Introduction
The reticulorumen offers a perfect environment for anaerobic bacteria, protozoa, and fungi to break down plant fibre carbohydrates to short-chain fatty acids (SCFA) (Nagaraja, Reference Nagaraja, Millen, de Beni Arrigoni and Lauritano Pacheco2016), the major energy source for ruminants (Bergman, Reference Bergman1990). The SCFA produced are mainly acetate, propionate and butyrate, in proportions of roughly 70:20:10, respectively (Dieho et al., Reference Dieho, Dijkstra, Schonewille and Bannink2016). Around 50–85% of the SCFA produced intraruminally are directly absorbed across the ruminal wall, i.e. the ruminal epithelium (Aschenbach et al., Reference Aschenbach, Penner, Stumpff and Gäbel2011). This illustrates the fundamental role of the epithelium for ruminal SCFA absorption in order to maintain health and energy balance of the animal. In addition, epithelial absorption of nutrients is also an important factor in preserving the delicate balance between SCFA as an energy source and their detrimental acidifying effects.
Besides its central role in nutrient absorption, another principal task of the reticuloruminal epithelium is sustaining the barrier against potential noxious substances in the lumen. As the forestomach system is one of the most proximal parts of the ruminant gastrointestinal tract, it is in contact not only with valuable nutrients, but also with harmful substances like bacterial toxins, amines derived from silages or excessive amounts of SCFA (Phuntsok et al., Reference Phuntsok, Froetschel, Amos, Zheng and Huang1998; Aschenbach et al., Reference Aschenbach, Penner, Stumpff and Gäbel2011; Guo et al., Reference Guo, Chang, Zhang, Xu, Jin, Bilal and Shen2017). To prevent damage of the whole organism, the microorganisms in the lumen as well as the epithelium itself exert certain mechanisms to render these substances innocuous.
Thus, the ruminal epithelium is a lynchpin for the preservation of ruminants' health and productivity. Effective transport of fermentation products is fundamental to maintain ruminal homoeostasis and thereby prevent acidosis, ruminal epithelial damage and thus intoxication of the whole animal. The current state of knowledge on these crucial functions will be reviewed.
Transport of SCFA across the epithelium
In order to balance pH on the one hand and to absorb the emerging products of microbial fermentation on the other hand, efficient mechanisms for SCFA transport are crucial for intraruminal as well as epithelial health, as shown in Figure 1. Due to the pK of ~4.8, 90–99% of SCFA are dissociated and thereby present as SCFA-anions (SCFA−) and H+, while only 1–10% are undissociated (HSCFA) at a physiological ruminal pH of 5.8–6.8 (Bergman, Reference Bergman1990). HSCFA may diffuse passively through the lipid bilayers along their concentration gradient (Fig. 1, route ①). However, not only because of the low amount of HSCFA, the significance of lipophilic transfer has to be questioned. Gäbel et al. (Reference Gäbel, Aschenbach and Müller2002) and Aschenbach et al. (Reference Aschenbach, Bilk, Tadesse, Stumpff and Gäbel2009) described no or only a minor influence of chain length, i.e. lipophilicity, on SCFA disappearance, thus contradicting a significant diffusion of HSCFA. A decline in pH, i.e. increasing [HSCFA], resulted in higher absorption rates of SCFA, but the effect was inconsistent and smaller than predicted by the Henderson–Hasselbalch equation (Dijkstra et al., Reference Dijkstra, Boer, van Bruchem, Bruining and Tamminga1993; Sehested et al., Reference Sehested, Diernæs, Møller and Skadhauge1999a; Gäbel et al., Reference Gäbel, Aschenbach and Müller2002). At the basolateral side, lipophilic diffusion seems even less likely, as almost 100% of SCFA are dissociated at a cytosolic pH of 7.4.

Fig. 1. Transport processes across the ruminal epithelium. By the current state of knowledge, SCFA are absorbed from the lumen via a number of different routes: ① passive diffusion, ② nitrate-sensitive anion exchangers, ③ HCO3−-exchangers, ④ MCT4, and an ⑤ anion channel. Their basolateral efflux is mediated by ⑥ MCT1, ③ HCO3−-exchangers, as well as an ⑤ anion channel. The latter may be driven by the ⑦ Na+/K+-ATPase. Occurring H+ can be extruded via ⑧ NHE and MCT1, while HCO3−-exchangers on the apical and basolateral side ensure buffer supply to the epithelium and lumen. For clarity only one cell layer is depicted, but this represents the multi-layered, stratified squamous epithelium.
All these results point to the existence of other, protein-mediated transport mechanisms for SCFA−. Several findings suggested anion exchangers as a promising group of proteins to transfer SCFA− across the apical membrane of the epithelium in an electroneutral manner. Uptake of acetate and especially propionate (butyrate to a smaller degree) has been shown to correlate negatively with the disappearance of chloride from the mucosal side and to be reduced by mucosal addition of nitrate under different incubation conditions (Fig. 1, route ②) (Aschenbach et al., Reference Aschenbach, Bilk, Tadesse, Stumpff and Gäbel2009). The same study revealed that acetate uptake is essentially driven in a HCO3−-dependent manner (Fig. 1, route ③). An increase in acetate concentration resulted in an increased acetate uptake, especially in HCO3−-containing solutions, indicating that the exchange of HCO3− and acetate is driven by the concentration gradient for acetate and might even counteract opposing HCO3− concentration gradients due to a higher impact of the acetate concentration gradient on transport activities (Aschenbach et al., Reference Aschenbach, Bilk, Tadesse, Stumpff and Gäbel2009). The authors hypothesized that, at lower ruminal pH, the increase of acetate uptake is not due to an increase of HSCFA, but to an additional driving force for HCO3− into the ruminal lumen. Net butyrate absorption was also proven to be HCO3−-dependent (Sehested et al., Reference Sehested, Diernæs, Møller and Skadhauge1999b). An early study by Gäbel et al. (Reference Gäbel, Bestmann and Martens1991) already demonstrated a great stimulatory impact of SCFA present in the lumen on HCO3− secretion, while the absence of SCFA reversed HCO3− movement to a net absorption. Regarding the basolateral extrusion of SCFA, the existence of anion exchangers is supported by Ussing chamber studies that detected a large part of the SCFA transport from the blood to the lumen to be sensitive to a serosal addition of nitrate (Dengler et al., Reference Dengler, Rackwitz, Benesch, Pfannkuche and Gäbel2014).
Thus, anion exchange mechanisms seem to play a role both for the uptake of SCFA into the epithelium as well as the extrusion into the blood. For these anion exchange mechanisms, several proteins have to be taken into account: Bilk et al. (Reference Bilk, Huhn, Honscha, Pfannkuche and Gäbel2005) demonstrated the expression of anion exchanger 2 (AE2), downregulated-in-adenoma (DRA) and putative anion exchanger 1 (PAT1) as potential candidates for a SCFA-anion-exchange across the ruminal epithelium on mRNA level. Stumpff (Reference Stumpff2018) proposed DRA and PAT1 to be located in the apical, and AE2 and Na+/HCO3−-cotransporter (NBC) in the basolateral ruminal membrane. However, in immunohistochemical analyses, PAT1 could be detected in the basolateral layers of the ruminal epithelium (Omer, Reference Omer2016). However, even though several studies detected a significant influence of ruminal parameters like pH and SCFA concentration on gene expression of PAT1, AE2 and DRA (Yan et al., Reference Yan, Zhang and Shen2014; Dengler et al., Reference Dengler, Rackwitz, Benesch, Pfannkuche and Gäbel2015), functional evidence that clearly shows the specific role of the various candidates in SCFA transport and their side-specificity is still lacking.
Another protein that was detected in the basolateral membrane immunohistochemically is the monocarboxylate transporter 1 (MCT1, Fig. 1, route ⑥), which works as a symporter with H+ and/or as an antiporter with HCO3− (Kirat et al., Reference Kirat, Masuoka, Hayashi, Iwano, Yokota, Taniyama and Kato2006; Dengler et al., Reference Dengler, Rackwitz, Benesch, Pfannkuche and Gäbel2014). It has been shown to transport SCFA-metabolites like lactate, β-hydroxybutyrate and acetoacetate (Müller et al., Reference Müller, Huber, Pfannkuche, Aschenbach, Breves and Gäbel2002), but also SCFA themselves, especially acetate and propionate (Kirat et al., Reference Kirat, Masuoka, Hayashi, Iwano, Yokota, Taniyama and Kato2006; Dengler et al., Reference Dengler, Rackwitz, Benesch, Pfannkuche and Gäbel2014). By doing so, it not only assures maintenance of intracellular osmolarity, but also alkalizes the cytosol and prevents epithelial acidosis. Kirat et al. (Reference Kirat, Masuoka, Hayashi, Iwano, Yokota, Taniyama and Kato2006) also detected a reduction of acetate transport from the lumen to the blood side, when they applied an MCT inhibitor to the mucosal buffer solution in vitro, but less pronounced compared to the serosal addition of the inhibitor. In a follow-up study, they demonstrated MCT4 (Fig. 1, route ④) in the apical membrane of the ruminal epithelium by immunofluorescence staining and postulated that it takes up SCFA− from the lumen in symport with H+ (Kirat et al., Reference Kirat, Matsuda, Yamashiki, Hayashi and Kato2007). In contrast to these findings, Aschenbach et al. (Reference Aschenbach, Bilk, Tadesse, Stumpff and Gäbel2009) did not find an influence of MCT inhibitors on the apical uptake of acetate. If MCT4 contributed substantially to apical SCFA− uptake, it would thereby also lead to an increase of luminal pH, while the concentration of H+ in the epithelium would rise. A similar transport, but without direct influence on pH, was postulated by Rackwitz (Reference Rackwitz2012) who detected functional evidence for an involvement of Na+-coupled MCT1 (SMCT1) in SCFA transport, especially of propionate and butyrate.
Whilst all of these pathways (except SMCT1) mediate an electroneutral SCFA uptake, a minor part of SCFA seems to be transported electrogenically (Fig. 1, route ⑤). Sehested et al. (Reference Sehested, Diernæs, Møller and Skadhauge1999b) could show in Ussing chamber studies that 30% of the butyrate transport from the lumen to the blood side was voltage dependent, ergo electrogenic. The authors concluded that an anion channel may be involved which might not play a quantitative role in vivo. In addition, both in cultured ruminal epithelial cells and in intact ruminal epithelial tissue, an acetate conductance (and in the case of cultured cells also a propionate and butyrate conductance) was detected (Stumpff et al., Reference Stumpff, Martens, Bilk, Aschenbach and Gäbel2009; Georgi et al., Reference Georgi, Rosendahl, Ernst, Günzel, Aschenbach, Martens and Stumpff2014; Rackwitz and Gäbel, Reference Rackwitz and Gäbel2018). Whereas Rackwitz and Gäbel (Reference Rackwitz and Gäbel2018) found evidence for an apical localization of this channel, Georgi et al. (Reference Georgi, Rosendahl, Ernst, Günzel, Aschenbach, Martens and Stumpff2014) postulate a basolateral occurrence of this anion conductance. Besides maxi-anion channels (Georgi et al., Reference Georgi, Rosendahl, Ernst, Günzel, Aschenbach, Martens and Stumpff2014), Rackwitz (Reference Rackwitz2012) also proposed an involvement of outwardly rectified Cl− channels. Regardless of its contribution in vivo, an apical localization of this channel would lead to an uptake of SCFA− while epithelial pH is not influenced. This may serve as an epithelial protection mechanism under luminally acidotic conditions (Rackwitz and Gäbel, Reference Rackwitz and Gäbel2018). Basolateral electrogenic anion transport in contrast could keep up electroneutrality of the epithelium and may be driven by the electrical gradient established by the basolateral Na+/K+-ATPase (Fig. 1, route ⑦).
All the mechanisms described above (except SMCT1 and electrogenic uptake of SCFA−) significantly contribute to an alkalinization of the ruminal content either due to coupling of SCFA absorption with HCO3− secretion or concomitant disappearance of H+. HCO3− secretion across the ruminal wall becomes even more important as more and more SCFA are produced. In high production dairy cows, the HCO3− secretion across the ruminal wall induced by SCFA uptake roughly equals salivary HCO3− delivery (approx. 30 M/d) (Allen, Reference Allen1997). Although SCFA− uptake both by anion exchange mechanisms or by MCT and HSCFA absorption leads to an alkalinization of the ruminal lumen, it strongly acidifies the epithelial cytosol either due to a loss of HCO3− or uptake of H+. Thus, a plethora of alkalizing mechanisms were detected in the ruminal epithelium. Intracellular acidification can be neutralized either by HCO3− uptake on the basolateral side or by H+ extrusion via Na+/H+-exchangers (NHE, Fig. 1, route ⑧) or MCT1 at the basolateral membrane (Müller et al., Reference Müller, Huber, Pfannkuche, Aschenbach, Breves and Gäbel2002; Penner et al., Reference Penner, Steele, Aschenbach and McBride2011). Basolateral anion exchangers could provide the HCO3− supply from the blood to deliver buffer not only to the epithelial cytosol but also for anion exchange mechanisms in the apical membrane. The extrusion of SCFA to the blood stream delivers nutrients to the organism whilst osmotic destabilization of the ruminal epithelium is prevented, thus it is equally important as their apical uptake. However, whereas the apical uptake of SCFA has been studied intensively, basolateral efflux is not yet understood to the same degree.
Summing up the findings on ruminal SCFA transport, the last decades have given further insight into different uptake pathways for SCFA, illustrating a broad set of diverse absorptive functions. This variety of transporters probably works redundantly, and their specific role in vivo is still not completely clear. Yet, it is likely that several mechanisms still need to be identified.
Catabolism of SCFA in the ruminal epithelium and its advantages
Intraepithelial break-down of SCFA serves several purposes: first of all, it guarantees the alimentation of the epithelium itself, which in contrast to most other tissues prefers SCFA, especially butyrate, as an energy source instead of glucose or glutamine (Britton and Krehbiel, Reference Britton and Krehbiel1993; Kristensen and Harmon, Reference Kristensen and Harmon2004a). Secondly, it maintains the concentration gradient for SCFA across the epithelium to ensure luminal uptake, while the produced metabolites (mainly ketones) also serve as energy fuels for peripheral tissues (Gäbel et al., Reference Gäbel, Aschenbach and Müller2002). Moreover, these metabolites may be exported via additional extrusion pathways to keep intracellular osmolarity stable, thus preventing an influx of water and consequently cell death by osmotic rupture. Finally, the catabolism of these acids prevents possibly harmful effects of excessive SCFA accumulation, in particular butyrate, as described at the end of this section.
As shown by Nozière et al. (Reference Nozière, Martin, Rémond, Kristensen, Bernard and Doreau2000) in portal recovery experiments, SCFA break-down in ovine ruminal epithelium comprises up to 16, 33 and 70% of the acetate, propionate and butyrate, respectively, taken up on the luminal side. Kristensen and Harmon (Reference Kristensen and Harmon2004b) assumed an even smaller proportion for propionate catabolism in studies in sheep. They also hypothesized that acetate might additionally derive from metabolism of butyrate and valerate, thus even more acetate might be metabolized than postulated by Nozière et al. (Reference Nozière, Martin, Rémond, Kristensen, Bernard and Doreau2000). This is in agreement with earlier studies, indicating an endogenous production of acetate, as in vitro transport studies show that (if chemically measured) it appears in a much higher degree on the blood side than the tracer-marked acetate applied on the lumen side (Sehested et al., Reference Sehested, Diernæs, Møller and Skadhauge1999a). The same study revealed a high degree of metabolization of propionate and butyrate.
The differences in metabolism rates of the SCFA are based on the one hand presumably on different activating acyl-CoA-enzymes for the individual SCFA and their metabolites (Bond et al., Reference Bond, Donaldson, Coumans, Austin, Ebert, Wheeler and Oddy2019), and on the other hand on different pathways for the diverse SCFA: while acetate and butyrate enter the Krebs cycle to be oxidized to CO2 (acetate) or ketones via β-oxidation and acetoacetyl-CoA (butyrate), propionate may be metabolized completely in Krebs cycle to CO2 or via malate to pyruvate and lactate. As a consequence of the different metabolic pathways, the metabolites produced differ between the three SCFA. Regarding the anaerobic pathways, propionate was shown to be degraded mostly to lactate, while butyrate delivers primarily ketones like β-hydroxybutyrate and acetoacetate (Kristensen and Harmon, Reference Kristensen and Harmon2004b). Concerning the aerobic pathways, a large production of CO2 by SCFA metabolism was detected for all three major SCFA when applied on the mucosal side (Sehested et al., Reference Sehested, Diernæs, Møller and Skadhauge1999a). Penner et al. (Reference Penner, Steele, Aschenbach and McBride2011) postulated that ketogenesis predominantly takes place in the basal layers, as most of the mitochondria were detected in these structures (Graham and Simmons, Reference Graham and Simmons2005).
As mentioned above, deficiency of this SCFA catabolism might provoke epithelial damage due to changes in intracellular pH and osmolarity. In the case of butyrate, additional effects must be expected, since butyrate has both systemic and local genomic effects. In vivo experiments delivered evidence for an induction of cell proliferation by a rapid butyrate administration (Sakata and Tamate, Reference Sakata and Tamate1978). In cell culture experiments, butyrate provoked increased apoptosis in epithelial ruminal cells (Neogrády et al., Reference Neogrády, Gálfi, Kutas and Sakata1989). Additionally, even though positive effects on papillae growth could be induced in feeding trials with butyrate supplementation (Malhi et al., Reference Malhi, Gui, Yao, Aschenbach, Gäbel and Shen2013), increased parakeratosis is found under acidotic conditions (Plaizier et al., Reference Plaizier, Danesh Mesgaran, Derakhshani, Golder, Khafipour, Kleen, Lean, Loor, Penner and Zebeli2018) which might be caused by increased butyrate concentrations. In the case of an overproduction of n-butyric acid by the ruminal microorganisms, the epithelial capacity to metabolize butyrate efficiently seems to be reduced (Kristensen et al., Reference Kristensen, Pierzynowski and Danfaer2000), presumably leading to the proliferative alterations observed. Thus, a balanced ruminal SCFA production from feed in line with the epithelial catabolic capacities is pivotal to ruminal health.
Acidosis and ruminal epithelium
These functions of the ruminal epithelium in absorption and catabolism of the SCFA as well as buffer secretion underline its crucial role in the prevention of homoeostatic imbalances in the rumen. When the SCFA production exceeds their absorption and catabolism and/or the aboral efflux of the acids as well as the buffering via saliva and epithelial HCO3− secretion, acidotic conditions occur (Humer et al., Reference Humer, Aschenbach, Neubauer, Kröger, Khiaosa-Ard, Baumgartner and Zebeli2018). As explained above, the epithelium alkalizes the ruminal lumen by absorbing H+ (via HSCFA or MCT) as well as secreting HCO3−. With increasing SCFA concentration, the epithelium also turns acidic due to the enhanced loss of HCO3− and uptake of H+ (Müller et al., Reference Müller, Aschenbach and Gäbel2000). This leads to severe damage of the epithelial lining and development of ruminitis (Steele et al., Reference Steele, Penner, Chaucheyras-Durand and Le Guan2016). In extreme situations, it is no longer able to fulfil its role in alkalinization of the ruminal lumen, as well as the catabolism of absorbed SCFA and detoxification of potential noxious substances (Aschenbach et al., Reference Aschenbach, Oswald and Gäbel2000; Kristensen et al., Reference Kristensen, Pierzynowski and Danfaer2000). As a result of missing luminal alkalinization, the microbial composition shifts, leading to elevated levels of D-lactate, which is not taken up in exchange for HCO3− and hardly metabolized in the ruminal epithelium where it accumulates (Gäbel et al., Reference Gäbel, Bestmann and Martens1991; Allen, Reference Allen1997; Snyder and Credille, Reference Snyder and Credille2017). This provokes a vicious circle as the lumen turns even more acidotic and osmotic balance of the lumen and the epithelium is endangered. The fatal combination of corrosive lactate accumulation and the osmotic disbalance due to an increase of SCFA and their metabolites in the ruminal epithelium finally leads to a disruption of the epithelium (Snyder and Credille, Reference Snyder and Credille2017) aggravating the situation and leading to clinical acidosis. While exact limits of physiological rumen pH cannot be defined due to differences according to the sampling site and individual susceptibility, acute clinical acidosis often becomes manifest when ruminal pH drops below a certain threshold due to the aforementioned accumulation of D-lactate, involving symptoms like a distended rumen, diarrhoea, anorexia, neurologic symptoms etc. (Snyder and Credille, Reference Snyder and Credille2017).
Far more common than acute clinical acidosis and often undiscovered clinically, is the condition known as subacute ruminal acidosis (SARA). According to Humer et al. (Reference Humer, Aschenbach, Neubauer, Kröger, Khiaosa-Ard, Baumgartner and Zebeli2018), SARA can be defined as a drop of pH below approximately 5.5–5.8 for several hours per day, with elevated levels of SCFA and a rise in luminal osmolarity while the physical examination is inconspicuous. Humer et al. (Reference Humer, Aschenbach, Neubauer, Kröger, Khiaosa-Ard, Baumgartner and Zebeli2018) reported that 11–26% of dairy cattle are considered to suffer from this form of acidosis, but it might often be undiscovered, especially in early lactation. However, SARA leads to pain and discomfort in the animals and, economically important, to a depression of milk yield (Humer et al., Reference Humer, Aschenbach, Neubauer, Kröger, Khiaosa-Ard, Baumgartner and Zebeli2018). To prevent acidotic digestive disorders, specific precautions should be exercised when calculating feed rations for high producing animals, but not all individuals will respond to the same diet similarly, as indicated by a large spectrum of pH responsiveness to excessive amounts of rapidly fermentable carbohydrates detected in sheep (Penner et al., Reference Penner, Aschenbach, Gäbel, Rackwitz and Oba2009). Even though the mechanisms of this individual susceptibility still need to be elucidated, the impact of SCFA absorption across the ruminal epithelium on rumen health and likewise on maintenance of the animal's health can be derived from this study: sheep with a higher SCFA absorption capacity were found to be less likely to suffer from an artificially induced SARA than sheep with a lower absorption rate (Penner et al., Reference Penner, Aschenbach, Gäbel, Rackwitz and Oba2009) which again emphasizes the role of ruminal epithelium in the prevention of systemic illness and will be discussed in the next section.
Adaptation capacities of the ruminal epithelium
Dairy cattle diets often involve feeding highly fermentable carbohydrates to ensure animal performance. In the young calf, this requires the development of the monogastric digestive system into a mature forestomach system. Changes of the microbiota, as well as structural and functional transformation of the ruminal wall induced by solid feed intake instead of milk, lead to an increased rumen size of growing calves (Steele et al., Reference Steele, Penner, Chaucheyras-Durand and Le Guan2016; Yohe et al., Reference Yohe, Schramm, Parsons, Tucker, Enger, Hardy and Daniels2019) accompanied by higher, wider and also denser ruminal papillae (Warner et al., Reference Warner, Flatt and Loosli1956; Tamate et al., Reference Tamate, McGilliard, Jacobson and Getty1962). Increased particle size, often modulated by inclusion of forage into the diet early in life, enhances total rumen growth and muscularization (Warner et al., Reference Warner, Flatt and Loosli1956; Mirzaei et al., Reference Mirzaei, Khorvash, Ghorbani, Kazemi-Bonchenari, Riasi, Nabipour and van den Borne2015). Additionally, the chemical composition of feed (usually concentrate in starter diets) seems to be crucial for the growth of ruminal papillae and epithelium (Warner et al., Reference Warner, Flatt and Loosli1956; Sander et al., Reference Sander, Warner, Harrison and Loosli1959). SCFA, and primarily butyrate and propionate, have been shown to stimulate neonatal papillae growth, including an increased epithelial cell proliferation leading to a thicker epithelial layer (Sander et al., Reference Sander, Warner, Harrison and Loosli1959; Yohe et al., Reference Yohe, Schramm, Parsons, Tucker, Enger, Hardy and Daniels2019).
This plasticity of the ruminal epithelium is still observable in adult ruminants under different feeding conditions. However, even though the well-developed adult ruminal epithelium can adjust to a great extent, a change of diet that is introduced too rapidly or too drastically might still overburden this adaptive ability. Various studies in vivo as well as in vitro indicate a high potential of the ruminal wall to adjust to different feeding situations: whereas a reduction or deprivation of feed leads to a dramatically diminished SCFA absorption across the epithelium already within 2 to 5 d (Gäbel et al., Reference Gäbel, Marek and Martens1993; Zhang et al., Reference Zhang, Albornoz, Aschenbach, Barreda and Penner2013), adjustment to increased amounts of feed or changes in feed composition seems to occur on the cellular as well as on the epithelial level. Within one week, changes of absorptive capacities for electrolytes and SCFA are detected without noticeable differences in epithelial morphology like epithelial surface area (Etschmann et al., Reference Etschmann, Suplie and Martens2009; Schurmann et al., Reference Schurmann, Walpole, Górka, Ching, Loewen and Penner2014). This is particularly apparent when high concentrate is fed once daily in comparison to constant feeding during the day (Sehested et al., Reference Sehested, Basse, Andersen, Diernæs, Møller, Skadhauge and Aaes1997). Changes in electrophysiological properties and the permeability of the ruminal wall indicate that enhanced HCO3−-dependent uptake is the main mechanism of this early functional adaptation for SCFA absorption, while increased passive diffusion was also observed (Klevenhusen et al., Reference Klevenhusen, Hollmann, Podstatzky-Lichtenstein, Krametter-Frötscher, Aschenbach and Zebeli2013; Schurmann et al., Reference Schurmann, Walpole, Górka, Ching, Loewen and Penner2014).
Besides these functional alterations (presumably at the cellular level) without detectable variations of epithelial structure, more conspicuous morphological modifications may also take place. These are usually seen later on and reach peak levels roughly 6 weeks after the change of diet (Etschmann et al., Reference Etschmann, Suplie and Martens2009). Similar to the development in calves, but probably less complex concerning their morphological and functional impact, the increased intake of easily fermentable carbohydrates leads to an increase of papillae growth and total surface area in adult ruminants (Gäbel et al., Reference Gäbel, Martens, Sündermann and Gálfi1987). An elevated mRNA expression of transporters for SCFA absorption and pH homoeostasis as well as changes in membrane composition (and thereby increasing epithelial permeability) occurred after several weeks of moderate concentrate diet or a supplementation with saturated lipids, respectively (Yan et al., Reference Yan, Zhang and Shen2014; Verdugo, Reference Verdugo2016). Additionally, a modification of uptake pathways rather than increasing total SCFA absorption 4 weeks after the alterations in feed composition were noticed (Chibisa et al., Reference Chibisa, Gorka, Penner, Berthiaume and Mutsvangwa2015). Finally, increases in splanchnic blood flow, SCFA absorption and subsequently liver metabolism can be induced by elevating the SCFA concentration in the rumen due to dietary changes during transition (Reynolds et al., Reference Reynolds, Aikman, Lupoli, Humphries and Beever2003; Storm et al., Reference Storm, Hanigan and Kristensen2011). When fed moderate concentrate diets, the rapid expansion of the epithelial uptake capacity of SCFA, both via passive diffusion and an increase in HCO3−-dependent pathways, shows that the ruminal epithelium is perfectly able to prevent the occurrence of SARA: it not only reduces the concentration of SCFA in the lumen and thereby removes acids as well as stabilizes ruminal osmolarity, but also leads to higher secretion of HCO3−, additionally helping to alkalize luminal pH. Morphological adaptations help to ensure high energy input while osmotic and acid–base homoeostasis are maintained.
Nonetheless, there seems to be a distinct individual variety of absorptive capability: sheep that were not susceptible to an induction of SARA were found to have a greater uptake of acetate and butyrate in comparison to sheep whose ruminal pH was below 5.8 for more than 20 min after a glucose drench (Penner et al., Reference Penner, Aschenbach, Gäbel, Rackwitz and Oba2009). This could be attributed to an increase of the HCO3−-dependent as well as the HCO3−-independent anion-exchange pathway for acetate and to an increase of HCO3−-independent transport of butyrate (Penner et al., Reference Penner, Aschenbach, Gäbel, Rackwitz and Oba2009; Chibisa et al., Reference Chibisa, Gorka, Penner, Berthiaume and Mutsvangwa2015). This individual predisposition to SARA is not well understood yet, but is of major importance for modern livestock feeding recommendations, as some animals will not be able to cope with an uncontrolled SCFA accumulation as easily as others, even though they are fed the same feed under the exact same conditions. In addition to dietary adaptation, the rumen and its epithelium must also be able to adapt to other environmental challenges such as water and salt stress. This has recently been reviewed by Giger-Reverdin et al. (Reference Giger-Reverdin, Domange, Broudiscou, Sauvant and Berthelot2020) and will not be considered further here.
Detoxification capacity of the ruminal epithelium
Besides providing valuable nutrients for the epithelium as well as the whole organism, the ruminal epithelium serves as an important barrier to potentially harmful substances like toxins and pathogens in the ruminal fluid as demonstrated in Figure 2. As the ruminal mucosa consists of a squamous, stratified multi-layered epithelium, its histological structure already lays the foundation for this protective task: tight-junction proteins between cells of the outer non-keratinized layers (i.e. the stratum granulosum on the interface with stratum corneum) seal up the apical layers to stop potential hazards from permeating the ruminal wall via the paracellular route, while substrates like SCFA can easily pass transcellularly through the medium layers to basal layers with the help of gap junctions in stratum granulosum to stratum spinosum (Graham and Simmons, Reference Graham and Simmons2005).
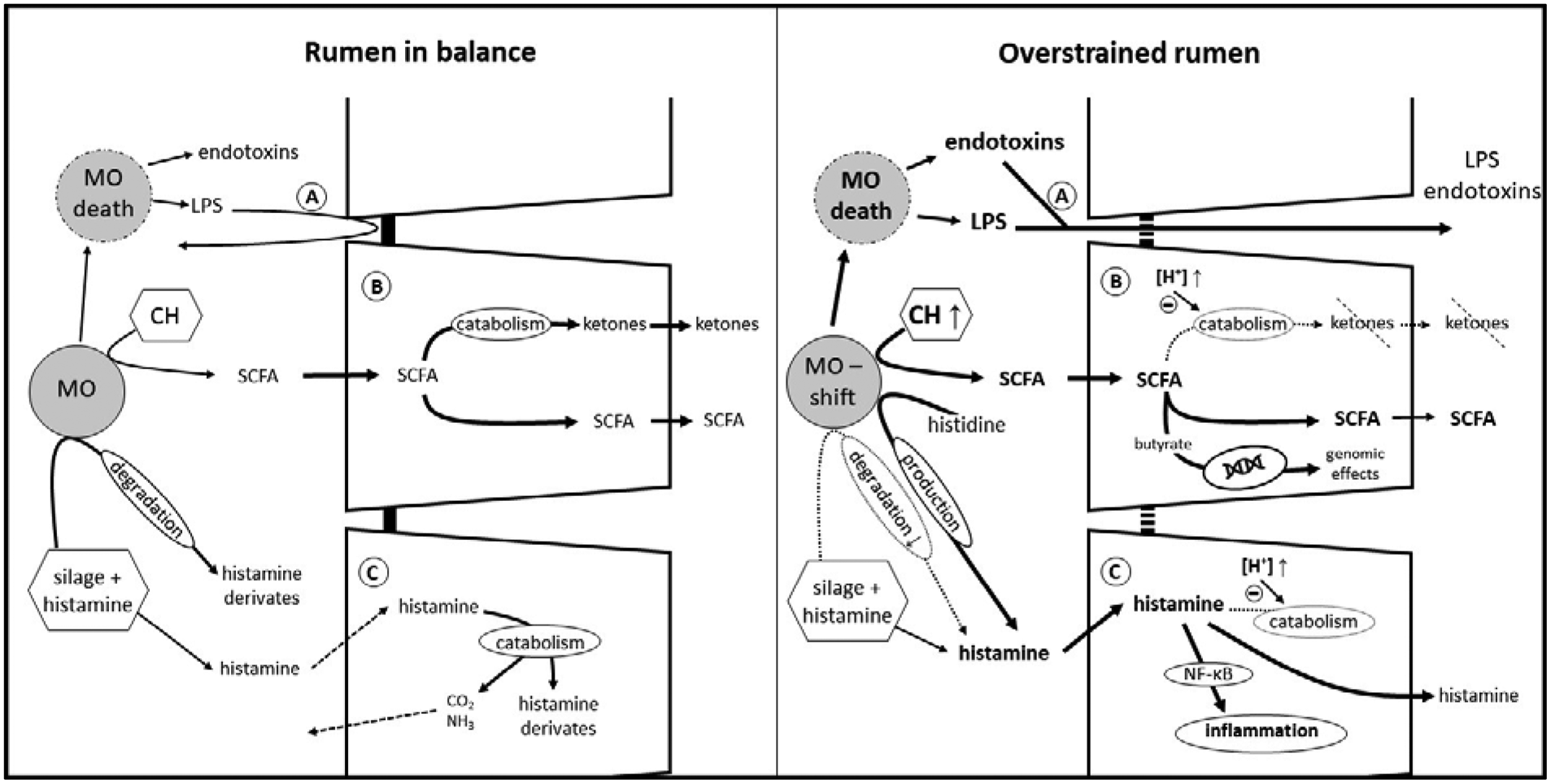
Fig. 2. Detoxification in the rumen and within the ruminal epithelium. Left picture: transport mechanisms as shown in Figure 1 work in a coordinated fashion. The production and absorption of SCFA is in balance, ruminal pH and osmolarity are within the physiological range. Moderate amounts of endotoxin, LPS and histamine produced by the microorganisms or taken up with the diet cannot cross the epithelial barrier. Right picture: increased amounts of easily fermentable carbohydrates lead to an increased production of SCFA. The absorptive and buffer capacity of the ruminal epithelium is overstrained, leading to epithelial acidosis and a shift in microorganisms. Toxins can cross the epithelial wall and the ruminal epithelium is confronted with larger amounts of histamine permeating the cell membrane. (A) toxin absorption, (B) SCFA transport and catabolism, (C) histamine absorption and catabolism. MO, microorganisms; LPS, lipopolysaccharides; CH, carbohydrates. For clarity only one cell layer is depicted, but this represents the multi-layered, stratified squamous epithelium.
As described above, high grain diets lead to an increase of luminal SCFA and potentially a higher fraction of butyrate (Dieho et al., Reference Dieho, Dijkstra, Schonewille and Bannink2016). Degradation of butyrate nourishes the epithelium and decreases the levels of acid that might provoke parakeratosis and other harmful effects on the epithelium (Fig. 2B). However, increasing the concentrate ratio is not only associated with a destabilization of ruminal pH, it also leads to increased metabolites in rumen fluid derived from methylated amines and lipopolysaccharides (LPS, released upon death of gram-negative bacteria) such as endotoxins, methylamine and others (Fig. 2A). Moreover, these associated toxins were found to translocate more easily across the ruminal wall at an acidic mucosal pH, leading to an aggravation of the ruminal imbalance induced by pH decompensation (Plaizier et al., Reference Plaizier, Danesh Mesgaran, Derakhshani, Golder, Khafipour, Kleen, Lean, Loor, Penner and Zebeli2018). When SARA was artificially induced by high-concentrate diets, increased levels of LPS were detected in the portal and hepatic veins. Simultaneously, liver inflammation could be observed, as blood chemistry, phosphorylation patterns and histopathology of the liver indicated an injury by ruminally derived LPS (Guo et al., Reference Guo, Chang, Zhang, Xu, Jin, Bilal and Shen2017). In contrast, Klevenhusen et al. (Reference Klevenhusen, Hollmann, Podstatzky-Lichtenstein, Krametter-Frötscher, Aschenbach and Zebeli2013) observed increased LPS concentrations in serum after a feeding trial with low barley diet, but no signs of systemic inflammation.
In addition to LPS and butyrate, histamine is another substance emerging in the rumen fluid that has the potential to endanger the animal's health (Fig. 2C). In cultured ruminal epithelial cells, an activation of inflammatory processes via the NF-κB pathway by histamine has been shown (Sun et al., Reference Sun, Yuan, Chen, Wang, Wang, Sun, Li, Li and Liu2017). Early pioneer studies by Kay and Sjaastad (Reference Kay and Sjaastad1974) already showed that the organism prevents excessive uptake of the biogenic amine: a certain amount of administered histamine is degraded within the forestomach and exhaled as CO2 or excreted with urine within 48 h. Studies that investigated adaptation mechanisms to diets with high amounts of biogenic amines detected a degradation of all biogenic amines examined, whereof histamine was the amine with the highest breakdown, followed by tyramine and putrescine. Degradation was much higher (70 v. 25%) in adapted animals than in non-adapted. This is most likely achieved by an increase of amine-degrading microorganisms in the rumen (van Os et al., Reference van Os, Lassalas, Toillon and Jouany1995). It is not only the ruminal microorganisms that prevent damage by amines, the epithelium itself also plays a major part. Ussing chamber experiments indicate that only a small portion of histamine is absorbed by the healthy epithelium (Aschenbach et al., Reference Aschenbach, Oswald and Gäbel2000), and of the histamine that is absorbed, more than 90% is efficiently catabolized within the epithelium under physiological conditions. Electrophysiological experiments even hint at a secretion of histamine, supporting the detoxification function of the ruminal epithelium (Aschenbach et al., Reference Aschenbach, Oswald and Gäbel2000). Nonetheless, when high concentrate rations are fed, histamine-producing microbes proliferate, especially Allisonella histaminiformans (Snyder and Credille, Reference Snyder and Credille2017). When changes of the microbiome and the accumulation of SCFA and H+ in the lumen exceed the ruminal adaptation capacity, this often escalates in a vicious circle: the epithelium becomes acidotic and epithelial uptake of histamine increases due to an elevation of its general permeability (Aschenbach et al., Reference Aschenbach, Oswald and Gäbel2000). In vitro experiments suggest reduced histamine catabolism in acidotic epithelia which finally leads to an aggravation of the clinical symptoms (Aschenbach et al., Reference Aschenbach, Oswald and Gäbel2000; Snyder and Credille, Reference Snyder and Credille2017). One study evaluated the impact of additional HCO3− administration to high-grain adapted ruminal fluid in an in vitro approach, discovering an increase in pH, gas production and total SCFA concentration while the production of biogenic amines was decreased (Mao et al., Reference Mao, Huo, Liu, Zhang and Zhu2017). Nonetheless, LPS concentrations were even more elevated in this study, making it difficult to give an undifferentiated recommendation to supplement HCO3− in high-concentrate diets.
As the mechanisms within the ruminal epithelium supporting its function as a healthy barrier are not widely understood to date, future research should focus on these defence pathways to gain a better understanding and to adapt livestock farming accordingly.
Conclusion
Modern ruminant husbandry demands a delicate balance between high energy input to ensure performance and a diet which guarantees that the ruminal microorganisms can ferment the ingested nutrients and that the epithelial uptake and metabolization capacities are not exceeded. By absorbing SCFA via passive diffusion and HCO3− exchange, the ruminal epithelium holds an outstanding position in maintenance of luminal osmolarity as well as acid–base homoeostasis. Furthermore, it ensures the delivery of nutrients for the epithelium itself and the whole organism while keeping track of the intraepithelial pH and osmolarity as well. Finally, it plays a decisive role in preserving the animal's health by exclusion, degradation and detoxification of potentially harmful substances.
Nonetheless, to warrant these beneficial functions, the epithelium must not be overburdened with excessive amounts of highly fermentable carbohydrates or a large number of toxins, as the physiological properties are limited even though the epithelium largely adapts to dietary challenges. Further research needs to be conducted to identify the individual factors that make some animals more prone to pH decompensation than others, and to determine how these factors can be modified.