indicator of the size of all hooks for comparative purposes with specimens from other collections.
d Bolded figures markedly vary from those of our Italian specimens.
We describe our northern Italian population morphologically as a geographical variant because of its distinct variability from other descriptive accounts elsewhere where information is available. Descriptive reports variably document its morphology using only line drawings. Among these, we consider the line drawings in Petrochenko (Reference Nelson and Ward1950) and Lisitsyna & Greben (Reference Lisitsyna2015) to be the most complete and adequately representative. Minor exceptions are noted. We, therefore, opted not to duplicate these line drawings of the species and, instead, produce SEM and microscope images for the first time for documentation and introduce new information on the EDXA to discern hook metal composition, Ga hook cuts, micropores and molecular analysis for the first time. Additional details of such structures as proboscis hook roots are described and inaccuracies and errors are being corrected.
The following morphological description is based on the microscopical examination of 25 specimens (12 males, 13 females) and others used in the SEM studies. These study specimens were collected from the common kestrel, F. tinnunculus (19 specimens), and the common buzzard, B. buteo (six specimens), and a complete set of measurements of each were made before later collections in 2019 were made.
Morphological description of our population from Ferrara Province, Italy
Centrorhynchus globocaudatus (Zeder, 1800) Lühe, 1911 (figs 1–3, 4a–d)
General. With characters of the genus Centrorhynchus and the family Centrorhynchidae, as defined by Amin et al. (Reference Amin, Heckmann, Wilson, Keele and Khan2015). Shared structures markedly larger in females than in males (table 3). Trunk long, cylindrical, slightly wider anteriorly in males and with prominent posterior swelling and terminal knob in females. Cuticle with cross-striations, especially anteriorly, and lacunar system with prominent transverse secondary lacunar canal. Body wall with many fractured nuclei, and micropores with diverse diameter and distribution in different trunk regions (fig. 2d–f). Proboscis elongate cylindrical, gradually widening posteriorly (fig. 1a), apically truncated and bare (fig. 1b), but apical organ occasionally evident by its anterior invagination (fig. 1c, d). Proboscis widening posteriorly past faint constriction at point of attachment of receptacle. Proboscis with 29–33 longitudinal rows of 19–22 hooks each. Anterior 5–7 hooks strongest (fig. 1e), with prominent core and thin cortical layer (fig. 2b, c) and prominent posteriorly directed roots variable in shape and size (fig. 5b); third or fourth hooks longest. Ventral surface of hooks with many pebble-like protrusions, especially near base (fig. 1f). Next 5–7 hooks transitional, spiniform, with prominent scutiform X-shaped roots at area of receptacle attachment near proboscis constriction. Subsequent posterior spiniform hooks (fig. 2a) with smaller scutiform roots becoming simpler and directed anteriorly in posteriormost hooks. Anterior hooks appear in alternating longitudinal rows, but they and all spiniform hooks also appear in definite spiral rows (fig. 1a). Neck relatively short with clear cuticular separation from basal proboscis region (fig. 2a). Proboscis receptacle (PR) double-walled, about twice as long as proboscis in females (c. 1.5 as long in males) with cephalic ganglion near its middle at level of anteriormost margin of trunk. PR inserts anteriorly near anterior third of proboscis where anterior robust hooks transform into transitional spiniform hooks at faint constriction. Lemnisci sacciform, equal, slightly shorter than receptacle but may reach level of anterior testis.

Fig. 1. SEM of specimens of Centrorhynchus globocaudatus from Falco tinnunculus and Buteo buteo from northern Italy at Ferrara. (a) The proboscis of a female specimen. Note the spiral pattern of the longitudinal hook rows. (b) The anterior end of a proboscis showing the bare apical surface. (c) The sensory pore is more visible (arrow) at the apical end of another proboscis in occasional specimens. (d) A higher magnification of the sensory pore in (c). (e) A series of anterior hooks. Smaller apical hooks are more anterior. (f) A high magnification of a hook near its base showing latero-ventral pebble-like protrusions.

Fig. 2. SEM of specimens of Centrorhynchus globocaudatus from Falco tinnunculus and Buteo buteo from northern Italy at Ferrara. (a) The distinct interface between the posterior end of a proboscis near a basal circle of small hooks and the neck. The arrow points to the interface between the proboscis and the neck. (b) A lateral view of a Ga-cut hook showing the solid prominent core and very thin cortical layer. (c) A cross section of another hook demonstrating the layering showed in (b). (d, e) Micropores of different sizes, distribution and shape in the anterior and middle trunk of a male specimen, respectively. (f) A higher magnification of micropores in (e) display their unusual elliptical elongated shape.

Fig. 3. SEM of female reproductive structures of specimens of Centrorhynchus globocaudatus from Falco tinnunculus and Buteo buteo from northern Italy at Ferrara. (a) The broad posterior portion of a female specimen showing the sub-ventral position of the gonopore where the trunk narrows into a terminal caudal papilla. (b) A lateral view of the enlarged posterior portion of a female trunk and the common dorsal curvature commonly described as shoe-like. (c) Egg with smooth surface. (d) Egg with tuberculated surface, which may be at a different stage of development. (e) A high magnification of a portion of the egg in (d) showing a sperm (arrow). (f) Part of a Ga-cut lateral section of an egg showing the following structures: A, acanthor; E, entoblast cells; FM, fertilization membrane; IM, inner membrane; OM, outer membrane; SN, subcuticular nuclei.

Fig. 4. SEM of the male reproductive structures of specimens of Centrorhynchus globocaudatus from Falco tinnunculus and Buteo buteo from northern Italy at Ferrara. (a) A lateral view of an extended elongated bursa. (b) A bursa bloated posteriorly showing the distribution of sensory plates only at its posterior end (arrow). (c) A high magnification of the bursa in (d) showing the distribution of the elevated ovoid sensory plates (arrows). (d, e) High magnifications of sensory plates showing their variable shapes, organization and elevation.

Fig. 5. Microscopic images of specimens of Centrorhynchus globocaudatus from Falco tinnunculus and Buteo buteo from northern Italy at Ferrara. (a) A lateral view of proboscis hooks showing their variable sizes and the variable shape and size of their roots. The roots vary in size and thickness antero-posteriorly. (b) The shape and size proportion of cement glands (CG) and CGD draining into the bursa (B). (c) The anterior end of Saefftigen's pouch (SP) overlapping the posterior end of the cement gland. (d) The posterior end of a female specimen showing the subterminal position of the gonopore, constriction of the body wall into the terminal papilla and the muscle band holding its dorsal and ventral sides together (arrow).
Males (based on 12 mature specimens with sperm). See table 3 for measurements and counts. Testes in anterior fifth of trunk, in tandem, not large, elliptical, nearly equal, contiguous or slightly overlapping. Four cement glands, tubular, tightly contiguous, often appearing as long cord, staggering anteriorly at posterior testis and extending posteriorly into prominent cement gland ducts (CGDs) (fig. 5b) discharging into bursa. Robust Saefftigen's pouch prominent, elongate-drop-shaped, widest anteriorly, contiguous with posterior end of cement glands (fig. 5c) and overlapping CGDs. Bursa large, longer than wide, with oblong sensory pits in round elevated rims. Common sperm duct, cement glands duct and Saefftigen's pouch jointly end in bursa. Gonopore terminal.
Females (based on 13 gravid adults). Few females had ovarian balls. See table 3 for measurements and counts. Posterior end of trunk inflating abruptly then constricting into terminal prominent papilla (fig. 3a, b). Female reproductive system largely masked by dark eggs, usually in two lateral fields along worm length. Vagina complex, subterminal, with three sphincters and slit orifice opening at base of terminal globular papilla. Wall of papilla thick, continuous with body wall, with prominent muscle band holding dorsal and ventral sides together (fig. 5d, arrow). Uterus comparatively long; uterine bell short with few but prominent cells. Eggs ovoid, with thick concentric shells, and surface varying between smooth (fig. 3c) and tuberculated (fig. 3d), with occasional sperms evident (fig. 3e). Three eggshells evident (arrows) and embryonic acanthor and its components seen in Ga-cut sections (fig. 3f).
Taxonomic summary
Italian hosts examined. The common kestrel, F. tinnunculus Linnaeus (Falconidae), and the common buzzard, B. buteo Linnaeus (Accipitridae).
Locality. Around Ferrara (44°50′N, 11°37′E), Province of Ferrara, Italy.
Localization. Intestine.
Specimens deposited. HWML collection number 216359.
EDXA
The unique metal composition of hooks (EDXA) (tables 4–6 and figs 6–10) demonstrated a considerably high level of calcium and phosphorus in hook tip, a middle but low level of sulphur and negligible levels of other metals (table 4 and figs 6, 7). The eggs had the highest levels of sulphur in the cortical and core areas, but high levels of phosphorus only in the core and not in the cortical layer (table 6 and fig. 10).
Table 4. Chemical composition of a Ga (LMIS)-cut hook (cross and longitudinal cuts) for Centrovhynchus globocaudatus using X-ray scans (EDXA).

Table 5. Chemical composition of tip cuts of hooks at three levels of proboscis of Centrorhynchus globocaudatus from Falco tinnunculus.

Table 6. Chemical composition of a Ga (LMIS)-cut egg of Centrorhynchus globocaudatus using X-ray scans (EDXA).

a Common protoplasmic elements (C, N, O) and processing elements (Au, Pd, Ga) omitted. Given in WT%.
b Bolded figures are used to generate spectra in fig. 10.

Fig. 6. Energy dispersive X-ray spectrum of the edge of a cross GA-cut hook tip of a Centrorhynchus globocaudatus specimen showing high levels of calcium and phosphorus (see bolded figures in table 4). Insert: SEM of a middle proboscis hook and a GA-cut cross section of a hook.

Fig. 7. Energy dispersive X-ray spectrum of a longitudinal GA-cut hook arch of a Centrorhynchus globocaudatus specimen showing high levels of calcium and phosphorus (see bolded figures in table 4). Insert: SEM of proboscis hooks and a longitudinal GA-cut section of a hook.

Fig. 8. Energy dispersive X-ray spectrum of the centre of a GA-cut middle hook of a Centrorhynchus globocaudatus specimen showing high levels of calcium and phosphorus (see bolded figures in table 5). Insert: SEM of a cross section of a middle proboscis hook.

Fig. 9. Energy dispersive X-ray spectrum of the centre of a Ga-cut basal hook of a Centrorhynchus globocaudatus specimen showing considerably low levels of calcium and phosphorous compared to middle hooks (fig. 8), but markedly higher levels of sulphur (see bolded figures in table 5). Insert: SEM of a cross section of a basal proboscis hook.

Fig. 10. Energy dispersive X-ray spectrum of the centre of the edge of a GA-cut egg of a Centrorhynchus globocaudatus female specimen showing a markedly high levels of sulphur (see bolded figures in table 6) and low levels of other metals tested. Insert: SEM of a part of Ga-cut egg; see fig. 3f for detail.
Micropores
The electron-dense micropores present throughout the epidermal surface of the trunk of C. globocaudatus are described. They have been found in various regions of the trunk in different diameters and distributions (fig. 4a, b).
Molecular results
Two identical partial 18S rDNA sequences (760 and 833 nt length) and two cox1 mitochondrial DNA (mtDNA) sequences (651 and 668 nt length) differing by 1 nt (0.16% divergence) were generated, one from each host (i.e. B. buteo and F. tinnunculus). Figures 11 and 12 display consensus trees constructed from 18S rDNA and cox1 alignments, respectively.

Fig. 11. BI phylogram reconstructed using two newly generated 18S rDNA sequences of Centrorhynchus globocaudatus and retrieved sequences from GenBank for Centrorhynchidae. Outgroup: Echinorhynchus truttae. Newly generated sequences are highlighted in bold. Nodal support from ML and BI analyses are indicated as BI/ML. Bootstrap values lower than 70 and posterior probability values lower than 0.9 are omitted. Scale bar indicates expected number of substitutions per site.

Fig. 12. ML phylogram reconstructed using two newly generated cox1 sequences of Centrorhynchus globocaudatus and retrieved sequences from GenBank for Centrorhynchidae. Outgroup: Echinorhynchus truttae. Newly generated sequences are highlighted in bold. Nodal support from ML and BI analyses are indicated as BI/ML. Bootstrap values lower than 70 and posterior probability values lower than 0.9 are omitted. Scale bar indicates expected number of substitutions per site.
According to phylogenetic analyses based on the 18S rDNA gene (fig. 11), present newly generated sequences were identical to that of C. globocaudatus published by García-Varela et al. (Reference García-Varela, Pérez-Ponce de León, de la Torre, Cummings, Sarma and Laclette2020a) (MN057696). Therefore, these three sequences formed a strongly supported clade. The sequences that differed the most from this clade corresponded to Centrorhynchus nickoli (MT161621) and Centrorhynchus microcephalus (AF064813) (by 0.051 and 0.045% (39 and 34 nt), respectively), although the rest of the Centrorhynchus 18S sequences differed from newly generated sequences by 0.033–0.041% (25–31 nt) (table 7).
Table 7. Matrix of the pairwise 18S (left) and cox1 (right) nucleotide genetic distances among Centrorhynchus and Sphaerirostris species.

In phylograms based on the cox1 gene, present sequences formed a well-supported clade apart from all other centrorhynchid sequences included in the analyses (fig. 12). The only other clade displaying high support included Sphaerirostris sequences, with 11–30 nt (0.017–0.047%) difference among them, and was embedded among Centrorhynchus sequences forming a sub-clade. However, nucleotide difference highly increased if Sphaerirostris sequences are compared with newly generated ones (0.248–0.255%, 158–163 nt difference) and other species of the genus Centrorhynchus (0.194–0.264%, 124–169 nt; table 7). Other Centrorhynchus cox1 sequences differed with present newly generated sequences by 0.250–0.277% (160–177 nt; table 7), and showed very weakly supported phylogenetic relationships.
Remarks
A morphometric comparison between our Italian specimens and those from other geographical locations where comparative measurements and counts were available is shown in table 3. Centrorhynchus globocaudaus has been reported from many other locations in Asia, Africa and Europe without taxonomic descriptions, leaving their morphologic variability unaccounted for. Most measured specimens were collected from F. tinnunculus, thus eliminating host species as a factor in observed differences in sizes (table 3). Our specimens had markedly longer proboscis hooks and larger receptacle, and smaller male reproductive system (testes and cement glands) and lemnisci, especially in males compared to specimens from Ukraine, India, Egypt, Kyrgystan, Russia, Georgia, Armenia and Asian Soviet Republics. The Indian specimens probably had an erroneous testis size of 468 × 360. Additionally, females from Ukraine and Kyrgystan had more proboscis hook rows, and those from Ukraine had relatively larger eggs (table 3). Such quantitative variations distinguishing the Italian population as a geographical variant have been previously demonstrated in the comparable case of Mediorhynchus papillosus Van Cleave, 1916 (Gigantorhynchidae) by Amin & Dailey (Reference Amin and Dailey1998). Amin & Dailey (Reference Amin and Dailey1998) studied the key taxonomic characteristics in various geographical populations of M. papillosus, which has a wide range of distribution in at least 73 species of birds outside of North and South America in Asia from Taiwan to the east into China, many of the former Soviet Republics and to Eastern Europe to the west. Amin & Dailey (Reference Amin and Dailey1998) compared measurements of specimens from birds in Maryland, Colorado (their study material), Taiwan, Yakutia, Trans-Baikal, Lower Yansi River basin, the Volga basin and Oren Byreg, Ukraine, Bulgaria, China and Brazil, and demonstrated a distinct geographically based variability, especially in the size of proboscis and its armature, neck, receptacle and testes, that appeared related to geographical restrictions, intermediate and definitive host specificity and distribution, and host feeding behaviour. ‘The U.S. population from Colorado and the Taiwanese population [were shown to be] at the opposite ends of the spectrum’ by Amin & Dailey (Reference Amin and Dailey1998), who dismissed the possibility of elevating them to a specific status. The population variant of C. globocaudatus from Italy is, nevertheless, comparable to the east–west-intraspecific clinal variants of M. papillosus and could have been considered as a distinct species, but this notion is dismissed here also for the same reasons.
Discussion
A qualitative comparison between the Italian specimens and those from other geographical locations in table 1 and elsewhere shows discrepancies that may be related to artifacts or inaccuracy of recording observations. These discrepancies are to be expected considering the large number of descriptive accounts of this common acanthocephalan that have been reported since 1800. These discrepancies are rectified below to avoid misinterpretations of the correct descriptive account of C. globocaudatus. Intraspecific variabilities, such as differences in the number of anterior, transitional and posterior spiniform hooks/row, will not be included.
Setting the record straight
• Lisitsyna & Greben (Reference Lisitsyna2015) stated that the proboscis has a ‘maximum width at the anterior part’ of males and females and their Fig. 3a shows a posterior proboscis constriction. The proboscis is actually widest posteriorly and Florescu & Ienistea (Reference Florescu and Ienistea1984, Fig. 21) and our observations (fig. 1a) demonstrate this character best.
• Lisitsyna & Greben (Reference Lisitsyna2015) stated that ‘Gonopore subterminal in both sexes.’ Actually, the male gonopore is terminal (Fig. 20). The bend at the posterior end of the male trunk makes the gonopore appear subterminal.
• Nelson & Ward (Reference Muhammad, Suleman, Saleem Ahmad, Li, Zhao, Ullah, Zhu and Ma1966) examined four juvenile specimens from the long-eared hedgehog, H. auritus Gmelin, from Egypt, showing a ‘nearly cylindrical’ proboscis also widest anteriorly (their Fig. 6), with unusually few (26–28) longitudinal rows of 20–22 hooks each.
• Only Ward (Reference Viitala, Korpimäki, Palokangas and Koivula1964) and the present paper document longer ventral and shorter dorsal proboscis hooks (table 3). All other accounts include only one set of measurements of hooks overlooking their dorso–ventro differentiation.
• Dimitrova et al. (Reference Dimitrova, Gerogiev and Genov1997), however, noted slightly longer latero-ventral hooks (60–65 long) than dorso-lateral hooks in one male worm from F. tinnunculus, but their other male worm from Falco vespertinus Linnaeus did not show such differentiation.
• Ward (Reference Viitala, Korpimäki, Palokangas and Koivula1964) referred to spiniform hooks on the posterior proboscis as being ‘without roots.’ Actually, these hooks have small scutiform roots, becoming more simple and directed anteriorly in posteriormost hooks, as recognized in our Italian specimens and many other reports.
• Ward (Reference Viitala, Korpimäki, Palokangas and Koivula1964) also stated that ‘hard-shelled embryos of mature females elliptical, with middle membrane slightly evaginated at poles.’ Such ‘evagination’ was not evident in our Italian specimens, nor has it been reported elsewhere.
• Gupta & Gupta (Reference Gazi, Kim, García-Varela, Park, Littlewood and Park1972) studied one male worm from Milvus migrans in Chandigarh, India, and also reported four cement glands, which has been confirmed by Dezfuli et al. (Reference Dezfuli, Rubini, DePasquale and Pironi2020) on histological grounds. It is easy to confuse the number of cement glands as they often appear to coalesce in one thick cord.
• Bhattacharya (Reference Bhattacharya2000) examined one male worm from Ottus sp. in Tripura, India, and reported ‘2 or 3’ cement glands. Bhattacharya (Reference Bhattacharya2007) later reported four cement glands (see above).
• Bhattacharya (Reference Bhattacharya2000) also reported that second, third and fifth hooks from anterior are ‘strongest.’ This may be true for the fifth hook, but not likely the second or third.
• Gupta & Gupta (Reference Gazi, Kim, García-Varela, Park, Littlewood and Park1972) created a very confused presentation of their work marred by erroneous interpretation of ordinary anatomical structures. They noted that the trunk is aspinose, but indicate that the neck is ‘short, rectangular and measures 0.180 × 0.378 mm. It is armed with 30 longitudinal rows of spines and 5–6 spines in each row.’ Their Fig. 14 shows the PR inserting at the anterior end of their ‘neck.’ The authors clearly confused the posterior proboscis with a neck; not a common mistake.
• Gupta & Gupta (Reference Gazi, Kim, García-Varela, Park, Littlewood and Park1972) further indicated that the posterior testis could not be measured because it was ‘distorted.’ However, their Fig. 13 clearly delineated a posterior testis as distinctly outlined as the anterior testis.
• Dimitrova et al. (Reference Dimitrova, Murai and Gerogiev1995) examined one male from Falco cherrug Gray in Hungary and reported 8–9 anterior hooks, with anteriormost hooks unusually long, 2–3 transitional hooks and 12–13 posterior spiniform hooks. Their disproportional and inaccurate Fig. 2, however, shows 9–10 hooks/row on an atypically spheroid anterior proboscis and only 2–5 spiniform hooks/row on the posterior proboscis.
• Dimitrova & Gibson (Reference Dimitrova and Gibson2005) examined only two female specimens. They indicated that ‘posterior trunk terminates in rounded conical papilla,’ but their Figs 6b and 2c of two female posterior ends show no such papillae. They also report ‘vagina with 2 sphincters.’ We find three vaginal sphincters.
Micropores
The micropores of C. globocaudatus, like those reported from other species of the Acanthocephala, are associated with internal crypts and vary in diameter and distribution in different trunk regions corresponding to differential absorption of nutrients. We have reported micropores in a large number of acanthocephalan species (Heckmann et al., Reference Heckmann, Amin, Radwan, Standing, Eggett and El Naggar2013) and in a few more since, and demonstrated the tunnelling from the cuticular surface into the internal crypts by transmission electron microscopy. Amin et al. (Reference Amin, Heckmann, Radwan, Mantuano and Alcivar2009) gave a summary of the structural–functional relationship of the micropores in various acanthocephalan species including Rhadinorhynchus ornatus Van Cleave, 1918, Polymorphus minutus (Goeze, 1782) Lühe, 1911, Moniliformis moniliformis (Bremser, 1811) Travassos (1915), Macracanthorhynchus hirudinaceus (Pallas, 1781) Travassos (1916, 1917) and Sclerocollum rubrimaris Schmidt & Paperna, 1978. Wright & Lumsden (Reference Whitfield1969) and Byram & Fisher (Reference Byram and Fisher1973) reported that the peripheral canals of the micropores are continuous with canalicular crypts. These crypts appear to ‘constitute a huge increase in external surface area . . . implicated in nutrient up take.’ Whitfield (Reference Ward1979) estimated a 44-fold increase at a surface density of 15 invaginations per 1 μm2 of M. moniliformis (Bremser, 1811) Travassos, 1915 tegumental surface. The micropores and the peripheral canal connections to the canaliculi of the inner layer of the tegument of Corynosoma strumosum (Rudolphi, 1802) Lühe, 1904 from the Caspian seal Pusa caspica (Gmelin) in the Caspian Sea were demonstrated by transmission electron micrographs in Amin et al. (Reference Amin, Heckmann, Halajian and El-Naggar2011).
EDXA
Results of the X-ray scans of the Ga-cut hooks (dual-beam SEM) of C. globocaudatus show differential composition and distribution of metals in different hook parts, with calcium and phosphorus levels being highest at the middle of hook tip and mid cuts, as well as at the basal arch of hooks where tension and strength are paramount for hook function. The sulphur levels were very low throughout but relatively higher at the edge of hook tip cuts (table 4 and fig. 6). The chemical elements present in the hooks are typical for acanthocephalans (Heckmann et al., Reference Gupta and Gupta2007, Reference Heckmann, Amin and Standing2012). Note the moderate outer layer (fig. 6) of the hook that relates to the sulphur content in the hook of C. globocaudatus, which is different than in other acanthocephalans. The high sulphur content shows up in the outer edge of X-ray scans of hooks (tables 4 and 5; Amin et al., Reference Amin, Heckmann and Bannai2018). The hook centre in mid cuts has a different chemical profile than the cortical layer (table 4). The distribution of metals varied along the longitudinal axis of hooks on the proboscis, with apical and middle hooks having the highest levels of phosphorous and calcium but the lowest levels of sulphur compared to basal hooks (table 5 and figs 8, 9). The increased level of phosphorous (9.01% weight) in the developing embryo at the centre of eggs but not in its cortical layer (1.24) is noteworthy (table 6). Amin & Larsen (Reference Amin and Larsen1989) found high levels of phospholipids in the core and nucleus of ripe eggs but none in the unripe eggs or ovarian balls of Neoechinorhynchus cylindratus (Van Cleave, 1913) Van Cleave, 1919. Sheema (Reference Santoro, Tripepi, Kinsella, Panebianco and Mattiucci2018) reported intense acid phosphate activity in the eggs of Echinorhynchus veli George & Nadakal, 1978 in agreement with the histochemical observations of Amin & Larsen (Reference Amin and Larsen1989), Crompton (Reference Crompton1963) in P. minutus (Goeze, 1782) Lühe, 1911 and Cain (Reference Cain1970) in M. hirudinaceus (Pallas, 1781) Travassos, 1917. Sheema (Reference Santoro, Tripepi, Kinsella, Panebianco and Mattiucci2018) noted intense phosphatase activity in eggs associated with accelerated cellular metabolism. Anantaraman & Ravindranath (Reference Anantaraman and Ravindranath1976) observed that the protein in the wall of the acanthor of Acanthosentis sp. was rich in aromatic and sulphur-containing amino acids, which agrees with our observations in table 5.
X-ray scans (EDXA) provide insight into the hardened components – for example, calcium, sulphur and phosphorus – of acanthocephalan hooks. The EDXA appears to be species specific, as in fingerprints. For example, EDXA is shown to have significant diagnostic value in acanthocephalan systematics – for example, Moniliformis cryptosaudi Amin, Heckmann, Sharifdini & Albayati, 2019 was erected based primarily on its EDXA pattern (Amin et al., Reference Amin, Heckmann, Sharifdini and Albayati2019b). Our methodology for the detection of the chemical profile of hooks in the Acanthocephala has also been used in other parasitic groups including the Monogenea (Rubtsova et al., Reference Rubtsova and Heckmann2018; Rubtsova & Heckmann, Reference Rainboth2019) and Cestoda (Rubtsova & Heckmann, Reference Rubtsova and Heckmann2020). We also provide chemical and molecular data to explain and clarify our findings.
Molecular discussion
The results of phylogenetic analyses further support the description of the material described herein as C. globocaudatus. Indeed, present sequences unequivocally group with members of the same species published by García-Varela et al. (Reference García-Varela, Pérez-Ponce de León, de la Torre, Cummings, Sarma and Laclette2020a) in the 18S phylogram. Unfortunately, no other sequences of this species are available to perform comparisons among different populations by cox1 gene.
Although low bootstrap values are observed in most nodes of the SSU gene-based tree, due to low genetic signal, DNA sequence identity, determined according the alignment average distance under the p-distance model, yielded a value of 0.05 (95% identity). Therefore, alignment accuracy is, by far, high enough to build a reliable phylogenetic tree (Kumar & Filipski, Reference Komorová, Špakulová, Hurníková and Uhrín2006).
Actually, the SSU gene would be more adequate to address inter-generic or inter-familiar phylogenetic relationships rather than interspecific ones, as is the case. Unfortunately, as already noted by Steinauer et al. (Reference Sheema2019), the study of the relationships among centrorhynchid taxa based on molecular data is difficult due to the low number of sequences available – in particular, no SSU gene sequences are available for other genera of Centrorhynchidae. Thus, there is a great lack of information on the genus under study and any additional phylogeny that could be provided for Centrorhynchus can be valuable; this is especially true in the present case, in which a new geographical variant characterized by novel morphological aspects is described.
Outcomes of phylogenetic analyses based on mtDNA data (Muhammad et al., Reference Muhammad, Ma, Khan, Li, Zhao, Ahmad and Zhu2020) suggest that Sphaerirostris is a sister group of Centrorhynchus aluconis, conforming a sub-clade within Centrorhynchus, which would, therefore, be a paraphyletic taxon. Our results seem to agree with this suggestion; however, the weak bootstrap support and the nucleotide differences associated to these relationships in phylogenetic analyses hamper its confirmation. In addition, the genera Centrorhynchus and Sphaerirostris have been well differentiated based on morphology (see Table 1 in Amin & Canaris, Reference Amin and Canaris1997). Specifically, one of the clearest diagnostic features allowing discrimination between both genera, the lacunar system pattern, is recognized as one of the most important taxonomic criteria in the classification of the Acanthocephala at the generic and higher levels (Amin & Canaris, Reference Amin and Canaris1997). The short spindle-shaped trunk and short globular anterior proboscis in Sphaerirostris clearly distinguish it from Centrorhynchus, with a long cylindrical trunk and long cylindrical anterior proboscis, among other features.
The two cox1 available sequences of Sphaerirostris lanceoides were obtained from cystacanths present in amphibians and ardeid birds (i.e. Ardeola grayii), respectively (Kang & Li, Reference Huelsenbeck, Ronquist, Nielsen and Bollback2018; Muhammad et al., Reference Muhammad, Ma, Khan, Li, Zhao, Ahmad and Zhu2020), and the only cox1 available sequence of Sphaerirostris picae was obtained from adults infecting the corvid Dendrocitta vagabunda (see Muhammad et al., Reference Miller, Pfeiffer and Schwartz2019). Indeed, while Sphaerirostris mostly infects passerine birds, Centrorhynchus mainly infects birds of prey instead. Therefore, ecological associations between parasites and their hosts further support morphological studies in marking a differentiation between these two centrorhynchid genera. Interestingly, a study based on 18S DNA gene analysis revealed the relatively close relation of S. picae with Plagiorhynchus cylindraceus (Goeze, 1782) (Plagiorhynchidae), even more than with other species of the genus Centrorhynchus (Radwan, Reference Petrochenko2012). However, 18S and 28S sequences obtained by Radwan (Reference Petrochenko2012) were too short, from our point of view, to yield fully reliable phylogenetic affinities (361 and 281 bp); thus, they were not included in present analyses. Apart from these ones, no 18S sequences have been made available for S. picae or other Sphaerirostris members up to date, which would have helped better elucidate their phylogenetic relationship to Centrorhynchus representatives.
In conclusion, phylogenetic relationships within the Centrorhynchidae remain unsolved. It becomes clear that more Sphaerirostris sequences obtained from well-characterized adult and properly identified specimens, as well as genetic data of the third genus within the family, Neolacunisoma, are needed in order to clarify phylogenetic associations within the Centrorhynchidae.
Acknowledgements
We are indebted to Dr Bahram S. Dezfuli, University of Ferrara, Italy, for meticulous coordination of the Italian operation run by S. Rubini at the Experimental Zooprophylactic Institute of Ferrara, where recovery of parasites took place with the US researchers. We thank Dr Nataliya Rubtsova (Parasitology Center, Inc.) and Madison Laurence, Bean Museum (Brigham Young University), Provo, Utah, for their expert help in the preparation and organization of plates and figures, and Michael Standing, Electron Optics Laboratory (Brigham Young University), for his technical help and expertise.
Financial support
This project was supported by an institutional grant from the Biology Department, Brigham Young University, Provo, Utah, and the Parasitology Center, Inc., Scottsdale, Arizona.
Conflicts of interest
None.
Ethical standards
The authors declare that they have observed all applicable ethical standards..