Social media summary: Gibbons and siamangs – the so-called ‘lesser apes’ – move on two legs a lot, and that might give us some clues into the origins of bipedal locomotion in human ancestors.
Introduction
Early twentieth-century physical anthropologists and anatomists had difficulty identifying the ape body plan from which the earliest human ancestors evolved. Keith (Reference Keith1923) identified homologous characters in living hominoids to posit an upright, orthograde ancestor that passed through a large-bodied, ‘Troglodytian’ phase. Gregory (Reference Gregory1928) also envisioned an orthograde ancestor but hypothesised that bipedalism evolved from a gibbon-like brachiator as was suggested later by Avis (Reference Avis1962). Morton (Reference Morton1924) imagined a more generalised, small-bodied, quadrumanous ape capable of arboreal bipedal locomotion, although he also wrote of humans passing through a terrestrial ‘gorilloid’ phase. Straus (Reference Straus1949) diverged from this line of thinking and instead hypothesised that the earliest human ancestors descended from a primate that was more cercopithecoid-like – an above-branch, pronograde, arboreal quadruped (Figure 1).

Figure 1. Evolution of bipedalism. The body form from which bipedalism evolved remains unknown. Scholars have proposed models based on (counterclockwise from top) brachiating hylobatids, pronograde monkeys, knuckle-walking African apes or quadrumanous orangutans. These models have deep roots in the anthropological literature and continue to be debated today. Figure based on Richmond et al. (Reference Richmond, Begun and Strait2001), redrawn using PhyloPics Creative Commons Attribution-ShareAlike 3.0 Unported licence (https://creativecommons.org/licenses/by/3.0/), courtesy of Gareth Monger, T. Michael Keesey and Nobu Tamura.
The molecular revolution provided much needed clarity of our place in the primate family tree. Given that humans were more closely related to the African apes than any other primate (Sarich & Wilson, Reference Sarich and Wilson1967), the knuckle-walking hypothesis became the most parsimonious (Gebo, Reference Gebo1996; Richmond, Begun, & Strait, Reference Richmond, Begun and Strait2001; Washburn, Reference Washburn1967) and the familiar March of Progress – in which a knuckle-walking ape steadily evolves into an upright human – became culturally (Howell, Reference Howell1965) and academically (Wrangham & Pilbeam, Reference Wrangham and Pilbeam2002) entrenched.
However, despite a century of fossil discoveries and even clearer genetic evidence of a Homo–Pan clade (e.g. Chen & Li, Reference Chen and Li2001), our discipline appears to be no closer to identifying the body form from which bipedalism evolved than we were nearly a century ago (Figure 1). Some scholars have identified anatomical and behavioural inconsistencies with a knuckle-walking ancestor (Kivell & Schmitt, Reference Kivell and Schmitt2009; Tuttle, Reference Tuttle1969), leading to the endorsement of a climbing, arboreal precursor to bipedalism (Crompton, Vereecke, & Thorpe, Reference Crompton, Vereecke and Thorpe2008; Fleagle et al., Reference Fleagle, Stern, Jungers, Susman, Vangor and Wells1981; Tuttle, Reference Tuttle1981). Data collected on wild orangutans further supports the hypothesis that bipedalism may have its roots in a clambering ape moving with hand-assisted bipedal locomotion in an arboreal environment (Thorpe, Holder, & Crompton, Reference Thorpe, Holder and Crompton2007). However, a more recent study of the 4.4 Ma Ardipithecus ramidus partial skeleton has resurrected Straus's (1949) cercopithecoid model in which the first hominins diverged from a pronograde, above-branch, arboreal quadruped (Lovejoy, Suwa, Simpson, Matternes, & White, Reference Lovejoy, Suwa, Simpson, Matternes and White2009; White, Lovejoy, Asfaw, Carlson, & Suwa, Reference White, Lovejoy, Asfaw, Carlson and Suwa2015). Still, many scholars continue to support the most parsimonious hypothesis that bipedalism evolved from a knuckle-walking ancestor (e.g. Pilbeam & Lieberman, Reference Pilbeam and Lieberman2017; Prang et al., Reference Prang, Ramirez, Grabowski and Williams2021). Whether using comparative anatomy, the fossil record or modern primate behaviour, our colleagues have presented reasonable, even convincing, evidence for their differing last common ancestor (LCA) reconstructions. Yet they cannot all be correct. One of these models (or another not yet proposed) is accurate, and the others will be scientifically refuted in time. The fact that our discipline has not reached that point evinces the need for more data.
A related argument has unfolded regarding the number of lumbar vertebrae present in the last common hominid ancestor. Humans possess an average of five lumbar vertebrae while modern great apes (chimpanzees, bonobos, gorillas and orangutans) have short, stiff lumbar regions with an average of three to four lumbar vertebrae (Williams, Gómez-Olivencia, & Pilbeam, Reference Williams, Gómez-Olivencia and Pilbeam2019). The hylobatids are more human-like, with longer lumbar regions of four to six vertebrae, and cercopithecoids have the longest lumbar regions, with six or more vertebral elements (Williams et al., Reference Williams, Gómez-Olivencia and Pilbeam2019). The polarity of vertebral number remains contentious, however. Some scholars favour an African ape-like, short-backed model for the common ancestor (Pilbeam, Reference Pilbeam2004; Williams, Reference Williams2012; Williams, Middleton, Villamil, & Shattuck, Reference Williams, Middleton, Villamil and Shattuck2016; Williams, Gómez-Olivencia, & Pilbeam, Reference Williams, Gómez-Olivencia and Pilbeam2019), whereas others envision a long-backed ancestor (Böhme et al., Reference Böhme, Spassov, Fuss, Tröscher, Deane, Prieto and Begun2019; Lovejoy et al., Reference Lovejoy, Suwa, Simpson, Matternes and White2009; Lovejoy & McCollum, Reference Lovejoy and McCollum2010; Machnicki & Reno, Reference Machnicki and Reno2020; McCollum, Rosenman, Suwa, Meindl, & Lovejoy, Reference McCollum, Rosenman, Suwa, Meindl and Lovejoy2010; Ward, Hammond, Plavcan, & Begun, Reference Ward, Hammond, Plavcan and Begun2019), although not all of these authors agree on whether the lumbar region would be human and gibbon-like (approximately five lumbar vertebrae) or more cercopithecoid-like (six or more lumbar vertebrae). Furthermore, a long-backed model would necessitate the parallel evolution of a short, stiff lumbar spine in orangutans, gorillas and the genus Pan.
Each of the different models for the body form of the African ape and human LCA is informed to various degrees by extant primate models. The terrestrial knuckle-walker hypothesis draws upon evidence from chimpanzees, bonobos and gorillas; the clambering arboreal bipedal hypothesis is based, in part, on evidence gathered from orangutans; the above-branch pronograde quadrupedal hypothesis draws upon skeletal evidence from extant cercopithecoids; and the hylobatian ancestor is informed by studies of gibbons and siamangs.
Fortunately, the pattern of positional behaviour and back anatomy in these primates lends itself to an examination of these differing hypotheses. If a short-backed, orthograde, knuckle-walking ape had a body most predisposed to bipedal locomotion, then chimpanzees, bonobos and gorillas might be expected to exhibit bipedalism most often. If, instead, the LCA was a clambering arboreal ape, orangutans might be the most frequent bipeds. If the last common ancestor was a long-backed, pronograde ape, then baboons and mandrills might be expected to exhibit the most frequent bipedal locomotion. The only animals in our sample with a combination of an orthograde body posture and a long lumbar region of the spine are hylobatids and, thus, high frequencies of bipedal locomotion in these lesser apes would align with aspects of the hylobatian model.
Here, we investigate the frequency of bipedalism in a large sample of zoo-housed primates. We posit that collecting these data on captive primates is preferable to wild observations for addressing our particular question given the different forest structures of the African and Asian rainforests, and in this way, zoo data may serve as a ‘control’ for ecological differences and allow us to focus specifically on anatomical predispositions for bipedal locomotion.
Materials and methods
Participants
To investigate which body form is most conducive to bipedal locomotion, we surveyed primate caretakers from 46 institutions with accreditation from the Association of Zoos and Aquariums (AZA) to quantify the frequency of bipedalism in a large sample (N = 496) of captive hominoids and cercopithecines (see Table 1). Specifically, we examined bonobos (Pan paniscus; n = 37), chimpanzees (Pan troglodytes; n = 82), gorillas (Gorilla gorilla; n = 115), orangutans (Pongo sp. n = 120), hylobatids (Hylobates sp. and Symphalangus syndactylus; n = 93), and cercopithecines (Papio sp. and Mandrillus sphinx; n = 49). We defined bipedal locomotion in our survey as movement of any distance on two legs. Postural bipedalism was not included.
Table 1. Sample demographic characteristics

Procedures
Requests for survey completion were sent to primate caretakers of AZA-accredited institutions that were also members of the Ape and Baboon Species Survival Plans. Surveys were developed and administered through the Qualtrics platform. The survey included six introductory/general questions and up to seven specific questions for each reported primate (see supplementary survey). Three key survey questions were used to assess the frequency of bipedalism in each primate (see Table 2): Question 1 assessed the occurrence of bipedalism using a multiple choice with multiple answer option format; Question 2 provided an open-ended response format to identify the average number of daily bipedal bouts in each primate; and Question 3 examined the average number of steps per bipedal bout in a multiple choice with single answer option format. If a respondent selected ‘No bipedal locomotion was observed in any of these timeframes’ in Question 1 for a particular primate, the survey terminated, and the respondent was prompted to begin a new set of responses for the next primate in their care. Accordingly, all responses for Questions 2 and 3 consisted of primates who were reported in Question 1 as bipedal.
Table 2. Survey questions assessing the frequency of bipedalism

To identify the percentage of each species that has ever been observed to move bipedally, responses from Question 1 were dichotomised into two categories: (a) ‘Bipedal locomotion observed’ (including responses ‘This past month’, ‘This past week’ and ‘Today’); and (b) ‘No bipedal locomotion observed’ (including the response ‘No bipedal locomotion was observed in any of these timeframes’). Question 1 responses were not analysed across the original four response options because Question 2 provided the same information as Question 1 with additional quantitative benefits such as the specific number of bipedal bouts within an identified timeframe. Question 3 was asked in a multiple-choice format given the anticipated likelihood that an open-ended response option would yield responses spanning different ranges, thereby complicating comparisons across primates.
Additional information about the circumstances prompting primate bipedal locomotion was also collected in the survey and is the subject of ongoing analysis. Chi square analysis and paired-sample t-tests analyses were conducted using IBM SPSS Statistics (version 26).
Results
We find that species significantly differ by the occurrence of bipedalism (χ 2 = 51.99, d.f.(5), p < 0.001; Figure 2). More specifically, post hoc analyses reveal significant differences in the occurrence of bipedalism between hylobatids and all other species, including bonobos (p = 0.001), chimpanzees (p < 0.001), gorillas (p = 0.001), orangutans (p < 0.001) and cercopithecines (p < 0.001). Additional significant differences were found between gorillas and cercopithecines (p = 0.005).
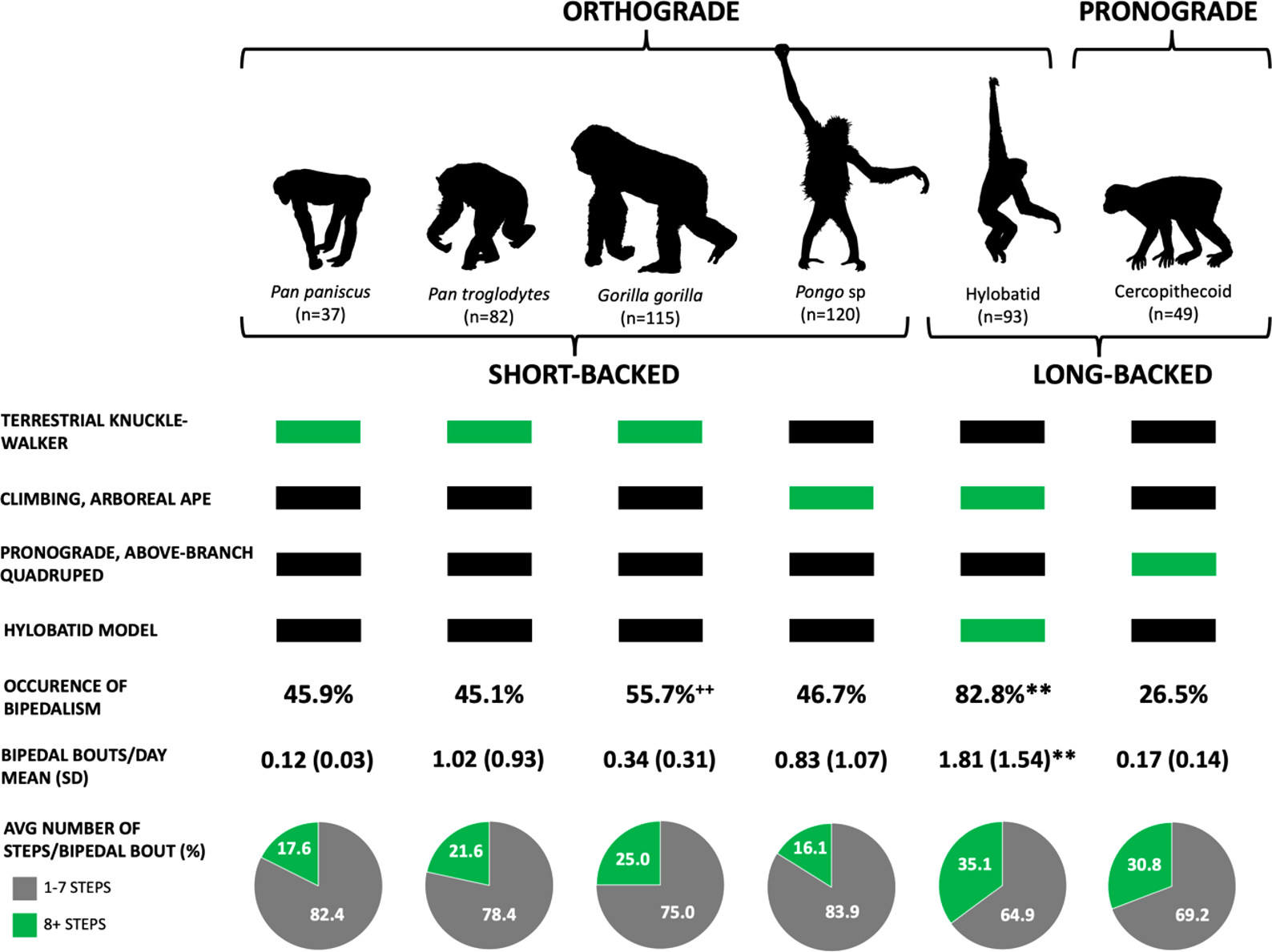
Figure 2. Bipedal behaviour in extant primates. Extant apes have an orthograde body plan, whereas monkeys are pronograde. Differences also exist in the number of lumbar vertebrae, with the great apes possessing three to four lower back vertebrae (short-backed) and hylobatids and cercopithecines having five to seven (long-backed). Humans and fossil hominins also have five lumbar vertebrae. We assess the different models for bipedal origins (listed along the left) and, if supported, which living apes are expected to exhibit the highest frequency of bipedalism (green rectangles). In our sample, 82.8% of the hylobatids (n = 77/93) moved bipedally, a value significantly higher than that for any other taxon. Furthermore, of the animals moving bipedally, hylobatids did it most frequently: 1.81 times per day. In contrast, a bipedal gorilla exhibits this behaviour, on average, once every three days. The long-backed primates – hylobatids and cercopithecines – also take the most steps per bipedal bout. ** p < 0.01; hylobatids were significantly more likely to be bipedal and had significantly more bipedal bouts per day compared with all other species. ++ p < 0.01; gorillas were significantly more likely to be bipedal compared with cercopithecines.
The results also show significant differences between species in the average number of daily bipedal bouts (F = 13.99, d.f.(5), p < 0.001). Post hoc analyses indicate that, among primates reported to move bipedally, significant differences exist between hylobatids and all other species, including bonobos (p < 0.001), chimpanzees (p = 0.008), gorillas (p < 0.001), orangutans (p < 0.001) and cercopithecines (p < 0.001). Species did not significantly differ relative to the number of steps per bipedal bout (χ 2=7.39, d.f.(5), p = 0.193) although here, as well, hylobatids achieved the highest value (Figure 2).
Discussion
The current findings demonstrate that hylobatids are both more likely to move bipedally and to do so more frequently than any other ape or monkey in a captive environment. While it has been suggested that bipedality in hylobatids is largely driven by their excessively long forelimbs (Lovejoy & McCollum, Reference Lovejoy and McCollum2010), captive hylobatids have been documented to employ various terrestrial gaits, including quadrupedal and tripedal locomotion (Vereecke, D'Août, & Aerts, Reference Vereecke, D'Août and Aerts2006). In fact, Vereecke et al. (Reference Vereecke, D'Août and Aerts2006) report frequent terrestrial quadrupedal locomotion in a population of captive gibbons unhindered by their elongated forelimbs. We thus interpret our findings as an indication that additional morphological features of the hylobatid body form, aside from their limb proportions, predispose them to walk bipedally more frequently than other primates – specifically, a long lumbar spine and orthograde positional behaviour. A limitation of this study is that we did not include any atelines, a family of primates that also possesses this combination of long lumbar spine and frequent orthograde positional behaviour.
To be sure, evolutionary trajectories do not always follow the path of least resistance. All of the primate taxa in our study practise some degree of bipedal locomotion, providing the behavioural and anatomical raw material for natural selection to favour this form of locomotion in early hominins no matter the body form of the LCA. In other words, if the strength of selection was sufficiently high, bipedal behaviour could have evolved from any of the models investigated in this study. Therefore, we cannot refute any of the hypotheses generated from modern models in a Popperian sense.
Yet, we also cannot ignore these data demonstrating that hylobatids – the only primates in our study with a long lumbar region and an orthograde body posture, essential characteristics for upright walking in humans (Williams & Russo, Reference Williams and Russo2015) – practice bipedal locomotion more frequently and for longer distances than the other non-human primates (Figure 3). Our data are therefore consistent with a long-backed, orthograde LCA, which are foundational elements of the hylobatian hypothesis (Avis, Reference Avis1962; Gregory, Reference Gregory1928; Keith, Reference Keith1923; Morton, Reference Morton1924; Tuttle, Butzer, & Blumenberg, Reference Tuttle, Butzer and Blumenberg1974). We are not suggesting that the LCA was the size of modern hylobatids (see Grabowski & Jungers, Reference Grabowski and Jungers2017; Tuttle et al., Reference Tuttle, Butzer and Blumenberg1974), nor was it likely to be a specialised brachiator, merely that it was orthograde (as all modern apes are) and may have lacked the short-stiff lumbar spine present in great apes. Importantly, several middle-to-late Miocene fossil hominoids such as Oreopithecus (Hammond et al., Reference Hammond, Rook, Anaya, Cioppi, Costeur, Moyà-Solà and Almécija2020), Rudapithecus (Ward et al., Reference Ward, Hammond, Plavcan and Begun2019), Danuvius (Böhme et al., Reference Böhme, Spassov, Fuss, Tröscher, Deane, Prieto and Begun2019) and Pierolapithecus (Machnicki & Reno, Reference Machnicki and Reno2020) are also reconstructed as long-backed, orthograde climbers with at least some capacity for arboreal bipedalism. Additionally, the earliest known hominin partial skeleton from 4.4 Ma Ardipithecus ramidus is hypothesised to possess an elongated lumbar region (Lovejoy et al., Reference Lovejoy, Suwa, Simpson, Matternes and White2009).

Figure 3. Bipedalism in Hylobates. Photograph courtesy of Evie Vereecke.
These lines of evidence tentatively indicate that humans and hylobatids reflect the ancestral body form with respect to lumbar mobility and positional behaviour (for more similarities see Zichello, Reference Zichello2018). If true, then the short, stiff lumbar regions and correlated terrestrial knuckle-walking locomotion evolved in parallel in Pan and Gorilla as some have hypothesised (Böhme et al., Reference Böhme, Spassov, Fuss, Tröscher, Deane, Prieto and Begun2019; Lovejoy et al., Reference Lovejoy, Suwa, Simpson, Matternes and White2009; Lovejoy & McCollum, Reference Lovejoy and McCollum2010; Machnicki & Reno, Reference Machnicki and Reno2020; McCollum et al., Reference McCollum, Rosenman, Suwa, Meindl and Lovejoy2010; Ward et al., Reference Ward, Hammond, Plavcan and Begun2019). This interpretation of our findings is subject to modification as more hominid fossils from the late Miocene of Africa and Eurasia are unearthed.
Supplementary material
To view supplementary material for this article, please visit https://doi.org/10.1017/ehs.2022.9.
Acknowledgements
We would like to thank E. Carpenter-Song, M. Stern, L. Perkins, A. Meinelt, and the Ape and Baboon Species Survival Plan coordinators for their assistance in contacting the participating AZA institutions. We would also like to thank each of the participating institutions and their primate caretakers for their time and effort to complete our survey; this research would not be possible without them. A full list of the institutions can be found in the Supplemental Information. E. Vereecke generously provided Figure 3. We are grateful to P. Reno and an anonymous reviewer for helpful suggestions that improved the paper.
Author contributions
Conceptualisation, K.H.R., C.E.J. and J.M.D.; methodology and formal analysis, K.H.R., C.E.J. and J.M.D.; investigation, K.H.R.; writing, K.H.R. and J.M.D.
Financial support
This work was supported by Dartmouth College.
Conflict of interest
There are no conflicts of interest to declare.
Research transparency and reproducibility
All raw data used to formulate the ideas in this paper are available as supplemental documents.
Supplemental information
Document S1, Excel file with a list of contributing AZA institutions and the primate sample size from each. Document S2, Sample survey distributed to primate caretakers at zoos. Movie S1, Female Gorilla gorilla moving bipedally at Zoo Atlanta.