Introduction
Ever since the discovery of subglacial Antarctic lakes, the possibility of life unique to this environment has fascinated science. Recent taxonomic classifications for gene sequences obtained from accretion ice of Lake Vostok in East Antarctica (Shtarkman et al., Reference Shtarkman, Kocer, Edgar, Veerapanemi, D'Elia, Morris and Rogers2013) and from lake water and sediments in subglacial lake Whillans in West Antarctica (Christner et al., Reference Christner, Priscu, Achberger, Barbante, Carter, Christianson, Michaud, Mikucki, Mitchell, Skidmore and Vick-Majors2014) again stimulate the discussion on the existence of subglacial lake life, and its origins. Such mostly pure water, high hydrostatic pressure, low temperature and locally oxygen-limited environments (Siegert et al., Reference Siegert, Ellis-Evans, Tranter, Petit, Slamantin and Priscu2001) may be challenging for life. However, improved knowledge of the physico-chemical environment prevailing in subglacial lakes has questioned how isolated – and unique – these environments really are. More attention must be paid to the physical and physiological instability of these systems over geological timescales when assessing conditions for the evolution – and endurance – of subglacial life. We hypothesize that the most stable conditions in subglacial lakes, allowing the development of unique metazoan life, may be around hydrothermal vent activity (Fig. 1).
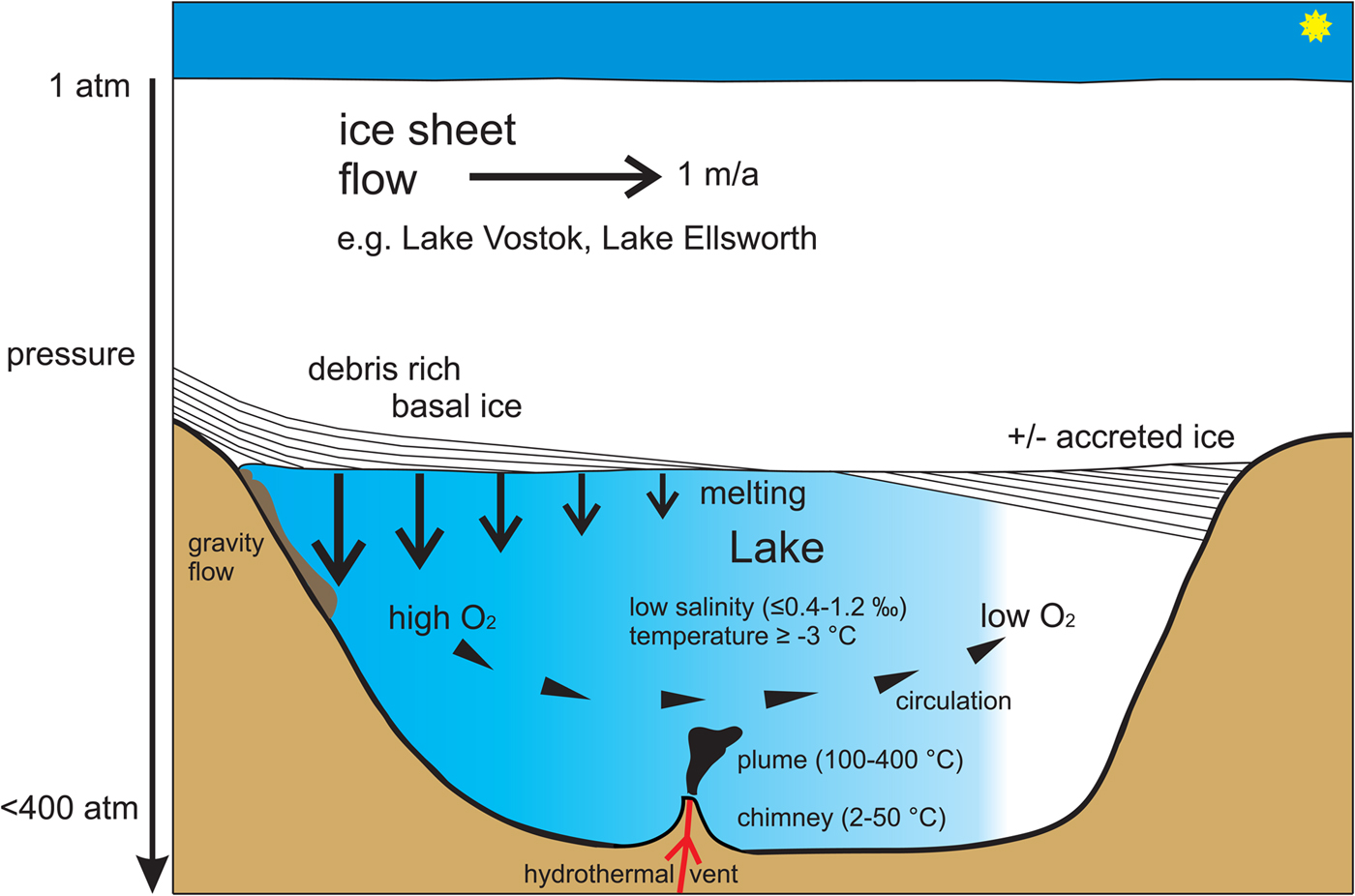
Fig. 1. Schematic representation of the physico-chemical conditions potentially found in a stable subglacial lake. Modified after Bentley et al. (Reference Bentley, Christoffersen, Hodgson, Smith, Tulaczyk and Le Brocq2011). Melting of basal ice releases oxygen, leading to circulation within the lake. Areas of low oxygen most likely prevail in areas of ice accretion. Under the high-pressure conditions found, water temperature reaches exceptionally low levels for a liquid water body.
Hyperbaric conditions to life
Lake Vostok is the largest of nearly 400 subglacial Antarctic lakes, which are often connected by rivers and streams, and is covered by nearly 4 km-thick ice (Siegert et al., Reference Siegert, Ellis-Evans, Tranter, Petit, Slamantin and Priscu2001; Wright and Siegert, Reference Wright and Siegert2012). The ice thickness varies between 3759 m over the south and 4150 m over the north of the lake. The depth of the lake also varies, reaching at least 1000 m on its southern side, although the lake floor may be covered with thick glacial sediment deposits typical of subglacial environments (Souchez et al., Reference Souchez, Petit, Tison, Jouzel and Verbeke2000; Siegert et al., Reference Siegert, Ellis-Evans, Tranter, Petit, Slamantin and Priscu2001; Bentley et al., Reference Bentley, Christoffersen, Hodgson, Smith, Tulaczyk and Le Brocq2011). The maximum pressure caused by the ice cap is ~337 bar at the southern side of the lake; pressure at the lake floor exceeds 400 bar (equivalent to 4000 m water depth). This makes Lake Vostok a unique high-pressure freshwater environment on Earth, ‘deeper’ than Lake Baikal (~1600 m); adaptation is certainly required to tolerate such pressures (Brown and Thatje, Reference Brown and Thatje2014). The water of Lake Vostok is also pure (salinity ≤0.4–1.2%), though the shallow embayment including accretion ice contains higher ion levels (Christner et al., Reference Christner, Royston-Bishop, Foreman, Arnold, Tranter, Welch, Lyons, Tsapin, Studinger and Priscu2006), and water temperature is as low as −3°C (Siegert et al., Reference Siegert, Ellis-Evans, Tranter, Petit, Slamantin and Priscu2001) (high pressure depresses the freezing point of water) (Fig. 1). The lowest temperature regime marine metazoan life is known to have adapted to that of the high Antarctic, where temperatures are generally as low as −1.85°C, the freezing point of surface seawater, but can drop down to −2.4°C under the Filchner Ice Shelf in the southern Weddell Sea (Nicholls et al., Reference Nicholls, Østerhus, Makinson, Gammelsrød and Fahrbach2009). Temperatures this low require special adaptations in metazoans, such as blood antifreeze found in ice-fish (Wohrmann, Reference Wohrmann1996). Oxygen is likely a limiting factor in subglacial Antarctic lake settings, too, in particular in the main basin of the lake, whether through chemical oxidation or microbial respiration; oxygen replenishment may depend upon release of gas hydrates from sediments or the melting underside of the overlying ice (Siegert et al., Reference Siegert, Ellis-Evans, Tranter, Petit, Slamantin and Priscu2001, Reference Siegert, Clarke, Mowlem, Ross, Hill, Tait, Hodgson, Parnell, Tranter, Pearce, Bentley, Cockell, Tsaloglou, Smith, Woodward, Brito and Waugh2012). Availability of dissolved oxygen probably varies throughout subglacial lakes, with oxygen decreasing with distance from areas of gas hydrate supply, i.e. from melting zones (Siegert et al., Reference Siegert, Ellis-Evans, Tranter, Petit, Slamantin and Priscu2001, Reference Siegert, Clarke, Mowlem, Ross, Hill, Tait, Hodgson, Parnell, Tranter, Pearce, Bentley, Cockell, Tsaloglou, Smith, Woodward, Brito and Waugh2012). Therefore, and unlike many other cold-water environments, Lake Vostok likely has some areas with hypoxic conditions, which should, in particular, be critical to the evolution of metazoans (Fig. 1). The suite of challenges arrayed in subglacial lakes is unique on Earth.
The age of the relatively stable centre of the East Antarctic Ice Sheet (up to 34 Ma) has often been suggested to favour the evolution of subglacial life. Evolution in situ is known to have resulted in the vast array of specialized metazoan life forms in the hydrographically isolated Southern Ocean (Fig. 2), and this analogy promotes ideas of fauna becoming isolated in subglacial lakes during ice sheet development, and/or the evolution of something completely new. However, overpressure accumulating in some subglacial lakes is released periodically, and large-scale outbursts may represent part of the lake cycle, resulting in substantial changes to pressure conditions found in some lakes on timescales of months to years (e.g. Bell Reference Bell2008; McMillan et al., Reference McMillan, Corr, Shepherd, Ridout, Laxon and Cullen2013). Tolerance to such physical change is crucial to organisms’ survival and most likely to occur in microorganisms. Such considerable changes in pressure, however, may not be problematic for life already adapted to deep-sea (high hydrostatic pressure) conditions; many deep-sea organisms are known to show a wide range of hydrostatic pressure tolerance, and sudden changes in pressure do affect microorganisms even less so, as long as these changes stay within their physiological tolerance (Brown and Thatje, Reference Brown and Thatje2014). Similar adaptations can be assumed for deep-water life from deep freshwater lakes such as Lake Baikal and Lake Tanganyika.
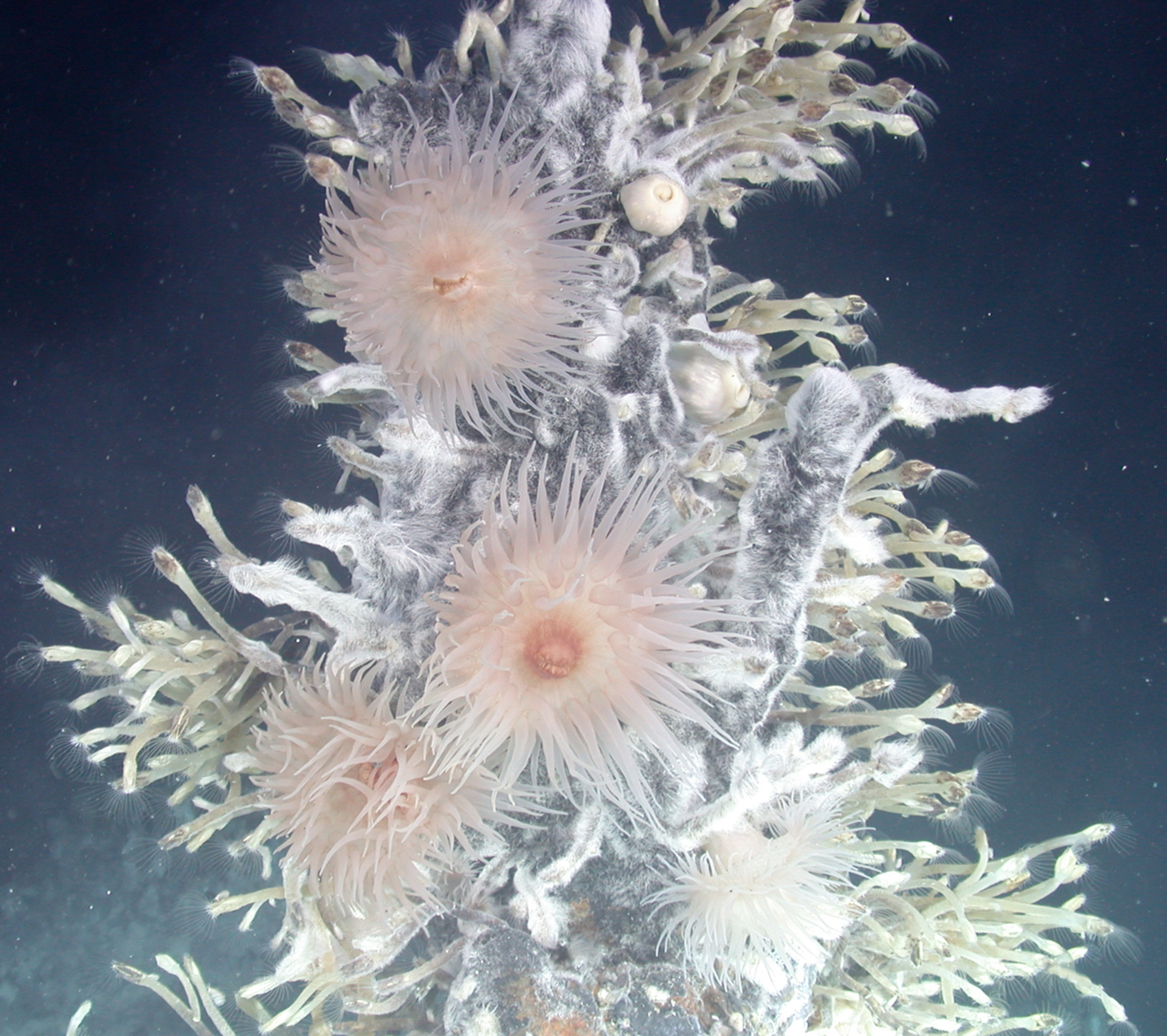
Fig. 2. A chemosynthetically driven hydrothermal vent system supporting a diverse array of life, including bacteria, stalked barnacles and anemones, East Scotia Ridge, Southern Ocean (Rogers et al., Reference Rogers, Tyler, Connelly, Copley, James, Larter, Linse, Mills, Naveira-Garabato, Pancost, Pearce, Polunin, German, Shank, Alker, Aquilina, Bennett, Clarke, Dinley, Graham, Green, Hawkes, Hepburn, Hilario, Huvenne, Marsh, Ramirez-Llodra, Reid, Roterman, Sweeting, Thatje and Zwirglmaier2012).
Hydrothermal activity
There is no doubt that Bacteria and at least some Archaea should find suitable conditions in subglacial lakes (Karl et al., Reference Karl, Bird, Björkman, Houlihan, Shackelford and Tupas2009; Rogers et al., Reference Rogers, Tyler, Connelly, Copley, James, Larter, Linse, Mills, Naveira-Garabato, Pancost, Pearce, Polunin, German, Shank, Alker, Aquilina, Bennett, Clarke, Dinley, Graham, Green, Hawkes, Hepburn, Hilario, Huvenne, Marsh, Ramirez-Llodra, Reid, Roterman, Sweeting, Thatje and Zwirglmaier2012; Christner et al., Reference Christner, Priscu, Achberger, Barbante, Carter, Christianson, Michaud, Mikucki, Mitchell, Skidmore and Vick-Majors2014; Boetius et al., Reference Boetius, Anesio, Deming, Mikucki and Rapp2015). However, the dissolved organic carbon input derived from overlying ice alone is calculated to be insufficient to support the reproductive growth of the microbial community inferred from cell concentrations in accretion ice above the lake (Christner et al., Reference Christner, Royston-Bishop, Foreman, Arnold, Tranter, Welch, Lyons, Tsapin, Studinger and Priscu2006). This strongly suggests that an alternative source of carbon is required to fuel life in subglacial lakes. For example, there is a large pool of dissolved organic carbon available, which probably results from the chemolithoautotrophic activity of ammonium oxidizing archaea and sulphide or iron oxidizing bacteria (Christner et al., Reference Christner, Priscu, Achberger, Barbante, Carter, Christianson, Michaud, Mikucki, Mitchell, Skidmore and Vick-Majors2014; Achberger et al., Reference Achberger, Christner, Michaud, Priscu, Skidmore and Vick Majors2016). The gene sequences reported from accretion ice above Lake Vostok are a striking indicator of active chemosynthetic processes and furthermore may indicate associated metazoans (Bulat et al., Reference Bulat, Alekhina, Blot, Petit, de Angelis, Wagenbach, Lipenkov, Vasilyeva, Wloch, Raynaud and Lukin2004; Shtarkman et al., Reference Shtarkman, Kocer, Edgar, Veerapanemi, D'Elia, Morris and Rogers2013); it should be noted that Christner et al. (Reference Christner, Priscu, Achberger, Barbante, Carter, Christianson, Michaud, Mikucki, Mitchell, Skidmore and Vick-Majors2014) did not confirm the presence of any eukaryotic sequences, which however may be the result of a limited set of primers used in their study.
Based on the sequence data (Shtarkman et al., Reference Shtarkman, Kocer, Edgar, Veerapanemi, D'Elia, Morris and Rogers2013), Lake Vostok appears to harbour a mixture of autotrophs and heterotrophs capable of performing nitrogen fixation, as well as carbon fixation, and nutrient recycling. Sequences found closest to those of psychrophiles and thermophiles indicate chemosynthesis driven by hydrothermal activity.
Hydrothermal vent activity may afford sustained physico-chemical conditions within the lake environment that allow for chemosynthetic primary production capable of sustaining a complex food web (Rogers et al., Reference Rogers, Tyler, Connelly, Copley, James, Larter, Linse, Mills, Naveira-Garabato, Pancost, Pearce, Polunin, German, Shank, Alker, Aquilina, Bennett, Clarke, Dinley, Graham, Green, Hawkes, Hepburn, Hilario, Huvenne, Marsh, Ramirez-Llodra, Reid, Roterman, Sweeting, Thatje and Zwirglmaier2012).
Hydrothermal activity is known from both Lake Baikal and Lake Tanganyika (Crane et al., Reference Crane, Hecker and Golubev1991; Tiercelin et al., Reference Tiercelin, Pflumio, Castrec, Boulégue, Gente, Rolet, Coussement, Stetter, Huber, Buku and Mifundu1993). Volcanism associated with continental rifting is widespread in West Antarctica (Van Wyk de Vries et al., Reference Van Wyk de Vries, Bingham, Hein, Siegert, Jamieson and White2018), where subglacial lakes such as Lake Whillans and Lake Ellsworth exist (Bentley et al., Reference Bentley, Christoffersen, Hodgson, Smith, Tulaczyk and Le Brocq2011). Lake Vostok lies within a very deep rift valley (Ferraccioli et al., Reference Ferraccioli, Finn, Jordan, Bell, Anderson and Damaske2011), which may increase the possibility of the existence of hydrothermal vents in the lake. Although the existence of any high-enthalpy mantle-derived hydrothermal input in Lake Vostok has been dismissed (Phillipe et al., Reference Phillipe, Petit, Lipenkov, Raynaud and Barkov2001), data support the existence of seismotectonically derived geothermal input (Petit et al., Reference Petit, Alekhina, Bulat, Gargaud, Barbier, Martin and Reisse2006). The presence of major ions in the shallower but not the deeper parts of the lake may also hint at hydrothermal activity (Christner et al., Reference Christner, Royston-Bishop, Foreman, Arnold, Tranter, Welch, Lyons, Tsapin, Studinger and Priscu2006); these shallow areas also showed high concentrations of biological material (Shtarkman et al., Reference Shtarkman, Kocer, Edgar, Veerapanemi, D'Elia, Morris and Rogers2013). A strong correlation between microbial signatures and higher ionic concentrations can be used to identify hydrothermal activity. Shtarkman et al. (Reference Shtarkman, Kocer, Edgar, Veerapanemi, D'Elia, Morris and Rogers2013) relate the presence of thermophilic bacteria to hydrothermal activity; however, and alternatively, geothermal activity may be sufficient to allow for chemosynthetic productivity to support the apparent diversity of life.
Hydrothermal activity provides a warm-water environment to life and temperatures of vent fluids can reach up to 400°C (Rogers et al., Reference Rogers, Tyler, Connelly, Copley, James, Larter, Linse, Mills, Naveira-Garabato, Pancost, Pearce, Polunin, German, Shank, Alker, Aquilina, Bennett, Clarke, Dinley, Graham, Green, Hawkes, Hepburn, Hilario, Huvenne, Marsh, Ramirez-Llodra, Reid, Roterman, Sweeting, Thatje and Zwirglmaier2012), and the deepest known hydrothermal vent site harbouring metazoans is situated in the Mid-Cayman spreading centre in the Caribbean at 4960 m (Connelly et al., Reference Connelly, Copley, Murton, Stansfield, Tyler, German, Van Dover, Amon, Furlong, Grindlay, Hayman, Hühnerbach, Judge, Le Bas, McPhail, Meier, Nakamura, Nye, Pebody, Pedersen, Plouviez, Sands, Searle, Stevenson, Taws and Wilcox2012). Deep-sea hydrothermal vents host some of the most thermophilic microorganisms known and some Archaea grow at temperatures of up to 122°C (Takai et al., Reference Takai, Nakamura, Toki, Tsunogai and Miyazaki2008). It should be highlighted, however, that vent metazoans thrive at much lower temperatures, in rare cases of up to 55°C (Ravaux et al., Reference Ravaux, Hamel, Zbinden, Tasiemski, Boutet, Leger, Tanguy, Jollivet and Shillito2013). Most sessile fauna shows preference for even lower temperatures of 5–11°C, while motile fauna is often found at higher temperatures (30–38°C), depending on the species and vent site (e.g. Takai et al., Reference Takai, Nakamura, Toki, Tsunogai and Miyazaki2008; Ravaux et al., Reference Ravaux, Hamel, Zbinden, Tasiemski, Boutet, Leger, Tanguy, Jollivet and Shillito2013; Thatje et al., Reference Thatje, Marsh, Roterman, Mavrogordato and Linse2015).
Life, as we know it
The unique challenges to life on Earth presented by Lake Vostok may also offer the best perspective on life to be expected in extra-terrestrial subglacial environments, such as on Jupiter's moon Europa (Carr et al., Reference Carr, Belton, Chapman, Davies, Geissler, Greenberg, McEwen, Tufts, Greeley, Sullivan, Head, Pappalardo, Klaasen, Johnson, Kaufman, Senske, Moore, Neukum, Schubert, Burns, Thomas and Veverka1998). Europa has a hot inner core and is suspected to have local tectonic activity, and its water is also pure or of very low salinity. Further, molecular hydrogen has been found in plumes of ice-covered Saturn's moon Enceladus, and it has been suggested that the most plausible source of this hydrogen is ongoing hydrothermal reactions of rock containing reduced minerals and organic materials (Waite et al., Reference Waite, Glein, Perryman, Teolis, Magee, Miller, Grimes, Perry, Miller, Bouquet, Lunine, Brockwell and Boltons2017). In such environments, some kind of chemosynthesis is the most likely source of energy for the evolution and sustenance of life, at least as we know it. Hydrothermal features in subglacial Lake Vostok may be the best place to look for deep branching lineages of metazoans that have evolved in a way that is analogous to life on Europa and Enceladus. Therefore, and despite all recent controversy, the increased evidence for chemosynthetic life in Lake Vostok should not be ignored; Lake Vostok may provide unique opportunities to the study of the origins of (metazoan) life.
Conclusions
The physico-chemical environment of subglacial lakes is from a physiological point of view strikingly different from any conditions found elsewhere in limnic and marine environments on Earth. Indeed, the most comparable conditions for life may be found at subglacial hydrothermal vents, which under subglacial conditions, however, do present a very limited size habitat for metazoans to thrive. While Bacteria and few Archaea appear to be widespread in sub-glacial lakes, the search for metazoan life should focus on areas surrounding hydrothermal activity. Away from such zones, physiological adaptations of life must be entirely different from what we currently know of life in polar deep seas and deep-water lakes, and such life form adaptations, though they may not be impossible, are currently difficult to imagine.
Acknowledgements
This study was supported by the University of Southampton, the British Antarctic Survey and the National Environment Research Council.
Author contributions
S.T. conceived the idea and wrote the paper. CDH and AB contributed to writing the paper.
Conflict of interest
None.
Author disclosure statement
No competing financial interests exist.