1. Introduction
Mesozoic sediments in East Greenland have been studied for almost 150 years. A detailed historical review of the expeditions in the area between 1870 and 1956 was given by Donovan (Reference Donovan1957) and updated by Surlyk (Reference Surlyk1978a). Cretaceous biostratigraphy and the dating of the Cretaceous successions in East and NE Greenland (Fig. 1) were sparse until the 1990s, especially compared with the studies of the Jurassic strata in the same area. During the historic exploration of East and NE Greenland (e.g. Surlyk, Reference Surlyk1978a), Cretaceous biostratigraphy of the sedimentary basins remained fragmentary and variably developed, partly because the majority of the succession is badly exposed and commonly severely intruded and covered by Palaeogene basalts providing poor recovery of fossils from the sedimentary successions. The most comprehensive biostratigraphy has been recorded in lowermost Cretaceous strata with relatively good exposures, for example in the Wollaston Forland – Kuhn Ø region (e.g. Surlyk, Reference Surlyk1978a; ‘Ø’ is Danish for island). The ammonite succession was documented by Maync (Reference Maync1949) and Donovan (Reference Donovan1964); Surlyk (Reference Surlyk1978a) provided a middle Volgian (c. middle Tithonian) – Lower Cretaceous ammonite zonation and an Upper Jurassic – Lower Cretaceous Buchia zonation was introduced by Surlyk & Zakharov (Reference Surlyk and Zakharov1982). The correlation to the Tithonian–Berriasian stages is based on Wimbledon (Reference Wimbledon2017). In contrast, mid-Cretaceous biostratigraphic data were sparse and Upper Cretaceous data were almost missing, despite the large efforts invested in fieldwork over many years (e.g. Ravn, Reference Ravn1911; Koch, Reference Koch1929a, b, Reference Koch and Krenkel1935; Frebold, Reference Frebold1932a, b, Reference Frebold1934, Reference Frebold1935; Frebold & Noe-Nyegaard, Reference Frebold and Noe-Nyegaard1938; Maync, Reference Maync1949; Donovan, Reference Donovan1949, Reference Donovan1953, Reference Donovan1954, Reference Donovan1955, Reference Donovan1957, Reference Donovan and Raasch1961, Reference Donovan1964, Reference Donovan, Callomon, Donovan and Trümpy1972a, b).
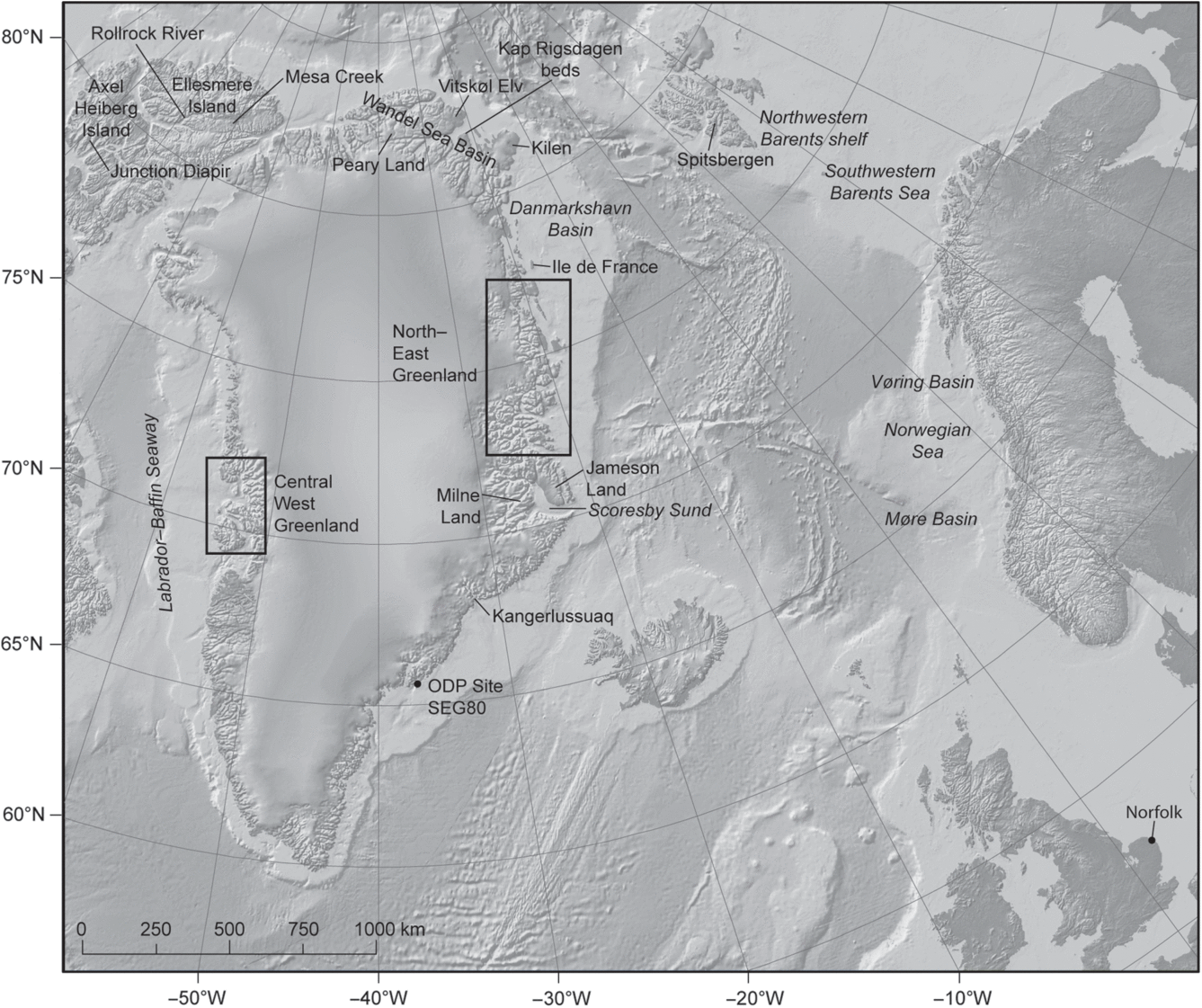
Fig. 1. Location map of Greenland and surrounding areas.
Nøhr-Hansen (Reference Nøhr-Hansen1993, Reference Nøhr-Hansen1994) introduced dinoflagelIate cysts (dinocysts) as a stratigraphic tool in the Cretaceous successions of East and NE Greenland. Based on a comprehensive study of the Lower Cretaceous dinocyst flora, a detailed zonation of the upper Hauterivian? – Lower Cenomanian? was established. Thirty-seven sections and three shallow cores without macrofossils were dated on the basis of dinocysts throughout NE Greenland.
In more recent times, Alsen (Reference Alsen2006) monographed an upper Ryazanian (c. upper Berriasian) – Hauterivian ammonite fauna in the Wollaston Forland region and later applied Upper Jurassic – Lower Cretaceous ammonite stratigraphy on the Rødryggen-1 core (Bojesen-Koefoed et al. Reference Bojesen-Koefoed, Alsen and Christiansen2014). Alsen & Mutterlose (Reference Alsen and Mutterlose2009) demonstrated the stratigraphic potential of Lower Cretaceous belemnites in this region. Recently, dinocysts (Nøhr-Hansen et al. Reference Nøhr-Hansen, Costa, Pearce and Alsen2018), foraminifers and nannofossils have been applied for stratigraphy on restricted intervals where calcareous tests are preserved (Pauly et al. Reference Pauly, Mutterlose and Alsen2012a, b; Rødryggen-1 core, Bojesen-Koefoed et al. Reference Bojesen-Koefoed, Alsen and Christiansen2014), Brorson Halvø-1 core (Bojesen-Koefoed et al. Reference Bojesen-Koefoed, Alsen and Christiansen2014), Store Koldewey study (Piasecki et al. Reference Piasecki, Alsen, Anthonsen, Bjerager, Bojesen-Koefoed, Fyhn, Japsen, Hovikoski, Kjøller, Morigi, Nielsen, Nøhr-Hansen, Sheldon, Weibel and Kazerouni2012) and the Nanok-1 core (Bojesen-Koefoed et al. Reference Bojesen-Koefoed, Alsen and Christiansen2014).
During the petroleum geological studies in East and NE Greenland (2008–2011), Cretaceous biostratigraphic data have been collected from the whole region between Traill Ø (72° N) in the south to Store Koldewey (latitude, 76–76.5° N) in the north (Figs 2–5). It incorporates previous work in the area but has been centred around a resent intensive programme of fieldwork, including drilling of cored wells (Bojesen-Koefoed et al. Reference Bojesen-Koefoed, Alsen and Christiansen2014). In order to develop a better understanding of the onshore geology, the goal was to identify basin evolutionary trends and describe depositional systems that would be valuable in interpreting existing and future datasets offshore NE Greenland, particularly in the Danmarkshavn Basin (Fig. 1) at the northern limit of the onshore project. Palynomorphs, primarily dinocyst, recorded from more than 100 sections throughout the region, dated the sequence as late Tithonian – late Campanian or ?Early Maastrichtian. The dinocyst zonation of Nøhr-Hansen (Reference Nøhr-Hansen1993) is expanded stratigraphically to now include the upper Tithonian – Valanginian strata in addition to the Cenomanian – Campanian or ?Lower Maastrichtian strata. Extensive collection of macrofossils has improved the calibration of dinocyst zonation with ammonite stratigraphy and higher precision has been achieved. The improved biostratigraphy has been used extensively to date the units of a revised and updated Cretaceous lithostratigraphy. This comprehensive revision is nearing completion (Geological Survey of Denmark and Greenland) but is presently unpublished; the lithostratigraphic framework utilized here (Fig. 6) is therefore provisional and hence informal. The informal units are in lower case in the text and in Figure 6.
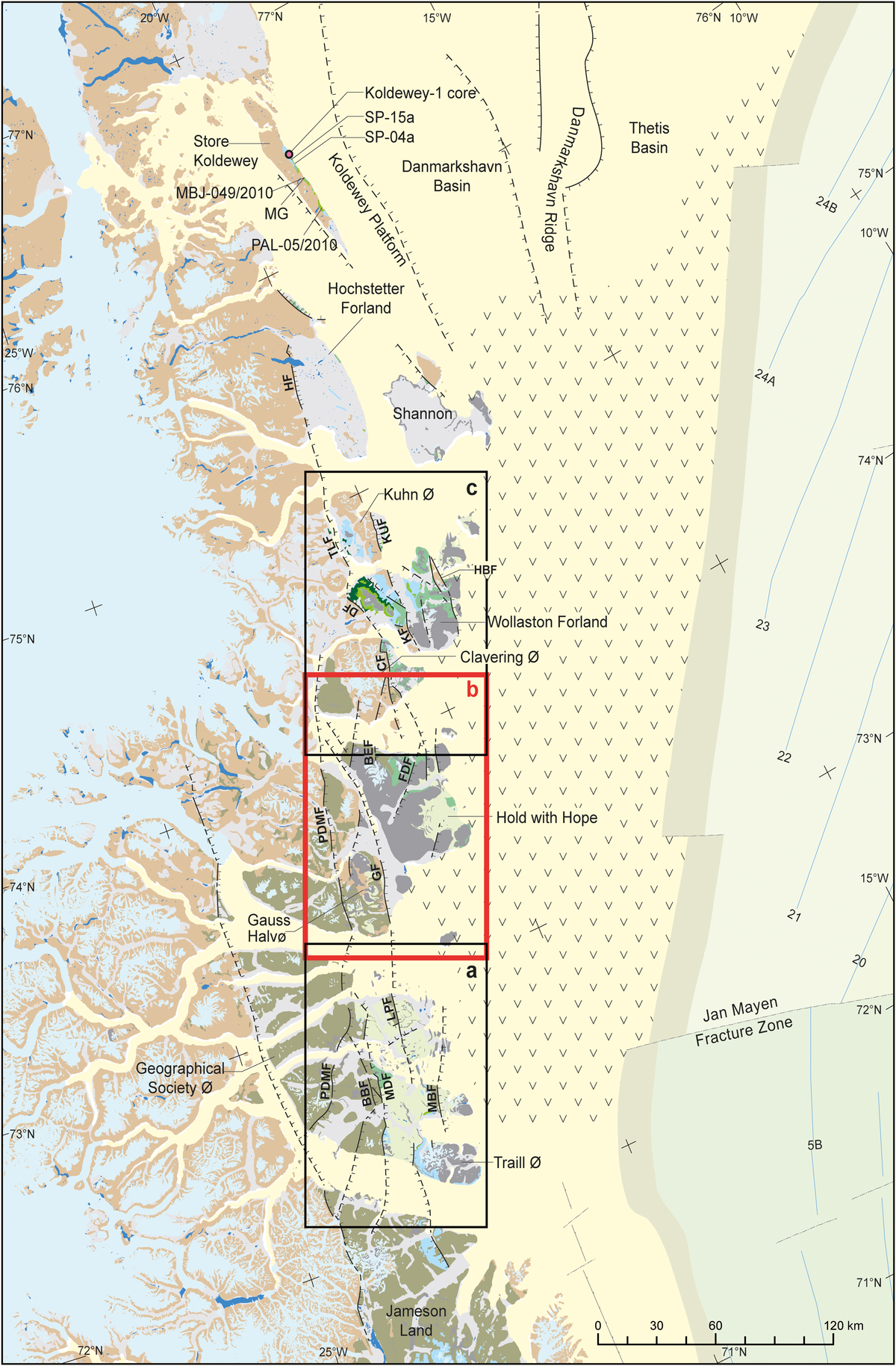
Fig. 2. Geological map of NE Greenland showing study area stretching from Traill Ø to Store Koldewey. Insert maps: a, Traill Ø and Geographical Society Ø (Fig. 3); b, Gauss Halvø and Hold with Hope (Fig. 4); and c, Clavering Ø and Wollaston Forland (Fig. 5). BBF – Bordbjerg Fault; BEF – Blå Elv Fault; CF – Clavering Fault; DF – Dombjerg Fault; GF – Gisecki Fault; HBF – Hühnerbjerg Fault; HF – Hochstetter Fault; FDF – Fosdalen Fault; KUF – Kuhn Fault; LPF – Laplace Bjerg Fault; MDF – Månedal Fault; MG – Midter Gneisnæs; MBF – Mols Bjerge Fault; PDMF – Post-Devonian Main Fault; TLF – Thomsen Land Fault.
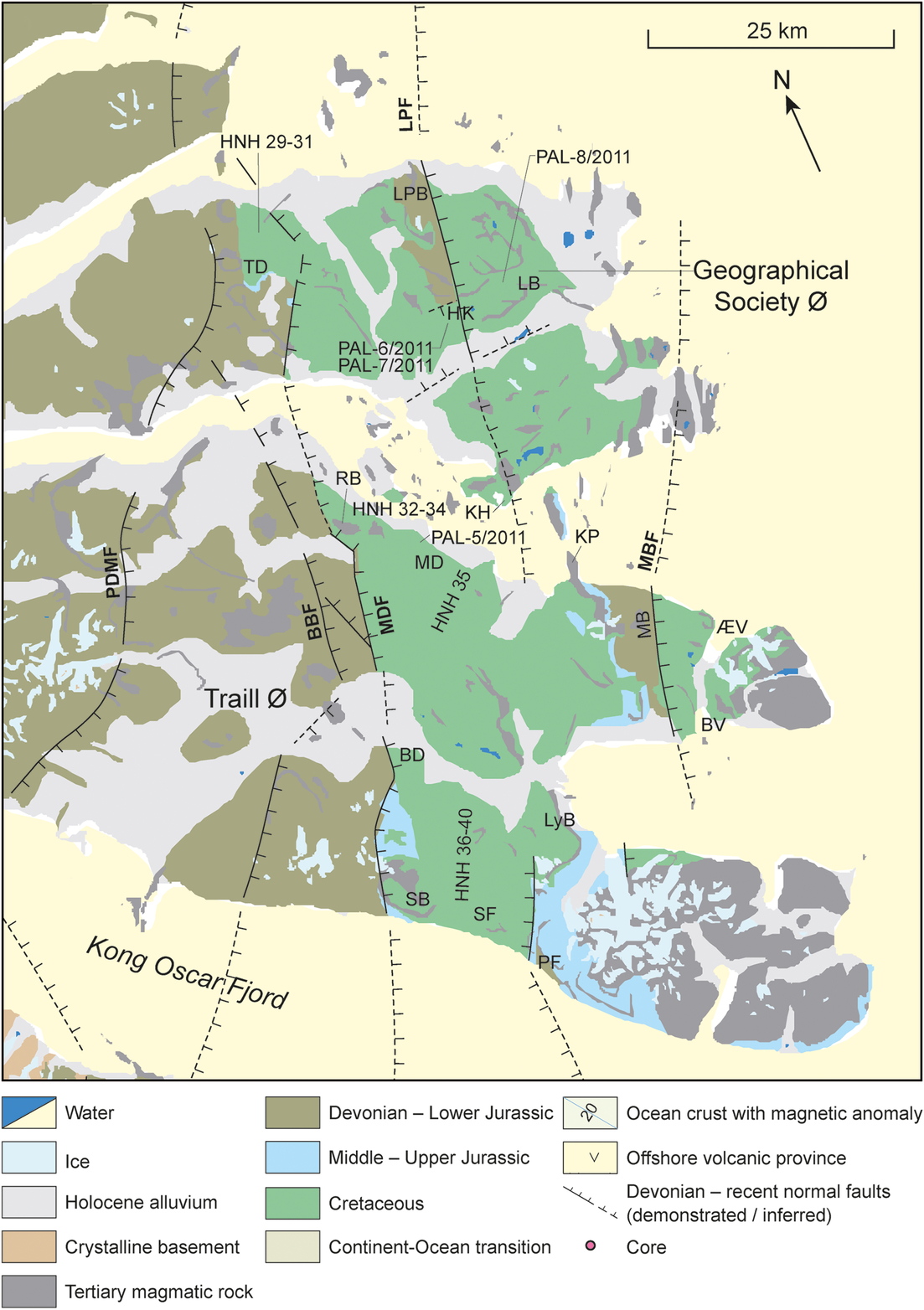
Fig. 3. Geological map of the Traill Ø and Geographical Society Ø. Faults: BBF – Bordbjerg Fault; LPF – Laplace Bjerg Fault; MDF – Månedal Fault; MBF – Mols Bjerge Fault; PDMF – Post-Devonian Main Fault. Localities: BD – Bjørnedal; BV – Beitrup Vig; HNH 29-40 (localities of Nøhr-Hansen; 1993); LB – Leitch Bjerg; LPB – Laplace Bjerg; LyB – Lycett Bjerg; HK – Hundeklemmen; KH – Kap Hovgaard; KP – Kap Pallander; MB – Mols Bjerge; PF – Prospekt Fjeld; RB – Rold Bjerge; SB – Svinhuvuds Bjerge; SF – Sorte Fjelde; TD – Tværdal; ÆV – Æbletoft Vig.
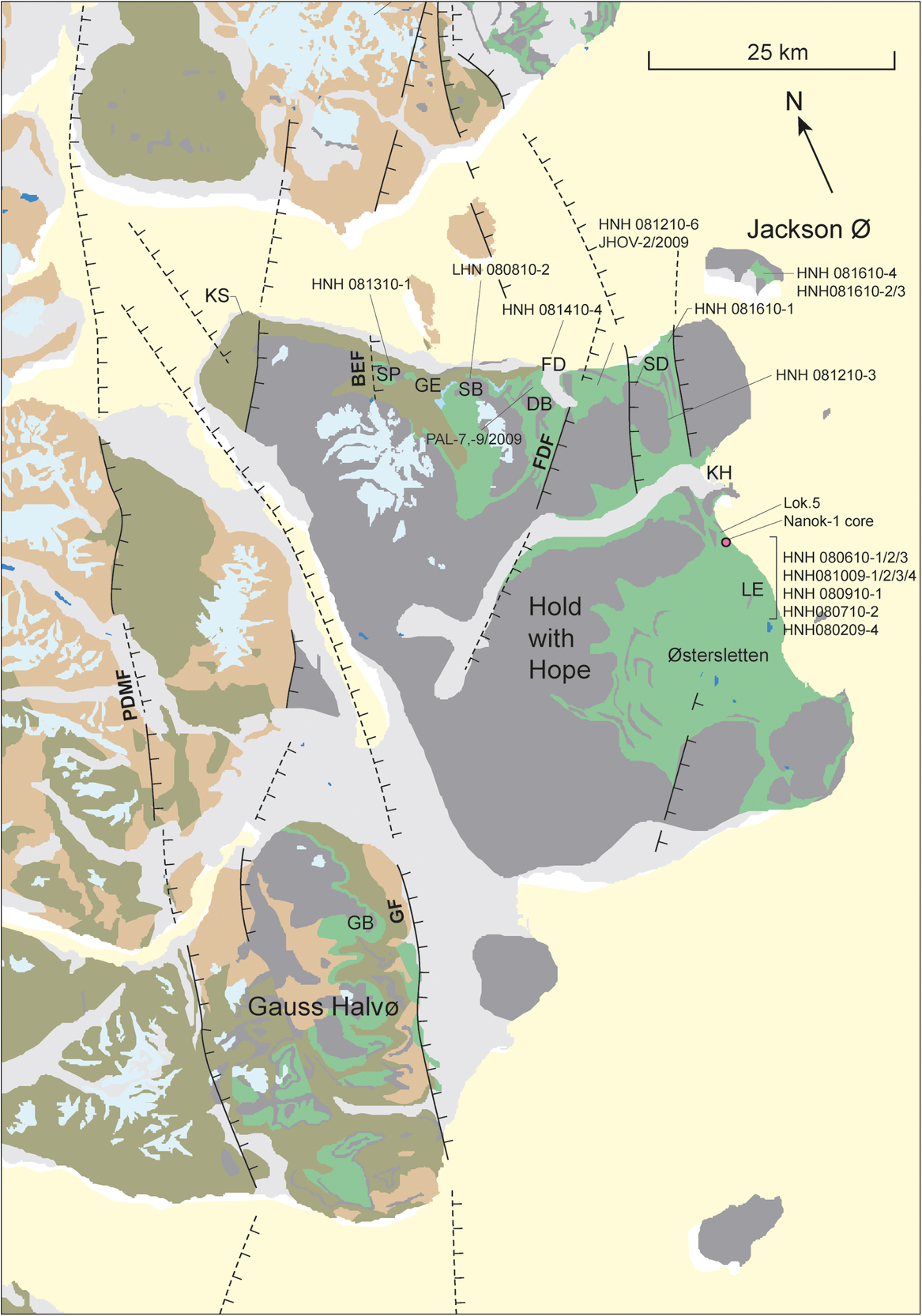
Fig. 4. Geological map of Hold with Hope and Gauss Halvø. Faults: BEF – Blå Elv Fault; GF – Gisecke Fault; FDF – Fosdalen Fault; PDMF – Post-Devonian Main Fault. Localities: DB – Diener Bjerg; FD – Fosdalen; GB – Gisecke Bjerge; GE – Gulelv; KH – Knudshoved; LE – Lygnaelv; KS – Kap Stosch; SB – Steensby Bjerg; SD – Snerta Dal; SP – Stensiö Plateau.
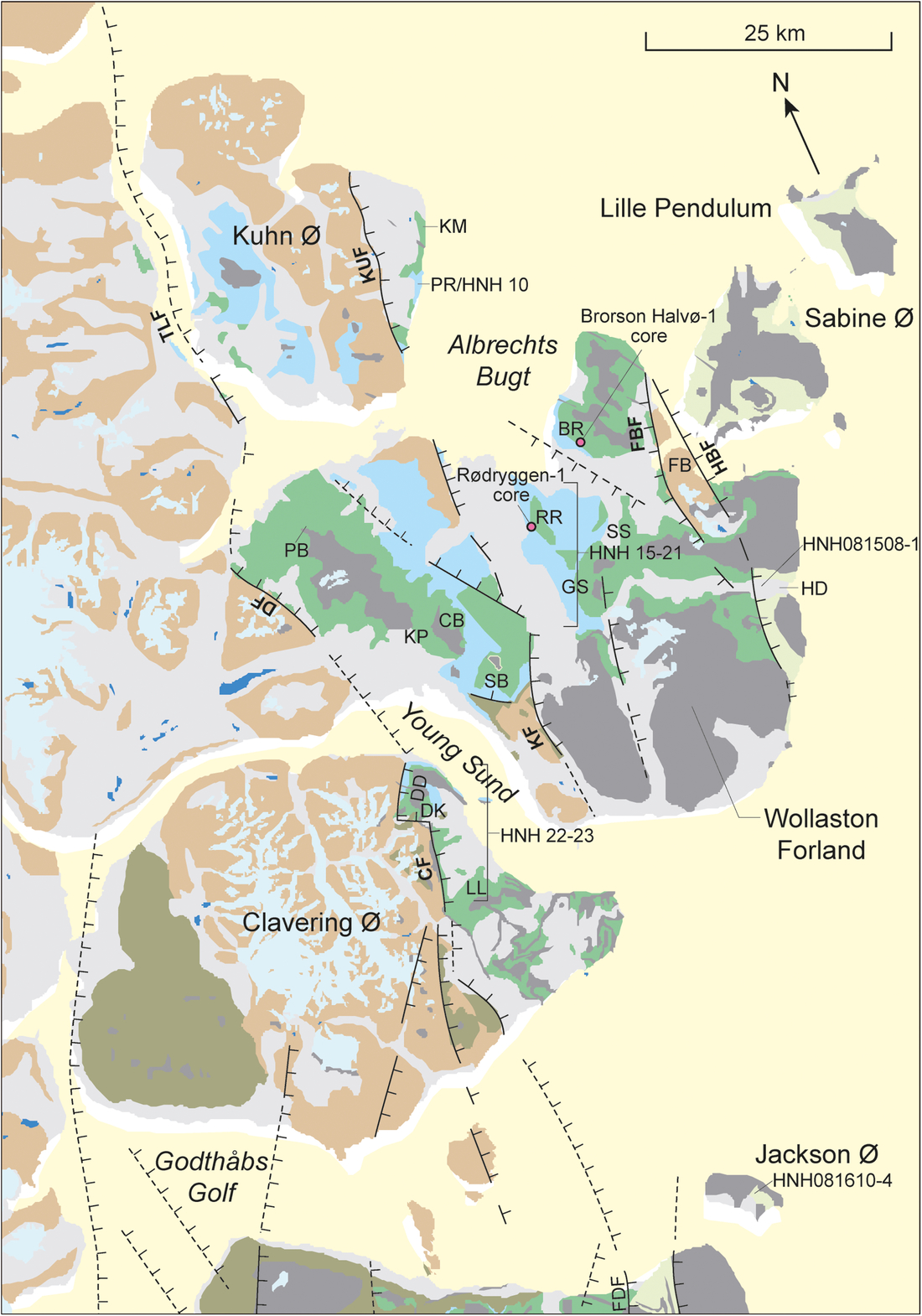
Fig. 5. Geological map of the Clavering Ø to Kuhn Ø area. Faults: CF – Clavering Fault; DF – Dombjerg Fault; FBF – Falkebjerg Fault; HBF – Hühnerbjerg Fault; KF – Kuppel Fault; KUF – Kuhn Fault; TLF – Thomsen Land Fault. Localities: BR – Brorson Halvø; CB – Cardioceras Bjerg; DD – Dolomit Dal; DK – Djævlekløften; FB – Falkebjerg; GS – Gyldenspids; HD – Haredal; HNH 15–23 (localities of Nøhr-Hansen, 1993); KM – Kap Mauer; KP – Kuhn Passet; LL – Langelinie PB – Palnatokes Bjerg; PR – Perisphinctes Ravine; RR – Rødryggen; SB – Stratumbjerg; SS – Stor Sletten.

Fig. 6. Regional lithostratigraphic correlation scheme. The lithostratigraphic framework utilized here for NE Greenland is provisional and hence informal, being based on a comprehensive revision nearing completion (Geological Survey of Denmark and Greenland, unpublished data). Informal units in lower case.
North of the studied area, from Germania Land north of Store Koldewey, macrofossils from loose boulders of Aptian age have been reported by Ravn (Reference Ravn1911) and Rosenkrantz (Reference Bøgvad and Rosenkrantz1934, p. 24), but no outcrops have been recorded. Reworked dinocysts of early Aptian and late Albian ages have been recorded as reworked in Pliocene deposits at Ile de France (Fig. 1; unpublished data). Ammonites of late Volgian (c. late Tithonian) – early Valanginian and Early–middle Albian ages have been reported by Birkelund & Håkansson (Reference Birkelund and Håkansson1983) and Alsen (Reference Alsen2018) from the Wandel Sea Basin in North Greenland (Fig. 1). Dinocysts from the same area indicate ages of middle Oxfordian – early Valanginian (Håkansson et al. Reference Håkansson, Heinberg and Stemmerik1981). Dinocysts of Early Albian age have also been recorded from the area by Århus (Reference Århus1991). Recently, Piasecki et al. (Reference Piasecki, Nøhr-Hansen and Dalhoff2018) described in situ Barremian and reworked Upper Cretaceous dinocyst assemblages from the Wandel Sea Basin (81° N), and Pedersen et al. (Reference Pedersen, Lauridsen, Svennevig, Bojesen-Koefoed, Nøhr-Hansen and Alsen2018) described middle Albian – late Coniacian dinocysts, inoceramid and ammonite assemblages from Kilen (Fig. 1; 81° N). From southern Jameson Land (Fig. 1), south of the studied area, the youngest Lower Cretaceous formation described is the Berriasian – lower Valanginian Hesteelv Formation (Surlyk et al. Reference Surlyk, Callomon, Bromley and Birkelund1973; Fig. 1). According to C. Marcussen (pers. comm. 1991), seismic data from Scoresby Sund (Fig. 1), south of Jameson Land, indicate that the Jameson Land basin continues to the south beneath the lower Cenozoic basalts on the southern shore of the fjord. The Lower Cretaceous successions possibly attain a total thickness here of up to 500 m. On Milne Land (Fig. 1; 70° N), dinocysts indicating middle Volgian (c. middle Tithonian) – late Ryazanian (c. late Berriasian – early Valanginian) ages have been reported by Piasecki (Reference Piasecki1979). Cretaceous dinocysts of Albian–Maastrichtian ages have been recorded by Nøhr-Hansen (Reference Nøhr-Hansen2012) further south in the Kangerdlugssuaq area (Fig. 1; 68° N), and dinocysts of Albian age have been reported from reworked sandstones obtained offshore Tasiilaq at Ocean Drilling Program (ODP) Site SEG80 during the transect EG65 (Fig. 1; Thy et al. Reference Thy, Lesher, Larsen, Thy, Lesher and Larsen2007).
2. Geological setting
The NE Greenland margin and the conjugate margin on the Norwegian continental shelf were formed by periodic rifting since the late Palaeozoic Era (Surlyk, Reference Surlyk, Hardman and Brooks1990). Extensional collapse and basin formation were initiated during the Devonian Period, following the culmination of the Caledonian Orogeny in Silurian and Devonian times as Laurentia (represented by Greenland and North America) collided with Baltica and Avalonia (Higgins & Leslie, Reference Higgins, Leslie, Higgins, Gilotti and Smith2008). A number of large, mostly N–S- to NNE–SSW-trending, normal faults confine the post-Caledonian sedimentary basins in the area, broadly mimicking the Caledonian structural grain of the region (Fig. 2). Devonian and Carboniferous rifting resulted in the formation of deep basins filled by more than 8 km of non-marine deposits (Larsen et al. Reference Larsen, Olsen, Clack, Higgins, Gilotti and Smith2008). Subsequent rift episodes affected the area during Permian, Early–middle Triassic and Middle Jurassic – earliest Cretaceous times (Surlyk et al. Reference Surlyk, Clemmesen, Larsen, Kerr and Fergusson1981; Surlyk, Reference Surlyk, Hardman and Brooks1990; Stemmerik et al. Reference Stemmerik, Christiansen, Piasecki, Jordt, Marcussen, Nøhr-Hansen, Vorren, Bergsager and Dahl-Stamnes1993). Further North Atlantic extension during Late Cretaceous and Early Palaeogene times ultimately led to continental separation during the Early Eocene Epoch and Atlantic seafloor spreading to present. The pre-Eocene extensional basins of NE Greenland, onshore and offshore, are thus complemented by the offshore record of the conjugate Norwegian margin. This is particularly relevant to the Cretaceous period, when the NE Greenland margin represented the proximal portion of a symmetrical marine trough. The axial deep-water part of the rift basin is represented by the Vøring and Møre basins and the terraced Norwegian margin, mirroring that of NE Greenland (Fig. 1).
The North Atlantic region experienced two major rift episodes during late Mesozoic – early Cenozoic times. Rifting related to widespread broadly E–W-aligned extension in the North Atlantic region, accompanying Central Atlantic spreading, began during late Bajocian times in NE Greenland (Doré et al. Reference Doré, Lundin, Jensen, Birkeland, Eliassen, Fichler, Fleet and Boldy1999). Middle–Late Jurassic extension in East Greenland resulted in broad, fault-controlled depocentres, propagating northwards from Jameson Land with time (Surlyk, Reference Surlyk2003); this phase of regional basin subsidence and expansion was accompanied by a eustatic sea-level rise and resulted in the accumulation of the world-renowned Kimmeridgian source rocks from the North Sea through the Greenland–Norway rift to the Barents Sea (Bojesen-Koefoed et al. Reference Bojesen-Koefoed, Bjerager, Nytoft, Petersen, Piasecki and Pilgaard2018). During latest Jurassic – earliest Cretaceous times, extension culminated in the development of a rugged rift topography due to marked rotation of narrow fault blocks (10–25 km across) along broadly N–S-aligned faults (Vischer, Reference Vischer1943; Surlyk, Reference Surlyk1978a, Reference Surlyk2003). This event and the resulting unconformity is an important feature throughout the North Atlantic rift and a key seismic marker on the Norwegian shelf (e.g. Tsikalas et al. Reference Tsikalas, Faleide, Eldholm, Wilson, Doré and Vining2005) and in the Danmarkshavn Basin (Hamann et al. Reference Hamann, Whittaker, Stemmerik, Doré and Vining2005; Fig. 1). The second phase of rifting during Late Cretaceous – Early Palaeogene times heralded the eventual break-up and onset of sea-floor spreading at the Paleocene–Eocene boundary. The onset of rifting in the Campanian is typically linked to the initiation of sea-floor spreading in the Labrador Sea (Fig. 1), and is associated with a shift in the extension direction in the North Atlantic region from N–S to NE–SW (Doré et al. Reference Doré, Lundin, Jensen, Birkeland, Eliassen, Fichler, Fleet and Boldy1999). In NE Greenland, this rift phase is poorly documented in the sedimentary record due to uplift and exhumation linked to the rift event. Much of the Cretaceous sedimentary record of onshore NE Greenland, from the Hauterivian–Barremian to the lowermost Campanian – ?Lower Maastrichtian strata, thus occupies the timespan between these two regional North Atlantic rift phases and can be broadly considered as ‘post-rift’, deposited during a time of relative tectonic quiescence (Færseth & Lien, Reference Færseth and Lien2002; Lien, Reference Lien2005).
3. Material and methods
The studied material includes samples from the Nanok-1 (517004, Fig. 4), Rødryggen-1 (517001, Fig. 5) and Store Koldewey-1 (517002, Fig. 2) cores and numerous outcrop sections, as well as a restudy of several of the old samples originally described by Nøhr-Hansen (Reference Nøhr-Hansen1993). The palynological zonation established below is based on the study of more than 1000 samples representing more than 100 sections throughout the area (Figs 2–5). The new established Upper Cretaceous zones follow the roman numerals system established for the Lower Cretaceous zones by Nøhr-Hansen (Reference Nøhr-Hansen1993), whereas the two lowermost Cretaceous zones are named NEG Cr 1 and NEG Cr 2 (NE Greenland Cretaceous 1 and 2). The Heterosphaeridium difficile, Aquilapollenites and Cerodinium diebelii intervals identified in West Greenland were originally not intended to be formal biostratigraphic zones (Nøhr-Hansen, Reference Nøhr-Hansen1996); they therefore do not preoccupy the names for the new Heterosphaeridium difficile (IX), Aquilapollenites (XII) or Cerodinium diebelii (XIII) zones. The majority of the sections are named with the initial of the collector (all Geological Survey of Denmark and Greenland (GEUS) employees), often followed by section number, for example SP-15a (Stefan Piasecki section 15a, Fig. 2); with initial, section number and year, for example MBJ-049/2010 (Morten Bjerager, section 49, 2010, Fig. 2), JHOV -2/2009 (Jussi Hovikoski section 2, 2009, Fig. 4) and PAL-8/2011 (Peter Alsen, section 8, 2011, Fig. 4); or with initial, month, date, year of collection and section number, for example HNH 081610-4 (Henrik Nøhr-Hansen 16th of August 2010 section 4, Fig. 4) or LHN 080810-1 (Lars Henrik Nielsen 8th of August 2010 section 1, Fig. 4). The sections named HNH followed by a number (e.g. HNH 29–31; Fig. 3) refers to the sections described by Nøhr-Hansen (Reference Nøhr-Hansen1993).
The palynology samples were processed by a methodology including treatment with hydrogen chloride (HCl), hydrogen fluoride (HF), oxidation with nitric acid (HNO3) and heavy liquid separation. At each preparation step, a slide was inspected to follow the process closely. Finally, the organic residue was sieved on a 21 μm, occasionally on 30 μm, filter (to concentrate palynomorphs in samples, poor in dinocysts), swirled and was finally mounted on glass slides using a glycerine jelly medium. The dinocyst content was analysed using a normal light microscope.
Approximately 100 identifiable dinocysts per sample were counted to perform a relative abundance analysis. Prasinophycean and freshwater algae and acritarchs in the slide were also counted in addition to the main dinocyst tally. Additional dinocyst species occurring outside the 100 counted specimens in the first slide or in other slides were recorded as present. The taxonomy used here follows that of Williams et al. (Reference Williams, Fensome and MacRae2017).
The stratigraphic results are illustrated in a stratigraphic event chart (Fig. 7a–d; timescale (Ma) from Ogg et al. Reference Ogg, Ogg and Gradstein2016). Since all analysed material is from cores or outcrops, caving is not an issue. Reworked dinocyst taxa are recorded. The spore and pollen flora is not recorded systematically, but reworked and selected stratigraphic marker mio- and macrospores are recorded.




Fig. 7. (a–d) Palynostratigraphic zonation and event stratigraphy (primarily based on dinocysts) for the upper Tithonian – Lower Maastrichtian stages in NE Greenland based on the study of three cores and more than 100 sections, of which 29 are selected as reference sections representing the studied Cretaceous succession. Timescale (Ma) from Ogg et al. (Reference Ogg, Ogg and Gradstein2016).
4. Stratigraphic methods
Based on the oldest or first occurrence (FO) and youngest or last occurrence (LO) of stratigraphically important dinocyst species (events), the studied succession has been calibrated to ammonite zones of the local Boreal ammonite zonation (Surlyk, Reference Surlyk, Casey and Rawson1973, Reference Surlyk1977, 1978a, b; Callomon & Birkelund, Reference Callomon, Birkelund, Embry and Balkwill1982; Birkelund et al. Reference Birkelund, Callomon and Fürsich1984; Birkelund & Callomon, Reference Birkelund and Callomon1985; Kelly & Whitham, Reference Kelly and Whitham1999; Alsen, Reference Alsen2006). Adjusted or new calibrations of dinocyst events calibrated by the local ammonite stratigraphy have been established in this work. The ammonite biozonation correlates with ammonite chronozones and the succession is dated on the basis of these chronostratigraphic units. Ammonite zonations vary with geographically separate faunal provinces and these diverging zonations are not precisely correlated at many stratigraphic levels; however, stratigraphic schemes generally ignore this fact. Calibrating dinocyst events with ammonite zones in different faunal provinces, and then comparing these ammonite zones across biogeographic boundaries to obtain a relevant age, is hazardous; however, this is the only possibility in some cases. The lowermost Cretaceous flora varies significantly in composition, diversity and abundance from sub-Boreal to Boreal and Arctic regions (e.g. Brideaux & Fisher, Reference Brideaux and Fisher1976; Brideaux, Reference Brideaux1977; Lebedeva & Nikitenko, Reference Lebedeva and Nikitenko1999; Riding et al. Reference Riding, Fedorova and Ilyina1999; Smelror & Dypvik, Reference Smelror and Dypvik2005); consequently, the age of stratigraphic events may also vary. Examples are documented in this work based on the common ammonite and dinocyst stratigraphy presented here.
5. Ammonite zonation
Since the biostratigraphic review of Donovan (Reference Donovan1957), the most important contribution to Cretaceous ammonite stratigraphy in NE Greenland has been on the Jurassic–Cretaceous boundary beds in Wollaston Forland and Kuhn Ø (Donovan, Reference Donovan1964; Surlyk, Reference Surlyk, Casey and Rawson1973, Reference Surlyk1978a), the lower Aptian beds from Hold with Hope (Kelly & Whitham, Reference Kelly and Whitham1999) and the upper Berriasian – Hauterivian strata in Wollaston Forland (Alsen & Rawson, Reference Alsen and Rawson2005; Alsen, Reference Alsen2006). Recent sampling has added data from levels and intervals previously not known to contain ammonites. The Berriasian (c. uppermost Volgian) –Hauterivian zones are mainly documented from the Lindemans Bugt Formation and Palnatokes Bjerg Formation in the Wollaston Forland area (Surlyk, Reference Surlyk1978a; Alsen, Reference Alsen2006; Fig. 6). The Hauterivian zone was only referred to as Simbirskites beds by Alsen (Reference Alsen2006) based on the first but poorly preserved finds of Simbirskites, but further collection now allow the identification of the Simbirskites (Speetoniceras) inversum Zone and the Simbirskites decheni Zone (Fig. 8). The former documents that the deposition of the Rødryggen Member of the Palnatokes Bjerg Formation extended into the upper Hauterivian Stage is also reflected by the recently established belemnite and calcareous nannofossil stratigraphies (Alsen & Mutterlose, Reference Alsen and Mutterlose2009; Pauly et al. Reference Pauly, Mutterlose and Alsen2012a). Finds of crioceratid heteromorphs at Store Koldewey and Stratumbjerg, Wollaston Forland (Figs 2, 5) are the first ammonite evidence of the Barremian Stage. The assemblage has close affinity with assemblages described from northern Germany (Kakabadze & Hoedemaeker, Reference Kakabadze and Hoedemaeker2010). The identified taxa indicate the presence of three zones that are adopted from the lower–upper Barremian boundary interval in northern Germany. However, sampling conditions did not allow a clear separation of the zones in Greenland. The zones are accordingly indicated as undifferentiated as Fissicostaticeras fissicostatus – Paracrioceras elegans – Paracrioceras denckmanni zones (Fig. 8). Rare material from Store Koldewey has shown the presence of the uppermost Barremian Paranclyloceras bidentatum Zone (Fig. 8), also adopted from NW Europe. Deshayesites in Greenland marks the base of the Aptian Stage. Occurrences are recorded from Kuhn Ø (Bøgvad & Rosenkrantz, Reference Bøgvad and Rosenkrantz1934), Hochstetter Forland (Surlyk, Reference Surlyk1978b) and Hold with Hope (Kelly & Whitham, Reference Kelly and Whitham1999). Deshayesites are now also recorded from Store Koldewey. Lower Aptian Deshayesitid zonation is partly adopted from the lowermost Aptian strata of NW Europe. It is overlain by strata with ammonites of the genus Tropaeum that allows the adoption of the Tropaeum bowerbanki Zone from NW Europe (Fig. 8). The remaining Cretaceous succession (Albian–Campanian) only contains rare and scattered ammonites (Donovan, Reference Donovan1957). The Albian Hoplites dentatus Zone is identified by the index species. The Cenomanian in places contains a rich Schloenbachia fauna, especially in Tværdal, Geographical Society Ø, where its marks the base of the Cenomanian Stage. Rare Schloenbachia occur in Lygnaelv, Hold with Hope, followed by a thick interval without ammonites. A few reports have been made on Turonian ammonites (Spath, Reference Spath1946; Donovan, Reference Donovan1953, Reference Donovan1954) that have not been confirmed by new material. Revisits to the Scaphitid-bearing succession in the eastern Geographical Society Ø, yielded well-preserved Hoploscaphites ikorfatensis, in addition to the reports of Hoploscaphites greenlandicus providing rare ammonite dated levels of middle and late Campanian, respectively. In summary, the Cretaceous ammonite succession and zonation remains incomplete and subject to improvements. In the present study, its main purpose is to provide calibration points between the Cretaceous succession in NW Europe (commonly well-dated by ammonites) and the locally established palyno-stratigraphy in NE Greenland.

Fig. 8. Cretaceous ammonite zonation of NE Greenland. Lower Cretaceous after Surlyk (Reference Surlyk1978a, Ryazanian approximately equivalent to Berriasian), Kelly & Whitham (Reference Kelly and Whitham1999, lower Aptian), Alsen (Reference Alsen2006, Ryazanian – Hauterivian) and this work. Zones shaded in grey indicate intervals that are not present or not yet proven in NE Greenland. Zones younger than Early Cenomanian have not been observed in NE Greenland. Zones shaded in grey and with indices in white are zones referred to in correlation with the palynological zonations for the central North Sea area of Duxbury (2001) and Davey (Reference Davey2001). The indices are adopted from the (NW European) Boreal ammonite zonation (Gradstein et al. Reference Gradstein, Ogg and Smith2004) since most of the zones in NE Greenland have close affinity to that area. The two upper Volgian zones are approximately equivalent to the upper Tithonian zone (Wimbledon, Reference Wimbledon2017).
6. Palynostratigraphic zonation
The Cretaceous succession is divided into 15 biozones and intervals and 20 subzones, and more than 100 stratigraphic events representing more than 70 stratigraphic levels have been recognized (Fig. 7a–d). Dinocysts and pollen markers are illustrated in Figures 9–15.
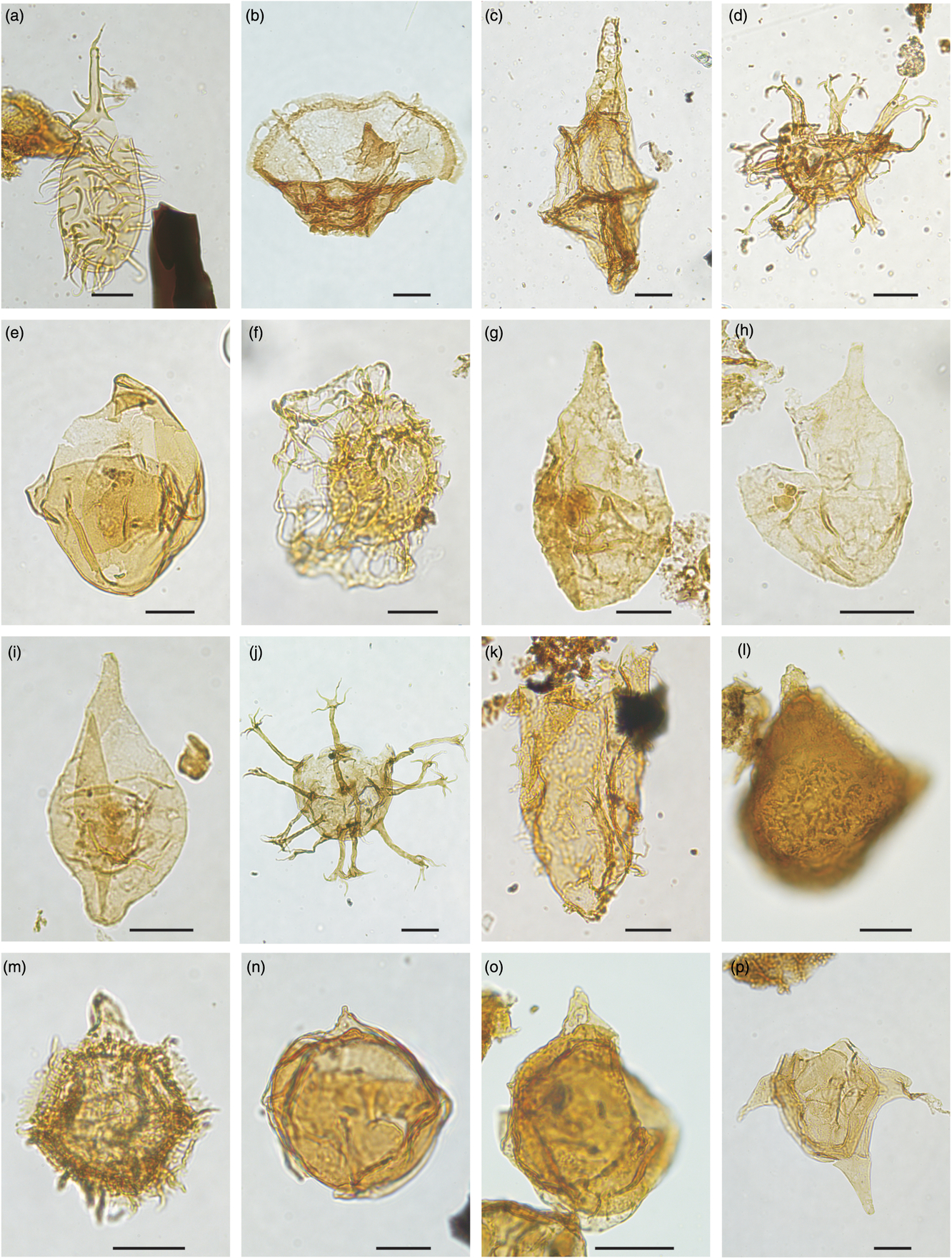
Fig. 9. Dinocysts marker of latest Jurassic – Early Cretaceous age (late Tithonian, Berriasian, Valanginian and earliest Hauterivian). Picture reference number, locality, slide number and England Finder co-ordinates are listed for the specimens illustrated. Scale bars all 20 μm. (a) Gochteodinia villosa subsp. villosa, 2324, Store Koldewey-1 core, 517002-86-7, Q37/1. (b) Isthmocystis distincta, 2332, Perisphinctes Ravine, Kuhn Ø, HNH 87-3, 342088-4, O27. (c) Scriniodinium pharo, 2329, Rødryggen-1 core, 517001-3-3, F35-4. (d) Palaecysta palmula subsp. palmula, 2328, Rødryggen-1 core, 517001-3-2, J20/1. (e) Lagenorhytis delicatula, 2307, SP-4a Store Koldewey, 523201, D23/3. (f) Rotosphaeropsis thule, 2288, Rødryggen-1 core, 517001-7-7, X29/2. (g) Paragonyaulacysta? borealis, 2286, Rødryggen-1 core, 517001-5-10, W27/1. (h) Paragonyaulacysta? borealis, 2287, Rødryggen-1 core, 517001-304-4, M18/3. (i) Paragonyaulacysta capillosa, 2298, Hvidekløft Store Koldewey, 517649-3, J60-4. (j) Oligosphaeridium complex, 2331, Rødryggen-1 core, 517001-330-2, W14/2. (k) Boreocysta isfjordica, 1411, PAL-5/2010 Store Koldewey, 495984-4, R29/3. (l) Apteodinium spongiosum, 2403, PAL-5/2010 Store Koldewey, 495988-5,W35/1. (m) Nelchinopsis kostromiensis, 2326, Store Koldewey-1 core, 517002-137-5, X70/3. (n) Endoscrinium hauterivianum, 1117, Store Koldewey-1 core, 517002-139-5, B39. (o) Endoscrinium hauterivianum, 1116, Store Koldewey-1 core, 517002-138-5, B60/3. (p) Muderongia australis, 2319, Store Koldewey-1 core, 517002-82-3, W27/4.
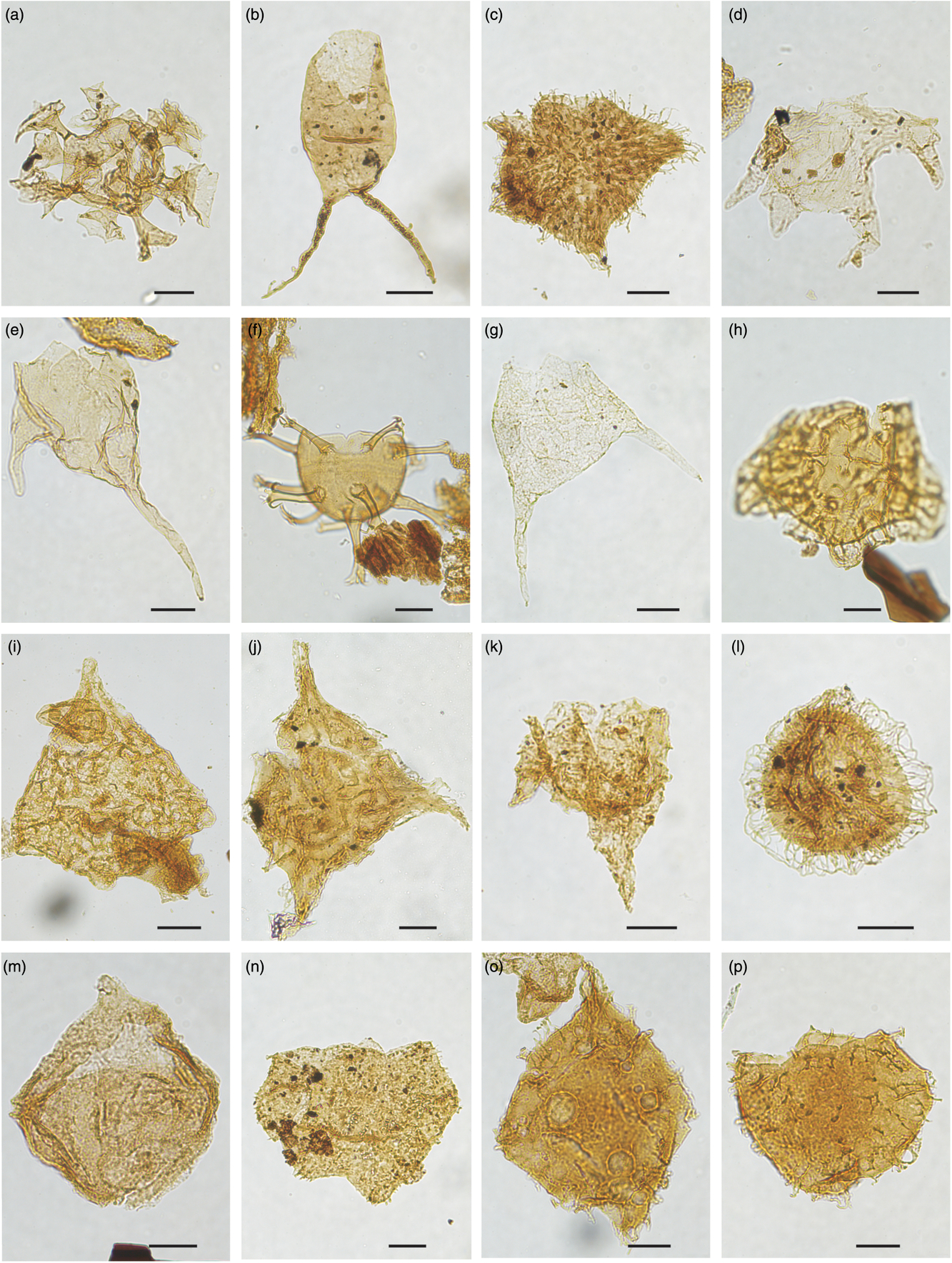
Fig. 10. Dinocysts marker of Early Cretaceous age (earliest Hauterivian, Barremian and Aptian). Picture reference number, locality, slide number and England Finder co-ordinates are listed for the specimens illustrated. Scale bars all 20 μm. (a) Oligosphaeridium umbraculum, 1111, Store Koldewey-1 core, 517002-82-4, N42-3. (b) Batioladinium longicornutum, 2334, HNH section 21 1993, 342239-4, M41/2. (c) Pseudoceratium anaphrissum, 2335, HNH section 16 1993, 351512-4, J40-3. (d) Muderongia simplex subsp. microperforata, 2316, Store Koldewey-1 core, 517002-79-6, P46. (e) Odontochitina nuda, 2317, Store Koldewey-1 core, 517002-79-6, X42. (f) Oligosphaeridium abaculum, 1096, Store Koldewey-1 core, 517002-75-5, V47/3. (g) Pseudoceratium toveae, 2336, HNH section 17 1993, 342161-5, Q49/2. (h) Pseudoceratium aff. iveri, 1098, Store Koldewey-1 core, 517002-75-3, A27/3. (i) Pseudoceratium aff. iveri, 1080, Store Koldewey-1 core, 517002-76-3, K52/2. (j) Pseudoceratium iveri, 2337, HNH section 17 1993, 342161-5, K46/2. (k) Pseudoceratium retusum, 2338, HNH section 7 1993, 342087-10, H31-2. (l) Exiguisphaera plectilis, 2339, HNH section 7 1993, 342087-4, Y39-2. (m) Apteodinium granulatum, 2310, PAL 9-2009, 518672-4, X39/4. (n) Circulodinium brevispinosum, 2340, HNH section 34 1993, 324556-4, E45/3. (o) Pseudoceratium distinctum, 2311, PAL 9-2009, 518674-4, T45. (p) Pseudoceratium distinctum, 2313, PAL 9-2009, 518674-4, H50/3.
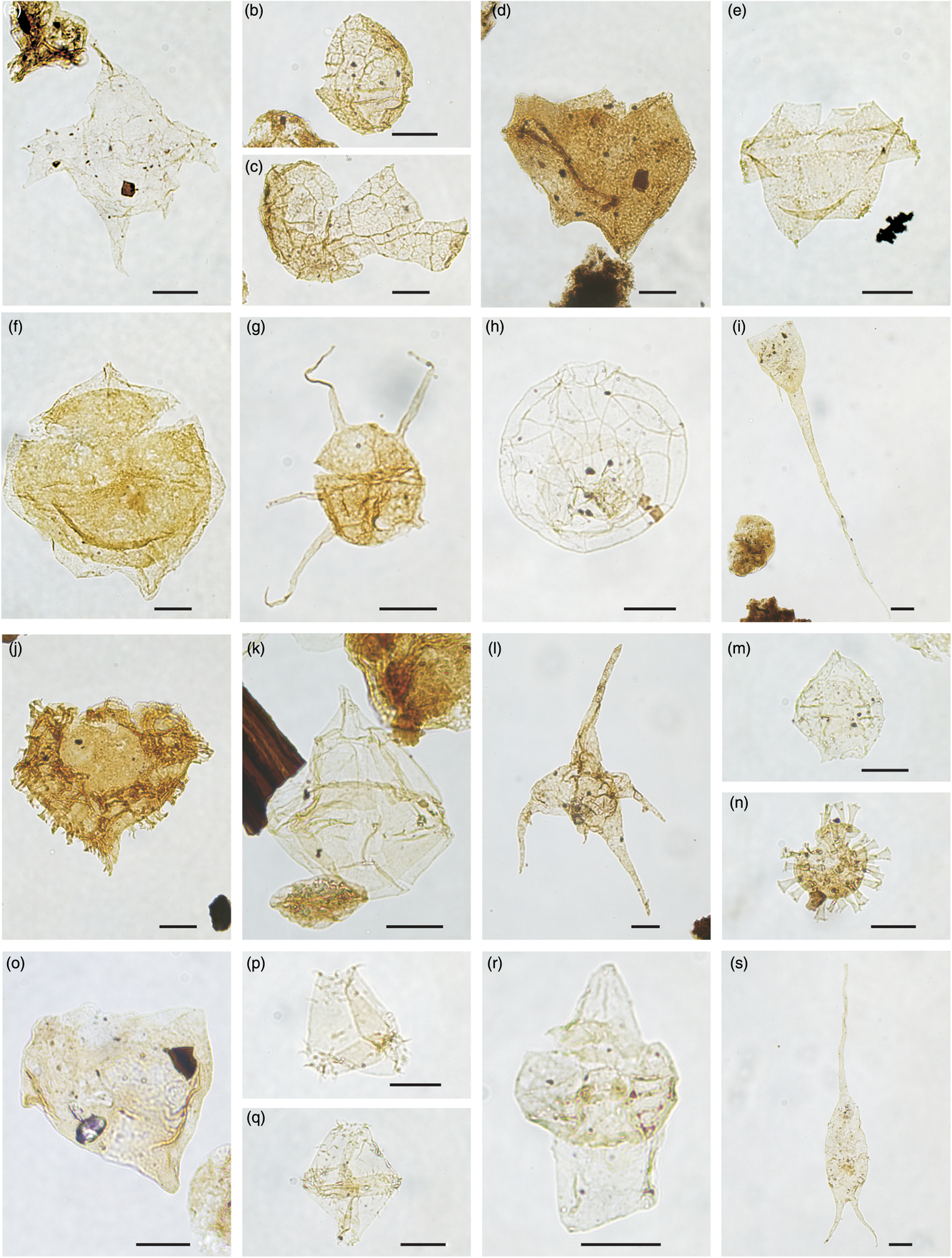
Fig. 11. Dinocysts marker of Early Cretaceous age (late Aptian – middle Albian). Picture reference number, locality, slide number and England Finder co-ordinates are listed for the specimens illustrated. Scale bars all 20 μm. (a) Vesperopsis mayi, 2341, HNH section 13 1993, 351587-4, Q35/1. (b) Leptodinium cancellatum, 2342, HNH section 12 1993, 351589-4, N34/4. (c) Leptodinium cancellatum, 2343, HNH section 20 1993, 342176-4, P50/4. (d) Canningia reticulata, 2344, HNH section 37 1993, 351592-4, F26/4. (e) Nyktericysta? vitrea, 2345, HNH section 17 1993, 342169-4, J53/4. (f) Senoniasphaera microreticulata, 2346, HNH section 17 1993, 342172-4, Q26/2. (g) Cauca parva, 2347, HNH section 30 1993, 342620-7, U20/3. (h) Hapsocysta? benteae, 2348, HNH section 30 1993, 342619-3, Q30/4. (i) Odontochitina singhii, 2349, HNH section 20 1993, 342183-4, N34/1. (j) Aptea polymorpha, 2351, HNH section 20 1993, 342191-5, U30/2. (k) Leptodinium? hyalodermopse, 2353, HNH section 17 1993, 342167-4, R23/2. (l) Vesperopsis longicornis, 2354, HNH section 34 1993, 342556-4, E33/3. (m) Chichaouadinium vestitum, 2355, HNH section 20 1993, 342202-4, T23/3. (n) Litosphaeridium arundum, 2356, HNH section 23 1993, 351670-4, F34/3. (o) Pseudoceratium expolitum, 2357, HNH section 20 1993, 342194-4, R25-1. (p) Rhombodella paucispina, 2358, HNH section 30 1993, 342615-3, S31/4. (q) Rhombodella paucispina, 2359, HNH section 30 1993, 342615-3, Q36/3. (r) Ovoidinium sp. 3 HNH 1993, 2360, HNH section 21 1993, 342240-7, D46/3. (s) Batioladinium shaftesburiense, 2361, HNH section 26 1993,351630-4, D43/3.
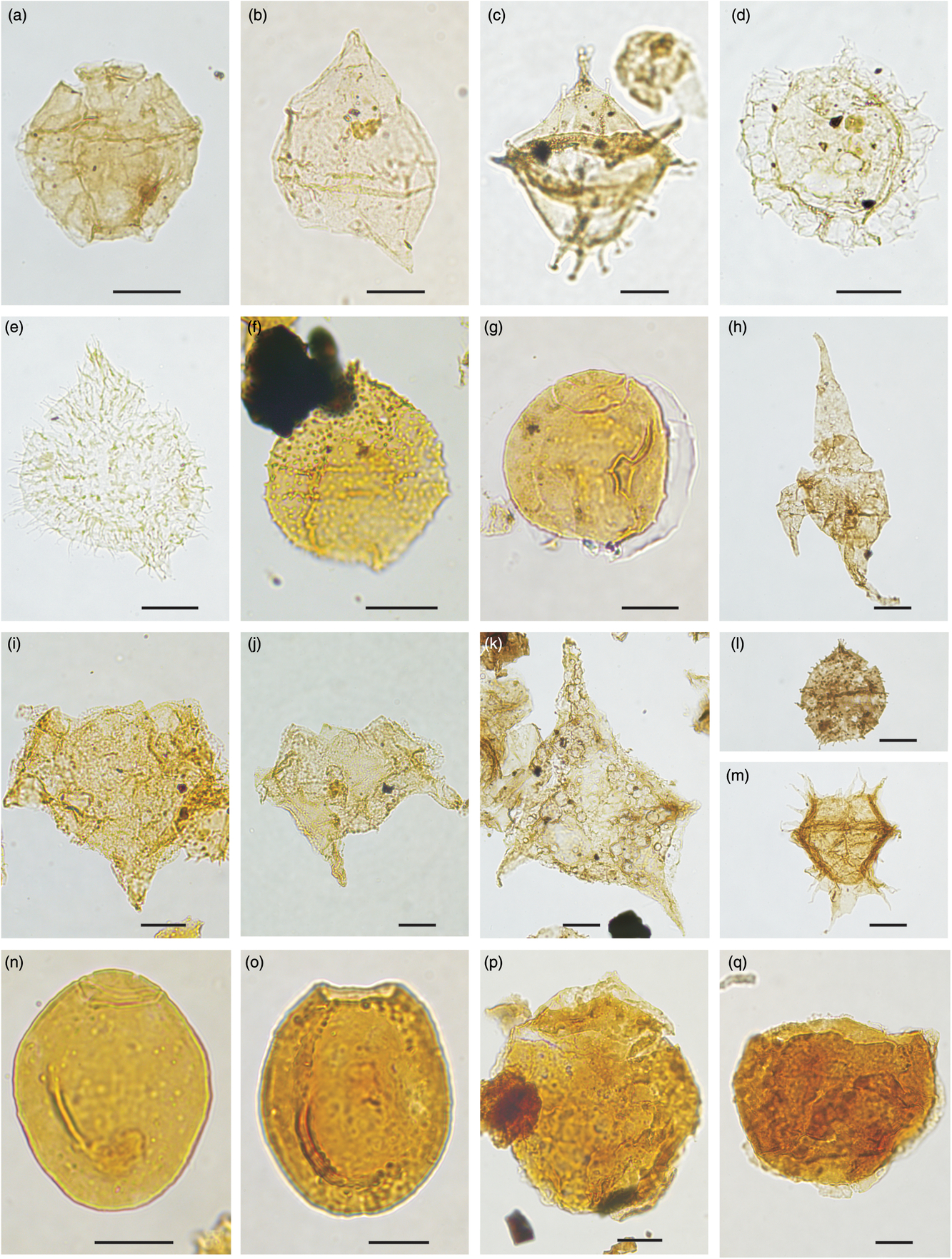
Fig. 12. Dinocysts marker of Early–Late Cretaceous age (middle Albian – earliest Cenomnian). Picture reference number, locality, slide number and England Finder co-ordinates are listed for the specimens illustrated. Scale bars all 20 μm. (a) Ellipsoidictyum imperfectum, 2363, HNH section 21 1993, 342240-4, W20/1. (b) Subtilisphaera kalaallitii, 1484, HNH section 30 1993, 522066-4, U66/2. (c) Wigginsiella grandstandica, 2364, HNH section 29 1993, 324599-4, X24/3. (d) Dino sp. 1 HNH 1993, 2371, HNH section 31 1993, 324623-4, C18/4. (e) Circulodinium sp. 1 HNH 1993, 2370, HNH section 30 1993, 324619-3, H53/4. (f) Epelidosphaeridia sp. 1 HNH 1993, JHOV 2/2009, 518602-7, R45/1. (g) Sindridinium? torulosa, 1560, HNH section 30 1993, 324608-8, W21/4. (h) Odontochitina ancala, 2367, HNH section 36 1993, 324092-7, K55/2. (i) Endoceratium exquisitum, 1221, HNH 081410-4, 487620-3, T17-4. (j) Endoceratium exquisitum, 1519, PAL3-2011, 522085-4, X45/3. (k) Endoceratium exquisitum, 2382, HNH 081009-4, 475197-3, W39/2. (l) Epelidosphaeridia spinosa, 2373, HNH section 39 1993, 335321-4, X34/4. (m) Dinopterygium alatum, 2368, HNH section 30 1993, 324613-8, N42/3. (n) Sindridinium borealis, 1463, PAL3-2011, 522076-4, U53/1. (o) Sindridinium borealis, 1462, PAL3-2011, 522076-4, W53/4. (p) Senoniasphaera aff. microreticulata, 1218, HNH 081410-4, 487640-3, D42/2. (q) Senoniasphaera aff. microreticulata, 1203, HNH 081410-4, 487633-3, P46/4.
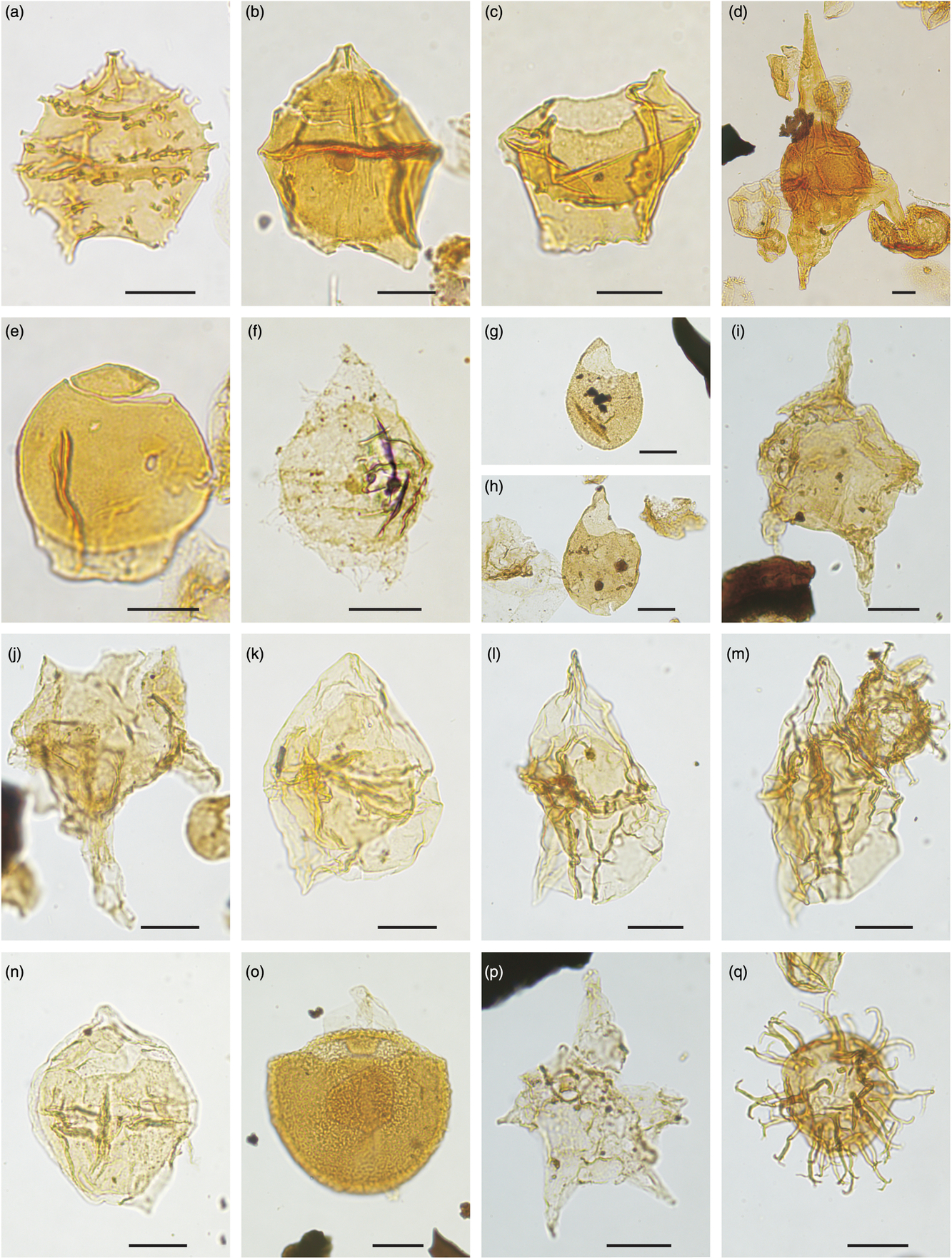
Fig. 13. Dinocysts marker of Late Cretaceous age (earliest Cenomnian – late Cenomanian). Picture reference number, locality, slide number and England Finder co-ordinates are listed for the specimens illustrated. Scale bars all 20 μm. (a) Epelidosphaeridia manifesta, 1206, HNH 081410-4, 487643-3, F52/4. (b) Ovoidinium epelidosphaeroides, 1507, PAL3-2011, 522082-3, Y27/1. (c) Ovoidinium epelidosphaeroides, 1516, PAL3-2011, 522084-5, K33/3. (d) Xenascus aff. plotei, 1551, HNH 081410-4, 487620-3, B27/4. (e) Sindridinium anaanae, 1464, HNH 081410-4, 487631-3, O28/2. (f) Palaeohystrichophora infusorioides, 2374, HNH section 30 1993, 324613-8, O51/3. (g) Ginginodinium aff. evittii, 2376, HNH 081009-1, 475185-2, C27. (h) Ginginodinium aff. evittii, 2377, HNH 081009-1, 475185-2, W48/2. (i) Endoceratium ludbrookiae, 2383, HNH 081009-2, 475190-2, Q41/4. (j) Endoceratium ludbrookiae, 2384, HNH 081009-2, 475195-3, G35/3. (k) Isabelidinium acuminatum, 0098, Nanok-1 core, 517004-136-5,S20/1. (l) Isabelidinium magnum, 2387, HNH 080209-4, 475175-2, Y32. (m) Isabelidinium magnum, 2386, HNH 080209-4, 475175-2, O20/2. (n) Trithyrodinium aff. rhomboideum, 2378, HNH 080710-4, 475435-6, Y23/3. (o) Trithyrodinium suspectum, 2380, Knudshoved locality 5, 433504-4, J37/1. (p) Nyktericysta aff. tripenta, 2381, HNH 081009-2, 475197-2, X36/2. (q) Surculosphaeridium longifurcatum, 2390, HNH 080209-4, 475179-2, K22/3.
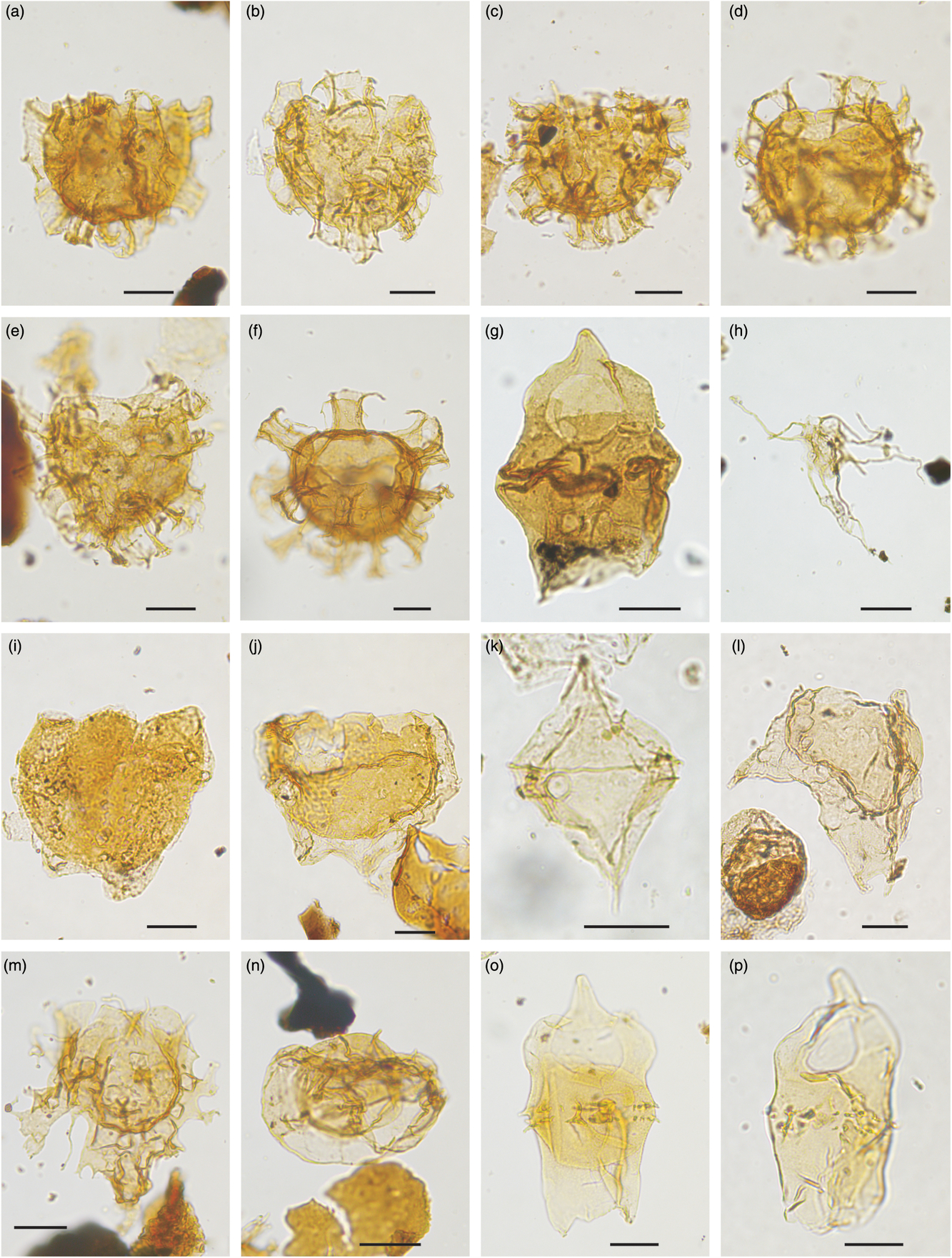
Fig. 14. Dinocysts marker of Late Cretaceous age (late Cenomanian – middle Coniacian). Picture reference number, locality, slide number and England Finder co-ordinates are listed for the specimens illustrated. Scale bars all 20 μm. (a) Cyclonephelium compactum-Cauveridinium membraniphorum complex, 118, Nanok-1 core, 517004-116-6, B42/2. (b) Cyclonephelium compactum-Cauveridinium membraniphorum complex, 97, Nanok-1 core, 517004-136-5, T48/2. (c) Cyclonephelium compactum-Cauveridinium membraniphorum, complex, 110, Nanok-1 core, 517004-117-7, W71/2. (d) Heterosphaeridium difficile, 116, Nanok-1 core, 517004-166-6, N58/4. (e) Heterosphaeridium difficile, 115, Nanok-1 core, 517004-166-6, G41/1. (f) Heterosphaeridium difficile, 125, Nanok-1 core, 517004-316-9, H53/1. (g) Chatangiella granulifera, 2379, Nanok-1 core, 517004-110-7, L59/2. (h) Raphidodinium fucatum, 2397, Nanok-1 core, 517004-113-3, F23/2. (i) Senoniasphaera rotundata, 1705, Nanok-1 core, 517004-101-5, M59/1. (j) Senoniasphaera rotundata, 1707, Nanok-1 core, 517004-317-5, B28/4. (k) Laciniadinium arcticum, 2300, 470105-2, HNH081508-1, M18/2. (l) Odontochitina rhakodes, 1767, Nanok-1 core, 517004-106-, Y63/3. (m) Xenascus gochtii, 124, Nanok-1 core, 517004-101-5, Y52/4. (n) Stephodinium coronatum, 126, Nanok-1 core, 517004-317-3, W31/4. (o) “Chatangiella spinosa”, 134, Nanok-1 core, 517004-311-10, M38/2. (p) “Chatangiella spinosa”, 130, Nanok-1 core, 517004-315-9, B40/1.
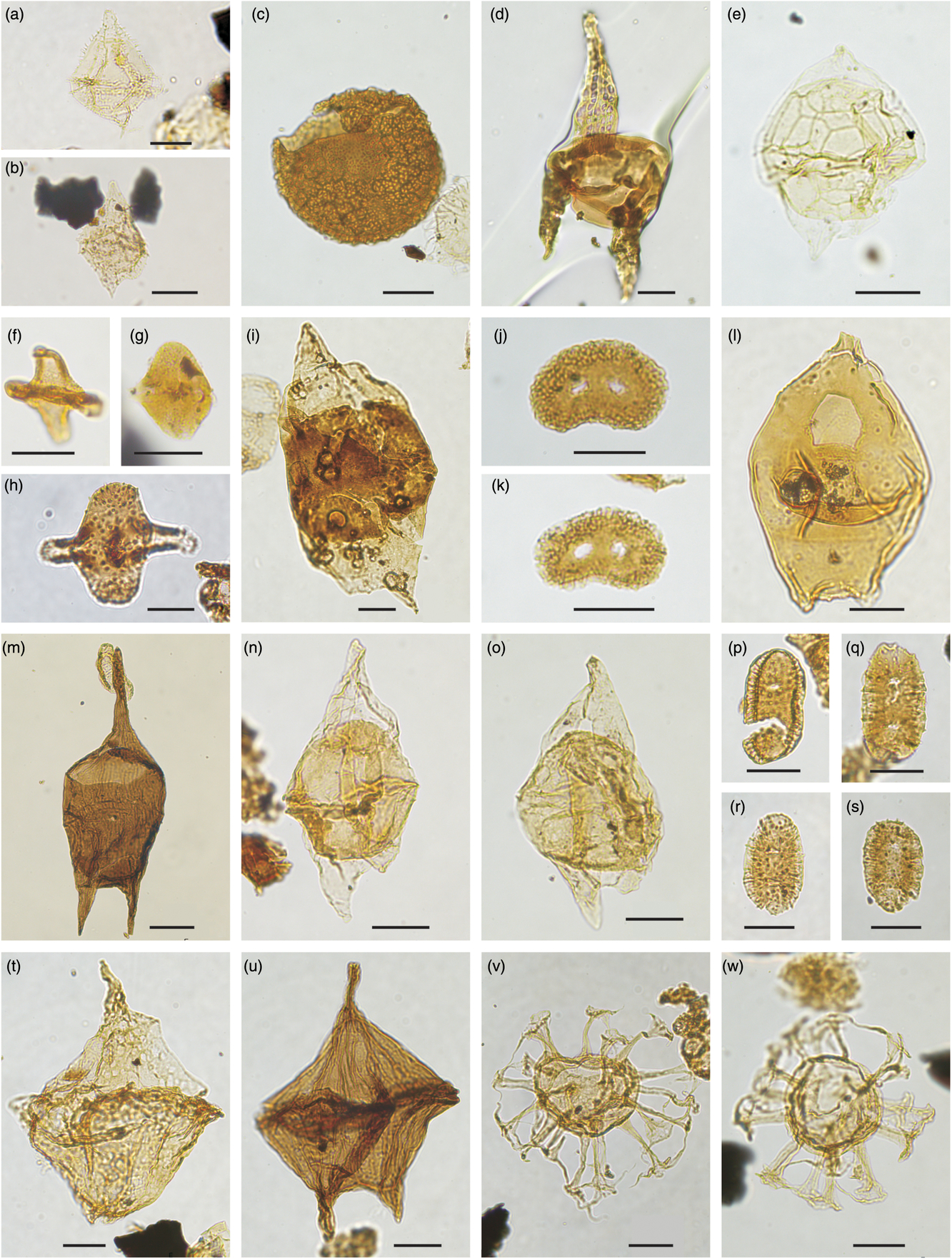
Fig. 15. Dinocysts marker of Late Cretaceous age (late Coniacian – ?Early Maastrichtian). Picture reference number, locality, slide number and England Finder co-ordinates are listed for the specimens illustrated. Scale bars all 20 μm. (a) Spinidinium echinoideum, 132, Nanok-1 core, 517004-314-9, T23/4. (b) Spinidinium echinoideum, 129, Nanok-1 core, 517004-317-3, F34/2. (c) Trithyrodinium vermiculatum, 2394, HNH 080209-4, 475134-2, S44/3. (d) Odontochitina porifera, 2391, Knudshoved locality 5, 433898-4, W20/2. (e) Alterbidinium ioannidesii, 2395, HNH 080209-3, 475113-8, V36/4. (f) Aquilapollenites sp., 136, Nanok-1 core, 517004-81-2, T47/2. (g) Aquilapollenites sp., 135, Nanok-1 core, 517004-313-4, G48. (h) Aquilapollenites sp., 2270, HNH081508-2, 470107-5, V15/2. (i) Chatangiella bondarenkoi, 2296, PAL-6/2011, 529719-6, V21-3. (j) Azonia fabacea, 2400, Knudshoved locality 5, 700802-4, V21/1. (k) Azonia fabacea, 2401, Knudshoved locality 5, 700802-4, K24/4. (l) Isabelidinium magnum, 2297, PAL-6/2011, 529716-4, T30/4. (m) Cerodinium diebelii, 2238, HNH081508-2, 470102-5, B17-2. (n) Alterbidinium biaperturum, 2241, HNH081508-2, 470102-4, J31/2. (o) Alterbidinium biaperturum, 2239, HNH081508-2, 470102-3, N40/3. (p) Wodehouseia gracile, 2272, HNH081508-2, 470107-5, Y25/1. (q) Wodehouseia gracile, 2243, HNH081508-2, 470102-6, Q18/2. (r) Wodehouseia gracile, 2234, HNH081508-2, 470102-1, X14/2. (s) Wodehouseia gracile, 2269, HNH081508-2, 470106-2, G42/2. (t) Spongodinium delitiense, 2247, HNH081508-2, 470104-3, C30. (u) Phelodinium kozlowskii, 2244, HNH081508-2, 470102-6, W20. (v) Hystrichosphaeridium sp. 3 McIntyre Reference McIntyre1974, 2268, HNH081508-2, 470106-4, X48. (w) Hystrichosphaeridium sp. 3 McIntyre Reference McIntyre1974, 2266, HNH081508-2, 470106-4, V39/2.
6.a. Zone: Gochteodinia villosa villosa (NEG Cr 1) new
Age: latest Tithonian – latest Berriasian, latest Jurassic – Early Cretaceous.
Definition: From the FO of Gochteodinia villosa villosa to the FO of Oligosphaeridium complex.
Comments: Gochteodinia villosa villosa appears in the Praechetaites tenuicostatum – Praetollia maynci ammonite zones in the Rødryggen-1 core (517001) Wollaston Forland (Fig. 5) near the Jurassic–Cretaceous boundary and in Perisphinctes Ravine, Kuhn Ø, section HNH 10 (Nøhr-Hansen, Reference Nøhr-Hansen1993). The nominate species G. villosa villosa has a stratigraphic range in NE Greenland, spanning the entire zone. The range of the G. villosa villosa Zone is from near the Jurassic–Cretaceous boundary to the uppermost Berriasian (c. uppermost Ryazanian) in NE Greenland. The autonym subspecies G. villosa villosa is applied here to distinguish this zone from earlier G. villosa zones and to focus on the range of this subspecies in contrast to the ranges of other present or future subspecies. Gochteodinia villosa refers to the species in the following discussion.
Woollam & Riding (Reference Woollam and Riding1983) defines the G. villosa Zone (Gv) in England based on Davey’s (Reference Davey1979) “Pareodinia dasyforma” Zone. The definition of the zone is based on species that are not common in East Greenland (e.g. Ctenidodinium panneum (now Dichadogonyaulax? pannea) and C. culmula (now D? cumula)). However, the zone nearly coincides with the range of G. villosa. The FO of G. villosa is in the basal Paracraspedites oppressum ammonite Zone (uppermost Tithonian) to the Peregrinus albidum ammonite Zone (uppermost Berriasian), that is, a range very similar to that recorded in East Greenland. The range of G. villosa may extend into basal Valanginian (e.g. Costa & Davey, Reference Costa, Davey and Powell1992), but may be reworked into higher strata.
On the Russian Platform, Riding et al. (Reference Riding, Fedorova and Ilyina1999) report the first appearance of rare G. villosa in the Kachpurites fulgens ammonite Zone (correlative to the Subcraspedites primitivus ammonite Zone of NW Europe) and then again very near the Tithonian–Berriasian (Jurassic–Cretaceous) boundary. Riding et al. (Reference Riding, Fedorova and Ilyina1999) suggest a G. villosa Zone (RPJ17) defined from the FO of G. villosa to the FO of Batioladinium spp. in the basal upper Ryazanian (c. upper Berriasian; Rjazanensis-Spasskensis ammonite Zone). The G. villosa villosa Zone does not correlate well with dinoflagellate zones in the corresponding successions on Svalbard, the Janusfjellet Formation, because G. villosa villosa is not recorded below the basal Valanginian, probably due to an impoverished Boreal dinocyst flora in the pre-Valanginian strata (Århus, Reference Århus1988). Consequently, the local FO of G. villosa villosa coincides with the FO of Oligosphaeridium complex (Århus, Reference Århus1988). Similarly, Boreal Volgian–Ryazanian (c. upper Berriasian) dinocyst assemblages without G. villosa villosa are reported from cores in the Barents Sea (Århus et al. Reference Århus, Kelly, Collins and Sandy1990).
Gochteodinia villosa occurs in the subboreal regions of NW Europe and the Russian Platform as well as the Boreal East Greenland apparently with similar FOs based on correlation of local ammonite zonations. The last occurrence of G. villosa is not well constrained; the upper boundary of the G. villosa villosa Zone is therefore selected at the widespread and distinct FO of O. complex. The G. villosa villosa Zone (NEG Cr 1) is a robust dinocyst biozone of the lowermost Cretaceous deposits in the North Atlantic region. However, G. villosa villosa is rare or absent in the high Boreal regions of Canada, North Greenland, the Barents Sea and northern Siberia (Davies, Reference Davies1983, Århus, Reference Århus1988; Århus et al. Reference Århus, Kelly, Collins and Sandy1990; Smelror et al. Reference Smelror, Mørk, Monteil, Rutledge and Leereveld1998; Pestchevitskaya, Reference Pestchevitskaya2007a, b; Nikitenko et al. Reference Nikitenko, Pestchevitskaya, Lebedeva and Ilyina2008).
Stratigraphic events: Istmocystis distincta has FO contemporaneous with G. villosa villosa. The FO of Lagenorhytis delicatula, Palaecysta palmula and Phoberocysta neocomica and the LO of Rotosphaeropsis thule occur in or above the Hectoroceras kochi ammonite Zone (near the lower–upper Berriasian boundary) in the Rødryggen-1 core, Wollaston Forland (Fig. 5). Gochteodinia villosa villosa, Paragonyaulacysta? borealis and P. capillosa have their stratigraphic LO in the upper part of the G. villosa villosa Zone, upper Berriasian in the Rødryggen-1 core, even though they are reported to continue into lower Valanginian successions in Canada, Svalbald, the Barents Sea, Siberia and Arctic Russia (e.g. Davies, Reference Davies1983; Århus, Reference Århus1988; Pestchevitskaya, Reference Pestchevitskaya2007a, b; Nikitenko et al. Reference Nikitenko, Pestchevitskaya, Lebedeva and Ilyina2008).
6.b. Zone: Oligosphaeridium complex (NEG Cr 2) new
Age: latest Ryazanian (c. latest Berriasian) – earliest Hauterivian, Early Cretaceous.
Definition: From the FO of Oligosphaeridium complex to the FO of Batioladinium longicornutum.
Comments: Oligosphaeridium complex appears (FO) in the Peregrinus albidum ammonite Zone, uppermost Berriasian (or lower Valanginian according to Gradstein et al. Reference Gradstein, Ogg, Schmitz and Ogg2012; in SP-04a, SP-15a Koldewey, Fig. 2) and is abundant in the lower Valanginian deposits (e.g. Rødryggen-1 core (517001) Wollaston Forland, Fig. 5; PAL05/2010 , Koldewey, Fig. 2). In NW Europe, Costa & Davey (Reference Costa, Davey and Powell1992) and Duxbury (Reference Duxbury2001) suggest that the FO of Oligosphaeridium complex occurs in the lowermost Valanginian strata.
Stratigraphic events: The FO of abundant Oligosphaeridium complex is followed by the FO of Apteodinium spongiosum, Boreocysta isfjordica and Nelchinopsis kostromiensis in the lower part of this zone (PAL05/2010 , Koldewey, Fig. 2) and by the FO of Endoscrinium hauterivianum and Muderongia australis in the uppermost part of the zone (Store Koldewey-1 core (517002), Fig. 2). Duxbury (Reference Duxbury2001) mentioned that Endoscrinium hauterivianum is essentially a lower Hauterivian form that can range into the upper Hauterivian Stage.
6.c. Zone: Batioladinium longicornutum (I) of Nøhr-Hansen (Reference Nøhr-Hansen1993) redefined
Age: early Hauterivian – latest Barremian.
Definition: From the FO to the LO of Batioladinium longicornutum.
Comments: Nøhr-Hansen’s (Reference Nøhr-Hansen1993) original definition of the lower boundary of the zone is here redefined from the FO of Muderongia australis to the slightly younger FO of Batioladinium longicornutum, which seems to be a more reliable marker. The zone was subdivided into the three subzones by Nøhr-Hansen (Reference Nøhr-Hansen1993): Nelchinopsis kostromiensis (1) redefined, Pseudoceratium anaphrissum (2) and Pseudoceratium toveae (3). Detailed studies of a core and numerous sections from Store Koldewey have enabled a refinement of the dinoflagellate stratigraphy of the Batioladinium longicornutum (I) Zone, Nøhr-Hansen (Reference Nøhr-Hansen1993).
Correlations: The FO of B. longicornutum correlates with the middle part of the lowermost Hauterivian Endomoceras amblygonium ammonite Zone according to Davey’s (Reference Davey2001; Fig. 8) dinocyst correlation with lower Hauterivian ammonite stratigraphy at Speeton, east England. B. longicornutum has its LO in the upper Barremian Stage (Nøhr-Hansen, Reference Nøhr-Hansen1993).
6.d. Subzone: Nelchinopsis kostromiensis (1) of Nøhr-Hansen (Reference Nøhr-Hansen1993) redefined
Age: early–late Hauterivian.
Definition: From FO of Batioladinium longicornutum to the LO of Nelchinopsis kostromiensis.
Comments: Nøhr-Hansen’s (Reference Nøhr-Hansen1993) original definition of the lower boundary of the zone is redefined from the FO of Muderongia australis to the slightly younger FO of Batioladinium longicornutum, which seems to be a more reliable marker.
Stratigraphic events: The FO of B. longicornutum at the base and LO of Endoscrinium hauterivianum in the middle part of the subzone. Other stratigraphic markers are the FO of Pseudoceratium anaphrissum and the LO of Apteodinium spongiosum, Muderongia australis and Stanfordella ordocavain the upper part of the subzone. The distinct species Oligosphaeridium umbraculum has been recorded from the lowermost part of the present subzone.
Correlations: The FO of B. longicornutum correlates with the middle part of the lowermost Hauterivian Endomoceras amblygonium ammonite Zone according to Davey’s (Reference Davey2001) dinocyst correlation with lower Hauterivian ammonite stratigraphy at Speeton, east England. The LO of Endoscrinium hauterivianum in the present subzone may correlate with the LO of the species in the lowermost part of the upper Hauterivian LKP16.1 Zone (Duxbury, Reference Duxbury2001), which is approximately equivalent to the Simbirskites (Milanowskia) speetonensis ammonite Zone (Fig. 8). Originally O. umbraculum was described from the lower Hauterivian Stage (Duxbury, Reference Duxbury2001). Nelchinopsis kostromiensis and Phoberocysta neocomica are common in the Nelchinopsis kostromiensis Subzone, which is well-represented in the Store Koldewey-1 core (517002, Fig. 2). Recently, the subzone has been recorded from Genetic Sequence 1 (approximately equivalent to parts of the Knurr and Klippfisk formations) Loppa High, southwestern Barents Sea (Fig. 6) by Marin et al. (Reference Marin, Escalona, Grundvåg, Nøhr-Hansen and Kairanova2018).
6.e. Subzone: Pseudoceratium anaphrissum (2) of Nøhr-Hansen (Reference Nøhr-Hansen1993)
Age: early Barremian.
Definition: From the LO of Nelchinopsis kostromiensis to the LO of Pseudoceratium anaphrissum.
Stratigraphic events: Hystrichosphaeridium arborispinum, Muderongia simplex subsp. microperforata, Oligosphaeridium asterigerum and Pseudoceratium anaphrissum are often common to abundant within the subzone. Muderongia simplex subsp. microperforata has FO in the lower part wheras Oligosphaeridium abaculum, Odontochitina nuda, Pseudoceratium aff. iveri and P. toveae have FO in the upper part of the subzone.
Correlation: The LO of Nelchinopsis kostromiensis correlates with the uppermost Hauterivian Simbirskites variabilis ammonite Zone (Gradstein et al. Reference Gradstein, Ogg and Smith2004). Previously, the Simbirskites variabilis Zone was thought to represent the lowermost Barremian Stage (Kemper et al. Reference Kemper, Rawson and Thieuloy1981). Recently, the subzone has been recorded from the Rurikfjellet and Helvetiafjellet formations on Spitsbergen and from Genetic Sequence 1 (approximately equivalent to the Knurr Formation) Barents Shelf by Grundvåg et al. (Reference Grundvåg, Marin, Kairanov, Śliwińska, Nøhr-Hansen, Escalona and Olaussen2017), and from Genetic Sequence 1 (approximately equivalent to parts of the Knurr and Klippfisk formations) Loppa High, southwestern Barents Sea (Fig. 6) by Marin et al. (Reference Marin, Escalona, Grundvåg, Nøhr-Hansen and Kairanova2018).
6.f. Subzone: Pseudoceratium toveae (3) of Nøhr-Hansen (Reference Nøhr-Hansen1993)
Age: late Barremian.
Definition: From the LO of Pseudoceratium anaphrissum to the LO of Batioladinium longicornutum.
Stratigraphic events: An acme of Pseudoceratium toveae occurs in this subzone. The species Pseudoceratium aff. iveri has its LO within the lower part and Pseudoceratium iveri has its FO in the upper part of the subzone. Pseudoceratium aff. iveri is common in the northern part of Store Koldewey, where it occurs in the major part of the Store Koldewey-1 core (517002, Fig. 2). The middle part of the subzone is well represented on Store Koldewey, for example at PAL-6/2010 (Fig. 2), between Ravn Pynt and Trækpasset where it can be calibrated with ammonites of the Fissicostaticeras fissicostaum – Paracrioceras denckmanni zones (Fig. 8) and in the Midter Gneisnæs area (MBJ-049, 053/2010, Fig. 2), where it is further characterized by the presence of brackish to marginal marine dinocysts (Vesperopsis longicornis and Hurlandsia rugara), the zygospore Tetrangulare conspicuum and the colonial algae Scenedesmus.
Correlations: Pseudoceratium aff. iveri has previously been recorded as ?Pseudoceratium anaphrissum in the lower part of the Pseudoceratium toveae (3) Subzone at Rødryggen and Stratumbjerg, Wollaston Forland (sections 16, 21, Nøhr-Hansen, Reference Nøhr-Hansen1993, Fig. 5).
The species P. iveri was previously recorded only from the upper part of the Pseudoceratium toveae (3) Subzone e.g. at Stratum Bjerg, Wollaston Forland (section 21, Nøhr-Hansen, Reference Nøhr-Hansen1993; Fig. 5) and from the Diener Bjerg area (PAL-9/2009, Fig. 4), northern Hold with Hope (Nøhr-Hansen, Reference Nøhr-Hansen1993). In the present study the species is also recorded from the lowermost part of the overlying Odontochitina nuda (II) Zone (previously the Pseudoceratium nudum Zone of Nøhr-Hansen, Reference Nøhr-Hansen1993) from the Stensiö Plateau Member of the Steensby Bjerg Formation (Figs 6, 7) at Stensiö Plateau (HNH 081310-1, Fig. 4), northern part of Hold with Hope. The record of the ammonite Prodeshayesites sp. by Kelly & Whitham (Reference Kelly and Whitham1999) at Stensiö Plateau dates the Stensiö Plateau Member as earliest Aptian Prodeshayesites fissicostatus Zone, and confirms that P. iveri range into the lowermost part of the lower Aptian Odontochitina nuda (II) Zone. The zone has previously been recorded from the south Sabine section, Melville Island, Arctic Canada (Nøhr-Hansen & McIntyre, Reference Nøhr-Hansen and McIntyre1998). The subzone has recently been recorded from the Kap Rigsdagen beds, Valdemar Gluckstad Land in North Greenland (Fig. 1) by Piasecki et al. (Reference Piasecki, Nøhr-Hansen and Dalhoff2018) and from the upper part of the Genetic Sequence 1 (approximately equivalent to the middle part of the Kolje Formation) from Loppa High, southwestern Barents Sea by Marin et al. (Reference Marin, Escalona, Grundvåg, Nøhr-Hansen and Kairanova2018; Fig. 6), and from the lower part of the Genetic Sequence 2 (approximately equivalent to the upper part of the Kolje Formation) from Barents Shelf and the north-central Barents Sea by Grundvåg et al. (Reference Grundvåg, Marin, Kairanov, Śliwińska, Nøhr-Hansen, Escalona and Olaussen2017) and Kairanov et al. (Reference Kairanov, Escalona, Mordasova, Śliwińska and Suslova2018), respectively.
6.g. Zone: Odontochitina nuda (II) (previously Pseudoceratium nudum Zone of Nøhr-Hansen, Reference Nøhr-Hansen1993)
Age: early Aptian.
Definition: From the LO of Batioladinium longicornutum to the LO of Odontochitina nuda.
Comments: Dinocysts characterizing the Odontochitina nuda (II) Zone from the Midter Gneisnæs area, Store Koldewey (MBJ-049, –53/2010, Fig. 2) have been recorded from a concretion with the ammonite Deshayesites (Prodeshayesites) cf. bodei, suggesting the Prodeshayesites bodei Subzone of the Prodeshayesites fissicostatus ammonite Zone is of earliest Aptian age (Fig. 8).
Stratigraphic events: Pseudoceratium aff. iveri and Pseudoceratium toveae both have their LO in the lower part, the FO of Pseudoceratium retusum is in the middle part and the LO of Odontochitina nuda and Exiguisphaera plectilis are in the top of the zone. Subtilisphaera perlucida and Vesperopsis longicornis are common within the zone and Protoellipsodinium clavulus is present.
Correlations: The presence of Protoellipsodinium clavulus seems to be a good lower Aptian marker in NE Greenland. It occurs in the Gulelv Member of the Steensby Formation at Diener Bjerg and in the laterally equivalent Rødelv Member of the Stratumbjerg Bjerg Formation (Fig. 6) at Steensby Bjerg (East) in the northern part of Hold with Hope. Previously, it was recorded only from the lower part of the Odontochitina nuda (II) Zone at Aucellabjerget, Wollaston Forland (Nøhr-Hansen, Reference Nøhr-Hansen1993; section 17, Fig. 5). The zone has previously been recorded from the Mesa Creek section, Ellesmere Island, Arctic Canada (Nøhr-Hansen & McIntyre, Reference Nøhr-Hansen and McIntyre1998; Fig. 1). Recently, the zone has been recorded from the Carolinefjellet Formation (Fig. 6), Spitsbergen by Grundvåg et al. (Reference Grundvåg, Marin, Kairanov, Śliwińska, Nøhr-Hansen, Escalona and Olaussen2017), and questionably from Genetic Sequence 2 (approximately equivalent to the upper Kolje Formation – lower Kolmule Formation) on the Barents Shelf by Grundvåg et al. (Reference Grundvåg, Marin, Kairanov, Śliwińska, Nøhr-Hansen, Escalona and Olaussen2017), southwestern Barents Sea by Marin et al. (Reference Marin, Escalona, Śliwińska, Nøhr-Hansen and Mordasova2017), the Loppa High, southwestern Barents Sea by Marin et al. (Reference Marin, Escalona, Grundvåg, Nøhr-Hansen and Kairanova2018) and possibly from Genetic Sequence 3 (approximately equivalent to the lower Kolmule Formation) north central Barents Sea by Kairanov et al. (Reference Kairanov, Escalona, Mordasova, Śliwińska and Suslova2018).
6.h. Zone: Circulodinium brevispinosum (III) of Nøhr-Hansen (Reference Nøhr-Hansen1993)
Age: early Aptian to Early Albian.
Definition: From the LO of Odontochitina nuda to the LO of Circulodinium brevispinosum.
Comments: The zone was subdivided into four subzones by Nøhr-Hansen (Reference Nøhr-Hansen1993): Vesperopsis longicornis (1), Vesperopsis mayi (2), Senoniasphaera microreticulata (3) and Leptodinium? hyalodermopse (4). Detailed studies of two sections through the Rødelv Member from Diener Bjerg (PAL-9/2009 , Fig. 4) and Steensby Bjerg (East) (LHN 080810-2, Fig. 4) in the northern part of Hold with Hope have refined the dinoflagellate stratigraphy of the Circulodinium brevispinosum (III) Zone (see below).
Correlations. The zone has previously been recorded from the Mesa Creek section and the Rollrock River section, Ellesmere Island and the Junction Diapir section, Axel Heiberg Island, Arctic Canada (Nøhr-Hansen & McIntyre, Reference Nøhr-Hansen and McIntyre1998; Fig. 1). Recently, the zone has been recorded from the genetic sequences 2–3/?4 (approximately equivalent to the Kolmule Formation; Fig. 6) Loppa High, southwestern Barents Sea by Marin et al. (Reference Marin, Escalona, Grundvåg, Nøhr-Hansen and Kairanova2018).
6.i. Subzone: Vesperopsis longicornis (1) of Nøhr-Hansen Reference Nøhr-Hansen1993
Age: late early – early late Aptian. This revised age assignment differs slightly from the late early Aptian age of Nøhr-Hansen (Reference Nøhr-Hansen1993).
Definition: From the LO of Odontochitina nuda to the FO Vesperopsis mayi.
Stratigraphic events: FO of Circulodinium brevispinosum and Apteodinium granulatum at the base of the zone, common Apteodinium granulatum in the middle part and FO and LO of Pseudoceratium distinctum in the middle and upper part, respectively. Vesperopsis longicornis is common to abundant throughout the subzone.
Correlations: The common occurrence of Apteodinium granulatum and the FO of P. distinctum (Rødelv Member sample 518674 from Diener Bjerg, PAL-9/2009, Fig. 4) in the middle part of the zone may correlate with the dinocyst zones LKP27.2 or LKP28 of Duxbury (Reference Duxbury2001), which he tentatively correlates with the upper part of the Tropaeum bowerbanki ammonite Zone (uppermost lower Aptian) and the lowermost Epicheloniceras martinoides ammonite Zone (lower upper Aptian; Fig. 8), respectively. A Tropaeum bowerbanki Zone ammonite has also been recorded from the V. longicornis Subzone in the Rødelv Member at Diener Bjerg (section PAL-7/2009). The LO of P. distinctum in the middle part of the subzone correlates with dinocyst LKP30 Zone of Duxbury (Reference Duxbury2001), tentatively correlated with the Parahoplites nutfieldensis ammonite Zone (middle upper Aptian; Fig. 8). The subzone has recently been recorded from Genetic Sequence 2 (approximately equivalent to the lower Kolmule Formation) on the Barents Shelf and from the southwestern Barents Sea by Grundvåg et al. (Reference Grundvåg, Marin, Kairanov, Śliwińska, Nøhr-Hansen, Escalona and Olaussen2017) and Marin et al. (Reference Marin, Escalona, Śliwińska, Nøhr-Hansen and Mordasova2017), respectively.
6.j. Subzone: Vesperopsis mayi (2) of Nøhr-Hansen (Reference Nøhr-Hansen1993)
Age: late Aptian. The age is revised and differs slightly from the latest early Aptian – late Aptian? age of Nøhr-Hansen (Reference Nøhr-Hansen1993), a consequence of the redating of the older Vesperopsis longicornis (1) Subzone.
Definition: From the FO of Vesperopsis mayi to the FO of Senoniasphaera microreticulata.
Stratigraphic events: FO of Vesperopsis mayi at the base and FO of Leptodinium cancellatum in the lower part of the subzone, whereas the FO of Canningia reticulata and the LO of Nyktericysta? vitrea occur in the upper part.
Correlations: Duxbury (Reference Duxbury2001) reported the FO of consistent L. cancellatum from his LKP32.2 Subzone, tentatively correlated with the middle part of the Leymeriella tardefurcata ammonite Zone (of earliest Albian age; Fig. 8), but mentions that the species can occur sporadically in his older, late Aptian LKP31 Zone. The study of 22 samples from the Vitskøl Elv section, Peary Land (Fig. 2) date the lower part of the section to possibly early–late Aptian, based on the presence of Vesperopsis mayi and Circulodinium brevispinosum indicating the presence of the Vesperopsis mayi Subzone (unpublished data). Recently, the subzone has been recorded from the middle part of Genetic Sequence 2 (approximately equivalent to the Kolmule Formation) in the southwestern Barents Sea by Marin et al. (Reference Marin, Escalona, Śliwińska, Nøhr-Hansen and Mordasova2017).
6.k. Subzone: Senoniasphaera microreticulata (3) of Nøhr-Hansen (Reference Nøhr-Hansen1993)
Age: late Aptian – Early Albian.
Definition: From the FO to the LO of Senoniasphaera microreticulata.
Stratigraphic events: FO of Cauca parva is in the lower part of the subzone, whereas the FO of Hapsocysta? benteae occurs in the upper part of the subzone.
Correlations: The study of 22 samples from the Vitskøl Elv section, Peary Land (Fig. 2) dates the upper part as late Aptian – Early Albian based on the FO of Senoniasphaera microreticulata indicating the presence of the Senoniasphaera microreticulata Subzone (unpublished data).
6.l. Subzone: Leptodinium? hyalodermopse (4) of Nøhr-Hansen (Reference Nøhr-Hansen1993)
Age: Early Albian.
Definition: From the LO of Senoniasphaera microreticulata to the LO of Circulodinium brevispinosum.
Stratigraphic events: FO of Aptea polymorpha and Odontochitina singhii occur in the middle part of the subzone, whereas the LO of Circulodinium brevispinosum, Leptodinium hyalodermopse and Vesperopsis longicornis occur at the top of the subzone.
Correlations: Duxbury (Reference Duxbury2001) recorded the FO of Odontochitina singhii from the base of his Lower Albian dinocyst LKP33 Subzone, tentatively correlated with the upper part of the Leymeriella tardefurcata ammonite Zone (of Early Albian age; Fig. 8).
6.m. Zone: Rhombodella paucispina (IV) of Nøhr-Hansen (Reference Nøhr-Hansen1993)
Age: middle Albian.
Definition: From the LO of Circulodinium brevispinosum to the FO of Subtilisphaera kalaallitii.
Comments: The zone was subdivided into the two subzones by Nøhr-Hansen (Reference Nøhr-Hansen1993): Litosphaeridium arundum (1) and Chichaouadinium vestitum (2). Studies of a section through the Rødelv Member from east Steensby Bjerg (LHN 080810-2, Fig. 4), northern part of Hold with Hope, have refined the dinocyst stratigraphy of the lower part of the Rhombodella paucispina (IV) Zone.
Correlations: The zone has previously been recorded from the Mesa Creek section, Ellesmere Island and the Junction Diapir section, Axel Heiberg Island, Arctic Canada (Nøhr-Hansen & McIntyre, Reference Nøhr-Hansen and McIntyre1998; Fig. 1). The Rhombodella paucispina (IV) Zone has been recorded from the Albian succession in Kangerlussuaq southern, East Greenland (Nøhr-Hansen, Reference Nøhr-Hansen2012; Fig. 1). The zone has recently been recorded from the lower part of Genetic Sequence 4 (approximately equivalent to the Kolmule Formation) from the Loppa High, southwestern Barents Sea by Marin et al. (Reference Marin, Escalona, Grundvåg, Nøhr-Hansen and Kairanova2018).
6.n. Subzone: Litosphaeridium arundum (1) of Nøhr-Hansen (Reference Nøhr-Hansen1993)
Age: middle Albian.
Definition: From the LO of Circulodinium brevispinosum to the FO of common specimens of Chichaouadinium vestitum.
Stratigraphic events: FO of Chichaouadinium vestitum, Litosphaeridium arundum, Pseudoceratium expolitum and Rhombodella paucispina at the base of the subzone followed by the FO of Batioladinium shaftesburiense in the middle part; Ovoidinium sp. 3 Nøhr-Hansen (Reference Nøhr-Hansen1993) is also present.
Correlations: The presence of the informal species Ovoidinium sp. 3 Nøhr-Hansen (Reference Nøhr-Hansen1993) seems to be a useful lower middle Albian marker in NE Greenland. It occurs in Rødelv Member at eastern Steensby Bjerg in the northern part of Hold with Hope, and was originally recorded from the lower part of the Litosphaeridium arundum (1) Subzone at Stratumbjerg, Wollaston Forland and at Kontaktravine, Clavering Ø (Nøhr-Hansen, Reference Nøhr-Hansen1993; section 21, 22, Fig. 5). Duxbury (Reference Duxbury2001) recorded the FO of L. arundum from the base of his Lower Albian dinocyst subzone LKP33 Subzone.
6.o. Subzone: Chichaouadinium vestitum (2) of Nøhr-Hansen (Reference Nøhr-Hansen1993)
Age: middle Albian.
Definition: From the FO of the acme of Chichaouadinium vestitum to the FO of Subtilisphaera kalaallitii.
Stratigraphic events: LO of Aptea polymorpha, Chichaouadinium vestitum, Ellipsoidictyum imperfectum, Leptodinium cancellatum and Odontochitina singhii occurs at the top of the subzone.
Correlations: Duxbury (Reference Duxbury2001) recorded an increase in abundance of E. imperfectum in the top of his dinocyst LKP34 Subzone upper middle Albian tentatively correlated with the Euhoplites lautus ammonite Zone and the LO of O. singhii in the dinocyst LKP35.1 Subzone , lowermost upper Albian, Mortoniceras inflatum ammonite Zone (Fig. 8). The subzone has recently been recorded from the upper part of Carolinefjellet Formation on Spitsbergen by Grundvåg et al. (Reference Grundvåg, Marin, Kairanov, Śliwińska, Nøhr-Hansen, Escalona and Olaussen2017) and from the older part of the Kangoq Ryg Member, Galadriel Fjeld Formation at Kilen, eastern North Greenland (Hovikoski et al. Reference Hovikoski, Pedersen, Alsen, Lauridsen, Svennevig, Nøhr-Hansen, Sheldon, Dybkjær, Bojesen-Koefoed, Piasecki, Bjerager and Ineson2018; Pedersen et al. Reference Pedersen, Lauridsen, Svennevig, Bojesen-Koefoed, Nøhr-Hansen and Alsen2018; Fig. 1).
6.p. Zone: Subtilisphaera kalaallitii (V) of Nøhr-Hansen (Reference Nøhr-Hansen1993) redefined
Age: late Albian – middle Cenomanian.
Definition: From the FO of Subtilisphaera kalaallitii to the FO of Endoceratium ludbrookiae.
Comments: Originally the top of the zone was defined at the LO of Epelidosphaeridia spinosa (Nøhr-Hansen, Reference Nøhr-Hansen1993); in the present study this is changed to the slightly higher FO of Endoceratium ludbrookiae, which seems to be a more reliable marker.
The original zone was subdivided into four subzones by Nøhr-Hansen (Reference Nøhr-Hansen1993): Wigginsiella grandstandica (1), Odontochitina ancala (2), Ovoidinium sp. 1 (3) and Epelidosphaeridia spinosa (4). The redefined Subtilisphaera kalaallitii Zone is subdivided into the following five subzones: Wigginsiella grandstandica (1), Odontochitina ancala (2), Sindridinium borealis (3) new, Epelidosphaeridia manifesta (4) new and Sindridinium anaanae (5) new.
Correlations: The zone has previously been recorded from the Junction Diapir section, Axel Heiberg Island, Arctic Canada (Nøhr-Hansen & McIntyre, Reference Nøhr-Hansen and McIntyre1998; Fig. 1). Recently, the Subtilisphaera kalaallitii (V) Zone Nøhr-Hansen (Reference Nøhr-Hansen1993) was recorded from the Albian–Cenomanian Sorgenfri Formation in Kangerlussuaq, southern East Greenland (Nøhr-Hansen, Reference Nøhr-Hansen2012; Fig. 6). The middle part of the Subtilisphaera kalaallitii Zone was reported from the Kolmule Formation in the southwestern Barents Sea and from the Langebarn Formation in the Norwegian Sea (Fig. 6) by Radmacher et al. (Reference Radmacher, Tyszka, Mangerud and Pearce2014) and by Radmacher et al. (Reference Radmacher, Mangerud and Tyszka2015), respectively. Recently, the lower part of the subzone has been questionably recorded from Genetic Sequence 4 (approximately equivalent to the Kolmule Formation) from the Loppa High, southwestern Barents Sea by Marin et al. (Reference Marin, Escalona, Grundvåg, Nøhr-Hansen and Kairanova2018).
6.q. Subzone: Wigginsiella grandstandica (1) of Nøhr-Hansen (Reference Nøhr-Hansen1993)
Age: early late Albian.
Definition: From the FO of Wigginsiella grandstandica to the FO of Odontochitina ancala.
Stratigraphic events: FO of Subtilisphaera kalaallitii, Wigginsiella grandstandica and Circulodinium sp. 1 Nøhr-Hansen (Reference Nøhr-Hansen1993) at the base of the subzone. Subtilisphaera kalaallitii and Rhombodella paucispina are common to dominant within the present subzone. The informal species Dinoflagellate cyst 1 Nøhr-Hansen (Reference Nøhr-Hansen1993) is present in a narrow interval within the lower part of the present subzone, and seems to be a good lowermost upper Albian marker in NE Greenland.
Correlations: Dinoflagellate cyst 1 Nøhr-Hansen (Reference Nøhr-Hansen1993) was originally recorded from the lower part of the Wigginsiella grandstandica (1) Subzone at Tværdal, Geographical Society Ø (Nøhr-Hansen, Reference Nøhr-Hansen1993; section 30, p. 162, Fig. 3). It also occurs together with W. grandstandica in the lower part of Fosdalen formation (Fig. 6) at Diener Bjerg, northern Hold with Hope (PAL-9/2009, Fig. 4) and in a more than 500 m thick, E-dipping succession in the river valley (HNH 080910-1, Fig. 4) of western Lygnaelv, southern Hold with Hope. Recently, the subzone has been recorded from the older part of the Kangoq Ryg Member, Galadriel Fjeld Formation at Kilen, eastern North Greenland (Hovikoski et al. Reference Hovikoski, Pedersen, Alsen, Lauridsen, Svennevig, Nøhr-Hansen, Sheldon, Dybkjær, Bojesen-Koefoed, Piasecki, Bjerager and Ineson2018; Pedersen et al. Reference Pedersen, Lauridsen, Svennevig, Bojesen-Koefoed, Nøhr-Hansen and Alsen2018; Fig. 1).
6.r. Subzone: Odontochitina ancala (2) of Nøhr-Hansen (Reference Nøhr-Hansen1993)
Age: middle–late Albian.
Definition: From the FO of Odontochitina ancala to the FO of Sindridinium borealis.
Comments: The Odontochitina ancala (2) Subzone is represented by a more than 200-m-thick succession at Svinhufvud Bjerge (section 37, Nøhr-Hansen, Reference Nøhr-Hansen1993; Fig. 3) on Traill Ø and less than 82-m-thick in Tværdal, Geographical Society Ø (section 30, Nøhr-Hansen, Reference Nøhr-Hansen1993; Fig. 3). Nøhr-Hansen (Reference Nøhr-Hansen1993, section 28; Fig. 4) dated two samples to middle–late Albian at the eastern mouth of Lygnaelv, southern Hold with Hope. The succession was referred to the Odontochitina ancala (2) Subzone Nøhr-Hansen (Reference Nøhr-Hansen1993) despite the lack of the zonal index species. Analyses of samples from this locality (HNH 080610-1&2, Fig. 4) confirmed the presence of Odontochitina ancala, together with the Lower Cenomanian zonal marker Sindridinium anaanae.
The Early Cenomanian age correlates with a latest Albian – Cenomanian age based on the recovery of Inoceramus dunveganensis McLean and the annelid Ditrupa almost at the same site (CASP Loc. W. 3141; Kelly et al. Reference Kelly, Whitham, Koraini and Price1998). The ammonite Schloenbachia ventiosa Stieler of probable Early Cenomanian age occurs further inland at Lygnaelv (Kelly et al. Reference Kelly, Whitham, Koraini and Price1998; CASP Loc. K.7359). Kelly et al. (Reference Kelly, Whitham, Koraini and Price1998) therefore regarded the Odontochitina ancala (2) Subzone of Nøhr-Hansen (Reference Nøhr-Hansen1993) as Cenomanian. However, palynological studies of two successions from this locality refer these successions to the new Epelidosphaeridia manifesta (4) Subzone of Early–middle Cenomanian age (see below).
Stratigraphic events: The FO of Epelidosphaeridia sp. 1 Nøhr-Hansen (Reference Nøhr-Hansen1993), Odontochitina ancala and Sindridinium? torulosa at the base of the subzone and LO of Circulodinium sp. 1 Nøhr-Hansen (Reference Nøhr-Hansen1993) in the lower part of the subzone. The FO of Endoceratium exquisitum in the middle of the subzone seems to be a good stratigraphic marker; the species was wrongly reported and illustrated as Endoceratium dettmanniae by Nøhr-Hansen et al. (Reference Nøhr-Hansen, Costa, Pearce and Alsen2018, pl. 9, figs 1–6). Circulodinium sp. 1 Nøhr-Hansen (Reference Nøhr-Hansen1993), Subtilisphaera kalaallitii and Rhombodella paucispina are common to dominant in this subzone.
Correlations: The Odontochitina ancala (2) Subzone was recently recorded from thin (less than 10 cm) mudstone layers within an approximately 5-m-thick sandy section and the approximately 40-m-thick overlying mudstone section situated at the northern part of Snertadal, Hold with Hope (HNH081210-6, Fig. 4). The section contains a low-diversity assemblage, including the brackish water form Nyktericysta dictyophora (former Quantouendinium dictyophorum according to Fensome et al. Reference Fensome, Nøhr-Hansen and Williams2016) previously recorded from the Odontochitina ancala Subzone at Svinhufvud Bjerge, Traill Ø (section 36, 37, Nøhr-Hansen, Reference Nøhr-Hansen1993; Figs 2, 3) as Vesperosis aff. V. fragilis. Recently, the subzone has been recorded from the younger part of the Kangoq Ryg Member, Galadriel Fjeld Formation at Kilen, eastern North Greenland (Hovikoski et al. Reference Hovikoski, Pedersen, Alsen, Lauridsen, Svennevig, Nøhr-Hansen, Sheldon, Dybkjær, Bojesen-Koefoed, Piasecki, Bjerager and Ineson2018; Pedersen et al. Reference Pedersen, Lauridsen, Svennevig, Bojesen-Koefoed, Nøhr-Hansen and Alsen2018).
6.s. Subzone: Sindridinium borealis (3) new
Age: latest Albian – Early Cenomanian.
Definition: From the FO of Sindridinium borealis to the FO of Epelidosphaeridia manifesta.
Comments: The Sindridinium borealis (3) Subzone corresponds to the lower part of the former Ovoidinium? sp. 1 Subzone of Nøhr-Hansen (Reference Nøhr-Hansen1993). Two morphologically almost similar forms of Ovoidinium sp. 1 Nøhr-Hansen (Reference Nøhr-Hansen1993) (non-cavate and hypocavate forms) were earlier assumed to occur together; however, Nøhr-Hansen et al. (Reference Nøhr-Hansen, Costa, Pearce and Alsen2018) recorded that S. borealis (the non-cavate form) has no or little overlap with the younger Sindidinium anaanae (the hypocavate form). The base of the up to 130-m-thick Sindridinium borealis (3) Subzone is recognized in four sections on Hold with Hope (Snertadal, JHOV-2/2009, east of Fosdalen HNH081410-4 and western Lygnaelv HNH 080910-1, HNH080610-3, Fig. 4) and from the Tværdal section, Geographical Society Ø (section 30 of Nøhr-Hansen Reference Nøhr-Hansen1993; PAL-3/2011, Nøhr-Hansen et al. Reference Nøhr-Hansen, Costa, Pearce and Alsen2018).
Stratigraphic events: FO of Epelidosphaeridia spinosa, Dinopoterygium alatum and Sindridinium borealis at the base of the subzone. LO of Epelidosphaeridia sp. 1 Nøhr-Hansen (Reference Nøhr-Hansen1993), FO of Senoniasphaera aff. microreticulata and FO of common S. borealis in the upper part of the subzone. Subtilisphaera kalaallitii and Rhombodella paucispina are common to dominant within the present subzone. The brackish to marginal marine indicator, the acritarch Limbicysta, is recorded from the upper part of the subzone at Lygnaelv, HNH080610-3.
Correlations: The Ovoidinium sp. 1 (3) Subzone Nøhr-Hansen (Reference Nøhr-Hansen1993) was recorded from the Albian Sorgenfri Formation in Kangerlussuaq, southern East Greenland (Nøhr-Hansen, Reference Nøhr-Hansen2012; Figs 1, 6). An acme of S. borealis was reported from the Komule Formation in the southwestern Barents Sea (Radmacher et al. Reference Radmacher, Tyszka, Mangerud and Pearce2014; Figs 1, 6). Sindridinium borealis also occurs in the Lower Cenomanian at Norfolk, onshore eastern England and in the Lower Cenomanian Kolmule Formation in Troms and Hammerfest basins, Barents Sea (Nøhr-Hansen et al. Reference Nøhr-Hansen, Costa, Pearce and Alsen2018; Fig. 1).
6.t. Subzone: Epelidosphaeridia manifesta (4) new
Age: Early Cenomanian.
Definition: From the FO Epelidosphaeridia manifesta to the FO of Sindridinium anaanae.
Comments: The Epelidosphaeridia manifesta (4) Subzone correlates with the middle part of the Ovoidinium? sp. 1 Subzone of Nøhr-Hansen (Reference Nøhr-Hansen1993). The subzone is represented by more than 100 m at Tværdal, Geographical Society Ø (section 30, Nøhr-Hansen, Reference Nøhr-Hansen1993; Fig. 3). Resampling and restudy of the approximately 415-m-thick succession in Tværdal on Geographical Society Ø (section 30 of Nøhr-Hansen, Reference Nøhr-Hansen1993; Pal-3/2011, Fig. 4) have yielded a rich assemblage of the ammonite Schloenbachia varians from 349 m, that is, a level in the upper part of the Epelidosphaeridia manifesta (4) Subzone indicating an earliest Cenomanian age (Mantelliceras mantelli – M. dixoni ammonite zones (undivided), Fig. 8; Nøhr-Hansen et al. Reference Nøhr-Hansen, Costa, Pearce and Alsen2018).
The subzone is also recorded from a more than 200-m-thick succession (HNH 081410-4, Fig. 4) in the hanging wall east of the Fosdalen Fault, east of Fosdalen, northern Hold with Hope.
The Epelidosphaeridia manifesta (4) Subzone has also been recorded in the uppermost part of an E-dipping succession along Lygnaelv (HNH 080610-3, Fig. 4), southern Hold with Hope. Here the ammonite Schloenbachia sp. is collected very close to Kelly et al.’s (Reference Kelly, Whitham, Koraini and Price1998) inland locality at Lygnaelv (CASP Loc. K.7359), where they found the ammonite Schloenbachia ventiosa that was probably of Early Cenomanian age. These records of Schloenbachia ammonites within the Epelidosphaeridia manifesta (4) Subzone both at Lygnaelv and in Tværdal provide an important palynostratigraphic means of placing the Albian–Cenomanian boundary in NE Greenland, and confirm that the boundary is in the Sindridinium borealis (3) Subzone and not in the underlying Odontochitina ancala (2) Subzone of Nøhr-Hansen (Reference Nøhr-Hansen1993) as suggested by Kelly et al. (Reference Kelly, Whitham, Koraini and Price1998).
Stratigraphic events: FO of Epelidosphaeridia manifesta and Ovoidinium epelidosphaeroides in the lower part of the subzone, both species are previously observed from the Lower Cenomanian Kolmule Formation in Troms and Hammerfest basins, Barents Sea (Nøhr-Hansen et al. Reference Nøhr-Hansen, Costa, Pearce and Alsen2018). The LO of E. manifesta occur in the middle of the subzone, whereas the FO of Xenascus aff. plotei and the LO of O. epelidosphaeroides, Senoniasphaera aff. microreticulata and common Sindridinium borealis occur in the uppermost part of the Subzone. Sindridinium borealis, Subtilisphaera kalaallitii and Rhombodella paucispina are common to dominant within the present subzone. The brackish to marginal marine indicator, the acritarch Limbicysta and the zygospore Tetrangulare conspicuum are recorded from the subzone east of Fosdalen (HNH081410-4) and the Tværdal sections (section 30 of Nøhr-Hansen Reference Nøhr-Hansen1993 and GEUS, PAL-3/2011).
6.u. Subzone: Sindridinium anaanae (5) new
Age: Early – ?middle Cenomanian.
Definition: From the FO of Sindridinium anaanae to the FO of Endoceratium ludbrookiae.
Comments: The Sindridinium anaanae (5) Subzone correlates with the upper part of the Ovoidinium? sp. 1 Subzone of Nøhr-Hansen (Reference Nøhr-Hansen1993). The subzone is represented by a total thickness of more than 190 m in three sections at the hanging wall east of the Fosdalen fault, east of Fosdalen, northern Hold with Hope (HNH 081410-4, Fig. 4, sections 24 and 25 of Nøhr-Hansen, Reference Nøhr-Hansen1993), overlying the older Epelidosphaeridia manifesta (4) Subzone, whereas the upper boundary has still to be recorded in a continuous exposed section.
Stratigraphic events: FO of Sindridinium anaanae and the LO of Xenascus aff. plotei at the lower part, whereas Rhombodella paucispina and Subtilisphaera kalaallitii are common to dominant within the present subzone. The brackish water to marginal marine indicator, the acritarch Limbicysta, is recorded from the lower part of the subzone at Fosdalen.
Correlations: Sindridinium anaanae are previously observed from the Lower Cenomanian Kolmule Formation in Troms and Hammerfest basins, Barents Sea (Nøhr-Hansen et al. Reference Nøhr-Hansen, Costa, Pearce and Alsen2018).
6.v. Zone: Endoceratium ludbrookiae (VI) new
Age: middle Cenomanian (pars)
Definition: From the FO of Endoceratium ludbrookiae to the FO of Isabelidinium magnum.
Comments: The Endoceratium ludbrookiae (VI) Zone has been recorded from three small sections: one 3-m- and two 25-m-thick successions south of Knudshoved at the east coast of Hold with Hope (HNH 081009-4, HNH 081009-2 and HNH 081009-1; Fig. 4). The incoming of E. ludbrookiae has not been observed in a continuous exposed section; in the Knudshoved sections the species occurs from the base of the exposures.
Stratigraphic events: FO of Endoceratium ludbrookiae, Ginginodinium aff. evittii and Palaeohystrichophora infusorioides together with the LO (or reworked specimens) of Endoceratium exquisitum at the base of the zone. Dorocysta litotes, Hapsocysta? benteae and Rhombodella paucispina are present and Subtilisphaera kalaalliti is abundant within this new zone.
Correlations: Endoceratium ludbrookiae has an upper Albian – upper Cenomanian range according to Helby et al. (Reference Helby, Morgan, Partridge and Jell1987) and a middle Lower – end upper Cenomanian range according to BioStrat (2018). The presence of G. aff. evittii may indicate an age not younger than Early Cenomanian according to Singh (Reference Singh1983); however, the absence of Epelidosphaeridia spinosa and Isabelidinium magnum may suggest a middle Cenomanian age. Based on the FO of Palaeohystrichophora infusorioides, this new E. ludbrookiae Zone may correlate with the lower part of the Cenomanian Palaeohystrichophora infusorioides – P. palaeoinfusa Interval Zone established from the intra-Early to intra-late Cenomanian part of the Kveite Formation from the southwestern Barents Sea by Radmacher et al. (Reference Radmacher, Tyszka, Mangerud and Pearce2014; Figs 1, 6) and later reported from the Langebarn and Blålange formations from the Norwegian Sea by Radmacher et al. (Reference Radmacher, Mangerud and Tyszka2015; Figs 1, 6). Recently, the FO of Endoceratium ludbrookiae has been recorded from the younger part of the Kangoq Ryg Member, Galadriel Fjeld Formation at Kilen, eastern North Greenland (Fig. 1), suggesting the presence of the subzone (Hovikoski et al. Reference Hovikoski, Pedersen, Alsen, Lauridsen, Svennevig, Nøhr-Hansen, Sheldon, Dybkjær, Bojesen-Koefoed, Piasecki, Bjerager and Ineson2018; Pedersen et al. Reference Pedersen, Lauridsen, Svennevig, Bojesen-Koefoed, Nøhr-Hansen and Alsen2018).
6.w. Zone: Isabelidinium magnum (VII) new
Age: ?middle–late Cenomanian.
Definition: From the FO of Isabelidinium magnum to the FO of Cyclonephelium compactum – Cauveridinium membraniphorumcomplex.
Comments: The Isabelidinium magnum (VII) Zone is recorded from two 15-m-thick successions (HNH080710-3 and HNH080710-4; Fig. 4) south of Knudshoved, and in the lowermost 5 m of the Nanok-1 core (517004) at the east coast of Hold with Hope.
Stratigraphic events: FO of Isabelidinium magnum, Isabelidinium acuminatum, Isabelidinium spp., Trithyrodinium aff. rhomboideum and Trithyrodinium suspectum at the base of the zone. LO of Hapsocysta? benteae is in the lower part and LO of Rhombodella paucispina and T. aff. rhomboideum are in the uppermost part of the zone. Subtilisphaera kalaalliti is abundant in the lower part and common in the upper part of the zone. Palaeohystrichophora infusorioides is common in the middle part of the zone and Isabelidinium spp. is common throughout the new zone.
Correlations: The FO of I. magnum suggests a late Cenomanian age (Costa & Davey, Reference Costa, Davey and Powell1992) and the FO of T. suspectum suggests a middle–late Cenomanian age (Costa & Davey, Reference Costa, Davey and Powell1992; Williams et al. Reference Williams, Brinkhuis, Pearce, Fensome, Weegink and Exon2004). Trithyrodinium rhomboideum is described from the middle Cenomanian Stage of Alberta, Canada (Singh, Reference Singh1983). The FO of Isabellidinium magnum and Trithyrodinium suspectum have recently been recorded from the older part of the Sølverbæk Formation at Kilen, eastern North Greenland (Fig. 1), suggesting the presence of the subzone (Hovikoski et al. Reference Hovikoski, Pedersen, Alsen, Lauridsen, Svennevig, Nøhr-Hansen, Sheldon, Dybkjær, Bojesen-Koefoed, Piasecki, Bjerager and Ineson2018; Pedersen et al. Reference Pedersen, Lauridsen, Svennevig, Bojesen-Koefoed, Nøhr-Hansen and Alsen2018).
6.x. Zone: Cyclonephelium compactum – Cauveridinium membraniphorum complex (VIII) new
Age: latest Cenomanian.
Definition: From the FO of Cyclonephelium compactum – Cauveridinium membraniphorum complex to the FO of Heterosphaeridium difficile.
Comments: The new Cyclonephelium compactum – Cauveridinium membraniphorum complex (VIII) Zone has been recorded from a 22-m-thick succession (HNH080710-2, Fig. 4) south of Knudshoved and represented by 37 m in the Nanok-1 core (517004) at the east coast of Hold with Hope. Cauveridinium membraniphorum was originally described as Cyclonephelium membraniphorum by Cookson & Eisenack (Reference Cookson and Eisenack1962, p. 495), and the Cyclonephelium compactum – Cauveridinium membraniphorum complex was reported as the Cyclonephelium compactum – C. membraniphorum complex until Fauconnier & Masure (Reference Fauconnier and Masure2004, p. 97) transferred the species Cyclonephelium membraniphorum into the genus Cauveridinium.
Stratigraphic events: The FO of Cyclonephelium compactum – Cauveridinium membraniphorum complex and Surculosphaeridium longifurcatum at the base of of the zone. Chlamydophorella nyei, Isabelidinium spp., Palaeohystrichophora infusorioides and Spiniferites spp. are common throughout the zone and S. longifurcatum are common in the upper part of the new zone. The last consistent occurrence of Subtilisphaera kalaalliti occurs in the middle part of the zone.
Correlations: The first common occurrence of C. compactum – C. membraniphorum complex is reported in upper Cenomanian onshore UK (Marshall & Batten, Reference Marshall and Batten1988; Dodsworth, Reference Dodsworth2000; Pearce et al. Reference Pearce, Jarvis and Tocher2009). Recently, Cauveridinium membraniphorum was recorded from an upper Cenomanian succession in Kangerlussuaq, southern East Greenland (Nøhr-Hansen, Reference Nøhr-Hansen2012; Fig. 1). Based on the FO of C. compactum – C. membraniphorum complex and S. longifurcatum, the base of the present new zone may correlate with the middle part of the Cenomanian P. infusorioides – P. palaeoinfusa Interval Zone established from the intra-Early to intra-late Cenomanian part of the Kveite Formation from the southwestern Barents Sea by Radmacher et al. (Reference Radmacher, Tyszka, Mangerud and Pearce2014; Figs 1, 6) and later reported from the Langebarn and Blålange formations from the Norwegian Sea by Radmacher et al. (Reference Radmacher, Mangerud and Tyszka2015; Figs 1, 6). Subtilisphaera kalaalliti becomes extinct in the uppermost part of their Palaeohystrichophora infusorioides – P. palaeoinfusa Interval Zone from the Norwegian Sea according to Radmacher et al. (Reference Radmacher, Mangerud and Tyszka2015). The last consistent occurrence of S. kalaalliti within the new C. compactum – C. membraniphorum complex Zone may indicate that its upper boundary is slightly younger than the Palaeohystrichophora infusorioides and P. palaeoinfusa Interval Zone of Radmacher et al. (Reference Radmacher, Tyszka, Mangerud and Pearce2014). Recently, the FO of Cauveridinium membraniphorum has been recorded from the older part of the Sølverbæk Formation at Kilen, eastern North Greenland, suggesting the presence of the subzone (Hovikoski et al. Reference Hovikoski, Pedersen, Alsen, Lauridsen, Svennevig, Nøhr-Hansen, Sheldon, Dybkjær, Bojesen-Koefoed, Piasecki, Bjerager and Ineson2018; Pedersen et al. Reference Pedersen, Lauridsen, Svennevig, Bojesen-Koefoed, Nøhr-Hansen and Alsen2018; Fig. 1).
6.y. Zone: Heterosphaeridium difficile (IX) new
Age: Early Turonian – ?middle Coniacian.
Definition: From the FO of Heterosphaeridium difficile to the FO of the undefined species informally named “Chatangiella spinosa” in industrial reports.
Comments: The Heterosphaeridium difficile (IX) Zone has been recorded from a 27-m-thick succession (HNH080209-4) and represented by 28 m in Fosdalen formation in the Nanok-1 core (517004) south of Knudshoved at the east coast of Hold with Hope (Fig. 4). The zone is subdivided into four new subzones: Chatangiella granulifera (1), Senoniasphaera rotundata (2), Odontochitina rhakodes (3) and Xenascus gochtii (4). The Heterosphaeridium difficile (IX) Zone differs from the informal Heterosphaeridium difficile interval of Coniacian – Early Santonian age described by Nøhr-Hansen (Reference Nøhr-Hansen1996) from West Greenland, which was defined from the LO of Arvalidinium scheii to the LO of Heterosphaeridium difficile.
Correlations: Parts of the informal Heterosphaeridium difficile interval of Nøhr-Hansen (Reference Nøhr-Hansen1996) were recently recorded from the Turonian–Coniacian succession in the Sorgenfri Formation at Kangerlussuaq, southern East Greenland (Nøhr-Hansen, Reference Nøhr-Hansen2012; Figs 1, 6) and from the Turonian – ?intra-early Coniacian part of the Kveite Formation in the southwestern Barents Sea (Radmacher et al. Reference Radmacher, Tyszka, Mangerud and Pearce2014) and from the Turonian – ?intra-early Coniacian part of the Blålange and Kvitnos formations in the Norwegian Sea (Radmacher et al. Reference Radmacher, Mangerud and Tyszka2015; Figs 1, 6). Recently, the FO of Heterosphaeridium difficile has been recorded from the older part of the Sølverbæk Formation at Kilen, eastern North Greenland, suggesting the presence of the subzone (Hovikoski et al. Reference Hovikoski, Pedersen, Alsen, Lauridsen, Svennevig, Nøhr-Hansen, Sheldon, Dybkjær, Bojesen-Koefoed, Piasecki, Bjerager and Ineson2018; Pedersen et al. Reference Pedersen, Lauridsen, Svennevig, Bojesen-Koefoed, Nøhr-Hansen and Alsen2018; Fig. 1).
6.z. Subzone: Chatangiella granulifera (1) new
Age: Early Turonian.
Definition: From the FO of Chatangiella granulifera to the FO of Senoniasphaera rotundata.
Comments: The Chatangiella granulifera (1) Subzone has been recorded from a 4-m-thick Fosdalen formation succession (HNH080209-4) south of Knudshoved and is represented by 6 m in the Nanok-1 core (517004) at the east coast of Hold with Hope (Fig. 4).
Stratigraphic events: The FO of Chatangiella granulifera and Heterosphaeridium difficile at the base of the subzone.
Correlations: Both C. granulifera and H. difficile have their FO at the base of the Turonian Stage according to Costa & Davey (Reference Costa, Davey and Powell1992). Pearce et al. (Reference Pearce, Jarvis, Swan, Murphy, Tocher and Edmunds2003), Williams et al. (Reference Williams, Brinkhuis, Pearce, Fensome, Weegink and Exon2004) and BioStrat (2018) suggest an age not older than Early Turonian for the FO of H. difficile. Dodsworth (Reference Dodsworth2000) recorded the FO of rare H. difficile from the Lower Turonian bed 95, 1.2 m above the global boundary stratotype section and point (GSSP) at the type section at Pueblo, Colorado, USA. Eldrett et al. (Reference Eldrett, Ma, Bergman, Lutz, Gregory, Dodsworth, Phipps, Hardas, Minisini, Ozkan, Ramezani, Bowring, Kamo, Ferguson, Macaulay and Kelly2015) recently dated the FO of H. difficile to middle Turonian (92.28 ma) from Texas, USA, based on astronomical calibration. An Early Turonian age for the FO of H. difficile is tentatively used in the present paper. Bell & Sellnes (1997) suggested an Early–middle Cenomanian FO age for H. difficile based on its co-occurrence with members of the Endoceratium dettmanniae – E. ludbrookiae plexus, offshore Norway. However, E. ludbrookiae has in the present study been recorded in assemblages together with H. difficile and the Turonian – middle Coniacian marker species Odontochitina rhakodes and Xenascus gochtii from Nanok-1 core (517004) at the east coast of Hold with Hope, and together with H. difficile and O. rhakodes from the middle – upper part of the Sølverbæk Formation at Kilen, eastern North Greenland (Hovikoski et al. Reference Hovikoski, Pedersen, Alsen, Lauridsen, Svennevig, Nøhr-Hansen, Sheldon, Dybkjær, Bojesen-Koefoed, Piasecki, Bjerager and Ineson2018; Pedersen et al. Reference Pedersen, Lauridsen, Svennevig, Bojesen-Koefoed, Nøhr-Hansen and Alsen2018; Fig. 1), suggesting either reworking or a Coniacian LO for E. ludbrookiae. Olde et al. (Reference Olde, Jarvis, Pearce, Uličný, Tocher, Trabucho-Alexandre and Gröcke2015) mention (based on Bell & Sellnes, Reference Bell and Sellnes1997; Bloch et al. Reference Bloch, Schröder-Adams, Leckie, Craig and McIntyre1999) the FO of H. difficile in the Cenomanian Stage in the high northern latitudes, suggests that H. difficile is a cold-water-tolerant species that migrated southwards with the predominant Late Cretaceous cooling; however, Lenniger et al. (Reference Lenniger, Nøhr-Hansen, Hills and Bjerrum2014, unpublished data) recorded its FO in the Lower Turonian Stage from Axel Heiberg Island, Artic Canada and from the Baffin Bay area.
6.aa. Subzone: Senoniasphaera rotundata (2) new
Age: middle Turonian.
Definition: From the FO of Senoniasphaera rotundata to the FO of Odontochitina rhakodes.
Comments: The Senoniasphaera rotundata (2) Subzone has been recorded from a 6-m-thick Fosdalen formation succession (HNH080209-4) south of Knudshoved, and is represented by 3 m in the Nanok-1 core (517004) at the east coast of Hold with Hope (Fig. 4).
Stratigraphic events: The FO of Senoniasphaera rotundata and Raphidodinium fucatum at the base of the subzone. Surculosphaeridium longifurcatum are common in the subzone.
Correlations: Senoniasphaera rotundata has a FO in the ?upper Turonian – lower Coniacian stages according to Costa & Davey (Reference Costa, Davey and Powell1992). However, an FO in the middle Turonian Stage and a first common occurrence in the uppermost Turonian Stage was reported by Pearce et al. (Reference Pearce, Jarvis, Swan, Murphy, Tocher and Edmunds2003). The FO of R. fucatum in the middle Turonian Stage is described by Costa & Davey (Reference Costa, Davey and Powell1992) and was also recorded from the North Sea area, Norwegian Sea and West Greenland by Radmacher et al. (Reference Radmacher, Mangerud and Tyszka2015), Pedersen & Nøhr-Hansen (Reference Pedersen and Nøhr-Hansen2014) and Nøhr-Hansen et al. (2016), respectively; an upper Coniacian FO was reported by Nøhr-Hansen (Reference Nøhr-Hansen2012) from the Sorgenfri Formation at Kangerlussuaq, southern East Greenland.
6.ab. Subzone: Odontochitina rhakodes (3) new
Age: ?middle Turonian – ?early Coniacian. The suggested age is tentative, based on the ages of the subzones below and above.
Definition: From the FO of Odontochitina rhakodes to the FO of Xenascus gochtii.
Comments: The Odontochitina rhakodes (3) Subzone has been recorded from a 12-m-thick Fosdalen formation succession (HNH080209-4) south of Knudshoved, and represented by 4 m in the Nanok-1 core (517004) at the east coast of Hold with Hope (Fig. 4).
Stratigraphic events: FO of Laciniadinium arcticum and Odontochitina rhakodes at the base of the subzone and LO of O. rhakodes at the top of the subzone. Palaeohystrichophora infusorioides and Spiniferites spp. and Surculosphaeridium longifurcatum are common in the subzone.
Correlations: A Lower Turonian LO for O. rhakodes was reported by Fensome et al. (Reference Fensome, Crux, Gard, MacRae, Williams, Thomas, Fiorini and Wach2008). The FO of Laciniadinium arcticum was reported as most likely in the lower Coniacian Stage from West Greenland by Nøhr-Hansen (Reference Nøhr-Hansen1996), whereas a Turonian FO was reported from Europe by Stover et al. (Reference Stover, Brinkhuis, Damassa, de Verteuil, Helby, Monteil, Partride, Powel, Riding, Smelror, Williams, Jansonius and Gregor1996). Recently, the FO of Odontochitina rhakodes has been recorded from the Sølverbæk Formation at Kilen eastern North Greenland, suggesting the presence of the subzone (Hovikoski et al. Reference Hovikoski, Pedersen, Alsen, Lauridsen, Svennevig, Nøhr-Hansen, Sheldon, Dybkjær, Bojesen-Koefoed, Piasecki, Bjerager and Ineson2018; Pedersen et al. Reference Pedersen, Lauridsen, Svennevig, Bojesen-Koefoed, Nøhr-Hansen and Alsen2018; Fig. 1).
6.ac. Subzone: Xenascus gochtii (4) new
Age: ?early–middle Coniacian.
Definition: From the FO of Xenascus gochtii to the FO of “Chatangiella spinosa” (undescribed species, informally named so in industrial reports).
Comments: The Xenascus gochtii (4) Subzone has been recorded from a 0.65-m-thick succession (HNH080209-4) from the uppermost part of Fosdalen formation underlying the Nanok Member (Kelly et al. Reference Kelly, Whitham, Koraini and Price1998) south of Knudshoved and from the uppermost 14 m of Fosdalen formation, in the Nanok-1 core (517004) at the east coast of Hold with Hope (Fig. 4). Kelly et al. (Reference Kelly, Whitham, Koraini and Price1998) mentioned that the records of inoceramids (e.g. Inoceramus lamarcki) near the top of the Fosdalen Member (referred to as Fosdalen formation here; Fig. 6) near Knudshoved indicate a late Turonian – possible Coniacian age.
Stratigraphic events: FO of Xenascus gochtii at the base of the subzone and LO of Stephodinium coronatum at its top. Palaeohystrichophora infusorioides is common to abundant in this subzone.
Correlations: An ?early–middle Coniacian age is suggested for the new Xenascus gochtii (4) Subzone based on the FO of X. gochtii that indicates an age not older than middle Coniacian (Prince et al. Reference Prince, Jarvis, Pearce and Tocher2008). The LO of S. coronatum indicates an age not younger than latest Turonian according to Costa & Davey (Reference Costa, Davey and Powell1992), whereas the middle Coniacian LO published by Williams et al. (Reference Williams, Brinkhuis, Pearce, Fensome, Weegink and Exon2004) is most likely based on misidentification (M. Pearce, pers. comm. 2019). In the present study, S. coronatum has been recorded in assemblages together with H. difficile and the Turonian – middle Coniacian marker species Odontochitina rhakodes and Xenascus gochtii from Nanok-1 core (517004) at the east coast of Hold with Hope. Recently, S. coronatum has been recorded together with H. difficile and X. gochtii from southern East Greenland (Nøhr-Hansen, Reference Nøhr-Hansen2012), and together with H. difficile and O. rhakodes from the middle to upper part of the Sølverbæk Formation at Kilen, eastern North Greenland (Hovikoski et al. Reference Hovikoski, Pedersen, Alsen, Lauridsen, Svennevig, Nøhr-Hansen, Sheldon, Dybkjær, Bojesen-Koefoed, Piasecki, Bjerager and Ineson2018; Pedersen et al. Reference Pedersen, Lauridsen, Svennevig, Bojesen-Koefoed, Nøhr-Hansen and Alsen2018; Fig. 1). These records may suggest either reworking or a Coniacian LO for S. coronatum. Based on the LO of S. coronatum, the top of the subzone may correlate with the top of the Turonian – ?intra-early Coniacian Heterosphaeridium difficile Interval Zone recorded from the Kveite Formation in the southwestern Barents Sea (Radmacher et al. Reference Radmacher, Tyszka, Mangerud and Pearce2014) and from the top of the Turonian – ?intra-early Coniacian part of the Blålange and Kvitnos formations in the Norwegian Sea (Radmacher et al. Reference Radmacher, Mangerud and Tyszka2015; Figs 1, 6). Recently, the FO of Xenascus gochtii has been recorded from the upper part of Sølverbæk Formation at Kilen, eastern North Greenland, suggesting the presence of the subzone (Hovikoski et al. Reference Hovikoski, Pedersen, Alsen, Lauridsen, Svennevig, Nøhr-Hansen, Sheldon, Dybkjær, Bojesen-Koefoed, Piasecki, Bjerager and Ineson2018; Pedersen et al. Reference Pedersen, Lauridsen, Svennevig, Bojesen-Koefoed, Nøhr-Hansen and Alsen2018; Fig. 1).
6.ad. Zone: “Chatangiella spinosa” (X) new
Age: middle Coniacian – middle Santonian.
Definition: From the FO of “Chatangiella spinosa” (informal name for an undefined species used in biostratigraphic reports within petroleum exploration drilling) to the FO of Alterbidinium ioannidesii.
Comments: The “Chatangiella spinosa” (X) Zone has been recorded from the lowermost 14.5 m of the Østersletten formation (including the Nanok Member, which forms the basal 2.5 m of the formation; Fig. 6) in the exposed section (HNH080209-4) south of Knudshoved, and from the lower 6 m of the Østersletten formation in the Nanok-1 core (517004, Fig. 4).
Stratigraphic events: FO of “Chatangiella spinosa”, Spinidinium echinoideum and Trithyrodinium vermiculatum at the base of this zone. LO of Senoniasphaera rotundata in the lower part and LO of Isabelidinium magnum and FO of Odontochitina porifera in the middle part of the zone. Palaeohystrichophora infusorioides and S. echinoideum are common in the subzone.
Correlations: The informal “C. spinosa” is assumed to have a middle Coniacian – middle Santonian range in the Norwegian Sea according to BioStrat (2018). Trithyrodinium vermiculatum was recorded from the middle Coniacian strata by Schiøler (Reference Schiøler1992). The FO of O. porifera may support a Santonian age. O. porifera has a Lower Santonian – lower Campanian range in Australia according to Helby et al. (Reference Helby, Morgan, Partridge and Jell1987), whereas Fensome et al. (Reference Fensome, Crux, Gard, MacRae, Williams, Thomas, Fiorini and Wach2008) and Fensome et al. (Reference Fensome, Nøhr-Hansen and Williams2016) recorded an LO of O. porifera in the upper Santonian deposits in the Scotian Margin and Labrador Sea, respectively. The zone may correlate with the lower part of the ?intra-early Coniacian – late Santonian Dinopterygium alatum Interval Zone in the Kveite Formation in the southwestern Barents Sea (Radmacher et al. Reference Radmacher, Tyszka, Mangerud and Pearce2014). Radmacher et al. (Reference Radmacher, Mangerud and Tyszka2015) reported abundant occurrence of T. vermiculatum, and abundant occurences of “C. spinosa” at the base of the D. alatum Interval Zone from the Kvitnos and Nise Formations from the Norwegian Sea (Figs 1, 6).
6.ae. Zone: Alterbidinium ioannidesii (XI) new
Age: late Santonian.
Definition: From the FO of Alterbidinium ioannidesii to the FO of Aquilapollenites spp.
Comments: The Alterbidinium ioannidesii (XI) Zone is recorded from the 26-m-thick succession representing the middle and upper part of the Østersletten formation at locality 5 south of Knudshoved and represented by 32 m in the Nanok-1 core (517004) at the east coast of Hold with Hope (Fig. 4), from a 20-m-thick succession at northeastern Hold with Hope (HNH081610-1, Fig. 4), from an approximately 100-m-thick succession on southern Jackson Ø (HNH081610-2, 3, Fig. 4) and from the upper 23 m of the 80-m-thick Kista Ø formation type section (Pal-5/2011, Figs 3, 6) eastern Månedal, Trail Ø.
Stratigraphic events: FO of Alterbidinium ioannidesii at the base of this zone and LO of “Chatangiella spinosa” and Heterosphaeridium difficile in the middle part of this zone.
Correlations: A. ioannidesii has a stratigraphical range from the ?upper Santonian – middle–lower Campanian strata in the Norwegian Sea (Pearce, Reference Pearce2010). “Chatangiella spinosa” has a top Early Santonian LO according to BioStrat (2018), whereas other consultants mention a middle Santonian LO. The LO of H. difficile indicates an age no younger than late Santonian. Heterosphaeridium difficile is often very abundant in Coniacian – Lower Santonian deposits in the North Sea, but isolated specimens are recorded from the upper Santonian onshore England (Prince et al. Reference Prince, Jarvis and Tocher1999).
6.af. Zone: Aquilapollenites (XII) new
Age: early–late Campanian.
Definition: From the FO of the pollen genus Aquilapollenites to the FO Cerodinium diebelii.
Comments: The lower part of the zone is subdivided into two new subzones: Aquilapollenites (1) and Isabelidinium microarmum (2). The Aquilapollenites Zone (XII) differs from the lower–middle Campanian informal Aquilapollenites interval of Nøhr-Hansen (Reference Nøhr-Hansen1996) by defining the upper boundary at the FO of Cerodinium diebelii instead at the LO of Isabelidinium microarmum. The upper boundary of the Aquilapollenites Zone has not been recognized in a continuous exposed succession in NE Greenland.
The Aquilapollenites Zone has been recorded from a 12-m-thick succession (locality 5) representing the uppermost 5 m of the Østersletten formation and the lowermost 7 m of the Knudshoved formation (Fig. 6) south of Knudshoved (east coast of Hold with Hope, Fig. 4); it is represented by 37 m in the nearby Nanok-1 core (517004) and from three sections at Geographical Society Ø, namely, a 65-m- and 20-m-thick succession at Hundeklemmen (PAL-6/2011, PAL-7/2011, Fig. 3) and a 45-m-thick succession west of Leitch Bjerg (PAL-8/2011, Fig. 3).
6.ag. Subzone: Aquilapollenites (1) new
Age: early Campanian.
Definition: From the FO of the pollen genus Aquilapollenites to the FO Isabelidinium microarmum.
Comments: The lower part of the Aquilapollenites Subzone has been recorded from a 12-m-thick succession (locality 5) representing the uppermost 5 m of the Østersletten formation and the lowermost 7 m of the Knudshoved formation south at Knudshoved, represented by 37 m in the Nanok-1 core (517004) at the east coast of Hold with Hope (Fig. 4). The Aquilapollenites Subzone (1) differs from the lower–middle Campanian informal Aquilapollenites interval of Nøhr-Hansen (Reference Nøhr-Hansen1996) by defining the upper boundary at the FO of Isabelidinium microarmum instead of at the LO of I. microarmum.
Stratigraphic events: FO of the pollen genera Aquilapollenites spp. and Chatangiella bondarenkoi. The presence of Alterbidinium ioannidesii, Odontochitina operculata and Trithyrodinium suspectum throughout the Knudshoved formation at locality 5 (Fig. 4) and the presence of Fromea nicosia in the middle part of the formation suggest an age not younger than early Campanian. The diverse assemblages from the lower part of the Knudshoved formation contain common Chatangiella spp., Palaeohystrichophora infusorioides, Palaeoperidinium pyrophorum, Spiniferites spp. and Trithyrodinium suspectum.
Correlations: Aquilapollenites has its first occurrence in the lower Campanian Stage (Nøhr-Hansen, Reference Nøhr-Hansen1996). The presence of the pollen Azonia fabacea in the lower part of the zone may support an early Campanian age. The species is representative of the lower Campanian deposits in Yukon, Canada (Nichols & Sweet, Reference Nichols, Sweet, Caldwell and Kauffman1993). Chatangiella bondarenkoi occurs in the middle Campanian Stage in West Greenland (Nøhr-Hansen, Reference Nøhr-Hansen1996), but in the Santonian–Campanian stages of the Canadian Arctic (Lentin & Vozzhennikova, Reference Lentin and Vozzhennikova1990). C. bondarenkoi is typical of the lower Campanian Stage in the Barents Sea (Radmacher et al. Reference Radmacher, Tyszka, Mangerud and Pearce2014). The present subzone may correlate with the early Campanian Palaeoglenodinium cretaceum Interval Zone from the Kveite Formation from the Barents Sea (Radmacher et al. Reference Radmacher, Tyszka, Mangerud and Pearce2014), and from the early Campanian Nise Formation from the Norwegians Sea (Radmacher et al. Reference Radmacher, Mangerud and Tyszka2015; Figs 1, 6). Based on the presence of radially ribbed sphenoceramid bivalves and the absence of the pollen genus Aquilapollenites, the former Knudshoved Member (referred to as Knudshoved formation here) was considered as late Santonian in age by Kelly et al. (Reference Kelly, Whitham, Koraini and Price1998). The present study suggests an early Campanian age for the lower studied part of the Knudshoved formation at Knudshoved.
6.ah. Subzone Isabelidinium microarmum (2) new
Age: early–middle Campanian.
Definition: From the FO to the LO of Isabelidinium microarmum.
Comments: The lower part of the Isabelidinium microarmum Subzone is recorded in the uppermost part of the Knudshoved formation in the Nanok-1 core (517004) at the east coast of Hold with Hope (Fig. 4) and in three sections at Geographical Society Ø, namely, a 65-m- and a 20-m-thick succession at Hundeklemmen (PAL-6/2011, PAL-7/2011, Fig. 3) and a 45-m-thick succession west of Leitch Bjerg (PAL-8/2011, Fig. 3). The outgoing of I. microarmum has not been observed in a continuous exposed section; the species occurs at the top of the studied exposures in NE Greenland.
Stratigraphic events: FO and LO of Isabelidinium microarmum, presence of Alterbidinium ioannidesii.
Correlations: The presence of Alterbidinium ioannidesii throughout the Knudshoved formation at Geographical Society Ø suggests an age not younger than early Campanian according to Pearce (Reference Pearce2010), who recorded a range up to the middle–lower Campanian in the Norwegian Sea and a range from the lower part of the echinoid Offaster pillula Zone (low lower Campanian) to the lower part of the belemnite Gonioteutus quadrata Zone (middle lower Campanian) at Norfolk, United Kingdom (Fig. 1). The present record of A. ioannidesii below and above the ammonite Scaphites ikorfatensis in the Hundeklemmen section, Geographical Society Ø (PAL-7/2011, Fig. 3) may indicate an extention of the range of A. ioannidesii into the upper middle Campanian Bostrychoceras polyplocum ammonite Zone (according to Kaplan et al. Reference Kaplan, Kennedy and Hiss2005, p. 152). The FO of I. microarmum at the base of the zone (uppermost part of the Nanok-1 core: 517004, at Knudshoved), and the presence of the species in all the three sections at Geographical Society Ø, may suggest that these sections are younger than the type locality of the Knudshoved formation (locality 5). Isabelidinium microarmum was described from the lower Campanian – Maastrichtian in northwestern Canada by McIntyre (Reference McIntyre1975), and recorded from the lower–middle Campanian Stage in West Greenland (Nøhr-Hansen, Reference Nøhr-Hansen1996) and from the lower Campanian Stage in northern Siberia (Lebedeva, Reference Lebedeva2006). In a palynological zonation scheme of the northern North Sea and Norwegian Sea, BioStrat (2018) shows a lower middle Campanian LO for A. ioannidesii and I. microarmum. The new subzone may correlate with the intra-Campanian Hystrichosphaeridium dowlingii – Heterosphaeridium spp. Interval Zone from Nise Formation in the Norwegian Sea (Radmacher et al. Reference Radmacher, Mangerud and Tyszka2015; Figs 1, 6).
6.ai. Zone: Cerodinium diebelii (XIII) new
Age: ?late Campanian – Early Maastrichtian.
Definition: From the FO of Cerodinium diebelii to the FO of the pollen Wodehouseia spinata.
Comments: The Cerodinium diebelii Zone has been recorded from a 25-m-thick succession on the south side of Haredal, Wollaston Forland (HNH081508-4, Fig. 3), and the lowermost part of the zone may be represented in an 80-m-thick succession east of Jacksontoppen, Jackson Ø (HNH081610-4, Fig. 4). The presence of C. diebelii has not been observed in a continuous exposed section, and the species occurs from the base of the exposures in NE Greenland. The FO of the pollen Wodehouseia spinata has not been observed in the studied section.
Stratigraphic events: FO of Cerodinium diebelii in the Jackson Ø section. All palynomorphs from this 80-m-thick succession are thermally overmature (thermal alteration index, TAI 3+ to 4−). C. diebelii, Hystrichosphaeridium tubiferum and Odontochitina operculata and the pollen Aquilapollenites spp. are present and Palaeperidinium pyrophorum is common, whereas the pollen genus Wodehouseia was not recorded in the very poorly preserved material.
The FO of Alterbidinium biaperturum, C. diebelii and Wodehouseia gracile are recorded from the base of the Haredal section followed by the FO of Hystrichosphaeridium sp. 3 McIntyre (Reference McIntyre1974). Phelodinium kozlowskii and Spongodinium delitiense are present throughout and A. biaperturum is common in the upper part of the Haredal section.
Correlations: McIntyre (Reference McIntyre1974, Reference McIntyre1975) recorded an Early Maastrichtian FO age for C. diebelii in the Northwest Territories of Canada and a late Campanian – Early Maastrichtian age for W. gracile, his Hystrichosphaeridium sp. 3 and Deflandrea biapertura (now Alterbidinium biaperturum, according to Fensome et al. Reference Fensome, Nøhr-Hansen and Williams2016). BioStrat (2018) recorded the FO of Hystrichosphaeridium sp. 3 McIntyre (Reference McIntyre1974) and the first commom occurrences of A. biaperturum and C. diebelii from the Early Maastrichtian Age in the northern North Sea and in the Norwegian Sea. A late Campanian FO for W. gracile is recorded in southern Alberta, Canada (Braman, Reference Braman2018). The Cerodinium diebelii Zone (XII) differs slightly from the Early Maastrichtian informal Cerodinium diebelii interval of Nøhr-Hansen (Reference Nøhr-Hansen1996) by its content of Wodehousia species, and the questionable extension into the latest Campanian Age. The informal C. diebelii interval of Nøhr-Hansen (Reference Nøhr-Hansen1996) was also reported by Radmacher et al. (Reference Radmacher, Tyszka, Mangerud and Pearce2014) from the Lower Maastrichtian part of the Kveite Formation in the Barents Sea. The present zone partly correlates with the interpreted Lower Maastrichtian Cerodinium diebelii interval recorded from southern East Greenland (Nøhr-Hansen, Reference Nøhr-Hansen2012). The Haredal section has previously been referred to an early to middle Campanian age based on the presence of the dinocysts Alterbidinium ioannidesii (one specimen only) and C. diebelii (Nøhr-Hansen et al. Reference Nøhr-Hansen, Nielsen, Sheldon, Hovikoski and Alsen2011). Re-examinations of the palynological material from Haredal revealed the presence of the pollen Wodehouseia gracile in all six studied samples, whereas the presence of one specimen of the Santonian – middle Campanian marker species A. ioannidesii in the uppermost sample is considered as reworked.
Discussion of the late Campanian and/or Early Maastrichtian age: Schiøler & Wilson (Reference Schiøler, Wilson and Odin2001) recorded the FO of C. diebelii, followed by a younger LO of the genus Odontochitina, within the uppermost Campanian in the Campanian–Maastrichtian stratotype at Tercis les Bains, France. The absence of the pollen genus Wodehouseia and the co-occurrence of C. diebelii and O. operculata may suggest a late Campanian age for the Jackson Ø section. Likewise, the FO A. biaperturum and W. gracile in the lower part of the Haredal may also indicate a late Campanian age, whereas the common occurrence of A. biaperturum and the FO of Hystrichosphaeridium sp. 3 McIntyre (Reference McIntyre1974) (=Rigaudella apenninica according to M. Pearce, pers. comm. 2019) may advocate for an Early Maastrichtian age for the upper part of the Haredal section.
7. Upper Maastrichtian
Records of late Maastrichtian palynomorphs in NE Greenland have been restricted to reworked material deposited in Palaeogene successions from Langsiden, Hold with Hope and Haredal, Wollaston Forland (Nøhr-Hansen et al. Reference Nøhr-Hansen, Nielsen, Sheldon, Hovikoski and Alsen2011; Fig. 5). The late Maastrichtian pollen marker species Wodehouseia spinata has not been observed in situ in NE Greenland, but is reported from upper Maastrichtian successions in southern East Greenland (Nøhr-Hansen, Reference Nøhr-Hansen2012) and in central West Greenland (Nøhr-Hansen, Reference Nøhr-Hansen1996; Pedersen & Nøhr-Hansen, Reference Pedersen and Nøhr-Hansen2014).
8. Conclusion
The palynostratigraphic zonation presented here is the first to cover the entire Cretaceous succession in East and NE Greenland. The zonation is correlated with other areas from where stratigraphic data are available.
The biostratigraphy is primarily based on dinocysts, which are present in all sections with marine deposits except when the sediments are severely thermally affected (e.g. by intrusions or subsidence).
The Cretaceous succession is divided into 15 palynozones. Six zones are subdivided into 20 subzones. More than 100 palynostratigraphic events representing more than 70 stratigraphic levels are recorded. The average age of zones is approximately 5 ma. Two new zones are erected in the lowermost Cretaceous succession, and the stratigraphy for the remaining Lower Cretaceous is based on the zonation by Nøhr-Hansen (Reference Nøhr-Hansen1993) in an updated and revised version. Eight Upper Cretaceous palynozones are all new. Several of these Upper Cretaceous stratigraphic units are also recognized in the Kangerlussuaq area, SW Greenland by Nøhr-Hansen (Reference Nøhr-Hansen2012). The Upper Cretaceous zonation partly correlates with the informal intervals identified from central West Greenland (Nøhr-Hansen, Reference Nøhr-Hansen1996) and with the zonations from the Barents and Norwegian seas (Radmacher et al. Reference Radmacher, Tyszka, Mangerud and Pearce2014, Reference Radmacher, Mangerud and Tyszka2015).
Dinocyst stratigraphy is the principal means of biostratigraphic dating of the Cretaceous deposits in NE Greenland. Cretaceous ammonite stratigraphy is incomplete in the area due to the occurrence of barren intervals. When ammonites are present, they are used for calibration of the palynostratigraphic units.
Acknowledgements
The present work is based on fieldwork and sample collections by several of our colleagues in GEUS during field seasons in east and NE Greenland, especially Morten Bjerager, Jørgen Bojesen-Koefoed, Michael B.W. Fyhn, Jussi Hovikoski, Jon Ineson, Lars Henrik Nielsen and Henrik Vosgerau. We are grateful to Charlotte Olsen, Annette Ryge and Dorthe Samuelsen (GEUS) who carefully prepared the samples in the Stratigraphic Laboratorium at GEUS and to Jette Halskov and John Robert Hopper (GEUS) who produced the figures. We thank Dr Bas Van de Schootbrugge, the editor of Geological Magazine and the two referees (Dr Martin Pearce and one anonymous) for their very valuable comments and suggestions. The paper is published with permission of the Geological Survey of Denmark and Greenland.