Introduction
Succulent plants with edible fruits that are able to grow in dry regions – where most traditional crops are unsuccessful – are currently thought to be promising new products as their water demand is low compared to that of other crops and they can tolerate aridity (Mizrahi et al., Reference Mizrahi, Nerd, Sitrit, Janick and Whipkey2002); a condition that, according to the global warming models, will prevail in the near future in extensive areas of the tropics north of the Equator (Collins et al., Reference Collins, Knutti, Arblaster, Dufresene, Friedlingstein, Gao, Gutowski, Krinner, Shongwe, Tebaldi, Ewaver and Wehner2013). Among these plants, cacti are increasingly serving as agricultural and industrial crops, providing not only fruits, but also vegetables and animal fodder in dry and semi-dry regions because they and their succulent organs are adapted to withstand dry conditions (Mizrahi and Nerd, Reference Mizrahi, Nerd and Janick1999). In particular, climbing cacti with edible fruits have been proposed as new dryland fruit crops due to their high degree of water-use efficiency (Mizrahi and Nerd, Reference Mizrahi, Nerd and Janick1999).
Notably, one lineage of climbers in the cactus family, the Hylocereus group of Selenicereus, includes several species bearing edible fruits and is currently cultivated around the world. Fruits are known as pitahayas, pitayas or dragon fruit (Mercado-Silva, Reference Mercado-Silva, Rodrigues, de Oliveria and Sousa de Brito2018; ), and have been utilized not only as food but also as peels and pulp have many compounds that are employed as food colorants, medicine and cosmetics (Vaillant et al., Reference Vaillant, Pérez and Dávila2004; Zanoildin and Baba, Reference Zanoildin and Baba2009; Ortiz-Hernández and Carrillo-Salazar, Reference Ortiz-Hernández and Carrillo-Salazar2012; Azwanida et al., Reference Azwanida, Normasarah and Afandi2014; Nurul and Asmah, Reference Nurul and Asmah2014).
The Hylocereus group is now recognized in Selenicereus and native to the Neotropics and several species of Mexico, Central America and Colombia are currently cultivated around the world: Selenicereus undatus, S. monacanthus, S. polyrhizus, S. costaricensis and S. megalanthus. The latter bears yellow fruits while the rest have red or purplish peels and the most widely cultivated pitahaya in the world is S. undatus (Nerd et al., Reference Nerd, Tel-Zur, Mizrahi and Nobel2002; Le Bellec and Vaillant, Reference Le Bellec and Vaillant2006; Korotkova et al., Reference Korotkova, Borsch and Arias2017). In contrast, Selenicereus ocamponis is only used locally in central Mexico. Recognition of species in this group remains controversial. Here S. ocamponis is recognized including S. purpusii, although they often are separated by some authors (Bauer, Reference Bauer2003; García-Rubio et al., Reference García-Rubio, Vargas-Ponce, Ramírez-Mireles, Munguía-Lino, Corona-Oceguera and Cruz-Hernández2015). S. costaricensis is poorly understood, similar to S. escuintlensis and S. guatemalensis (Bauer, Reference Bauer2003); here it is identified as the species with purplish peel and pulp distributed in Costa Rica. S. undatus is well characterized by having a whitish pulp with variable red-purplish coloured peel (Bauer, Reference Bauer2003) (Fig. 1).
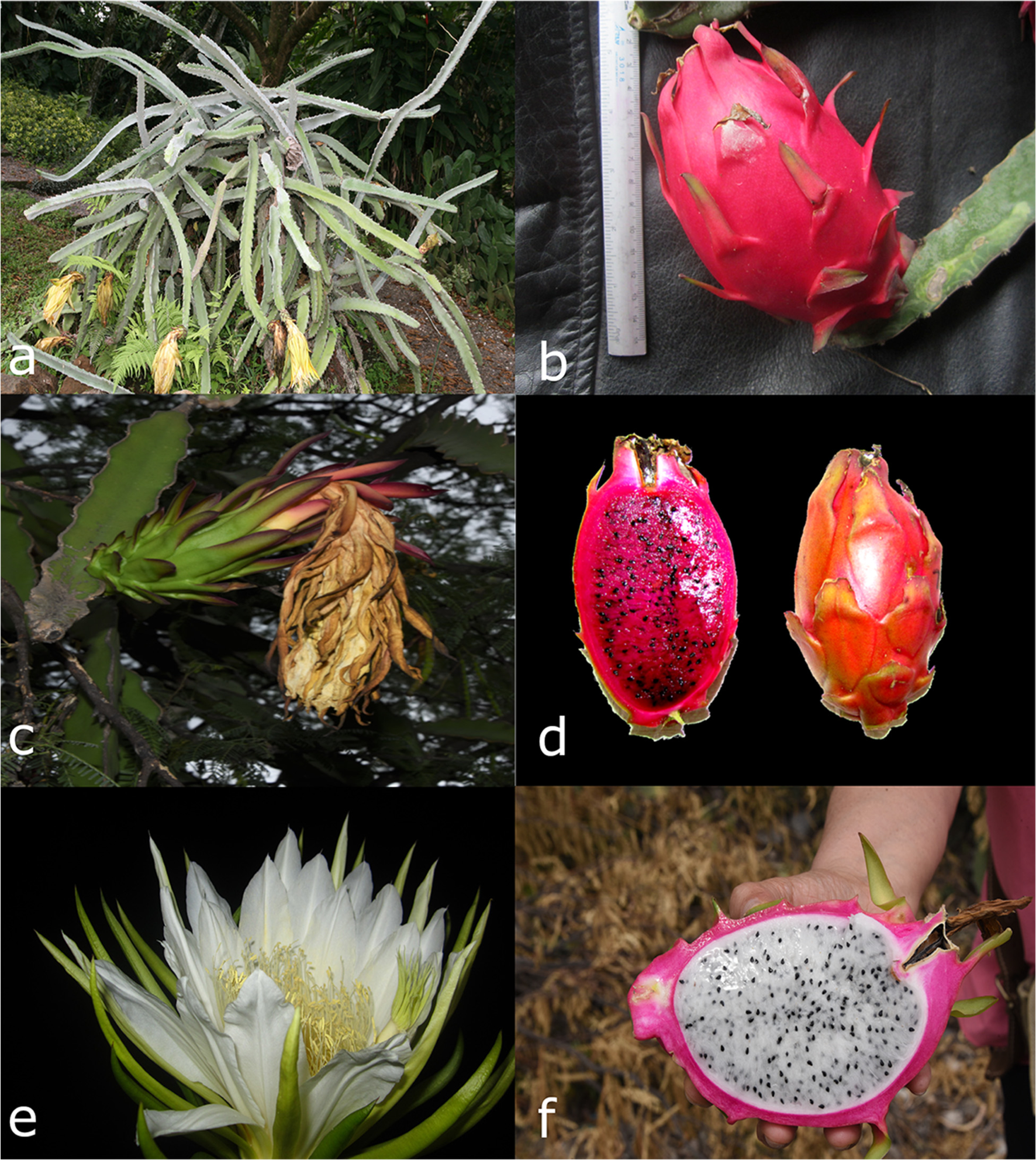
Fig. 1. (Colour online) Flowers and fruits of the three species studied in the Hylocereus group of Selenicereus: (a) plant with flowers of Selenicereus costaricensis, (b) fruit of S. costaricensis, (c) incipient fruit of S. ocamponis, (d) mature fruit and fruit cut in half showing pulp with seeds of S. ocamponis, (e) flower of S. undatus and (f) fruit cut in half of S. undatus showing pulp and seeds.
S. undatus has been cultivated in the Yucatan Peninsula, in the Maya area, since pre-Hispanic time according to historical records (Rodríguez Canto, Reference Rodríguez Canto, Inglese, Liguori and Nefzaoui2015). This species was introduced into the known territories of former Indochina (now Cambodia, Laos and Vietnam) from the Lesser Antilles by the French in the 16th century, and its cultivation has extended to other countries in Asia (Le Bellec and Vaillant, Reference Le Bellec and Vaillant2006). Currently, dragon fruit production mainly occurs in Vietnam and China, in Malaysia and in some countries where they are native, such as Honduras, Nicaragua and Mexico (Mercado-Silva, Reference Mercado-Silva, Rodrigues, de Oliveria and Sousa de Brito2018). They are important crops in the latter countries; for instance, in the north of the Yucatan Peninsula, an area of 25 ha of cultivation is expected to produce 400 tonnes from which 150 tonnes will be exported to the United States and Spain in the harvest of 2020 (Servicio de Información Agroalimentaria, 2020). In Mexico, pitahaya is produced under different management regimes, with production originating from different sources such as family orchards or home gardens in the Yucatan Peninsula (De Clerck and Negreros-Castillo, Reference De Clerck and Negreros-Castillo2000; Castro et al., Reference Castro, Lascurain-Rangel, Gómez-Díaz and Sosa2018). Moreover, only a few large farms produce pitahaya fruit for exportation; in contrast, there are many small plantations in the Pacific slopes of Mexico (Michoacan to Oaxaca and limits with Puebla) where hundreds of tonnes of fruit are produced (Ortiz-Hernández et al., Reference Ortiz-Hernández, Carrillo-Salazar and Livera-Muñoz2012a, Reference Ortiz-Hernández, Livera-Muñoz, Carrillo-Salazar, Valencia-Botín and Castillo-Martínezb; Ortega Hernández et al., Reference Ortega Hernández, León Andrade, Rosas Vargas, Egurrola and Luter2018). Our field work found that pitahayas are also favoured over trees of hedgerows in the north of the Gulf of Mexico lowlands, where they are native, mainly on mesquites (Prosopis laevigata, P. glandulosa and P. velutina). In plantations, it is cultivated on the turpentine tree, known as ‘chakah’, Bursera simaruba, while in Mayan home gardens pitahayas are grown on a number of trees, many legumes, comps, spurges, borages or even in palms such as Sabal mexicana (Cálix de Dios, Reference Cálix de Dios2005; Cálix de Dios et al., Reference Cálix de Dios, Castillo-Martínez and Caamal-Canché2014; Manzanero-Acevedo et al., Reference Manzanero-Acevedo, Isaac-Márquez, Zamora-Crescencio, Rodríguez-Canché, Ortega-Haas and Dzib-Castillo2014). Our field work has identified plants of S. ocamponis growing on several species of seasonally tropical dry forests such as Hura polyandra, Myrcia sp., Urera sp. and on rock outcrops. Furthermore, S. undatus was introduced into the north western drylands of Mexico and grown over the legume Pithecellobium dulce (Osuna-Enciso et al., Reference Osuna-Enciso, Valdez-Torres, Sañudo-Barajas, Muy-Rangel, Hernández-Vedugo, Villarreal-Romero and Osuna-Rodríguez2016).
Planning food production, including the detection of suitable areas for agriculture, is necessary to ensure current and future food security. The effectiveness of producing edible products will depend on the extent of the producers’ multi-disciplinary knowledge about productive systems. For the main cultivated species in the Hylocereus group (S. undatus, S. costaricensis, S. polyrhizus and S. megalanthus), several aspects of their propagation, agronomics, crop production, harvest and the post-harvest conservation of fruit, pests and diseases are known (see review papers by Mizrahi et al., Reference Mizrahi, Nerd, Sitrit, Janick and Whipkey2002; Le Bellec and Vaillant, Reference Le Bellec and Vaillant2006; Tel-Zur et al., Reference Tel-Zur, Mizrahi, Cisneros, Mouyal, Schneider and Doyle2011; Ortiz-Hernández et al., Reference Ortiz-Hernández, Carrillo-Salazar and Livera-Muñoz2012a, Reference Ortiz-Hernández, Livera-Muñoz, Carrillo-Salazar, Valencia-Botín and Castillo-Martínezb; Cisneros and Tel-Zur, Reference Cisneros and Tel-Zur2013; Valencia-Botín et al., Reference Valencia-Botín, Kokubu and Ortiz-Hernández2013, Mercado-Silva, Reference Mercado-Silva, Rodrigues, de Oliveria and Sousa de Brito2018). Moreover, various aspects of the physiology of the Hylocereus group have been documented; e.g. these plants utilize the CAM photosynthetic pathway and have an efficient system for retaining water by closing their stomata during the day (Nobel and De la Barrera, Reference Nobel and De la Barrera2000). S. undatus plants can be photo-inhibited if grown in direct solar radiation, with their photosynthetic activity peaking at only 35–45% photosynthetic photon flux (Andrade et al., Reference Andrade, Rengifo, Ricalde, Simá, Cervera and Vargas-Soto2006). Total net CO2 uptake is maximum at 30°C/20°C day/night, but is inhibited at 42°C/32°C (Nobel and De la Barrera, Reference Nobel and De la Barrera2000). Maximum temperature for S. undatus was estimated to be 39°C, and the minimum temperature for this species is 7°C (Mizrahi and Nerd, Reference Mizrahi, Nerd and Janick1999).
Ecological requirements and physiology of economically important plants, combined with climate models, is an excellent strategy for identifying areas with climate constraints that are likely to limit crop productivity; a sign of the importance of having agro-climate zoning information for farmlands (Patel et al., Reference Patel, Mandal and Pande2000). Furthermore, changes in climate will have important effects on the agricultural sector with potential implications for food security and production (Ciscar et al., Reference Ciscar, Fisher-Vanden and Lobell2018). Thus, research is focusing on present and future climate changes for predicting suitable areas for cultivation of many important plants (Shabani and Kotey, Reference Shabani and Kotey2016; Allbed et al., Reference Allbed, Kumar and Shabani2017; Rasmussen et al., Reference Rasmussen, Thyrring, Muscarella and Borchsenius2017; Barrueto et al., Reference Barrueto, Merz, Hodel and Eckert2018; Golbon et al., Reference Golbon, Cotter and Sauerborn2018; Raza et al., Reference Raza, Mahmood, Khan and Liesenberg2018; Moatt et al., Reference Moatt, Gole and Davis2019; Román-Figueroa et al., Reference Román-Figueroa, Herrera, Cortez, Uribe and Paneque2019; Tshabalala et al., Reference Tshabalala, Ncube and Moyo2019). When attempting to detect suitable areas for cultivating crops, during the decision-making process, information from different sources might use analytical hierarchical algorithms to rank significant variables in addition to climate variables (e.g. Saaty Reference Saaty1987, Reference Saaty2008; Figuera et al., Reference Figuera, Greco and Ehrgott2005).
The objective of this paper was to predict current and future suitable areas for managing three Mesoamerican Selenicereus species in the Hylocereus group: two widely cultivated species, S. undatus and S. costaricensis, and the more locally known S. ocamponis, using multi-criteria suitability analyses and ecological niche-based modelling (ENM). Our hypothesis is that future climate warming will broaden favourable areas for cultivation of these species in which not only the most amply cultivated species, S. undatus, but also S. ocamponis can be cultivated in extended areas.
Materials and methods
Study species and preferred hosts
For this study, three species: S. undatus (Haw.) Britton & Rose, S. costaricensis Britton & Rose and S. ocamponis (Salm-Dyck) Britton & Rose were selected (Fig. 1).
Ecological niche-based modelling
The geographic coordinates of the species utilized in this study were collected by our project in the field or by visits to herbaria; the rest, by accessing collections from databases, corroborating each record that it was not observed directly. Records from these herbaria were considered: AUU, B, BOLV, CICY, COL, CR, EAP, ENCB, F, FLAS, GOET, HEM, HUA, HULE, IBUG, IEB, INB, K, LAGU, LOJA, LPB, MEDEL, MEXU, MNHN, MO, NY, P, TEFH, UADY, UC, UMO, UPRRP, US, USCG, USJ, XAL and ZSS (acronyms follow Index Herbariorum, Thiers 2019). They were used to carry out ENM for each species (Table S1 lists the number of records used for each species). Environmental inputs were based on the 19 climate variables from the WorldClim database, version 1.4 (Hijmans et al., Reference Hijmans, Cameron, Parra, Jones and Jarvis2005) at a resolution of 2.5 min. These variables represent global precipitation and temperature conditions for the years 1960–90.
Prior to estimating the ENMs, the raster package in the statistical software R was used to run a principal component analysis (PCA) and identify the most significant climate variables and only these variables were utilized (Thuiller et al., Reference Thuiller, Georges, Engler, Breiner, Georges and Thuiller2016). Species distribution modelling was carried out using the maximum entropy (MaxEnt) algorithm implemented in the biomod2 package of R (Thuiller et al., Reference Thuiller, Georges, Engler, Breiner, Georges and Thuiller2016). For each species, 70% of the occurrence data records were used for training the models and 30% for testing them. There were ten replicates per species and geographic predictions and performance were averaged per species with these data. Model performance was estimated for the projections in the climate scenario for the present using the ROC statistical metrics (receiver operating characteristic curve), TSS (true skill statistic) and Kappa. Values for ROC range from 0 to 1, with values >0.5 meaning the model predicts testing points better than would be expected by random chance (Table S2). TSS and Kappa values range from −1 to 1 where values close to 0 indicate that a prediction is not different from random, whereas positive values indicate predictions better than random.
To understand the outcome of future climate change in suitable regions for the studied Selenicereus species, ENMs were predicted for the decade of 2061–80. We base future climate scenarios on the Couple Model Intercomparison Project Phase 6 (CMIP6, Eyring et al., Reference Eyring, Bony, Meehl, Senior, Stevens, Stouffer and Taylor2016). We used the sixth version of the MIROC climatic model 6 (MIROC6) as it presents considerable improvements over previous models, especially on the estimated amount of rain over the tropical Pacific (AORI CCSR-NIES 2019, Tatebe et al., Reference Tatebe, Ogura, Nitta, Komuro, Ogochi, Takemura, Sudo, Sekiguchi, Abe, Saito, Chikira, Watanabe, Mori, Hirota, Kawatani, Mochizuki, Yoshimura, Takana, O'ishi, Yamazaki, Suzuki, Kurogi, Kataoka, Watanabe and Kimoto2019). Based on this model, we further selected the most optimistic (ssp216) and pessimistic (ssp585) shared socioeconomic pathways (König et al., Reference König, Loibl, Haas, Kranzl, Steininger, König, Bednar-Friedl, Kranzl, Loibl and Prettenthaler2014, Riahi, et al., Reference Riahi, van Vuuren, Kriegler, Edmonds, O'Neill, Fujimori, Bauer, Calvin, Dellink, Fricko, Lutz, Popp, Crespo-Cuaresma, Samir, Leimbach, Jian, Kram, Rao, Emmerling, Ebi, Hasegawa, Havlik, Humpenöder, DaSilva, Smith, Stehfest, Bosetti, Eom, Gernaat, Masui, Rogelj, Strefler, Drouet, Krey, Luderer, Harmsen, Takahashi, Baumstark, Doelman, Kainuma, Klimont, Marangoni, Lotze-Campen, Obersteiner, Tabeau and Tavoni2017). Ssp126 predicts low radiative forcing by the end of the century (2.6 W/m2 in 2100), while the ssp585 predicts a high radiative forcing (8.5 W/m2 in 2100). All data downscaled and based on WorldClim v1.4 (Hijmans et al., Reference Hijmans, Cameron, Parra, Jones and Jarvis2005). For future predictions on the distribution of suitable areas we used the same bioclimatic variables that best predicted the distribution in the scenario of current conditions.
Similarly, to consider an additional variable for the hierarchical decision modelling, in particular for the most cultivated species S. undatus, ENM of the three species of mesquite (P. laevigata, P. glandulosa and P. velutina) important hosts of this species was carried out. The three species of Prosopis were considered simultaneously using a random forest approach of tree model classification (Evans, et al., Reference Evans, Murphy, Holden, Cushman, Drew, Wiersma and Huettmann2011; Rodríguez-Galiano et al., Reference Rodríguez-Galiano, Ghimire, Rogan, Chica-Olmo and Rigol-Sánchez2012). Based on the set of selected variables an exhaustive series of model tests were carried out, that included all possible combinations of the climate variables selected in the random forest process, from pairs up to the saturated model (Phillips et al., Reference Phillips, Dudík and Schapire2004). Detailed description of the strategy for this modelling is presented in the Supplementary material.
Hierarchical decision modelling
In addition to the traditional ENM, hierarchical decision models were used to predict the suitable areas for the development of the three species of Selenicereus studied. Here, based on physiological criteria reported in the literature as relevant to the distribution of the focal species of the Hylocereus group of Selenicereus, the following environmental variables were selected: annual accumulated solar radiation, average water vapour pressure (Fick and Hijmans, Reference Fick and Hijmans2017), temperature seasonality (BIO4), the maximum temperature of the warmest month (BIO5), minimum temperature of the coldest month (BIO6), precipitation seasonality (BIO14), precipitation of the wettest quarter of the year (BIO15) and precipitation of the driest quarter of the year (BIO16) (Hijmans et al., Reference Hijmans, Cameron, Parra, Jones and Jarvis2005). Besides, based on the ecology of the studied species, the distribution of the three Prosopis species (P. laevigata, P. glandulosa and P. velutina, important phorophites for S. undatus) was identified as a relevant factor. Habitat suitability (HS) values were used as the probability of encountering trees of these Prosopis in any given cell. A decision matrix (9 × 9) was built with the nine variables following Saaty (Reference Saaty1987). For every pair of variables in the matrix, a relative weight was assigned above the diagonal based on the previous studies reporting on the physiology and ecology of the studied species (Table 1). The categories considered were equal, moderately more, strongly more, very strongly more and extremely more and were coded numerically as 1, 3, 5, 7 and 9 (or their reciprocal values, e.g. 1/3), respectively. Also, for a finer classification of the importance between some pairs of variables a coded of 2, 4, 6 or 8 (and their corresponding reciprocal values) was assigned.
Table 1. Suitable criteria influencing cultivation of three Mesoamerican species of the Hylocereus group: S. costaricensis, S. ocamponis and S. undatus, based on the analytical hierarchical process and on the ENM

a BIO5, maximum temperature of the warmest month; BIO6, minimum temperature of the coldest month; BIO15, precipitation of the wettest quarter of the year.
b Temperature seasonality: standard deviation × 100.
c Precipitation seasonality: coefficient of variation; BIO4, temperature seasonality.
The decision matrix was normalized by dividing each cell by the corresponding column total. The adequacy of the consistency index (CI) of the matrix, CI = (λ max− k)/(k − 1), where k is the number of variables included, was tested by simulating 1000 random matrixes of weights. For every iteration, the CIr (CI of the random matrix) was calculated, and the ratio between CI and CIr, used a criterium to assess whether if the assigned weights in the decision matrix were consistent. Ratio values below 0.1 indicate that the decision matrix was consistent (Saaty, Reference Saaty1987). HS was modelled using MaxEnt (Thuiller et al., Reference Thuiller, Georges, Engler, Breiner, Georges and Thuiller2016), where each variable was weighted by the relative weight calculated from the normalized decision matrix. HS = ∑Xiwi, where Xi is each of the layers and wi is the relative weight estimated from the normalized matrix.
Results
Ecological niche-based modelling
The PCA analyses identified the following climate variables as significant for all three species S. costaricensis, S. ocamponis, S. undatus: mean diurnal temperature range, isothermality, precipitation of the driest month, precipitation seasonality, precipitation of the warmest quarter and precipitation of the coldest quarter (BIO2, BIO3, BIO14, BIO15, BIO18 and BIO19, respectively). Mean temperature of the wettest quarter and precipitation of the wettest month (BIO8 and BIO13) were also relevant for S. costaricensis and S. undatus. The mean temperature of the driest quarter (BIO9) was relevant for S. ocamponis and S. undatus. Temperature seasonality (BIO4) and precipitation of the wettest quarter (BIO16) were relevant for S. costaricensis and S. ocamponis, respectively (Fig. S1).
ENM for current conditions predicted the potential distribution areas for the three species and these are shown in Fig. 2. In all cases, areas extending beyond the currently known distribution range were forecasted. The species with the smallest area of potential distribution was S. costaricensis (Fig. 2a), while the potential distribution prediction for S. ocamponis is a large area on the Pacific side of Mexico and Central America, and also includes the southern part of the Baja California Peninsula and some regions on the Atlantic side, at the Gulf of Mexico border of the Yucatan Peninsula (Fig. 2b). The largest predicted potential distribution was for S. undatus for which essentially only the more xeric areas were not identified as suitable or optimal, with the rest being suitable for cultivating this species (Fig. 2c). ENM for the three species indicates suitable areas on the Pacific side of Mesoamerica from Sonora in Mexico to Costa Rica in Central America (Fig. 2d).

Fig. 2. (Colour online) Forecasted potential distribution areas by the ENM with current climate displaying the georeferences recorded for every species and the suitability likelihood: (a) S. costaricensis, (b) S. ocamponis, (c) S. undatus and (d) predictions for the three species altogether. Current climate is represented by global precipitation and temperature conditions for the years 1960–90.
The random forest model selected six bioclimate variables as relevant for predicting the current distribution of the three selected species of Prosopis: minimal temperature of the coldest month, mean temperature of the coldest quarter, annual precipitation, precipitation of the wettest quarter, precipitation of the driest quarter and precipitation of the coldest quarter (BIO6, BIO11, BIO12, BIO16, BIO17 and BIO19, respectively). After running all possible combinations of the MaxEnt models (57 models), we found that the model including annual precipitation and precipitation of the driest quarter (BIO12 and BIO17) had the greatest ratio of positive matches (84%, Fig. 3a).

Fig. 3. (Colour online) Habitat suitability identified by ENM showing continuous climate HS by discretized values: (a) Prosopis spp. (Fabaceae), the preferred hosts of S. undatus, (b) S. undatus, (c) S. costaricensis and (d) S. ocamponis.
ENM for future climate conditions forecasted in all scenarios, larger suitable areas for S. undatus, and for S. costaricensis (Fig. 4). These future scenarios predicted less suitable areas of S. ocamponis in comparison with its current potential distribution (Fig. 4). Furthermore, in terms of surface estimation, modelling future climate scenarios forecasted more than twice the prediction for current climate for S. undatus, while it is clear that much less surface is forecasted for S. ocamponis with MIROC scenarios and in S. costaricensis the predicted area in future scenarios is similar to prediction of current climate modelling (Table 2).

Fig. 4. (Colour online) Forecasted potential distribution areas by the ENM with two scenarios of future climate change, MIROC6 ssp126 and ssp585 for the three species studied, S. costaricensis, S. ocamponis and S. undatus. ENMs were forecasted for the decade of 2061–80. (a) S. costaricensis, (b) S. ocamponis, (c) S. undatus, (d) S. costaricensis, (e) S. ocamponis, (f) S. undatus, (g) S. costaricensis, (h) S. ocamponis, (i) S. undatus.
Table 2. Forecasted suitable area by current and future climate ENM for the three studied species

Future scenarios MIROC6-ssp126 and MIROC6-ssp585 were selected. Estimations are given in million ha.
Suitable area for the cultivation of Mesoamerican species of the Hylocereus group
The prediction of environmental niche suitability for Prosopis spp. was used in the hierarchical decision procedure together with accumulated solar radiation, average water vapour pressure, temperature seasonality, maximum temperature of the warmest month, minimum temperature of the coldest month, precipitation of the driest month, precipitation seasonality and precipitation of the wettest quarter (BIO4, BIO5, BIO6, BIO14, BIO15 and BIO16, respectively). Of the nine variables analysed for S. undatus, the suitability index of Prosopis spp. (36%) was the variable with the greatest weight in the hierarchical analytical model followed by precipitation seasonality (BIO15) (16%) and temperature seasonality (BIO4) (14.4%), while all other variables had less weight wand ranged from 1.7 to 10.9% (Table 2, Fig. 3b). For S. costaricensis and S. ocamponis, the variables with the greatest weight were solar radiation (24%), precipitation seasonality (BIO15) (22.1) and temperature seasonality (BIO4) (14.7%), while for the other variables weight ranged from 2.6 to 11.4% (Table 2, Fig. 3c, d). For all three species, the ROC curves (Fig. S2) showed that the weighted MaxEnt model produced a ratio of true positives to false positives well above the one to one ratio and increased sharply as the detection threshold increased. In S. costaricensis, the species with the most restricted distribution, the increase in the ratio of true positives to false positives with the detection threshold was more pronounced than it was in the other two species (Fig. S2).
The suitability maps showed that optimal conditions for the growth of S. undatus (Fig. 3b) occur over large areas, but exclude the northernmost part of Mexico. The optimal areas for this species were identified on the Pacific side of Mexico down to Central America and in areas of the Balsas Basin. On the Atlantic side, the optimal areas are scattered and on the Yucatan Peninsula, large areas on the Gulf of Mexico were identified. For S. costaricensis, suitable areas were identified on the Pacific side of southern Mexico down to Costa Rica (Fig. 3c). For S. ocamponis, the optimal area was more restricted but similar to that of S. undatus (Fig. 3d).
Hierarchical analytical modelling
The hierarchical analytical model carried out based on the pairwise comparison matrix of the nine environmental variables for the three species of the Hylocereus group determined that for S. undatus the most significant were precipitation seasonality (BIO15), the maximum temperature of the warmest month (BIO5) and solar radiation (similar duration of day/night) (Table 3). For S. costaricensis and S. ocamponis, solar radiation, precipitation seasonality (BIO15) and temperature seasonality (BIO15) were the most significant (Table 3).
Table 3. Pairwise relative importance among nine environmental variables for three species of the Hylocereus group in Mexico and Central America, and overall priority weights (W) for the nine variables

CI, consistency index; CIrandom, mean consistency index of 1000 permutations of the matrix weights under the rule that the lower triangle must be the reciprocal of the upper triangle values; CR, consistency ratio, CI/CIrandom; SR, solar radiation over 12 months; WVP = average water vapour pressure, BIO4, temperature seasonality; BIO5, maximum temperature of the warmest month; BIO6, minimum temperature of the coldest month; BIO14, precipitation seasonality; BIO15, precipitation of the wettest quarter of the year; BIO16, precipitation of the driest quarter of the year.
Discussion
The ecological preferences identified by ENM coincided with the findings of previous research on the optimal ecological conditions for proper physiological functions for S. undatus. It has been determined that plants of this species can be photo-inhibited if they are grown under direct solar radiation (Andrade et al., Reference Andrade, Rengifo, Ricalde, Simá, Cervera and Vargas-Soto2006), and the adequate temperatures for CO2 uptake are a maximum of 30°C/20°C day/night, which is inhibited at 42°C/32°C (Nobel and De la Barrera, Reference Nobel and De la Barrera2000).
Forecasting potential distribution using ENM for each of the three species studied S. costaricensis, S. ocamponis and S. undatus suggests that they can be cultivated in larger areas than those covered by their current, more restricted distribution. Moreover, the forecast for the three species suggests that Pacific side of Mesoamerica, from Sonora and Sinaloa, and south to Costa Rica have suitable areas for these species to be cultivated.
The ENM for the three species of mesquite – important hosts of S. undatus according to our field work – forecasted diverse regions, and this could be another factor that would influence the decision to promote dragon fruit crops in these regions. The hierarchical analytical model confirmed that the most significant variable for understanding the distribution of S. undatus is the presence of mesquite, which was even more significant than several of the climate, precipitation and temperature variables. In contrast, for S. costaricensis and S. ocamponis other factors such as solar radiation and maximum temperature were important to understand their distribution.
The results of ENM for S. costaricensis, S. ocamponis and S. undatus indicate that all three have similar climate preferences and the ENMs identified areas where these species can be cultivated. Based on this information, it is suggested that home gardens of the Yucatan Peninsula be enriched by cultivating the three species studied. Inventories of the home gardens have recorded only S. undatus (De Clerck and Negreros-Castillo, Reference De Clerck and Negreros-Castillo2000). Moreover, it has been said that these domestic agrosystems are being abandoned and that measures need to be taken to encourage owners to continue managing their home gardens, producing edibles and generating income (Castro et al., Reference Castro, Lascurain-Rangel, Gómez-Díaz and Sosa2018). By adding species that have coloured pulp in their fruits, such as S. costaricensis and S. ocamponis, the betalains, the pigments responsible for their colour, could be utilized as food colorants and in cosmetics, providing additional benefits.
Another opportunity to promote the cultivation of these three pitahayas is on trees utilized as hedgerows. It is known that mesquites are amply used as hedgerows in Mexico making it possible to cultivate these three studied species of the Hylocereus group in borders of cultivation zones or regions (Palacios, Reference Palacios2006; Zuria and Gates, Reference Zuria and Gates2006; Alanís-Rodríguez et al., Reference Alanís-Rodríguez, Molina-Guerra, Jiménez-Pérez, Rubio-Camacho, Mora-Olivo, Collantes-Chávez-Costa and Marroquín-Castillo2017). Furthermore, combining these three species of the Hylocereus group would increase their economic value because S. costaricensis and S. ocamponis have coloured pulp, while S. undatus fruits can be bigger and have white pulp.
ENM with the two scenarios for future climate change demonstrated that S. undatus, the most amply cultivated species in the world, can be grown in extensive habitats in Mesoamerica. Moreover, plantations of this species in arid regions of north-western Mexico can be very successful, as it has been already promoted in these regions (Osuna-Enciso et al., Reference Osuna-Enciso, Valdez-Torres, Sañudo-Barajas, Muy-Rangel, Hernández-Vedugo, Villarreal-Romero and Osuna-Rodríguez2016). Also, these models predicted extended areas for S. costaricensis. However, MIROC models predicted less suitable areas with negative impacts for S. ocamponis. Nevertheless, dragon fruits are corroborated as promising fruits in scenarios of climate change.
With exception of the hybrid South American species S. megalanthus, the rest of edible pitahayas are naturally distributed in the Lowland Maya area (Ortiz-Hernández and Salazar, Reference Ortiz-Hernández and Carrillo-Salazar2012). The Maya recognize the hypoglycaemic, diuretic and healing properties of its fruit and stems (Ortiz-Hernández et al., Reference Ortiz-Hernández, Carrillo-Salazar and Livera-Muñoz2012a). Furthermore, suitable areas for cultivating not only S. undatus, but also S. ocamponis were identified by our results in these territories. Pitahayas are cultivated either in the home gardens or in small plantations and there are a number of projects for increasing production of S. undatus in the Yucatan Peninsula (Cáliz de Dios et al., Reference Cálix de Dios, Castillo-Martínez and Caamal-Canché2014). Therefore, our study confirmed that the species of pitahayas share ecological preferences and are able to withstand future climate change, thus they should be favoured and cultivated amply in the Maya lowlands.
Finally, it is worth noting that predicting the areas suitable for cultivation should take into consideration other factors such as domestication history, anthropogenic influences, etc., as their importance has been researched for underutilized crops (Vihotogbé et al., Reference Vihotogbé, Idohou and Gebauer2019).
Conclusions
All three studied Mesoamerican species of dragon fruit have similar climate preferences and the ENMs identified areas where these species can be cultivated. They can be grown in trees in hedgerows, in trees from the seasonally tropical dry forests common in these areas or can be grown to enrich orchards with other useful trees. Forecasting potential distribution for current and future climate conditions have shown that S. undatus and S. costaricensis can be cultivated in larger areas than those covered by their current, more restricted distribution. Dragon fruits are corroborated as promising fruits in scenarios of climate change with global warming.
Supplementary material
The supplementary material for this article can be found at https://doi.org/10.1017/S0021859620000775
Acknowledgements
We thank Bianca Delfosse for her editorial work on English language of the manuscript.
Financial support
This research was supported by CONACYT, Mexico to V. S. (PNDP2015/1023). C.R.-D. was supported by a Doctor of Science scholarship (300231) and B.-E G.-R by a Master of Science scholarship (CVU935962), both from CONACyT, Mexico.
Conflict of interest
The authors declare there are no conflicts of interest.