1. Introduction
Greenhouse gas emissions from agriculture are an important environmental impact to consider. Plant and livestock production currently account for 13% of global greenhouse gas (GHG) emissions (EPA, 2020). Fertilizer use, tillage, and irrigation contribute to carbon dioxide (CO2), nitrous oxide (N2O), and methane (CH4) emissions (Tilman et al., Reference Tilman, Fargione, Wolff, D’Antonio, Dobson, Howarth, Schindler, Schlesinger, Simberloff and Swackhamer2001), yet are necessary to support continued cultivation of soils. Humans convert new land for agriculture because of issues with soil fertility and climate change (Galford et al., Reference Galford, Melillo, Kicklighter, Cronin, Cerri, Mustard and Cerri2010). Such changes in land cover and land-use can make up 24 to 49% of the GHG budget associated with agricultural land management (Galford et al., Reference Galford, Melillo, Kicklighter, Cronin, Cerri, Mustard and Cerri2010). While extensification, the cultivation of new land, is one way to maintain or increase production, intensification, or increasing inputs per area, can support continued cultivation of land (Pellegrini & Fernandez, Pellegrini & Fernández, Reference Pellegrini and Fernández2018). Farm management practices, such as the use of cover crops and conservation tillage can improve soil fertility allowing for continued cultivation and possibly reduce GHG emissions (Kallenbach et al., Reference Kallenbach, Rolston and Horwath2010; Snyder et al., Reference Snyder, Bruulsema, Jensen and Fixen2009; Tully & Ryals, Reference Tully and Ryals2017). Considering agriculture is a significant contributor to GHGs, there is a need to understand how land cultivation and farm management practices impact GHG emissions from agricultural soils.
2. Objective
The objective of this study was to compare soil characteristics and GHG emissions between newly converted and historically cultivated subtropical agricultural soils. GHG measurements from soils located at the University of Florida Institute of Food and Agricultural Sciences Tropical Research and Education Center in Homestead, Florida, USA are relevant for future large-scale research in agroecosystem management in south Florida and other regions with similar calcareous soil type and agricultural management. More broadly, information on GHG emissions associated with cultivation status and history of agricultural soils can help quantify future GHG emissions associated farm management.
3. Methods
Krome gravelly loam soils (Nobel et al., Reference Nobel, Drew and Slabaugh1996), were sampled once in May, July and August 2018 for GHG emissions and soil moisture in four 20m x 20m plots. Two plots were historically cultivated for vegetable production (> 20 years). The other two plots were covered in turf and scattered trees until the top 0.30m of oolithic limestone rock was crushed and plowed in March 2018. Plots used in this study were neither fertilized nor irrigated but divided into 36 sub-plots and planted with multiple cover crops. Vented static flux chambers (Holland et al., Reference Holland, Robertson, Greenberg, Groffman, Boone and Gosz1999) were randomly installed at 4 sub-plots in each of the 4 larger plots at a depth of 2-3 cm. Headspace samples (20 mL) were taken 0, 15, 30, and 45 minutes after chambers were capped and analyzed for CO2, N2O, and CH4, using a gas chromatograph with ECD and FID detectors. Fluxes were determined from the four time points using linear regressions fitted to the changes in concentration over time when the regression line had an R2 > 0.65. Our minimal detectable flux for CO2, CH4, and N2O was 1.98, 1.70, and 2.04 g ha‒1 day‒1, respectively. N2O and CH4 were converted to carbon dioxide equivalents (CO2 eq.) using conversion factors 298 and 25, respectively. Soil samples (n=36) were collected from each sub-plot of the four plots at 10 cm depth and analyzed for organic matter (OM), extractable ammonium (NH4+) and nitrate (NO3‒) in March 2018. Soil porosity and bulk density were collected from all sub-plots in one cultivated and one converted plot. Mixed effects models were used to compare GHG fluxes and soil properties between cultivation history.
4. Results
A majority of the CH4 (37/48) and N2O (40/48) fluxes were below the detection limit; detectable fluxes (CH4 (10/11) and N2O (8/8)) were from newly converted soils. The average flux for CH4 and N2O was 0.0013 and 0.0036 kg ha‒1 day‒1, respectively, while the average CO2 flux was 12.66 kg ha‒1 day‒1. In CO2 eq, CH4 and N2O accounted for 1.5% of the overall CO2 emission. CO2 eq were 73% higher in newly converted soils compared to cultivated soils (Figure 1). Soil OM and soil porosity were significantly higher in newly converted soils compared to cultivated lands (Table 1). Cultivation decreased soil moisture compared to converted soils (Table 1). Bulk density was lower in newly converted soils than cultivated soils. Soil NH4+ and NO3‒ content were approximately 2.5 times and 8 times higher in the converted soils, respectively.
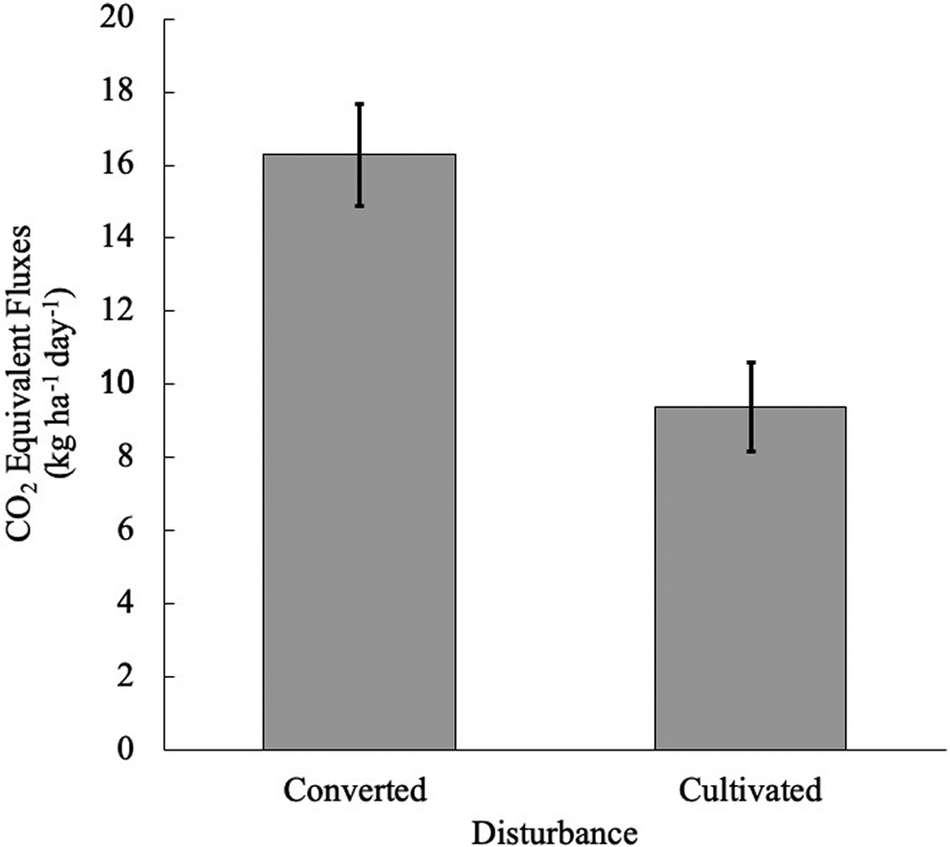
Figure 1. Mean (±SE) CO2 Equivalent fluxes (sum of CO2 and non-CO2 fluxes) from converted and cultivated soils (F1,2 = 13.82 p = 0.065). Fluxes were measured three times over 4 months during the summer rainy season.
Table 1. Summary table of results for soil physical and chemical properties (± SE). Different letters indicate mean values are significantly different (p < 0.05).

5. Discussion
Land conversion to agriculture in subtropical calcareous soils had higher GHG emission shortly after conversion than soils historically used for subtropical agriculture. Given the soil type and surface bedrock, we were unable to have an undisturbed control plot. Higher nitrogen content and soil moisture, as found in the newly converted soils, are both driving factors for GHG production in soils (Snyder et al., Reference Snyder, Bruulsema, Jensen and Fixen2009). Lower porosity and higher bulk density in the cultivated soils might reflect the frequent tillage, compaction, and loss of organic matter, compared to the newly converted soils, which were only tilled once prior to the experiment. The recently converted soils had higher OM, NO3‒, and NH4+ content, which is more desirable for agriculture in the short term.
6. Conclusions
Conversion of land for cultivation breaks up the structure of undisturbed soils and disrupts natural biogeochemical cycles. Soil structure and nutrient availability in converted lands may be desirable, at least in the short term despite the increase in GHG emissions. Farm management practices that facilitate nutrient storage (i.e., cover crops, conservation tillage) could allow for continued cultivation of lands and help reduce GHG emissions from agricultural soils (Galford et al., Reference Galford, Melillo, Kicklighter, Cronin, Cerri, Mustard and Cerri2010; Tully & Ryals, Reference Tully and Ryals2017). Our results suggest that GHG emissions may decrease overtime as subtropical land is continuously cultivated and soil OM and porosity decrease. Additional studies that assess the indirect as well as direct sources of GHG emissions from subtropical agriculture are needed to accurately identify farm management practices that can decrease the GHG costs of agriculture (Gelfand & Robertson, Reference Gelfand, Robertson, Hamilton, Doll and Robertson2015).
Acknowledgements
We thank Dr. Willm Martens-Habbena for help with GHG sample analysis. Asher Williams, Ariel Freidenreich, Dr. Jayachandran Krishnaswamy, Nicole Picon and Daniel Calzadilla provided field assistance and equipment.
Author Contributions
TF, ZB and ARS conceived and designed the study. TF conducted data collection. TF and KH performed the statistical analysis. All authors contributed to writing the article.
Funding Information
This research was funded through the USDA, National Institute of Food and Agriculture, Hatch project #FLA-TRC-005661 and #FLA-TRC-005764, and an Early Career Seed Grant from the UF/IFAS Office of Research to ZB.
Conflict of Interest
The authors have no conflicts to declare.
Data Availability Statement
The data that support these findings are openly available upon request to the corresponding author.
Comments
Comments to the Author: Interesting paper that found greater soil GHG emissions on newly vs. historically cultivated land in FL. This is important work due to threats to loss of habitat and biodiversity due to land conversion, and the need for accurate accounting of human GHG emissions. My comments below are in allyship of this work!
I recommend mentioning the context of ag intensification v. extensification (e.g. https://www.pnas.org/content/115/10/2335).
The statistics could be considered pseudoreplication (see https://doi.org/10.2307/1942661). I suggest including additional testing of the data to demonstrate statistical independence and show the correct calculation of the F-ratio.
Soil structure is surprising, I would expect the cultivated plots to have higher bulk density and lower porosity because of the erosion of soil aggregates, meaning soil particles fill pore spaces, and compaction. The newly converted soils having more soil moisture is expected with 2x the SOM. In line 89 moisture didn’t really double, it’s that the cultivated lands were halved by human impacts. In line 53 I suggest making humans the ones doing the conversion of land, rather than the passive voice insinuation of humans.
Line 48: demand for food is driven by increasing levels of consumption as well as population; also hunger is not just a product of not enough food, but the systems by which people access food. (FYI, in general, tying environmental damage to human population growth, insinuates mainly in nations with majority people of color, is problematic.)
Does “scarification” mean plowed? How deep? Were the new fields planted into anything, was N fertilizer used?
The paper does not include any caveats. Before we can conclude that new cultivation has less GHG emissions than historically cultivated land, we need the net GHG impact (e.g., see https://lter.kbs.msu.edu/citations/3465/download/Gelfand-2015-Ecology-Agric-Landscapes.pdf), I recommend adding a statement about this as a next step.
Thank you for the opportunity to review your work.