Management Implications
We found that inoculating freshly cut Ligustrum lucidum (glossy privet) logs with Trametes versicolor (turkey tail) is an easy and effective method for expediting wood decomposition and treating slash generated from removal of invasive hardwoods. Slash is typically left to decompose on its own, transported offsite, piled and burned, or mulched. We offer an alternative approach that accelerates in situ recycling of the woody debris. Inoculating logs with a native wood-decaying fungus allows the biomass to remain in the ecosystem and provide ecological services while reducing fire risk concerns. Inoculated logs can in turn be used to assist with habitat restoration, including erosion control, water retention, and seedling establishment.
Introduction
Glossy privet (Ligustrum lucidum W. T. Aiton) is a fast-growing evergreen shrub or tree native to China, Japan, and Korea (Maddox et al. Reference Maddox, Byrd and Serviss2010). Introduced as an ornamental and hedge plant, this invasive species occupies a wide range of habitats, including open woodlands, closed-canopy forests, grasslands, riparian areas, rain forests, and waste areas. It can easily escape cultivation and has been recorded on every continent except Antarctica (CABI 2018). Highly aggressive, it spreads by animal-dispersed seeds, particularly birds, as well as by sprouts from roots and stumps. As a result, L. lucidum can form dense, impenetrable monocultures, replacing native trees and reducing biodiversity if not controlled (CABI et al. 2018; Coutts-Smith and Downey Reference Coutts-Smith and Downey2006; Global Invasive Species Database 2018). Ligustrum lucidum invasions have been shown to alter below- and aboveground communities, having effects such as lower total seed density and richness in the soil seedbank (Ferreras et al. Reference Ferreras, Giorgis, Tecco, Cabido and Funes2015), suppression of native tree recruitment (Lichstein et al. Reference Lichstein, Grau and Aragón2004), reduced understory cover (Hoyos et al. Reference Hoyos, Gavier-Pizarro, Kuemmerle, Bucher, Radeloff and Tecco2010), more homogenous vertical structure, increased canopy cover, lower litter depth, and declining bird species abundance, richness, and diversity (Ayup et al. Reference Ayup, Montti, Aragón and Grau2014).
Recommended management of heavy L. lucidum infestations includes gradual removal combined with replacement with native plants (Johnson Reference Johnson2009). The resulting deadwood can be slow to degrade, creating additional management challenges. To address this issue, slash may be transported offsite or piled and burned to reduce wildfire risks. Pile burning can cause long-term soil damage (Page-Dumroese et al. Reference Page-Dumroese, Busse, Archuleta, McAvoy and Roussel2017) and persistent canopy gaps (Rhoades and Fornwalt Reference Rhoades and Fornwalt2015). Page-Dumroese et al. (Reference Page-Dumroese, Busse, Archuleta, McAvoy and Roussel2017) provide alternative methods of burning forest residues to create biochar for use as a soil amendment and to sequester carbon. All of these methods remove biomass from the ecosystem, which can be disadvantageous, particularly for areas that have previously experienced significant biomass losses (e.g., due to land clearing). Alternatively, mechanical mulching and chainsaw felling of the closely related Chinese privet (Ligustrum sinense Lour.), with residues left onsite, have been shown to be effective strategies to increase percent cover, species richness, diversity, and evenness of herbaceous plants (Hudson et al. Reference Hudson, Hanula and Horn2014). While the plant communities were similar 5 yr after treatment, mulched plots had higher percent cover and number of plants than hand-felled plots (Hudson et al. Reference Hudson, Hanula and Horn2014). However, mulching may not be feasible due to costs and/or inaccessibility. Further, Marcot (Reference Marcot2017b) recommends “large chunks” of decaying wood to provide the “most beneficial, long-term (‘time-released’) ecological services.”
In areas where hand felling is the preferred option, selective introduction of wood-destroying fungi can be used to accelerate decomposition of the debris (Pavlík et al. Reference Pavlík, Hrasko and Pavlikova2008; Pavlík and Pavlík Reference Pavlík and Pavlík2013). Saprotrophic fungi are primary recyclers that break down surface detritus on the forest floor, where it then becomes accessible to other organisms (Arnstadt et al. Reference Arnstadt, Hoppe, Kahl, Kellner, Krüger, Bauhus and Hofrichter2016; Marcot Reference Marcot2017a). The process of wood decomposition contributes to soil development and productivity, nutrient cycling, moisture retention, and forest regeneration (Bednarz et al. Reference Bednarz, Huss, Benson and Varland2013; Lonsdale et al. Reference Lonsdale, Pautasso and Holdenrieder2008; Marcot Reference Marcot2017b; Pavlík et al. Reference Pavlík, Hrasko and Pavlikova2008; Piškur et al. Reference Piškur, Bajc, Robek, Humar, Sinjur, Kadunc, Oven, Rep, Petkovšek, Kraigher, Jurc and Pohleven2011). Turkey tail (Trametes versicolor Pilat) is a saprotrophic fungus with a circumpolar distribution (Lepp Reference Lepp2012; Stamets Reference Stamets2000; Veena and Pandey Reference Veena and Pandey2012). It is a nonparasitic but aggressive decomposer of dead wood, growing mainly on hardwoods and infrequently on conifers (Cotter Reference Cotter2014). A white rot fungus, T. versicolor is among the only organisms known to completely digest lignin (van der Wal et al. Reference van der Wal, Ottosson and de Boer2015), with the resulting wood becoming soft and spongy with a whitish color (Gao et al. Reference Gao, Du, Yang, Wu and Liang2010). Trametes versicolor also has well-documented medicinal properties, which include antitumor, antimicrobial, immune-enhancing, antioxidant, and antiradical compounds (Chu et al. Reference Chu, Ho and Chow2002; Hobbs Reference Hobbs2004; Janjušević et al. Reference Janjušević, Karaman, Šibul, Tommonaro, Iodice, Jakovljević and Pejin2017), as well as the ability to degrade several organic pollutants (Gao et al. Reference Gao, Du, Yang, Wu and Liang2010) and herbicides (da Silva Coelho-Moreira et al. Reference da Silva Coelho-Moreira, Maciel, Castoldi, da Silva Mariano, Inácio, Bracht, Peralta, Price and Kelton2013).
The Balcones Canyonlands Preserve near Austin, TX, is managed to conserve its diverse ecosystems, which support rare and endangered songbirds, karst invertebrates, and plants. Management activities focus on promoting healthy forests, from the forest floor to the tree canopy. Due to the rapid growth of L. lucidum, this invasive hardwood can quickly form dense stands, particularly along riparian corridors, which pose a threat to these endangered habitats. Thus, removal of L. lucidum and other nonnative, invasive plant species is a critical component of preserve management (Balcones Canyonlands Preserve 2007). At the same time, wildfires that occurred during a severe drought in 2011 have increased concerns about wildfire risks and curtailed planned invasive plant removal in areas where slash could not be easily removed or mulched. To explore a possible solution to this dilemma, we conducted a 3-yr pilot study to evaluate whether inoculating L. lucidum logs with a native strain of T. versicolor would accelerate wood decomposition and present a viable option for treating slash of an invasive woody species.
Materials and Methods
Study Area
We conducted our study from February 27, 2015, to March 5, 2018, on two tracts within the Balcones Canyonlands Preserve (30.40°N, 97.86°W), located on the eastern Edwards Plateau west of Austin, TX, USA. This region is characterized by shallow soils over Cretaceous limestone plateaus dissected by steep canyons and has a subtropical subhumid climate. We chose two forested riparian study sites (Site 1, Site 2) that were ∼6 km apart, both of which were dominated by mature Ashe juniper (Juniperus ashei J. Buchholz) and oak (Quercus spp.) trees on gentle east-facing slopes at elevations of ~230 to 260 m above sea level and <100 m from an intermittent creek. Total annual rainfall amounts for the Austin area during the years of our study were 1,523 mm (2015), 987 mm (2016), and 882 (2017), and mean annual temperatures ranged from 21.2 to 22.4 C, with a low of 9.4 C in January to a high of 31.8 C in July (Camp Mabry, Weather Underground 2015–2017). We chose sites 1 and 2 because of their similar environments, proximity of dense L. lucidum stands, and accessibility. Site 2 also has a team of volunteers who were able to assist with log inoculations and monitoring.
Preparation of Trametes versicolor Inoculum
Although T. versicolor is ubiquitous in North America and many parts of the world, we chose to clone a local subvariety of T. versicolor to create our spawn for inoculation. This was done to ensure that the mycelium had already adapted to and proliferated in the climate of our study sites. Furthermore, the T. versicolor cloning sample was harvested specifically from a dead L. lucidum stump to ensure that T. versicolor would grow on the intended wood type. We used standard cloning procedures typical in commercial mushroom production (Stamets Reference Stamets2000). The sample was found growing on a dead L. lucidum stump in October 2014 and brought into the lab for cloning on the same day. Exterior debris was removed from the sample, and the sample was then placed in the steam of a 99.99% efficiency laminar flow hood, where it was manually split to expose the fleshy interior of the fruit body. Using sterile equipment, we extracted three grain-sized flakes of tissue with a surgical scalpel from the interior of the fruit body near its base and placed them on three separate sterile agar petri dishes. The dishes were sealed with Parafilm® tape; labeled A, B, and C; and placed in a dark room at 24 C for 72 h to allow the mycelium to begin growing on the surface of the agar. It is typical that, in the first tissue transfer from the wild harvest samples, other contaminants also will be present growing on the agar. Pure T. versicolor mycelium was isolated away from these contaminants by excising another grain-sized portion of mycelium from the leading edge of mycelial growth on the original agar dish 3 d later. Seventy-two hours after the original transfer, and with mycelial growth visually present on all three agar dishes, the dishes were returned to the laminar flow hood. The leading edge of mycelium was transferred from each of the three dishes onto three new sterilized agar dishes. Five days later all three dishes showed mycelial growth without signs of contamination from other organisms. The dish with the fastest mycelial growth (dish B) was chosen to continue for spawn production.
Roughly 1.4 L of sorghum [Sorghum bicolor (L.) Moench] grain was left to hydrate 12 h in tap water, drained for 45 min, and divided equally among four 1-L heat-resistant glass jars that were then capped with synthetic filter disks (99.97% filtration down to 0.3 microns). The four jars were sterilized in a pressure cooker for 2 h at 103.4 kPa (~121 C). The pressure cooker was left to cool for 24 h and was then opened in front of a laminar flow hood, and the jars were placed in the sterile stream of air. Four separate 1 cm by 1 cm mycelial patches were cut from dish B, following sterile protocol, with one mycelial patch being placed in each of the four sterilized grain jars. The filter disks were then replaced. The jars were placed in a dark 24 C room for 10 d to allow the mycelium to colonize the grain. The jars were shaken by hand on days 2, 5, and 7 to speed colonization by evenly distributing the myceliated grains throughout the jars.
Under sterile conditions, the fully colonized grain was transferred to four separate commercial mushroom production bags, each containing 2 kg of hydrated and sterilized oak sawdust, and the bags were then heat-sealed. The four bags were immediately shaken to evenly distribute the grain, placed in a dark 24 C room, and left to colonize the oak sawdust for 14 d. On day 15, the four fully colonized bags were placed in 2 C cold storage until ready for use in field inoculation of the L. lucidum logs. City of Austin staff received the spawn shipment on February 20, 2015, and stored it in the refrigerator at 7 C until the day of log inoculation.
Inoculation of Ligustrum lucidum Logs
We inoculated 25 freshly cut L. lucidum logs that were 3.8 to 11.4 cm in diameter and 53 to 123 cm in length. We placed the 25 inoculated logs and 5 untreated control logs ~10 to 15 cm apart, end to end, along slope contours and buried the lower half of each log in the soil. We began the study at Site 1 on February 27, 2015, where City of Austin staff made a row of 7 shallow cuts ~1.9 to 2.5 cm below the depth of the bark, spaced ~10 to 15 cm apart, into the upper half of 9 logs by running a battery-operated circular saw perpendicular to the length of each log (Figure 1A). We covered the open cuts with a thin layer of sawdust spawn topped with newspaper wetted with creek water collected from the site (Figure 1B). For the controls, we scored 2 logs in the same manner as the treated logs but did not inoculate them. We placed the 2 controls on either end of the row of 9 treated logs.

Figure 1. Inoculation of Ligustrum lucidum logs with Trametes versicolor at Site 1, Balcones Canyonlands Preserve, Austin, TX, February 27, 2015. (A) A log with 7 shallow cuts made using a circular saw; (B) a log after cuts were inoculated with sawdust spawn and covered with wet newspaper.
At Site 2, City of Austin staff and volunteers inoculated 16 logs on March 15, 2015. While working with volunteers, we used a battery-operated drill and a 12-mm soft-steel drill screw-tip bit with stop (Figure 2A). We packed each hole with sawdust spawn and covered the top with plug wax (Figure 2B). To evaluate the labor intensity needed for effective treatments, we varied the density of inoculations at Site 2 by a factor of 3, with sets of 4 logs treated with 1, 3, 6, and 9 inoculation points, respectively. We then placed 1 untreated log on the south end and 2 untreated logs on the north end of the row of 16 treated logs as controls. No further treatment was provided at either site.
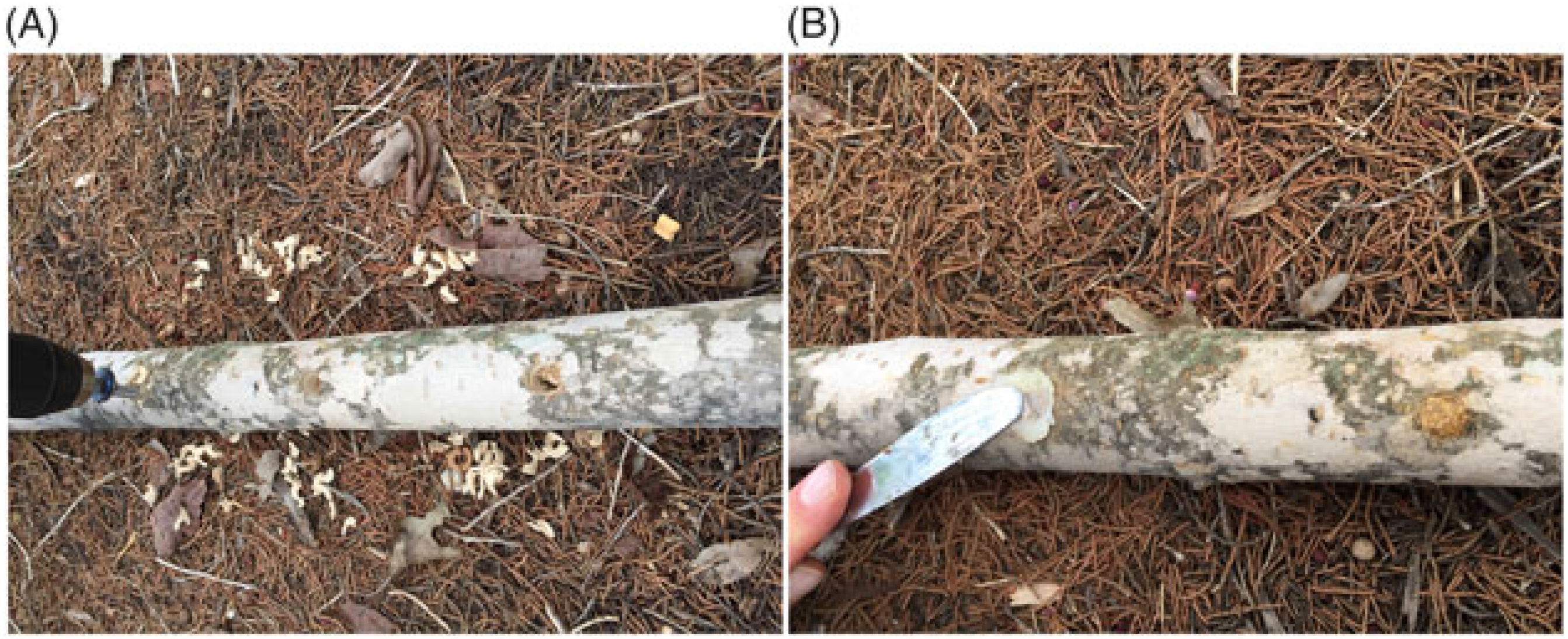
Figure 2. Inoculation of freshly cut Ligustrum lucidum log with Trametes versicolor at Site 2, Balcones Canyonlands Preserve, Austin, TX, March 15, 2015. (A) A log with holes drilled using a 12-mm drill bit with stop; (B) the holes filled with sawdust spawn and being covered with plug wax.
We conducted four post-inoculation visits at Site 1 on November 23, 2015, February 24, 2016, June 22, 2016, and March 5, 2018, and at Site 2 on November 15, 2015, January 31, 2016, August 16, 2016, and March 4, 2018. On each visit, we measured the decomposition stage of each log by assigning a decay class based on visual and tactile criteria (Russell et al. Reference Russell, Fraver, Aakala, Gove, Woodall, D’Amato and Ducey2015). For the first three visits, we conducted nondestructive examinations of the surface of each log by looking for visual evidence of wood decay, such as presence of mycelia and fruit bodies, and assigned a decay class using Grove et al. (Reference Grove, Stamm and Barry2009, p. 390) as follows: (1) “few signs of wood decay,” (2) “early signs of wood decay or discolouration,” and (3) “fungi may be prominent.” On the last visit, we used a 5-mm galvanized steel nail (13.1-cm long) to penetrate each log to estimate the level of decay based on the five decay classes adapted from Mäkipää and Linkosalo (Reference Mäkipää and Linkosalo2011, p. 1137): “(1) still hard wood—[nail] penetrates a few millimeters into the wood; (2) still fairly hard wood—[nail] penetrates 1–2 cm into the wood; (3) outer layer of the log fairly soft, core still hard—[nail] penetrates 3–5 cm into the wood; (4) soft throughout—[nail] penetrates all the way into the wood; (5) very soft—wood structure of logs already collapsed.” We took three measurements and used the average for each log.
In the analysis of decay of the L. lucidum logs, the response variable consists of the final five decay classes described. Because the decay classes were ordered (from low to high), we used an ordinal logistic regression (JMP software, v. 14.0.0, SAS Institute, Cary, NC) to examine how the number of inoculation points (0, 1, 3, 6, 7, 9) impacted decay in L. lucidum.
Results and Discussion
We observed early signs of wood decay (decay class 2) in the form of white mycelia on the ends and underneath the treated logs at both sites at 8 and 11 to 12 mo post-inoculation, and few signs of decay on the controls at either site (Figure 3). Within 16 to 17 mo post-inoculation, 100% of the logs with 6 to 9 inoculation points were covered with fruit bodies (decay class 3; Figures 3 and 4), as were 75% of the logs with 3 inoculation points. No mycelia or fruit bodies were observed on the control logs (Figure 3).

Figure 3. Decay class of 30 freshly cut Ligustrum lucidum logs (25 treatments, 5 controls) at two sites within the Balcones Canyonlands Preserve, Austin, TX, at 8, 11–12, and 16–17 mo following inoculation with Trametes versicolor, by the number of inoculation points (0, 1, 3, 6, 7, 9) per log. Controls were not inoculated. Decay classes were assigned based on a nondestructive visual assessment using Grove et al. (Reference Grove, Stamm and Barry2009, p. 390): (1) “few signs of wood decay,” (2) “early signs of wood decay or discolouration,” and (3) “fungi may be prominent.”
Three years after inoculation, all logs with >3 inoculation points were in decay classes 3 to 5 (Figures 3 and 5). At Site 1, 100% of the treated logs (7 inoculation points) were in decay classes 4 to 5. One of the control logs was developing T. versicolor fruit bodies and in decay class 2, but the other control remained in decay class 1. At Site 2, we found 3 of the treated logs with 1 inoculation point were still in decay classes 1 to 2, while the remaining 13 treated logs at Site 2 were in decay classes 3 to 5. None of the control logs at Site 2 had progressed beyond decay class 1 by the end of the study (Figures 5 and 6). The ordinal logistic regression (Table 1) shows that including the number of T. versicolor inoculations as a covariate significantly improves the model (P = 0.0006). With each unit of increase in the number of inoculation points, there was a 0.48 increase in the odds of the log being in a higher decay class (P = 0.0005) when site was held constant (Table 2).

Figure 4. Trametes versicolor fruit bodies on Ligustrum lucidum logs at Site 1 (16 mo post-inoculation, A) and Site 2 (17 mo post-inoculation, B), Balcones Canyonlands Preserve, Austin, TX.

Figure 5. Decay class of 30 freshly cut Ligustrum lucidum logs (25 treatments, 5 controls) at two sites within the Balcones Canyonlands Preserve, Austin, TX, 3 yr after inoculation with Trametes versicolor, by the number of inoculation points (0, 1, 3, 6, 7, 9) per log. Controls were not inoculated. Final decay classes were adapted from Mäkipää and Linkosalo (Reference Mäkipää and Linkosalo2011, p. 1137): “(1) still hard wood—[nail] penetrates a few millimeters into the wood; (2) still fairly hard wood—[nail] penetrates 1–2 cm into the wood; (3) outer layer of the log fairly soft, core still hard—[nail] penetrates 3–5 cm into the wood; (4) soft throughout—[nail] penetrates all the way into the wood; (5) very soft—wood structure of logs already collapsed.”
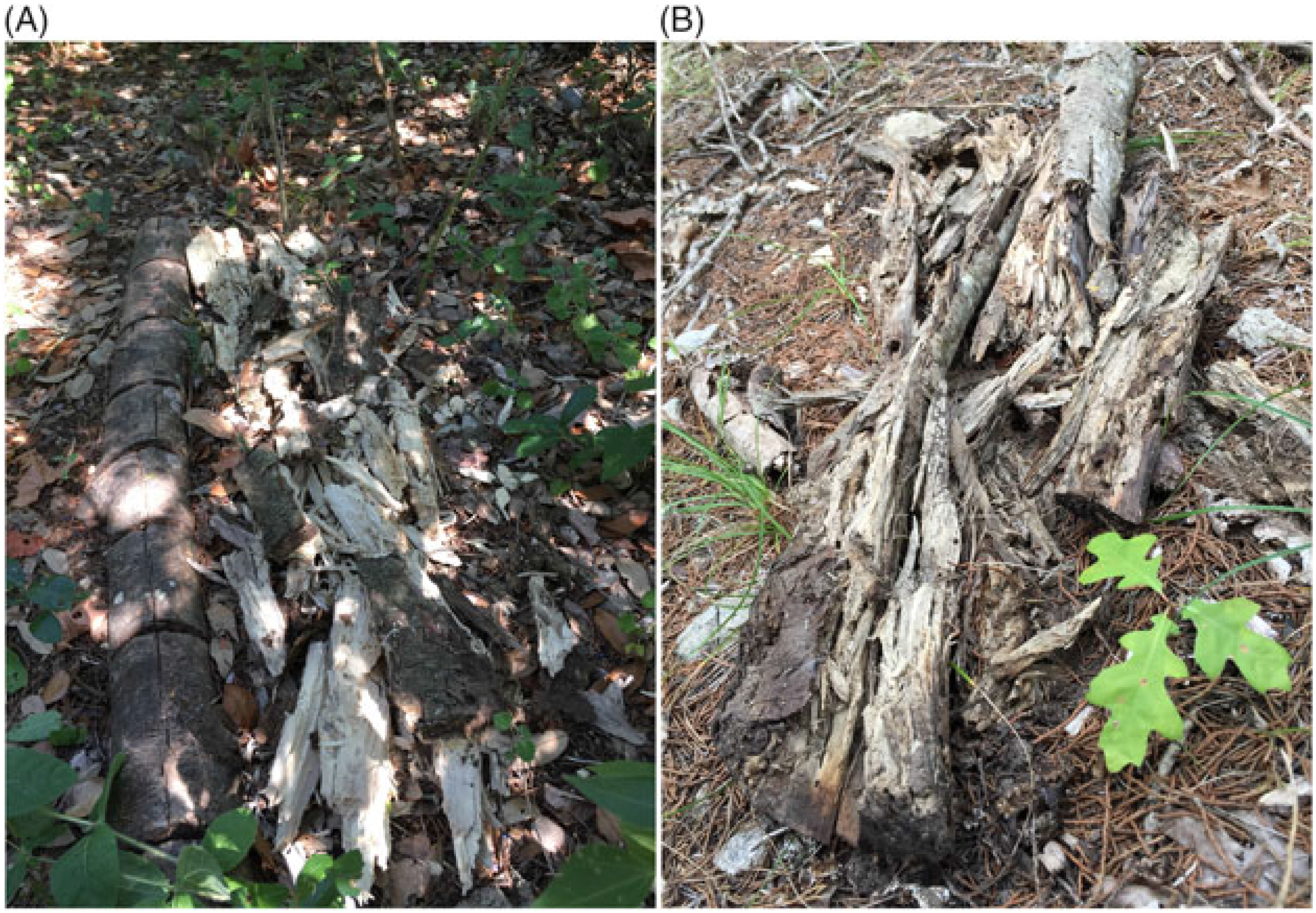
Figure 6. Comparison of control (not inoculated) Ligustrum lucidum log (left) and decomposed L. lucidum log 3 yr following inoculation with Trametes versicolor at Site 1 (A) and decomposed L. lucidum log 3 yr following inoculating with T. versicolor at Site 2 (B), Balcones Canyonlands Preserve, Austin, TX.
Table 1. Whole-model test of full versus reduced ordinal logistic regression examining the impact of the number of Trametes versicolor inoculation points (0, 1, 3, 6, 7, 9) on decay of Ligustrum lucidum logs 3 yr after inoculation within the Balcones Canyonlands Preserve, Austin, TX.

Table 2. Parameter estimates of ordinal logistic regression model examining the impact of the number of Trametes versicolor inoculation points (0, 1, 3, 6, 7, 9) on decay of Ligustrum lucidum logs 3 yr after inoculation within the Balcones Canyonlands Preserve, Austin, TX.

Critique of Our Pilot Study and Suggestions for Future Research
Wood-decaying fungi play an essential role in forest ecosystems. Their unique ability to digest wood releases nutrients (Marcot Reference Marcot2017b), contributes to carbon and water storage (Arnstadt et al. Reference Arnstadt, Hoppe, Kahl, Kellner, Krüger, Bauhus and Hofrichter2016), and provides habitat to a host of other organisms, including free-living nitrogen-fixing bacteria and mycorrhizal fungi (Lonsdale et al. Reference Lonsdale, Pautasso and Holdenrieder2008). The end product of the wood decomposition process is soil development needed for tree growth and forest regeneration. We explored whether wood-decaying fungi could be used to speed up this process to recycle an invasive hardwood back into the ecosystem. While the data set from our pilot study is limited, we found that inoculating L. lucidum logs with T. versicolor significantly accelerated wood decomposition at both of our study sites. By the end of our 3-yr study, all of the logs with >3 inoculation points were in advanced stages of decay. Interestingly, one of the control logs was covered with T. versicolor fruit bodies and beginning to decompose, so T. versicolor could have spread from the row of inoculated logs to the control log, or it could have been colonized from native spores or mycelia already present at the site. None of the other control logs showed noticeable signs of decay. Based on our preliminary results, we recommend spacing inoculation points every ~15 cm to promote more rapid growth of T. versicolor and wood decay.
While the potential for incorporating mushroom cultivation into sustainable forest management has been recognized (Garibay-Orijel et al. Reference Garibay-Orijel, Córdova, Cifuentes, Valenzuela, Estrada-Torres and Kong2009; Lonsdale et al. Reference Lonsdale, Pautasso and Holdenrieder2008; Marcot Reference Marcot2017a, Reference Marcot2017b), we have found few published studies that have tested the feasibility of using wood-decaying fungi to accelerate decomposition of wood debris in the field (Pavlík and Pavlík Reference Pavlík and Pavlík2013; Piškur et al. Reference Piškur, Bajc, Robek, Humar, Sinjur, Kadunc, Oven, Rep, Petkovšek, Kraigher, Jurc and Pohleven2011). Piškur et al. (Reference Piškur, Bajc, Robek, Humar, Sinjur, Kadunc, Oven, Rep, Petkovšek, Kraigher, Jurc and Pohleven2011) evaluated the mycoremediation potential of oyster mushrooms [Pleorotus ostreatus (Jacq.) P. Kumm.] a thermal power plant site covered with fly ash by inoculating fresh European beech (Fagus sylvatica L.) wood chips and comparing the results with noninoculated wood chips 6 and 17 mo later. Although temperature, relative humidity, and lignin degradation increased in the first 6 mo, after 17 mo they concluded that the naturally occurring processes of wood degradation at sites not inoculated with P. ostreatus were as efficient as those at the inoculated sites. Pavlík and Pavlík (Reference Pavlík and Pavlík2013) inoculated F. sylvatica and European aspen (Populus tremula L.) logs with P. ostreatus and reported that all logs produced fruit bodies and resulted in effective and rapid wood decomposition during their 5-yr study; none of the control logs formed P. ostreatus fruit bodies.
Trametes versicolor fruit bodies were flourishing on 75% of our treated logs with >3 inoculation points following a 16- to 17-mo incubation period. Used medicinally for centuries, fruit bodies can be consumed in teas and soups. Wild strains have been shown to inhibit tumor growth and are used to treat a wide variety of cancers (Hobbs Reference Hobbs2004). Research has shown that T. versicolor boosts the immune system by increasing natural killer cells, contains antiviral agents that may inhibit HIV replication, and has strong antibiotic compounds effective against several human pathogens (Chu et al. Reference Chu, Ho and Chow2002). More recent research shows promise of antioxidant and antiradical activity in treating neurodegenerative diseases (Janjušević et al. Reference Janjušević, Karaman, Šibul, Tommonaro, Iodice, Jakovljević and Pejin2017). Trametes versicolor is also known to break down a host of pollutants, including polycyclic aromatic hydrocarbons (Gao et al. Reference Gao, Du, Yang, Wu and Liang2010), contaminants in wastewater (Shreve et al. Reference Shreve, Brockman, Hartleb, Prebihalo, Dorman and Brennan2016; Torán et al. Reference Torán, Blánquez and Caminal2017), and several herbicides (da Silva Coelho-Moreira et al. Reference da Silva Coelho-Moreira, Maciel, Castoldi, da Silva Mariano, Inácio, Bracht, Peralta, Price and Kelton2013). We have anecdotally observed T. versicolor fruit bodies growing on an L. lucidum stump 3 yr after it was cut and the stump was painted with imazapyr (50% v/v), and along the root of a stump ~1 yr after it was cut and treated with triclopyr (50% v/v). These observations show promise for successful inoculations following herbicide treatment and bioremediation potential of T. versicolor.
While not part of our study, our volunteers have since inoculated ~35 additional L. lucidum logs, all with prolific fruit bodies and comparable results. They have also inoculated photinia (Photinia spp.) logs with T. versicolor and subsequently observed abundant fruit bodies and accelerated wood decay. Given its widespread distribution and aggressive growth, we expect T. versicolor would colonize and accelerate decomposition of most dead invasive hardwoods. Similarly, we chose the ubiquitous T. versicolor because we found it growing on L. lucidum logs within our study area. However, for any given site, we recommend conducting inventories (Garibay-Orijel et al. Reference Garibay-Orijel, Córdova, Cifuentes, Valenzuela, Estrada-Torres and Kong2009) to identify, collect, and culture native saprotrophic fungi suitable for inoculations. We provide detailed methods we used to culture a local strain of T. versicolor so they can be repeated. Alternatively, cultures of T. versicolor and other medicinal mushrooms are commercially available and can be custom made by commercial mycology labs. We have had successful results using commercially available T. versicolor spawn and recommend that future research investigate whether this could have an effect on native fungal communities.
We used freshly cut L. lucidum logs in our study because we wanted to test this method under likely field scenarios. We anticipated that a crew would inoculate the logs on the same day of tree felling rather than having to return on a different day, especially at a remote site. This method worked well in our study. Pavlík et al. (Reference Pavlík, Hrasko and Pavlikova2008) also found that P. ostreatus grew best on freshly cut F. sylvatica wood. Other investigations we believe would help inform land management decisions include inoculation of older logs, standing dead trees and cut stumps (e.g., following girdling or herbicide treatment via stem injection or application to cut stumps), and small stems with leaves and seeds, along with documenting the successional cascade following inoculation and comparing results with native deadwood.
In addition to accelerated decay, we are incorporating inoculated L. lucidum logs into forest restoration and remediation efforts. We use them as check logs (Jacke and Toensmeier Reference Jacke and Toensmeier2005) to help protect soils and control erosion; improve soil structure, diversity, and nutrients; infiltrate and store water; and provide habitat for soil biota and small wildlife. We bury the lower half of the inoculated check logs into the soil along slope contours in small groupings to capture and disperse runoff. Where native mulch is available, we add this to the upslope side of the logs to help absorb more water. By the end of our 3-yr study, our inoculated logs were spongy and moist, even during the hot, dry summer, demonstrating the importance of fungi for retaining moisture in the understory. We are taking advantage of these moisture reservoirs by planting seeds of native trees and shrubs on either side of inoculated logs to provide a source of water for the seedlings. These are some of the many ways that L. lucidum logs inoculated with T. versicolor can be used as a functional land management tool to help restore degraded environments.
Conclusions and Management Implications
Our experiment demonstrates that inoculating freshly cut L. lucidum logs with T. versicolor significantly accelerates the rate of wood decomposition and suggests a promising approach for more widespread application. The accelerated decay rate would allow for in situ recycling of the dead biomass to support soil formation, input of organic matter, and nutrient cycling, improving growing conditions for forest trees and promoting biodiversity conservation (Marcot Reference Marcot2017b). The accelerated decomposition of wood debris in turn reduces fire risk concerns. Inoculated logs can be strategically placed to assist with erosion control, water retention, pollutant filtering, and seedling establishment. An added benefit of inoculating logs with an aggressive wood-decaying fungus like T. versicolor is the proliferation of medicinal mushrooms, a potential nontimber forest product and revenue source for local communities (Garibay-Orijel et al. Reference Johnson2009). Inoculating logs in the field was a favorite activity of our volunteers, requiring little or no previous experience to achieve successful results. Applied on a larger scale, these medicinal saprotrophs have the potential to transform nonnative woody plants from a threat to an asset.
Author ORCIDs
O’Donnell, Lisa 0000-0002-0825-4553
Acknowledgments
We would like to thank the City of Austin for its support of this project. Many City of Austin staff and volunteers assisted with the inoculations and monitoring. Lynne Stokes provided statistical advice. This research received no specific grant from any funding agency or the commercial or not-for-profit sectors. No conflicts of interest have been declared.