1. Introduction
In the outer part of most of mountain belts, several factors, such as lithology of sedimentary rocks that are deformed in the fold-and-thrust belt, basement morphology of foreland basin, mechanical property of thrust sheets, lithosphere rheology and slip rate of thrusting, are known to cause various styles of frontal thrusts (Morley, Reference Morley1986; Vann, Graham & Hayward, Reference Vann, Graham and Hayward1986; Dunne & Ferrill, Reference Dunne and Ferrill1988; Mouthereau, Watts & Burov, Reference Mouthereau, Watts and Burov2013). In some cases, such as the tectonic environment of pre-orogenic rifted continental margin characterized by array of the basement-involved normal faults and basement highs, stresses induced by arc-/continent-continent collision could transmit far beyond the mountain front (Letouzey, Reference Letouzey1986), cause the pre-existing tectonic settings to be reactivated and form high-angle thrusts or strike-slip faults (Sengor, Reference Sengor1976; Sengor, Burk & Dewey, Reference Sengor, Burk and Dewey1978; Jackson, Reference Jackson1980; Cohen, Reference Cohen1982; Brown, Reference Brown1984; Etheridge, Branson & Sturt-Smith, Reference Etheridge, Branson and Stuart-Smith1985; Letouzey, Reference Letouzey1986; Harding & Tuminas, Reference Harding and Tumimas1988; Cooper & Williams, Reference Cooper and Williams1989; Coward, Reference Coward and Hancock1994; Buchanan & Buchanan, Reference Buchanan and Buchanan1995; Lacombe & Mouthereau, Reference Lacombe and Mouthereau2002; Butler, Tavarnelli & Grasso, Reference Butler, Tavarnelli and Grasso2006; Nemčok, Mora & Cosgrove, Reference Nemčok, Mora, Cosgrove, Nemčok, Mora and Cosgrove2013), influencing foreland tectonics and thus shaping characteristic tectonic trends and structural styles of the belt front (Welbon & Butler, Reference Welbon, Butler, Larsen, Brekke, Larsen and Talleraas1992; Macedo & Marshak, Reference Macedo and Marshak1999; Lacombe et al. Reference Lacombe, Mouthereau, Angelier, Chu and Lee2003; Marshak, Reference Marshak and McClay2004; Yang et al. Reference Yang, Huang, Wu, Ting and Mei2006; Butler, Tavarnelli & Grasso, Reference Butler, Tavarnelli and Grasso2006). Variations in trends of the pre-existing normal fault with respect to the maximum compressional stress induced by crustal contraction cause various amounts and types of reactivated or inverted normal faults (Etheridge, Branson & Stuart-Smith, Reference Etheridge, Branson and Stuart-Smith1985; Sassi et al. Reference Sassi, Colletta, Bale and Paquereau1993). The style and the extent to which the pre-existing normal faults are involved in the orogenic belt would also determine the estimation of shortening across the deformed terranes (Mouthereau et al. Reference Mouthereau, Angelier and Lee2001; Lacombe & Mouthereau, Reference Lacombe and Mouthereau2002; Butler, Tavarnelli & Grasso, Reference Butler, Tavarnelli and Grasso2006; Mouthereau & Lacombe, Reference Mouthereau and Lacombe2006; Carola et al. Reference Carola, Tavani, Ferrer, Granado, Quinta, Butillé, Muñoz, Nemčok, Mora and Cosgrove2013; Carrera & Muñoz, Reference Carrera, Muñoz, Nemčok, Mora and Cosgrove2013; Jimenez et al. Reference Jimenez, Mora, Casallas, Silva, Tesón, Tamara, Namson, Higuera-Díaz, Lasso, Stockli, Nemčok, Mora and Cosgrove2013; Moreno et al. Reference Moreno, Silva, Mora, Tesón, Quintero, Rojas, Lopez, Blanco, Castellanos, Sanchez, Osorio, Namson, Stockli, Casallasw, Nemčok, Mora and Cosgrove2013; Tesón et al. Reference Tesón, Mora, Silva, Namson, Teixell, Castellanos, Casallas, Julivert, Taylor, Ibáñez-mejía, Valencia, Nemčok, Mora and Cosgrove2013).
In the young and active tectonic belt of Taiwan, the geological records in the foreland area, including the Western Foothills and the foreland basin offshore and onshore western Taiwan, not only reveal the early evolutionary history of the foreland itself but also provide key insights into thrust kinematics during mountain belt development. Taiwan is located at the convergent plate boundary between the Eurasia and Philippine Sea plates, and is one of the most active orogenic belts in the world caused by arc-continent collision (Biq, Reference Biq1972; Chai, Reference Chai1972; Tsai, Reference Tsai1978; Teng & Wang, Reference Teng and Wang1981) with a converging rate of 70km Ma−1 (Seno, Reference Seno1977; Suppe, Reference Suppe1984; Seno, Stein & Gripp, Reference Seno, Stein and Gripp1993). Before the orogeny, western Taiwan was located on the rifted passive margin and encountered at least two phases of early–middle Cenozoic rifting (Sun, Reference Sun1982; J. Yuan et al., unpub. report, 1989; Lin, Watts & Hesselbo, Reference Lin, Watts and Hesselbo2003; Yang et al. Reference Yang, Huang, Wu, Ting and Mei2006). The youngest pre-orogenic extensional phase began by middle–late Miocene time (Hsiao, Reference Hsiao1974; Tang, Reference Tang1977; Sun, Reference Sun1982; Leu, Chiang & Huang, Reference Leu, Chiang and Huang1985; Chow, Yuan & Yang, Reference Chow, Yuan and Yang1986; Chow, Yang & Chen, Reference Chow, Yang and Chen1987, Reference Chow, Yang and Chen1988; Yang, Ting & Yuan, Reference Yang, Ting and Yuan1991; Yuan et al. Reference Yuan, Yang, Chi, Hu and Chou1988; J. Yuan et al., unpub. report, 1989; Lin, Watts & Hesselbo, Reference Lin, Watts and Hesselbo2003) and formed two eastwards wide-opened rifted basins, the Tainan and Taihsi basins (Fig. 1), which are separated by the Peikang Basement High standing nearly perpendicular to the front of the thrust belt (Sun, Reference Sun1982; Yang, Ting & Yuan, Reference Yang, Ting and Yuan1991; Yang et al. Reference Yang, Huang, Wu, Ting and Mei2006). Since the initiation of orogeny at 7.1–4Ma (Chi, Namson & Suppe, Reference Chi, Namson and Suppe1981; Teng, Reference Teng1990; Huang et al. Reference Huang, Wu, Chang, Tsao, Yuan, Lin and Xia1997; Huang, Yuan & Tsao, Reference Huang, Yuan and Tsao2006; Mesalles et al. Reference Mesalles, Mouthereau, Bernet, Chang, Lin, Fillon and Sengelen2014), the entire mountain belt has gradually migrated westwards (Chi, Namson & Suppe, Reference Chi, Namson and Suppe1981; Suppe, Reference Suppe1981) while cannibalizing the pre-orogenic sequences accumulated in the sedimentary basins located on the margin of the Eurasian Plate (Covey, Reference Covey1984; Teng, Reference Teng1990).

Figure 1. Tectonic map of Taiwan and its adjacent areas (from Yang et al. Reference Yang, Huang, Wu, Ting and Mei2006). In this paper, the foreland areas cover the Taihsi and Tainan basins, the Peikang Basement High and the outer and inner fold-and-thrust belts (OFATB and IFATB). The horizon of time contours is a regional unconformity separating the Palaeogene and Neogene rifted basins.
Several models have been proposed to describe the kinematics and deformation mechanisms in arc-continent collision (Suppe, Reference Suppe1984; Teng, Reference Teng1990; Sibuet & Hsu, Reference Sibuet and Hsu1997; Lee et al. Reference Lee, Byrne, Wang, Lo, Rau and Lu2015), and they all refer to pre-orogenic basin architecture. The geometry of the geological structures of the basement highs and basement-involved normal faults which coexisted with the pre-orogenic sedimentary basins had a great influence on the spatial and temporal distribution of structural styles in the mountain belt (Meng, Reference Meng1962, Reference Meng1967; Chiu, Reference Chiu1970, Reference Chiu1971; Huang, Reference Huang1986, Reference Huang1987; Biq, Reference Biq1992; Lu, Reference Lu1994; Lu & Malavielle, Reference Lu and Malavieille1994; Lu et al. Reference Lu, Jeng, Chang and Jian1998; Yang et al. Reference Yang, Ting, Wu and Chi1997; Lacombe et al. Reference Lacombe, Mouthereau, Angelier and Deffontaines2001, Reference Lacombe, Mouthereau, Angelier, Chu and Lee2003; Mouthereau et al. Reference Mouthereau, Deffontaines, Lacombe, Angelier, Byrne and Liu2002). While some previous studies regarded the mountain belt in western Taiwan as a typical thin-skinned fault-and-thrust belt (Chiu, Reference Chiu1970, Reference Chiu1971; Suppe, Reference Suppe1976, Reference Suppe1980; Suppe & Namson, Reference Suppe and Namson1979; Namson, Reference Namson1981, Reference Namson1983, Reference Namson1984; Hung & Wiltschko, Reference Hung and Wiltschko1993; Hung et al. Reference Hung, Wiltschko, Lin, Hickman, Fang and Bock1999; Hickman et al. Reference Hickman, Wiltschko, Hung, Fang, Bock, Byrne and Liu2002), a few reactivated high-angle normal faults have been described in the deformation belt (Namson, Reference Namson1981; Suppe, Reference Suppe1984, Reference Suppe1986; Brown et al. Reference Brown, Alvarez-Marron, Schimmel, Wu and Camanni2012; Alvarez-Marron et al. Reference Alvarez-Marron, Brown, Camanni, Wu and Kuo-Chen2014). The effects of the pre-existing normal faults on the development of the fold-and-thrust belt have been investigated and evaluated in order to explain the complexity of geological structures in the outer part of the belt (Lee et al. Reference Lee, Chang, Mao and Tseng1993; Yang et al. Reference Yang, Wu, Ting, Wang, Chi and Kuo1994, Reference Yang, Wu, Wickham, Ting, Wang and Chi1996, Reference Yang, Ting, Wu and Chi1997; Mouthereau et al. Reference Mouthereau, Angelier and Lee2001, Reference Mouthereau, Deffontaines, Lacombe, Angelier, Byrne and Liu2002; Lacombe & Mouthereau, Reference Lacombe and Mouthereau2002; Lacombe et al. Reference Lacombe, Mouthereau, Angelier, Chu and Lee2003). Some previous studies depicted the major thrusts as the high-angle reactivated normal faults in Western Foothills and insist that the fold-and-thrust belt there has been primarily shaped by thick-skinned deformation (Chang et al. Reference Chang, Lee, Lin, Hsu and Mao1996; Mouthereau et al. Reference Mouthereau, Angelier and Lee2001; Lee, Chang & Coward, Reference Lee, Chang, Coward, Byrne and Liu2002). Lately, the thin- and thick-skinned models seem to be reconciled that all the reactivated normal faults in NW Taiwan are regarded as parts of the imbricate system and share a common décollement in the basement of the fold-and-thrust belt (Lacombe & Mouthereau, Reference Lacombe and Mouthereau2002; Mouthereau et al. Reference Mouthereau, Deffontaines, Lacombe, Angelier, Byrne and Liu2002). In our previous studies (Yang et al. Reference Yang, Wu, Wickham, Ting, Wang and Chi1996, Reference Yang, Ting, Wu and Chi1997, Reference Yang, Huang, Wu, Ting and Mei2006), we suggested that pre-existing normal faults have been selectively reactivated and interacting with low-angle thrust faults that were developed by thin-skinned process, and that their effects and occurrences in the Western Foothills and foreland basin should be confined to a limited extent.
The aforementioned various models indicate that the role of pre-existing basement-involved normal faults in the mountain-building process is still controversial and three key issues remand to be addressed. First, the pre-orogenic tectonics in the foreland area of the Taiwan Strait and western Taiwan is characterized by at least two phases of rifting. The normal faults which formed during the oldest phase and led to the opening of the South China Sea have been regarded as those reactivated latterly in the Western Foothills and Hsuehshan Range (Yue, Suppe & Hung, Reference Yue, Suppe and Hung2005; Mouthereau & Lacombe, Reference Mouthereau and Lacombe2006; Brown et al. Reference Brown, Alvarez-Marron, Schimmel, Wu and Camanni2012; Alvarez-Marron et al. Reference Alvarez-Marron, Brown, Camanni, Wu and Kuo-Chen2014). Reactivation of the normal faults that were formed during the younger phase of rifting has received less attention, except for that mentioned in Yang et al. (Reference Yang, Wu, Ting, Wang, Chi and Kuo1994). In the following we will demonstrate that the latter is more important than, or at least as important as, the former in shaping the foreland tectonics in western Taiwan. Second, the general trend of pre-existing normal faults is oblique to that of the orogenic belt, and the kinematics of interaction between pre-existing normal faults and low-angle thrusts in such a situation has been seldom addressed. Third, tectonic implications of variations in structural style and active tectonics related to normal fault reactivation along the strike of the fold-and-thrust belt need to be unravelled to infer inhomogeneity of crustal/lithospheric deformation, as addressed by Mouthereau et al. (Reference Mouthereau, Deffontaines, Lacombe, Angelier, Byrne and Liu2002). Yang et al. (Reference Yang, Huang, Wu, Ting and Mei2006) provided a thorough review on the studies of foreland tectonics in western Taiwan. Based on the previous results and some extra new data, this paper revisits some of the key structural settings in the foreland of western Taiwan and aims to reassess the role of pre-existing basement-involved normal faults in the development of recent tectonics. After providing detailed descriptions and discussing these, we comment on the applicability of various proposed tectonic models on the orogeny of Taiwan.
2. Regional geology and tectonics
2.a. Tectonic compartments
The tectonics in offshore and onshore western Taiwan are characterized by the structures of the pre-orogenic extensional tectonics and those of the outer fold-and-thrust belt that mingle with normal fault reactivation of the syn-orogenic tectonics (Fig. 1). Through the entire offshore western Taiwan, a regionally widespread unconformity corresponding to strata of upper Eocene – lower Oligocene age divides the Cenozoic basin system into Palaeogene and Neogene basins (Sun, Reference Sun1982; Lin, Watts & Hesselbo, Reference Lin, Watts and Hesselbo2003; Yang et al. Reference Yang, Huang, Wu, Ting and Mei2006). In the western part of the Taiwan Strait, the Palaeogene basins are half-grabens lining up in a left-lateral en échelon NE–SW trend, covered by thin Neogene and younger strata. In the eastern part of the strait, the Palaeogene basins have been overprinted by younger rift structures and covered by thick sediments in the Neogene basins. While the northwestern margin of the Neogene rift basins also strike NE–W, the single normal faults in the basins are E–W- to ENE–WSW-striking (Fig. 1; Sun, Reference Sun1982; Yang, Ting & Yuan, Reference Yang, Ting and Yuan1991; Huang, Chen & Chi, Reference Huang, Chen and Chi1993).
Tectonically, onshore Taiwan can be divided into five geological provinces (Fig. 1; Ho, Reference Ho1986; Huang et al. Reference Huang, Xia, Yuan and Chen2001): (1) the Coastal Range, composed of terranes of accreted volcanic island to the orogenic belt during the collision (Huang, Yuan & Tsao, Reference Huang, Yuan and Tsao2006); (2) the Central Range, characterized by metamorphic complex (schists in the eastern limb, the Basement Complex) and accreted terranes (slates in the western limb, the Backbone Range) (Huang et al. Reference Huang, Wu, Chang, Tsao, Yuan, Lin and Xia1997; Beyssac et al. Reference Beyssac, Simoes, Avouac, Farley, Chen, Chan and Goffé2007); (3) the Hsuehshan Range, formerly an Early Cenozoic graben formed in the continental margin and later accreted to the orogenic belt during the collision (Teng et al. Reference Teng, Wang, Tang, Huang, Huang, Yu and Ke1991); (4) the Western Foothills, the fold-and-thrust belt containing sedimentary sequences deposited in the passive margin and then in the foreland basin settings (Suppe, Reference Suppe1981; Yang et al. Reference Yang, Huang, Wu, Ting and Mei2006); and (5) the Coastal Plain in the central and southwestern parts of the island, the nearly undeformed foreland area. All these provinces are usually separated by large-scale thrusts or strike-slip faults.
The structures in the Western Foothills, which are mainly addressed in this paper, are generally characterized by unmetamorphosed deformation, open folding and imbricate thrust sheets (Ho, Reference Ho1982; Suppe, Reference Suppe1985). The tightness of folds decreases from east to west in the foothills belt, which indicates that the magnitude of deformation decreases toward the west (Namson, Reference Namson1981, Reference Namson1983, Reference Namson1984). The deformation front of the Western Foothills in northwestern Taiwan is now around the coastal line (Fig. 2).

Figure 2. Tectonic map of northwestern Taiwan showing spatial distribution of various types and regional trends of all faults (compiled and modified from Huang, Chen & Chi, Reference Huang, Chen and Chi1993 and Chinese Petroleum Corporation, 1978, 1994). The rose diagrams in the upper left and lower right represent regional trends of faults in offshore and onshore areas, respectively. The locations of faults in offshore area are from the contours of the Miocene top. The trends of faults in onshore area are divided into groups of that trending E–W (green) and that trending NE–SW (purple). See the discussions of their tectonic meaning in the text. HCUT – Hsinchu thrust; HCNT – Hsincheng thrust; CTU-PPT – Chutung-Peipu thrust; THPF – Touhuanping fault; LCKT – Luchukeng thrust; LKF – Lungkang fault; FTKF – Futoukeng fault; SIT – San-I thrust; TZCF – Tunzuchiao Fault. Anticlines: CHK – Chuhuangkeng; PS – Paoshan; YHS – Yunghoshan; CS – Chinshui; CTH – Chingtsaohu; CT – Chiting; CN – Chunan; PST – Paishatun; SH – Sanhu.
2.b. Characteristics of the pre-Neogene basement in the foreland
The pre-Neogene basement and its characteristics have been defined and discussed in Mouthereau et al. (Reference Mouthereau, Deffontaines, Lacombe, Angelier, Byrne and Liu2002) and Yang et al. (Reference Yang, Huang, Wu, Ting and Mei2006). Well-bore data in the foreland areas of western Taiwan reveal that the pre-Neogene basement is not metamorphosed but more compacted than the overlying Neogene sedimentary rocks (Huang, Reference Huang1967; Leu, Chiang & Huang, Reference Leu, Chiang and Huang1985; Fuh, Reference Fuh2000; Yang et al. Reference Yang, Wu, Cheng, Chen, Huang, Tsai, Wang and Ting2014) and its top has been regarded as the décollement below the fold-and-thrust belt in some balanced cross-sections across the Western Foothills (Suppe & Namson, Reference Suppe and Namson1979; Namson, Reference Namson1981, Reference Namson1983, Reference Namson1984; Hung & Wiltschko, Reference Hung and Wiltschko1993; Yang et al. Reference Yang, Huang, Wu, Ting, Mei, Lee, Hsu and Lee2007; Brown et al. Reference Brown, Alvarez-Marron, Schimmel, Wu and Camanni2012; Alvarez-Marron et al. Reference Alvarez-Marron, Brown, Camanni, Wu and Kuo-Chen2014). Stratigraphic sections based on well-bore data also show that the age of the basement rocks underlying the regional unconformity ranges from Mesozoic to Eocene across the basin (Yang et al. Reference Yang, Huang, Wu, Ting and Mei2006); only one deep well bore penetrates the Palaeozoic crystalline limestone in the subsurface of coastal plain in southwestern Taiwan (Jahn, Chi & Yui, Reference Jahn, Chi and Yui1992). The amount of erosion corresponding to the unconformity is up to 3500m in the basement high, an estimate based on sonic transit time log (Fuh, Reference Fuh2000), indicating that significant uplifting would have brought deeply seated rocks upwards in contact with much younger strata at shallow depth. The normal faults discussed in this paper all cut through the regional unconformity, and can therefore be defined as basement-involved normal faults (Leu, Chiang & Huang, Reference Leu, Chiang and Huang1985; J. Yuan et al., unpub. report, 1989; Yang, Ting & Yuan, Reference Yang, Ting and Yuan1991; Yang et al. Reference Yang, Huang, Wu, Ting and Mei2006, Reference Yang, Wu, Cheng, Chen, Huang, Tsai, Wang and Ting2014; Lin, Watts & Hesselbo, Reference Lin, Watts and Hesselbo2003).
2c. Fault structures in the foreland
Fault structures in the foreland of offshore and onshore western Taiwan can be grouped into three types: a high-angle normal fault; a high-angle thrust fault with or without significant strike-slip component; and a low-angle thrust fault. The first and second types are the normal faults formed during the youngest rift tectonics and the reactivated tectonics, respectively. The low-angle thrust faults are the major thrusts in the fold-and-thrust belt of Western Foothills. Distribution and intersecting relationships among these fault types can be shown in the tectonic maps depicting the detailed fault structures (Figs 2, 3).

Figure 3. Tectonic map of southwestern Taiwan showing spatial distribution of various types and regional trends of all faults (compiled and modified from J. Yuan et al., unpub. report, 1989 and Chinese Petroleum Corporation, 1986, 1989, 1992). The rose diagrams in the upper left and lower right represent regional trends of faults in offshore and onshore areas, respectively. The locations of faults in the offshore area are from the contours of the Palaeogene top, the regional unconformity separating the Palaeogene and Neogene strata. The trends of faults in onshore area are divided into groups of that trending E–W (green) and that trending NE–SW (purple). It is noticeable that, in the offshore area and coastal plain, all the faults are normal faults. CHT – Changhua thrust; CLPT – Chelungpu thrust; HMT – Hsiaomao thrust; SLKT – Shuilikeng thrust; CCKT – Chiuchiungkeng thrust; CKT – Chukou thrust; TCST – Tachienshan thrust; PLCT – Pinlangchai thrust; LKT – Luku thrust; MTST – Matoushan thrust; MSF – Meishan fault; HHAF – Hsinhua active fault.
In the Neogene Taihsi Basin of offshore northwestern Taiwan, the distribution and strike of the two types of high-angle fault are not uniform but vary in some spatial characteristics (Fig. 2). In the westernmost part of the basin, almost all normal faults still have their original attitude and sense of slip (Fig. 2; Huang, Reference Huang1986; Huang, Chen & Chi, Reference Huang, Chen and Chi1993). In the area closer to the coastal line, some normal faults have been inverted as high-angle thrusts with a strike-slip component (Huang, Reference Huang1986; Huang, Chen & Chi, Reference Huang, Chen and Chi1993). In this area normal faults and high-angle thrusts are alternatively distributed, but the high-angle thrusts appear more often in the eastern part of the faults of long lateral length in the eastern part of offshore the Taihsi Basin. Most of the faults are mainly striking in a range from N60°E to N100°E. There are also some fault segments striking N30°E. It is noticeable that some faults with main N60°E to N100°E strike extend onto the onshore area and connect with, or are parallel to, the exposed faults that mainly strike N70°E to N90°E.
In the onshore area, the Western Foothills can be divided into the outer and inner fold-and-thrust belts (Ho, Reference Ho1982) with a boundary along the Chuhuangkeng anticline, the Fangpokeng anticline (Peipu-Chutung thrust) and the Juanchiao thrust (Fig. 2). The trends of the anticlines and thrust faults in the outer fold-and-thrust belt are not uniform and can be grouped into two sets (Fig. 2). In the eastern part of the area that is adjacent to the Chuhuangkeng anticline, the trends of the anticlines and the associated low-angle thrust faults, including the Paoshan (Hsincheng thrust), Yunghoshan (Luchukeng thrust) and Chinshui anticlines, are mostly NNE–SSW, parallel to that of the mountain range which is ranging from N30°E to N60°E. In the western part of the area, which is adjacent to the coastal line, the high-angle faults and the accompanied anticlines, including the Chingtsaohu, Chiting, Chunan, Paishatun and Sanhu anticlines, are striking N70°E to N90°E, parallel to the high-angle normal faults, non-reactivated and reactivated, in the offshore area but at a high angle to the general trend of the orogenic belt. The high-angle faults segment the low-angle thrusts and the accompanied anticlines in the outer fold-and-thrust belt are cut off by the thrust faults in the inner fold-and-thrust belt to the east.
In the Neogene Tainan Basin, offshore southwestern Taiwan, all the faults are high-angle normal faults striking from N70°E to N90°E (Fig. 3) in contrast with that in offshore northwestern Taiwan (Fig. 2). In the coastal plain area most normal faults are E–W-striking. Some normal faults and a few normal fault-inverted thrusts in the frontal area of the fold-and-thrust belt are striking N10°E to N40°E. The normal faults in the coastal plain area extend to the east and are cut off by the low-angle frontal thrust of the Western Foothills.
3. Structural styles of normal fault reactivation
In western Taiwan, normal fault reactivation can be manifested by fault plane geometry of reactivated single normal faults and interaction between reactivated normal faults and low-angle major thrusts. The structural styles of normal fault reactivation are demonstrated in the seismic and balanced cross-sections. Abbreviations used for the formations and faults in the interpreted sections are listed in Figure 4 and Table 1, respectively.

Figure 4. Lithostratigraphic units of western Taiwan.
Table 1. Faults within the Western Foothills of Taiwan

3.a. Reactivation of single normal faults
This paper uses a grid of seismic sections and well-bore data to constrain strata on both sides of reactivated normal faults and to reconstruct geometry of a fault plane (Figs 2, 5a) in the northeastern margin of the Taihsi Basin. Thickening of sedimentary strata in the hanging wall of a high-angle thrust indicates that a high-angle normal fault (F3-E) has been partially reactivated as a high-angle thrust (Fig. 5b). To the west, the upper part of the N-dipping fault plain is rotated and replaced by a S-dipping high-angle thrust (F3) (Fig. 5c). Further westwards, the inversion displacement is transferred southwards to another S-dipping high-angle thrust (F1) (Fig. 5d). The displacement along the high-angle thrust is the largest in the middle part of the fault plane (Fig. 5d) and decreases laterally to its ends (Fig. 5e), where the fault still maintains normal fault displacement.

Figure 5. Reactivation of single normal faults in offshore northwestern Taiwan. (a) Geometry of fault plane and the lateral variation in fault type and dip direction of reactivated normal fault array. See the location of map in Figure 2. (b–e) A series of seismic sections, showing how displacement of reactivation increases laterally from the eastern part of a reactivated normal fault (F3) to its western part and is transferred to the next reactivated normal fault (F1). Section lines 1 and 3 show that F3-E has been rotated by reactivation displacement along F3 and its shallow part merges with F3. See ages of the formations in Figures 2 and 4.
Similar structural styles associated with reactivation of single normal faults can be observed in onshore northwestern Taiwan. Most of them have been documented in Yang et al. (Reference Yang, Wu, Wickham, Ting, Wang and Chi1996, Reference Yang, Ting, Wu and Chi1997) and cited in Lacombe & Mouthereau (Reference Lacombe and Mouthereau2002) and Lacombe et al. (Reference Lacombe, Mouthereau, Angelier, Chu and Lee2003) to propose their basement-involved tectonic model of NW Taiwan. The latest review of the studies of this subject can be found in Yang et al. (Reference Yang, Huang, Wu, Ting and Mei2006). However, detailed discussion on the characteristics of this kind of structure is still rare. In Yang et al. (Reference Yang, Wu, Wickham, Ting, Wang and Chi1996) the subsurface structure of the Hsinchu thrusts, which is E–W-striking and is usually regarded as having originated from normal fault reactivation, is characterized by three splays with one cutting downwards at a high angle and the others becoming soling in the lower Miocene strata (Fig. 6a). The upper Miocene and lower Pliocene sequences deposited during the latest extensional tectonics are thicker in the footwall of the high-angle thrust (Fig. 6a), indicating that the thrust was first an oppositely dipping normal fault. The structural style can be compared with that in the offshore area (Figs 5c, 6). Since the soled-out splays cut through the forelimb of the anticline in the hanging wall of the high-angle thrust, it is obvious that they started to develop after the high-angle normal fault was reactivated. This interpretation suggests that, without any late-coming low-angle or high-angle thrusting, the normal fault could be rotated and reactivated simply by regional contractional tectonics.

Figure 6. Seismic section in outer fold and thrust of northwestern Taiwan showing splays of high-angle reactivated normal faults. Line DA shows three splays of the Hsinchu fault in the subsurface (modified from Yang et al. Reference Yang, Wu, Wickham, Ting, Wang and Chi1996). The splays that are soled-out in the lower Miocene strata are constrained by well-bore data and the high-angle splay is interpreted using the cut-off strong reflectors in the footwall of the splays. The splays cut through the forelimb of the anticline in the hanging wall of the high-angle Hsinchu fault. See locations of the seismic sections and ages of the formations in Figure 2.
3.b. Interaction between reactivated normal faults and low-angle thrusts
Since the trend of pre-existing normal faults is oblique to the structural grain of the orogenic belt in western Taiwan, the late development of structural features affected by the inherited structures would be more complex than in any other orogenic belt. There are two aspects regarding the complexity of structural evolution in the Western Foothills of Taiwan: how the single low-angle thrusts in the fold-and-thrust belt of Western Foothills evolved and linked with the adjacent thrusts; and how they propagated towards the foreland. What will be addressed in the following is the effect of a pre-existing normal fault on the development of low-angle major thrusts.
3.b.1. Segmentation of major thrusts in the outer fold-and-thrust belt
Segmentation of the NNE–SSW-trending major thrusts by high-angle reactivated normal faults in the outer fold-and-thrust belt of onshore northwestern Taiwan (Fig. 2) has been described and analysed by Yang et al. (Reference Yang, Wu, Wickham, Ting, Wang and Chi1996, Reference Yang, Ting, Wu and Chi1997). Their studies show that, taking the Luchukeng thrust-Touhuangping fault-Hsincheng thrust for example, the structural features of low-angle thrusts are not symmetrical on both sides of the high-angle transverse fault. In the geological map (Fig. 2), the southern part of the low-angle Luchukeng thrust and the accompanied fold are cut through, not terminated, by two E–W-trending transverse faults: the Lungkang fault and the other transverse fault to its south. The northern end of the thrust is cut off by another transverse fault, the Touhuanping fault, and separated from the low-angle Hsincheng thrust to the north. While the sense of offset between the Hsincheng and Luchukeng thrusts is sinistral across the Touhuanping fault, the southwestern segments of the Luchukeng thrust are offset by the Lungkang and the second transverse faults in a dextral sense. An interpreted seismic section striking parallel to the low-angle thrust (Fig. 7) demonstrates that, on the southern side of the transverse fault, the low-angle Luchukeng thrust cuts up-section through a lateral ramp to meet the transverse fault, whereas on the other side the low-angle Hsincheng thrust was sharply cut off by the transverse fault (Yang et al. Reference Yang, Ting, Wu and Chi1997, Reference Yang, Huang, Wu, Ting and Mei2006).
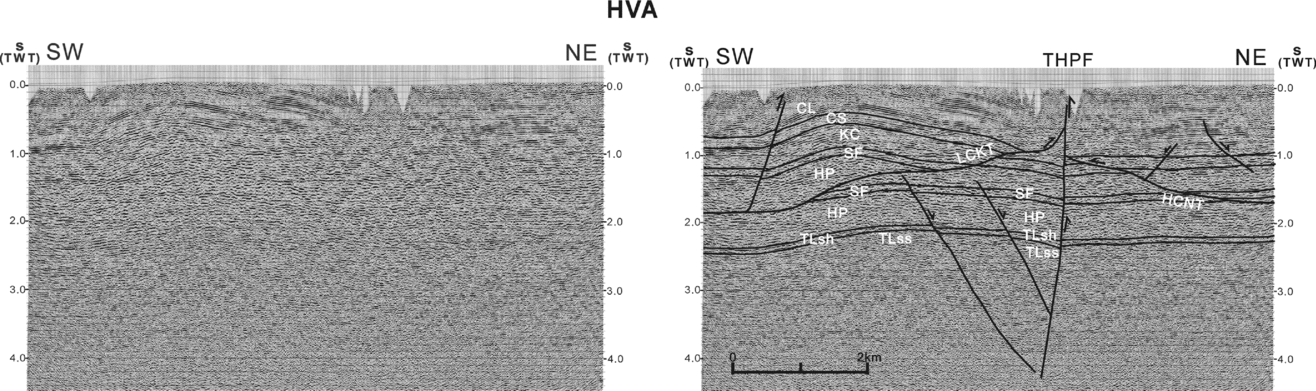
Figure 7. Seismic sections demonstrating interaction between the NNE-SSW trending major thrusts and high-angle reactivated normal faults in the fold-and-thrust belt of onshore northwestern Taiwan (Yang et al. Reference Yang, Huang, Wu, Ting and Mei2006). See locations of seismic sections in Figure 2. Seismic section is running across a transverse fault, the Touhuanping fault, in the outer fold-and-thrust and demonstrating different structural features on both sides of the fault. On the southwestern side of the transverse fault, a thrust fault, the Luchukeng thrust, is merged with the shallow part of the high-angle fault and forms its own lateral ramp while another thrust, the Hsincheng thrust, on the northeastern side of the high-angle fault is cut off by the fault. See locations of the seismic sections and ages of the formations in Figure 2.
The subsurface structure of the Luchukeng thrust is reconstructed in detail using a grid of interpreted seismic sections across the structure. The Yunghoshan anticline is cut through by the low-angle Luchukeng thrust, which is folded conformably with the anticline in the subsurface (Fig. 8a). Such a structural style is structurally different from the Chinshui structure, which is rather characterized by a simple anticline (Fig. 8b) although cut through by a high-angle thrust. The reconstructed geometry of the fault plane shows that the low-angle thrust extends southwards and changes to a high-angle thrust cutting through the forelimb of the Chinshui anticline (Fig. 9). There are two lateral ramps striking ENE–WSW at both ends of the NNE–SSW-trending low-angle thrust. The separation between the stratal cutoff lines on the hanging wall and footwall decreases gradually towards the SW and diminishes abruptly at the place where the thrust is cut through by the Lungkang fault.

Figure 8. Seismic sections running through the Luchukeng NNE–SSW-trending thrust in the outer fold-and-thrust belt, showing variation in the structural styles in the two adjacent Yunghoshan and Chinshui structures. (a) Seismic section showing structural feature of the low-angle Luchukeng thrust, and (b) that showing structural feature of the high-angle Luchukeng thrust in the subsurface. See locations of the seismic sections and ages of the formations in Figure 2.
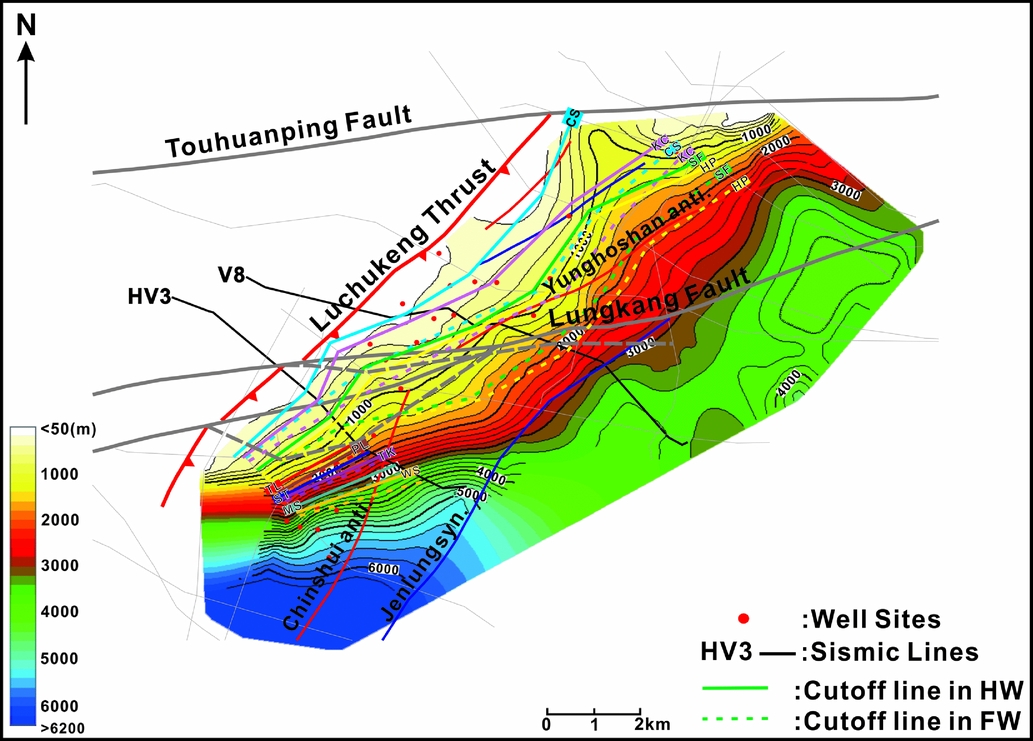
Figure 9. 3D geometry of the Luchukeng thrust in the subsurface and cutoff lines of the hanging wall and footwall strata. The thrust has two lateral ramps, which are parallel to transverse faults at both ends of the fault. It is noticeable that the southern lateral ramp, although cut through by the Lungkang fault, extends all the way down to the lower part of the Chinshui structure. See locations of the seismic sections and ages of the formations in Figure 2.
3.b.2. Development of major thrust geometry in inner fold-and-thrust belt
In the southern part of onshore northwestern Taiwan, the San-I thrust strikes NNE–SSW on the surface, extending northwards and turning into an E–W-aligned trend at its northern end (Fig. 2). In its hanging wall adjacent to the fault trace, the stratal boundaries are running almost parallel to the bending fault trace. The bend of fault trace implies a 3D subsurface geometry such that the E–W-trending fault segment reflects the lateral ramp of the low-angle thrust (Hung & Wiltschko, Reference Hung and Wiltschko1993). In the footwall of the E–W-striking San-I thrust, the southern end of the steeply dipping western limb of the Chuhuangkeng anticline is cut off by the thrust. In Hung & Wiltschko (Reference Hung and Wiltschko1993), a balanced cross-section across the northernmost part of the hanging wall of the thrust shows that the cut-off Chuhuangkeng anticline on the surface actually extends southwards in the subsurface and becomes the structure in the footwall of the thrust, where the low-angle thrust is characterized by an upfolded geometry. The folded strata in the central part of hanging wall of the thrust are striking NE–SW (Fig. 2), implying another characteristic of subsurface 3D geometry of the thrust and footwall structure.
A series of interpreted seismic sections and published balanced cross-sections (Yang et al. Reference Yang, Huang, Wu, Ting, Mei, Lee, Hsu and Lee2007) show that the San-I thrust in the subsurface changes from convex-upwards to concave-upwards toward the SW (Figs 10a–c, 11a–c). The convex-upwards geometry is related to upfolding in its footwall, in which the localized fold structure is tighter towards the NE. The structure of the footwall of the thrust is also characterized by a series of pre-existing high-angle normal faults. The fold structure is closely accompanied by a convex-upwards high-angle fault, which coexists with a series of pre-existing high-angle normal faults (Figs 10a–c, 11a–c). A reconstructed structural contour map indicates that the convex-upwards high-angle fault is striking ENE–WSW (Figs 10d, 11d), parallel to the general trend of the reactivated normal faults to the north. The magnitude and sense of displacement are not uniform along the fault plane. The largest magnitude of original normal fault displacement along the high-angle fault appears in the northernmost part of the area, where it is characterized by the tightest fold in the footwall of the San-I thrust (Fig. 11a). The convex-upwards high-angle fault is a reactivated normal fault and its formation mechanism is similar to that of the Hsinchu fault.

Figure 10. Variation in subsurface structural feature of the San-I thrust and its footwall constrained by seismic interpretation. (a–c) Seismic sections showing that the San-I thrust in the subsurface changes from convex-upwards to concave-upwards toward the SW. The seismic sections also show a series of non-reactivated normal faults and a reactivated high-angle normal fault in the footwall of the thrust. Interpretation of seismic section V5 is from Yang et al. (Reference Yang, Huang, Wu, Ting, Mei, Lee, Hsu and Lee2007). (d) Structural contours of the Talu Formation top in the footwall of the San-I thrust showing fault structures with various trends. The contour map shows the epicentre of the Hsinchu-Taichung Earthquake (1935) at the reactivated normal fault (Lin, Reference Lin2005), which is trending ENE–WSW. See locations of the seismic sections in Figure 10d and ages of the formations in Figures 2 and 4.

Figure 11. Balanced cross-sections and 3D geometry of faults showing variation in subsurface structural features of the San-I thrust and its footwall. (a–c) Balanced cross-sections run through the the San-I area and show variation in the geometry of upfolded San-I thrust and tightness of the fold in its footwall in the subsurface (Yang et al. Reference Yang, Huang, Wu, Ting, Mei, Lee, Hsu and Lee2007). In the balanced cross-section running through the southern part of the area San-I thrust is characterized by concave-upwards geometry, which represents the original geometry before the thrust was upfolded. The non-reactivated normal faults and the high-angle reactivated normal fault in the footwall of the thrust is interpreted based on seismic interpretation. (d) The 3D geometry of the low-angle San-I thrust and the high-angle reactivated normal fault is constructed by linking the faults on the balanced cross-sections. It is shown that, in the footwall of the low-angle San-I thrust (yellow colour), the high-angle reactivated normal faults (dark red colour) is trending ENE–WSW, parallel to that of the Tunzuchiao fault, the surface rupture of the Hsinchu-Taichung Earthquake (1935). See locations of the balanced cross-sections in Figure 2 and ages of the formations in Figures 2 and 4.
In central Taiwan where the Peikang Basement High stands in front of the Western Foothills, the thickness of the upper Miocene and Pliocene strata in the hanging wall of the Chelungpu-Tachienshan-Chukou thrust is twice that in the footwall (Fig. 12; Yang et al. Reference Yang, Huang, Wu, Ting, Mei, Lee, Hsu and Lee2007). Balancing the thickening of Pliocene strata in the hanging wall of the major thrusts requires that normal faults exist somewhere to the east of the exposed major thrusts and beneath the décollement (Figs 13, 14). The subsurface geometry of the Tachienshan-Chukou thrust also varies from the north to the south, depending on the magnitude of the slip along the Chiuchiungkeng fault (Fig. 14b–d). In the northern part of the area, the greater slip along the Chiuchiungkeng fault formed the lower-angle Tachienshan thrust. To the south where displacement along the Chiuchiungkeng fault decreases to zero, the Chukou thrust fault appears as a high-angle thrust cutting up through the surface. The variations in structural features along-strike of the thrust system and the restoration of two balanced cross-sections (Figs 13–16) suggest that, during the development of the Chelungpu and Tachienshan thrusts, the foreland-ward propagating décollement or low-angle thrusts met the high-angle normal faults and utilized the shallow part of the normal fault as a ramp to climb up to the surface. The ramps were then translated by slip along the Changhua and Chiuchiungkeng thrusts, and left the cut-off normal faults beneath the décollements. The localized thrust ramps and normal faults in different cross-sections can be connected to demonstrate the 3D geometry of the subsurface high-angle fault plane, indicating that their strikes are oblique to that of the major thrust in Western Foothills but parallel to that of the pre-existing normal fault in the foreland basin (Figs 13d, 14e).

Figure 12. Stratal thickening across the major thrusts in (a) central and (b) southwestern parts of the fold-and-thrust belt (Yang et al. Reference Yang, Huang, Wu, Ting, Mei, Lee, Hsu and Lee2007). The thickened strata in the hanging wall of the major thrusts are those deposited during the latest extension and foreland basin periods. The strata on both sides of the major thrusts are given different formation names, indicating different lithofacies for the strata. This implies that either the major thrusts result from reactivation of large-scale normal faults or they are low-angle thrusts with significant horizontal component of slip. Balanced cross-sections in Figures 13 and 14 favour the latter.

Figure 13. A series of balanced cross-sections through the fold-and-thrust belt in central Taiwan (Yang et al. Reference Yang, Huang, Wu, Ting, Mei, Lee, Hsu and Lee2007). (a) On line 4, the décollements of the Changhua and Chelungpu thrusts are along different formations but merged with each other in the subsurface. A normal fault exists underneath the common décollement and accommodates the thickened Cholan Formation in the hanging wall of the Chelungpu thrust. (b) The star on line 5 represents the main shock of the Chi-Chi Earthquake and is located very close to the pre-existing normal fault underneath the common décollement. (c) The structural features on line 6 are very similar to that on lines 4 and 5, but the normal fault underneath the décollement is located closer to the bend from the décollement to the ramp of the Chelungpu thrust. (d) The 3D geometry of the low-angle Chelungpu thrust and the high-angle reactivated normal fault is constructed by linking the faults on the balanced cross-sections and shows that, in the footwall of the low-angle Chelungpu (yellow colour), the high-angle reactivated normal faults (dark red colour) is trending ENE–WSW. See locations of the balanced cross-sections in Figure 3.

Figure 14. (a–d) A series of balanced cross-sections through the foreland basin and fold-and-thrust belt in southwestern Taiwan. Also shown in the figure is (e) the 3D geometry of the Tachienshan thrust (yellow colour) and a normal fault (blue colour with contours) that acts as the frontal ramp of the thrust in the subsurface. Lines 7, 8 and 10 are from Yang et al. (Reference Yang, Huang, Wu, Ting and Mei2006, Reference Yang, Huang, Wu, Ting, Mei, Lee, Hsu and Lee2007). The contours of the normal fault underneath the Tachienshan thrust indicate that the strike of the normal fault is parallel to that of the Meishan fault.

Figure 15. Restoration of line 6 showing evolution of slip along the Chelungpu and Changhua thrusts. (a) Balanced cross-section of line 6. (b–c) Restorations made on the slip along the Changhua thrust and then the Chelungpu thrust. The restoration also demonstrates how the locations of the normal faults underneath the décollement in all the balanced cross-sections are determined by restoring the slip along the Changhua thrust to make the ramp of the Chelungpu thrust on the line of the normal fault.

Figure 16. (a) Restoration along the thrusts on the balanced cross-section line 8. (b) Restoration is made first on the slip along the Chiuchiungkeng thrust to locate the shallow ramp of the Tachienshan thrust on the line of the normal fault. (c) At the next stage, slip along the Tachienshan thrust is restored to eliminate the fault-bend structure in the hanging wall of the thrust. The geometry of the Tachienshan thrust fault in (b) can be compared to that of the Chukou thrust on line 10.
4. Today's active normal fault reactivation
In onshore western Taiwan, the surface traces of some thrusts that were addressed in the previous sections are still active today and have been defined as active faults by the Central Geology Survey, Taiwan (Lin et al. Reference Lin, Chang, Lu, Shih and Huang2000). Some of them and the others located in the front of the fold-and-thrust belt are striking parallel to the structural trends of the regional extensional tectonics, and may therefore be related to normal fault reactivation. Some active faults are major thrusts that are viewed as a boundary between the outer and inner fold-and-thrust fault (Fig. 3). Some historical earthquakes that have been connected to the surface rupture of such an active fault could be related to high- angle normal faults that have been involved in low-angle thrusting. In this section, we attempt to identify the normal fault reactivation in today's active tectonics via a group of earthquakes with similar properties to that of fault plane solutions and subsurface structural features of coseismic surface ruptures. Earthquakes used in this paper were relocated using a previously published 3D velocity model (Rau & Wu, Reference Rau and Wu1995). The average uncertainties of earthquake locations relocated by the 3D model are estimated to be 3km horizontally and 5km vertically. By comparing with a few recent tomographic studies of Taiwan (e.g. Wu et al. Reference Wu, Chang, Zhao, Shyu, Chen, Sieh and Avouac2007) using more recent data, the main features of the model do not differ much in comparison with those of Rau & Wu (Reference Rau and Wu1995). For the earthquake focal mechanisms with M L ≥ 3.0 which occurred prior to June 2006, we used the focal mechanism database determined by the approach incorporating first motions and SH/P amplitude ratios (Rau, Wu & Shin, Reference Rau, Wu and Shin1996). For the events that occurred between June 2006 and December 2014, we used either the centroid moment tensor (CMT) or the first-motion solutions determined by the Central Weather Bureau for earthquakes with M L > 3.5. For the fault plane solutions of the main shock of the earthquake which occurred early last century, we refer to the studies of Sheu, Kosuga & Sato (Reference Sheu, Kosuga and Sato1982), Cheng (Reference Cheng1995) and Lin et al. (Reference Lin, Chen, Rau and Hu2013).
4.a. Active normal fault reactivation in NW Taiwan
The hypocentre of the main shock of the Hsinchu-Taichung Earthquake which occurred in 1935 has been relocated by Sheu, Kosuga & Sato (Reference Sheu, Kosuga and Sato1982), Cheng (Reference Cheng1995) and Lin et al. (Reference Lin, Chen, Rau and Hu2013) and is very close to the reactivated normal fault beneath low-angle San-I thrust in northwestern Taiwan (Lin, Reference Lin2005). The trend of the Tuntzuchiao fault, a surface rupture of the earthquake, is consistent with the strike of the reactivated normal fault beneath the low-angle thrust (Figs 2, 10d, 11d). A series of earthquake epicentres in that area that are characterized by strike-slip component are mainly located along a belt that is also parallel to the main reactivated normal faults in the footwall of the San-I thrust (Figs 2, 17). The close relationship among the seismicity, historical earthquake and the reactivated normal fault indicate that normal fault reactivation in the footwall of the San-I thrust, although not exposed, remains ongoing.

Figure 17. Distribution of strike-slip fault plane solutions in onshore northwestern Taiwan and a rose diagram showing the main trend of the fault planes in the San-I area. One set of the fault planes is trending ENE–WSW, parallel to that of the Tuntzuchiao fault.
4.b. Active normal fault reactivation in SW Taiwan
The epicentre of the main shock of the Meishan fault which occurred in 1906 has been relocated on the fault in the subsurface of southwestern Taiwan (Fig. 3; Cheng, Reference Cheng1995). The surface rupture is characterized by thrusting its southern side over the northern side, with dextral strike-slip component (Lin et al. Reference Lin, Chang, Lu, Shih and Huang2000). A subsurface structural feature of the Meishan fault can be depicted with interpretation of seismic profiles running through the fault and the frontal thrust (Fig. 3). Interpreted seismic sections across the Meishan fault show a typical positive flower structure for the fault, indicating that the surface rupture is one of the splays in the subsurface (Fig. 18a, b). The positive flower structure implies deformation with contractional component across the fault. However, all the splays, except one or two that are characterized by overthrusting on its southern side and may represent the main active fault on the surface, are normal faults. In map view, the Meishan fault can be extended southwestwards to link a non-reactivated normal fault with downthrow on its northern side (Fig. 3). The high-angle fault feature occurs not only in the front of the fold-and-thrust belt but also in the footwall of the frontal thrust (Fig. 18c). All the subsurface structural features of the Meishan fault indicate that the fault originated from a reactivated normal fault that has been partly thrust by the frontal thrust. In addition, the fault plane solutions for today's earthquakes indicate that those with strike-slip component are highly related to the deformation along the main reactivated normal fault (Fig. 19).

Figure 18. Interpreted seismic sections presented as constraints to depict the subsurface structure and the strike of the Meishan fault and accompanied structural style. (a) Seismic sections VA and (b) V1 show the flower structure of the Meishan fault in the subsurface of the foreland basin, indicating strike-slip component along the fault. (c) Seismic section GB reveals the Meishan fault in the footwall of the Chiuchiungkeng thrust. (d) Seismic section GA is running through a set of N-dipping normal faults and shows rising Neogene strata southwards to the Meishan fault. Similar monoclonal structure also appears in seismic sections V1 and GB. See locations of the seismic sections in Figure 3.

Figure 19. Distribution of strike-slip fault plane solutions in onshore southwestern Taiwan and a rose diagram showing the main trend of the fault planes in the Meishan area (red frame). One set of the fault planes is trending ENE–WSW, parallel to that of the Meishan fault.
4.c. Active normal fault reactivation in the inner fold-and-thrust belt
In central Taiwan the 1999 Chi-Chi Earthquake caused a surface rupture of more than 80km in length, which is almost identical to the exposed Chelungpu thrust. However, a connection between the Chi-Chi Earthquake and the subsurface structure of the Chelungpu thrust is still under debate (Mouthereau, Angelier & Lee, Reference Mouthereau, Angelier and Lee2001; Hung & Suppe, Reference Hung and Suppe2002; Wang et al. Reference Wang, Chien, Su, Leu, Wu, Lai and Chern2002; Yue, Suppe & Hung, Reference Yue, Suppe and Hung2005; Yang et al. Reference Yang, Huang, Wu, Ting, Mei, Lee, Hsu and Lee2007). The fault plane solution for the main shock indicates that the dip angle of the slip surface is around 30° and that the hypocentre is at a depth of 8km below sea level (Fig. 13b). Both dip angle and depth of the main shock are not consistent with those of the décollement of the Chelungpu thrust, but very close to the normal fault beneath the décollement (Fig. 13b; Yang et al. Reference Yang, Huang, Wu, Ting, Mei, Lee, Hsu and Lee2007). This suggests that the basement-involved normal fault plane could still play an important role in triggering disastrous earthquakes. Analysis of the recent (2013) M L 6.2 and M L 6.5 Nantou Earthquakes (Chuang et al. Reference Chuang, Johnson, Wu, Ching and Kuo2013) seems to justify such a viewpoint.
In some cases, the location of the hypocentres of some major earthquakes in the frontal part of the Western Foothills in southwestern Taiwan strongly suggest that normal faults used as high-angle ramps for the major thrusts could have triggered these earthquakes. The situation appears in southwestern Taiwan, where several earthquakes have been located very close to the normal fault plane where the shallow part has been utilized as the ramp of the Tachienshan thrust (Yang, Reference Yang2007; Huang, Reference Huang2009).
5. Discussion
5.a. Development of reactivated basement-involved normal faults
Comparing with that in offshore southwestern Taiwan (Fig. 3), the high-angle faults in offshore northwestern Taiwan (Fig. 2) are longer and continuously extend into the onshore. Linkage of shorter normal faults into longer faults through normal fault reactivation must have played an important role. The inversion structures in northwestern Taiwan show several important characteristics of normal fault reactivation occurring in the frontal part of the fold-and-thrust belt. First, some examples of high-angle normal faults reactivated by low-angle thrusting with opposite dipping direction have been documented in some orogenic belts (Welbon & Butler, Reference Welbon, Butler, Larsen, Brekke, Larsen and Talleraas1992; Butler, Tavarnelli & Grasso, Reference Butler, Tavarnelli and Grasso2006). What we have observed in offshore northwestern Taiwan is the case of a normal fault reactivated by another high-angle reactivated normal fault (Fig. 5a–c). Second, in the situation of oblique inversion such as northwestern Taiwan, reactivation could happen in any segments of the fault instead of always from the hinterlandwards end of the fault. Third, the transfer of inversion displacement between two adjacent faults could happen, very similar to that in the fold-and-thrust belt. It is also noticeable that the apparently ‘curved’ reactivated normal fault (F1 in Fig. 5a) is in fact composed of two segments with different trends. The large-scale symmetrical anticline and the corresponding largest inversion displacement also occur right at the location of the largest fault plane curvature. We propose that the two segments of the reactivated normal faults were originally two softly linked normal faults separated by a relay between the faults, and that normal fault reactivation during contractional tectonics caused the linkage of the separated normal faults and made the relay the place of the largest thrust offset along the high-angle fault (Fig. 5). The reactivated normal faults with large lateral length in the foreland basin (Fig. 2) could therefore have been formed by linkage of separated pre-existing normal faults through normal fault reactivation. Similar evolutionary models have been documented by regional geological studies (Sinclair, Reference Sinclair, Buchanan and Buchanan1995; Carrera & Muñoz, Reference Carrera, Muñoz, Nemčok, Mora and Cosgrove2013) or by physical modelling (Eisenstad & Withjack, Reference Eisenstadt, Withjack, Buchanan and Buchanan1995; Keller & McClay, Reference Keller, McClay, Buchanan and Buchanan1995; Amilibia et al. Reference Amilibia, McClay, Sàbat, Muñoz and Roca2005).
The tectonic map of northwestern Taiwan (Fig. 2) also shows that, in general, non-reactivated normal faults, reactivated normal faults and low-angle thrusts were spatially distributed in an order from west to east. To the east, the reactivated normal faults terminate at the front of boundary thrust between the outer and inner fold-and-thrust belts on the surface. The high-angle reactivated normal fault can be observed in the footwall of the low-angle Peipu-Chutung thrust in the inner fold-and-thrust belt (Yang et al. Reference Yang, Ting, Wu and Chi1997, Reference Yang, Huang, Wu, Ting and Mei2006).
In the outer fold-and-thrust belt, the strike of the subsurface Luchukeng thrust changes right across the Lungkang and the second transverse faults, parallel to that of the Lungkang fault (Fig. 9). Such structural geometry indicates two important characteristics about the interaction between the reactivated normal fault and low-angle thrust. First, during the development of a lateral propagating low-angle thrust, a pre-existing reactivated normal fault causes abrupt changes in both geometry and displacement of the thrust. Second, the Lungkang fault and the second transverse fault can apparently be viewed as tear faults to the Luchukeng thrust; this situation is however not the same as what happens on both sides of the Touhuanping fault to the north, where the Hsincheng and Luchukeng thrust are independent structures (Yang et al. Reference Yang, Ting, Wu and Chi1997). Rather, it is cut through by and runs across the confining high-angle transverse faults in the subsurface. To the south, the high-angle ramp cutting through the base of Chinshui anticline should be regarded as the southwards extensional part of the low-angle Luchukeng thrust. The high-angle transverse faults might extend into the inner fold-and-thrust belt and become tear faults trending ENE–WSW. Such structures appear in some places in the inner fold-and-thrust belt and can be manifested by a series of tear faults trending ENE–WSW in the inner fold-and-thrust belt (Fig. 2).
In onshore southwestern Taiwan, where there is a lack of reactivated normal fault in the foreland basin (Fig. 3), pre-existing normal faults have been involved in the inner fold-and-thrust belt as ramps of the major thrusts (Figs 13, 14). In this part of the Western Foothills, tear faults are mostly striking WNW–ESE to NW–SE, representing the orientation of thrust sheet translation (Fig. 3). Comparison between the tectonic maps of northwestern and southwestern Taiwan suggests that the reactivated normal fault in the foreland basin would influence the orientation of the tear faults in the inner fold-and-thrust belt and determine how the low-angle thrusts are segmented.
5.b. Possible mechanical conditions for normal fault reactivation
The tectonic map (Fig. 2) and seismic sections (Figs 6, 7) of northwestern Taiwan also show that not all the normal faults were reactivated, indicating that normal fault reactivation is a highly selective process; those faults that were reactivated are dipping to the south, the orientation being optimum for the normal faults to be reactivated under the condition of northwestwards vergence of contractional deformation. The Hsinchu fault may be the only exception (see description of the fault in Section 3.b.1). However, this factor is not the only reason for the normal fault to be reactivated; we also observe that many normal faults that are dipping to the south are not reactivated in the offshore and outer fold-and-thrust belt (Figs 5, 7). Sibson (Reference Sibson1985, Reference Sibson, Buchanan and Buchanan1995) predicts that, for a certain internal friction coefficient, the range of dip angles for the normal fault to be reactivated is limited. It is around <50°, and any normal faults with a larger dip angle would not be reactivated under any conditions unless the internal friction coefficient of rock or fault zone decreases. If the strike of the normal fault is not perpendicular to that of the maximum horizontal stress, such as the situation in northwestern Taiwan, diagrams of variations in internal friction coefficient and relative angle between normal fault plane and stress axes (as proposed by Sassi et al. Reference Sassi, Colletta, Bale and Paquereau1993) can be used to predict structural characters of the reactivated normal fault. The relative angle between normal fault plane, with 60° dip in average, and maximum horizontal stress in northwestern Taiwan can be plotted in such diagrams; the results indicate that only those normal faults with an internal friction angle of <20° of fault plane could be reactivated with dextral strike-slip component.
5.c. Definition of deformation front of orogenic belt
As reactivated normal faults are quite common in northwestern Taiwan, the deformation front in this part of orogenic belt cannot be clearly classified as any of the types proposed by Morley (Reference Morley1986). Mouthereau et al. (Reference Mouthereau, Deffontaines, Lacombe, Angelier, Byrne and Liu2002) proposed a reactivation front that marks the boundary of appearance of the reactivated normal faults in an offshore area and gave another definition for the deformation front based on mechanics viewpoint. They drew a highly curved reactivation front in the western margin of the Taishih Basin. From a long-term deformation point of view, we would maintain the definition of deformation front sensu stricto to describe the boundary of the orogenic belt. We suggest that, because the reactivated normal faults have been involved in the outer fold-and-thrust belt, the deformation front in onshore northwestern Taiwan is a wide zone rather than a boundary that can be defined by any specific major thrusts. As for the reactivated normal faults in the offshore area, their formation can be attributed to far-field stress transmitted into the foreland basin.
In southwestern Taiwan, the deformation front can be marked along the western limb of the fold structures that are located right next to the frontal thrusts of the fold-and-thrust belt. These fold structures also cover the exposed active faults on the surface (Fig. 3). The difference in the characteristics of deformation fronts in the two foreland areas is basically due to the contrast in structural style of normal fault reactivation.
5.d. Contrast in structural style of normal fault reactivation
The tectonic maps (Figs 1–3) show significant difference in normal fault reactivation in the offshore and coastal plain of southwestern Taiwan in that none of the normal faults (except the Meishan active fault) has been reactivated, in contrast to that in northwestern Taiwan. Seismic sections located on the front of the fold-and-thrust belt in southwestern Taiwan show that all the high-angle faults still display normal offsets and that the foreland basin strata thicken and tilt up towards the frontal thrust (Fig. 18b–d). This monocline structure has been ascribed to a buried thrust with forelandwards vergence underneath the Neogene strata (Yang, Reference Yang2007), which cannot be observed in the seismic sections and is not likely to create the structure with hinterlandwards vergence. The structural feature can be viewed as an inverted structure and its trend is parallel to that of the fold-and-thrust belt or the overprinted Palaeogene normal faults, not with that of the pre-existing Neogene normal faults. We speculate that it could be caused by reactivation of another set of normal faults in the deeper part of the basement, which cannot be identified in the seismic sections, or simply by ‘basement folding’ (Bellahsen et al. Reference Bellahsen, Jolivet, Lacombe, Bellanger, Boutoux, Garcia, Mouthereau, Le Pourhiet and Gumiaux2012). However, this might be the only clue indicating that the Palaeogene extensional tectonic settings are involved in the foreland tectonics.
The contrast in foreland tectonics in different segments of the fold-and-thrust belt also provides some important clues to test tectonic models relating orogenic modes (thin-skinned v. thick-skinned models) with the crustal/lithospheric strength (as reflected by the equivalent elastic thickness). Mouthereau & Petit (Reference Mouthereau and Petit2003) proposed that, taking the Taiwan orogenic belt as an example, low crustal/lithospheric strength would have favoured thick-skinned deformation. In their model, the lower crustal/lithospheric strength also corresponds to the higher curvature of the reactivation front in offshore southwestern Taiwan and thick-skinned deformation is manifested by a thicker seismogenic zone in the crust, which corresponds to more numerous reactivated normal faults. Alternatively, Butler, Tavarnelli & Grasso (Reference Butler, Tavarnelli and Grasso2006) proposed that it is the relative strength between the pre-existing normal fault and the crust that determines whether the pre-existing normal faults are easily reactivated; in the situation of relative weaker crust, ‘pre-existing faults may have served to create initial perturbation upon which large-scale interfacial buckles nucleated’ and ‘the surrounding basement rocks are deformed’ (Butler, Tavarnelli & Grasso, Reference Butler, Tavarnelli and Grasso2006).
The equivalent elastic thickness, estimated by flexural modelling of the foreland basin in western Taiwan, indicates that the crust/lithosphere is weaker in the southwestern part than that in the northwestern part of Taiwan foreland (Lin & Watts, Reference Lin and Watts2002; Mouthereau & Petit, Reference Mouthereau and Petit2003). In response to the weaker crust/lithosphere of southwestern Taiwan, the basement is deformed while the pre-existing basement-involved normal faults remain non-reactivated; only a segment with limited length along a normal fault is reactivated (Fig. 18b–d). What we observed and described in southwestern Taiwan suggests that the deforming basement rocks in a weaker crust/lithosphere would respond to the tectonic stress in the foreland and prevent the basement-involved normal faults from being reactivated. As for those reactivated normal faults in northwestern Taiwan, mechanical analysis based on the theories of Sibson (Reference Sibson1985, Reference Sibson, Buchanan and Buchanan1995) and Sassi et al. (Reference Sassi, Colletta, Bale and Paquereau1993) described in Section 5.b suggests a lower internal friction coefficient for a pre-existing normal fault plane. We suggest that these normal faults embedded in stronger crust would be easier to be reactivate than those in southwestern Taiwan.
5.e. Implications of normal fault reactivation in active tectonics
The examples of active faults that we present in this paper occur in different parts of the fold-and-thrust belt (Figs 10c, 11c, 13b, 18) and are related to reactivated basement-involved normal faults trending obliquely to the front of the orogen. Although the main shocks of historical earthquakes occurring on these fault planes vary in fault type and orientation (Figs 2, 3) –those in the inner fold-and-thrust belt are characterized by thrust faults trending parallel to the surface major thrusts – the fault plane solutions of the main shocks in the outer fold-and-thrust belt and coastal plain indicate strike-slip faults trending ENE–WSW. In the former situation, the shallow part of the pre-existing normal fault acts passively as a ramp of the low-angle major thrust and the fault plane solutions reflect the long-term orientation of slip along the low-angle thrust. Active faults in the outer fold-and-thrust belt and coastal plain are likely truly reactivated normal faults that actively deform in response to the regional collisional stress. We may therefore classify normal fault reactivation into active and passive types, which play different roles in the deformation of the foreland tectonics in western Taiwan.
6. Conclusions
Basement-involved pre-existing normal faults play important but various roles in the different parts of the Taiwan fold-and-thrust belt and create various structural styles. In offshore northwestern Taiwan, long reactivated normal faults with a strike-slip component have developed by linkage of reactivated single pre-existing normal faults in the foreland basin. The reactivated normal faults extend into the onshore outer fold-and-thrust belt and play the roles of transverse structures in the development of low-angle thrusts. The length of the low-angle thrusts is confined by the transverse structures, which formed a tear fault or lateral ramp for the terminated thrusts. At a later stage, the transverse structures were overthrust and appear underneath the low-angle thrust or became ENE–WSW-trending tear faults in the inner fold-and-thrust belt.
In southwestern Taiwan, where the foreland basin is almost devoid of reactivated normal faults, the pre-existing normal faults also influenced the development of the geometry of low-angle thrust in the outer fold-and-thrust belt; the shallow parts of pre-existing normal faults, acting passively as a ramp for the low-angle thrusts when the major thrusts propagated forelandwards, stepped up along the normal fault plane and cut upwards to the surface. The way the pre-existing normal faults involved in the inner fold-and-thrust belt also results in a WNW–ESE-trending tear fault in the belt, different from that in northwestern Taiwan.
The deformation front sensu stricto in onshore northwestern Taiwan is now around the coastal line and is a wide zone rather than a boundary that can be defined by any specific major thrust. In contrast to that, the deformation front in the foreland basin of onshore southwestern Taiwan can be marked along the western limb of the monoclonal structures that are located right next to the frontal thrusts of the outer fold-and-thrust belt.
Some of the faults active today in western Taiwan may be related to the reactivated normal faults which, with right-lateral slip component, occur in the frontal area of the fold-and-thrust belt or even beneath the low-angle thrusts. These active faults have similar structural trends as those formed during the regional extensional tectonics. Some of the earthquake main shocks, with hypocentres in the footwall of the low-angle thrust, reveal thrust types of fault plane solutions. The hypocentres of these major earthquakes can however be located on the pre-existing normal fault planes. The fact that these normal faults act as a high-angle ramp for the major thrusts implies a relationship between the existence of the normal fault and the triggering of the recent major earthquakes.
The lack of a reactivated normal fault in southwestern Taiwan, where the basement has been regarded weaker than that in northwestern Taiwan, may suggest that a weaker crust would respond to the tectonic stress by taking most of the deformation in the foreland and prevent the basement-involved normal faults from being reactivated. In contrast, normal faults embedded in stronger crust as in northwestern Taiwan would be easier to reactivate than those in southwestern Taiwan.
Acknowledgements
The authors would like to thank Exploration and Development Research Institute, CPC Corporation, Taiwan for providing invaluable well-bore and seismic data and their long-term support of this study. The authors would also like to thank Dr Chih-Wei Chien, Ms E.-Wen Cheng, Mr Tzu-Ruei Yang and Mr Chia-Hsun Yang from the Department of Earth Sciences, National Cheng Kung University for preparing the figures and editing the text. Special thanks are extended to Drs Brown and Lacombe for their invaluable comments and suggestions. The research results reported here are part of the projects MOST 103-3113-E-006-012 and MOST 105-2119-M-865-002, supported by the Ministry of Science and Technology of Taiwan Government, and FED0111001, supported by Exploration and Development Research Institute, CPC Corporation, Taiwan.