Introduction
Reality monitoring is a crucial cognitive process in the daily life to differentiate memories of thoughts and imagination from memories of externally derived information [Reference Johnson, Hashtroudi and Lindsay1]. For instance, this process allows us to determine whether an event was generated by our imagination or if it really did occur.
A deficit in the reality-monitoring abilities has been repeatedly observed in patients with schizophrenia compared with healthy individuals (e.g., [Reference Keefe, Arnold, Bayen and Harvey2]; for recent review, see [Reference Simons, Garrison and Johnson3]). More specifically, several studies have pointed out that patients with schizophrenia and auditory hallucinations (AHs) were more likely to misattribute internally generated stimuli as being perceived from the environment than patients with schizophrenia without AHs and healthy individuals ([Reference Brunelin, Combris, Poulet, Kallel, D’Amato and Dalery4–Reference Woodward, Menon and Whitman6]; for review, see [Reference Waters, Woodward, Allen, Aleman and Sommer7,Reference Brookwell, Bentall and Varese8]). This tendency to misattribute imagined events as being perceived is called an externalization bias and is assumed to partly underlie AHs. Indeed, a prominent cognitive model of AHs suggests that they might arise from a misattribution of internal mental events such as inner speech as being externally perceived [Reference Bentall9,Reference Frith10].
The neural network underlying reality-monitoring process has been explored in both healthy individuals and patients with schizophrenia in the literature. The prefrontal cortex (PFC), and particularly its medial and anterior part, was found to be a key structure of this network (for review, see [Reference Simons, Garrison and Johnson3,Reference Mitchell and Johnson11]). Interestingly, a functional neuroimaging study has reported that the externalization bias was correlated with a reduced activation in this specific brain region [Reference Simons, Davis, Gilbert, Frith and Burgess12]. In patients with schizophrenia, deficits in the neural activity of the medial PFC have been observed during reality-monitoring performances [Reference Garrison, Fernandez-Egea, Zaman, Agius and Simons13,Reference Vinogradov, Luks, Schulman and Simpson14]. The medial PFC is not the only brain region that may account for the reality-monitoring process. Indeed, the contribution of temporoparietal areas, and particularly their abnormal overactivation, into the experience of externalization bias has been supported by neuroimaging studies [Reference Sugimori, Mitchell, Raye, Greene and Johnson15] as well as noninvasive brain stimulation studies [Reference Mondino, Poulet, Suaud-Chagny and Brunelin16].
Although neuroimaging studies have broadly investigated brain activity linked to reality-monitoring performances, less is known about the neuroanatomical correlates of reality monitoring. In recent years, the morphology of a specific structure of the medial PFC, the paracingulate sulcus (PCS), has been investigated. The PCS is a tertiary sulcus that lies in the medial wall of the PFC and runs dorsal and parallel to the cingulate sulcus in a rostro-caudal direction. The PCS presents a great morphological variability within the general population, in that it can be found in none, one, or both hemispheres [Reference Paus, Tomaiuolo, Otaky, MacDonald, Petrides and Atlas17], and its presence affects the morphometry [Reference Fornito, Wood, Whittle, Fuller, Adamson and Saling18,Reference Fornito, Whittle, Wood, Velakoulis, Pantelis and Yücel19] and the cytoarchitectonic organization of surrounding cortices [Reference Amiez, Sallet, Hopkins, Meguerditchian, Hadj-Bouziane and Ben Hamed20,Reference Vogt, Nimchinsky, Vogt and Hof21]. The PCS was found to be associated with a wide array of executive and cognitive functions [Reference Amiez, Wilson and Procyk22], including reality monitoring [Reference Buda, Fornito, Bergström and Simons23]. Namely, healthy individuals with bilaterally absent PCS showed significantly reduced reality-monitoring performances compared with individuals with present PCS in at least one hemisphere [Reference Buda, Fornito, Bergström and Simons23]. In schizophrenia patients, some studies showed that reduced PCS length was associated with AHs [Reference Garrison, Fernyhough, McCarthy-Jones, Simons and Sommer24,Reference Garrison, Fernyhough, McCarthy-Jones, Haggard and Simons25]. However, the relationship between the PCS length and reality-monitoring performances remains unclear in patients with schizophrenia and AHs. Particular anatomical features in the medial PFC and specific morphology of the PCS could underpin the relationship between brain activity within these areas and reality-monitoring process.
The present study aimed to identify whether reality-monitoring performances were linked to specific neuro-anatomical features, including PCS length and gray-matter volume (GMV), in hallucinating patients with schizophrenia. Therefore, we conducted a magnetic resonance imaging (MRI) study combining an investigation of reality-monitoring performances, a morphological analysis of the PCS, and a whole-brain voxel-based morphometry (VBM) analysis. We hypothesized that reality-monitoring deficits, and particularly the externalization bias, will be negatively correlated with the PCS length. These hypotheses were based on three lines of work presented above showing that: (a) the absence of PCS is associated with poor reality-monitoring performances [Reference Buda, Fornito, Bergström and Simons23], (b) shorter PCS length is associated with AHs [Reference Garrison, Fernyhough, McCarthy-Jones, Simons and Sommer24], and (c) AHs are associated with a specific deficit in reality monitoring: the externalization bias [Reference Brookwell, Bentall and Varese8]. In addition, we hypothesized that poorer reality-monitoring performances, including higher externalization bias, would be associated with lower GMV in the brain regions that were identified as functionally involved in reality monitoring [Reference Mitchell and Johnson11] and in the externalization bias [Reference Simons, Davis, Gilbert, Frith and Burgess12] (e.g., the medial PFC).
Methods
Participants
Thirty-five patients meeting the DSM-IV-TR criteria for schizophrenia were recruited from our clinical unit for treatment-resistant schizophrenia at Le Vinatier Hospital between 2009 and 2015. All participants were native French speakers and presented daily treatment-resistant AHs, defined as persistent daily AHs despite an antipsychotic treatment at an adequate dosage for more than 6 weeks. Patients’ diagnoses were assessed through a formal interview with a trained psychiatrist using the Mini-International Neuropsychiatric Interview [Reference Sheehan, Lecrubier, Sheehan, Amorim, Janavs and Weiller26]. Participants were assessed for the severity of their symptoms using the Positive and Negative Syndrome Scale (PANSS) [Reference Kay, Fiszbein and Opler27]. Patients’ current antipsychotic medication classes (typical, atypical including clozapine, and combination of classes) were reported in Table 1. Written informed consent was obtained from all participants. All experiments were approved by a local ethic committee (CPP SUD EST VI, Clermont-Ferrand, France) and performed in compliance with relevant guidelines and regulations.
Table 1. Summary of demographic, clinical, and reality-monitoring measures and paracingulate sulcus (PCS) lengths of the 35 patients with schizophrenia and auditory hallucinations.

Abbreviation: SD, standard deviation.
a N = 34 (one missing data).
Reality-monitoring task
The task was divided in a presentation phase and a test phase, according to the task used and validated by Brunelin et al. [Reference Brunelin, Poulet, Marsella, Bediou, Kallel and Cochet28]. Briefly, during the presentation phase, 16 words were presented one by one on a computer screen for 3 s, all preceded by an instruction also presented during 3 s. Instructions were “Imagine yourself hearing the following word” or “Listen to the following word.” During the test phase, performed immediately after the presentation phase, a 24-word list was presented including the 16 words previously presented (8 imagined and 8 listened) and 8 new words (distractors). Patients had to determine the source for each word (i.e., “Imagined,” “Heard,” or “New”). Before the task, patients performed a short practice trial to acquaint with requirements of the task and to ensure for their good comprehension.
Three main outcomes were computed according to previous studies [Reference Garrison, Moseley, Alderson-Day, Smailes, Fernyhough and Simons29,Reference Moseley, Mitrenga, Ellison and Fernyhough30]. (a) Reality-monitoring accuracy was calculated using the following formula:

where $ fii $ is the number of imagined words that were correctly recognized as imagined,
$ fih $ is the number of imagined words identified as being heard,
$ fhh $ is the number of heard words correctly identified as heard, and
$ fhi $ is the number of heard words identified as imagined. This measure of reality monitoring, also known as average conditional source identification measure [Reference Murnane and Bayen31], reflects the proportion of correct source judgments among the item correctly recognized as old. (b) The externalization bias was defined as the number of imagined words recognized as heard among all imagined words incorrectly judged (i.e., as new or heard). (c) Item memory accuracy was calculated as the standardized hit rate (z-score of hit rate, i.e., the proportion of old items identified as old) minus the standardized false alarm rate (z-score of false alarm rate, i.e., the proportion of new items identified as old). Before calculation, hit and false alarm rates were corrected to avoid the values of 0 and 1, as recommended by Snodgrass and Corwin [Reference Snodgrass and Corwin32]. This measure of item memory, also known as the Signal Detection Theory metrics’ d’ [Reference Macmillan and Creelman33], reflects the sensitivity to discriminate between old and new items.
Magnetic resonance imaging acquisition
MRI acquisitions were performed on a 1.5-T Siemens Magneton scanner. A 3D anatomic T1-weighted sequence covering the whole brain volume was acquired with the following parameters: 176 transverse slices, TR = 1,970 ms, TE = 3.93 ms, field of view = 256 mm2, and voxel size = 1 mm3.
Paracingulate sulcus measurements
The PCS was measured following the measurement protocol described by Garrison et al. [Reference Garrison, Fernyhough, McCarthy-Jones, Haggard and Simons25] (see Figure 1 as an example). To validate the procedure, inter- and intrarater reliabilities were calculated. See the Supplementary Material for more details.

Figure 1. Example of the paracingulate sulcus (PCS) measurement on anatomical magnetic resonance imaging. The PCS is marked in red line and lies dorsal and parallel to the cingulate sulcus. Measurement was performed within the first quadrant (defined by z < 0 and y > 0) and on the fourth sagittal slice for both hemispheres.
Voxel-based morphometry analysis
All images were preprocessed and analyzed with the Computational Anatomy Toolbox (CAT, version 12.6; http://www.neuro.uni-jena.de/cat/) implemented in Statistical Parametric Mapping (SPM12) (Welcome Trust Center for NeuroImaging, London, UK; http://www.fil.ion.ucl.ac.uk.gate2.inist.fr/spm/software/spm12/) using MATLAB (R2018a, MathWorks, Inc., Massachusetts, USA). Both processing and analysis were performed following the standard protocol (http://www.neuro.uni-jena.de/cat12/CAT12-Manual.pdf) with default settings, unless otherwise indicated. This method has been previously validated and provides a great compromise between good quality and speed of processing [Reference Farokhian, Beheshti, Sone and Matsuda34]. Prior to preprocessing, each image was visually inspected for artifacts. Then, T1 images were corrected for bias field inhomogeneities, segmented into gray matter, white matter, and cerebrospinal fluid, spatially normalized into a standard Montreal Neurological Institute (MNI) space using the DARTEL algorithm, and modulated to allow comparison of the absolute amount of tissue. A second quality control for intersubject homogeneity and overall image quality was achieved using the automated quality check protocol of the CAT12 toolbox. After quality check, the total intracranial volume of each subject was estimated to be used as covariate on the second-level analyses to take into account intersubjects brain size variations. Finally, images were smoothed using an 8-mm Full Width-Half Max (FWHM) kernel.
Statistical analyses
Statistical analyses were conducted using R software (version 3.5.2)[35]. Normality of the data was tested using the Shapiro–Wilk test. Partial Spearman’s rank correlations were calculated to assess the relationship between PCS lengths (separately for each hemisphere) and outcomes of the reality-monitoring task (reality-monitoring accuracy, externalization bias, and item memory), with total intracranial volume and age as confounding variables. For all analyses, a significance level of p < 0.05 was employed. As exploratory analyses, we investigated whether PCS lengths were also related to total positive symptoms, by computing partial Spearman’s rank correlations between PCS lengths and total PANSS positive scores, with total intracranial volume and age as confounding variables.
VBM statistical analyses were performed with the CAT12 toolbox (version 12.6). A multiple linear regression model was used to test for voxel-wise correlations between GMV and reality-monitoring outcomes. Total intracranial volume and age were used as confounding covariates in these analyses. A 0.1 absolute masking threshold was applied to avoid artifact on the gray/white matter limit. For all voxel-based analyses, we thresholded statistical maps with an uncorrected p < 0.001 at voxel level and with an false discovery rate (FDR)-corrected p < 0.05 at the cluster level. Significant clusters were labeled using the Anatomical Automatic Labelling in SPM.
Results
Patients’ demographic and clinical characteristics, as well as reality-monitoring outcomes, total intracranial volumes, and PCS lengths for each hemisphere, are presented in Table 1. Details on patients’ scores at each individual item of the PANSS positive subscale are provided in the Supplementary Material.
Reality monitoring and PCS length
While controlling for age and total intracranial volume, the PCS length was positively correlated with reality-monitoring accuracy in the right hemisphere (Spearman’s partial ρ = 0.431, p = 0.012; Figure 2A) but not in the left hemisphere (Spearman’s partial ρ = 0.052, p = 0.773). There was a significant negative correlation between the length of the right PCS and the externalization bias (Spearman’s partial ρ = −0.379, p = 0.029; Figure 2B), but no significant correlation was found for the left PCS length and the externalization bias (Spearman’s partial ρ = 0.171, p = 0.340). No significant correlations were found between PCS lengths and item memory (for the right PCS: Spearman’s partial ρ = 0.137, p = 0.448; for the left PCS: Spearman’s partial ρ = −0.003, p = 0.988).

Figure 2. Scatter plots showing the partial correlations between the paracingulate sulcus length in the right hemisphere and (A) reality-monitoring accuracy and (B) the externalization bias, controlling for total intracranial volume and age (n = 35).
Exploratory analyses revealed no significant correlations between PCS lengths and total PANSS positive scores (for the right PCS: Spearman’s partial ρ = −0.021, p = 0.908; for the left PCS: Spearman’s partial ρ = −0.108, p = 0.555).
Reality monitoring and GMV
VBM analysis revealed a significant positive correlation between reality-monitoring accuracy and GMV in the right angular gyrus (peak MNI coordinates [23 −59 44], t = 4.03, p < 0.001; see Table 2 and Figure 3A).
Table 2. Clusters showing significant correlations between gray-matter volume and reality-monitoring measures (n = 35).

Note: Statistical threshold of p < 0.001 at the peak-level and FDR-corrected p < 0.05 at the cluster level.
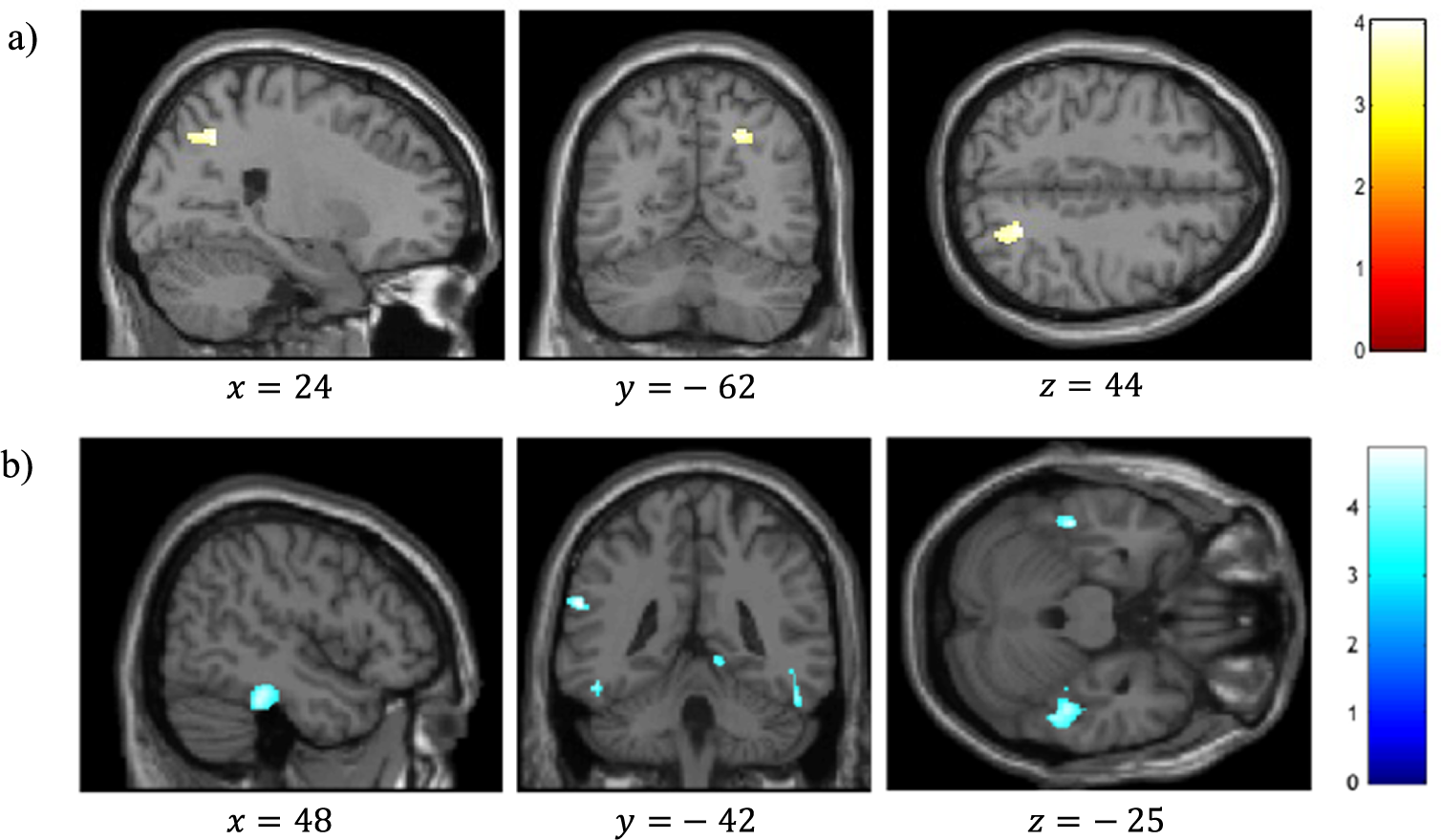
Figure 3. Voxel-based morphometry analysis of correlation between gray-matter volumes and reality-monitoring performances, corrected for total intracranial volume and age (n = 35). Results are reported using z-values presented in the SPM canonical single subject template with MNI coordinates of the section. Regions that survived a statistical threshold of p < 0.001 at the peak-level (FDR-corrected p < 0.05 at the cluster level) are shown in (A) for positive correlation with reality-monitoring accuracy and (B) for negative correlation with externalization bias.
The analysis also revealed a significant negative correlation between the externalization bias and GMV in a cluster encompassing the left supramarginal gyrus and the left superior temporal gyrus ([−60 −42 24], t = 4.83, p < 0.001), in the right lingual gyrus ([15 −51 −3], t = 4.71, p < 0.001), and both in the left and the right inferior temporal gyrus and fusiform gyrus (respectively, [−46 −33 −26], t = 4.60, p < 0.001, and [48 −33 −24], t = 4.48, p < 0.001; Table 2 and Figure 3B).
Additional VBM analyses revealed a significant negative correlation between item memory and GMV in the right superior frontal gyrus (peak MNI coordinates [26 45 22], t = 4.57, p < 0.001; Table 2).
Discussion
The present study sought to identify the neuroanatomical correlates of reality monitoring in a sample of schizophrenia patients with AHs. We reported two main findings: (a) the right hemisphere PCS length was positively correlated with reality-monitoring accuracy and negatively correlated with the externalization bias, that is, the misattribution of imagined words to an external source, and (b) the reality-monitoring accuracy was positively correlated with the GMV in the right angular gyrus, whereas the externalization bias was negatively correlated with the GMV in a set of temporal and parietal areas.
We demonstrated a significant correlation between the reality-monitoring abilities of hallucinating patients with schizophrenia and the length of the PCS in the right hemisphere: the shorter the PCS, the poorer the reality-monitoring accuracy and the greater the externalization bias. On the one hand, these results are highly coherent with those found in healthy subjects associating the absence of PCS with worse overall reality-monitoring accuracy [Reference Buda, Fornito, Bergström and Simons23]. On the other hand, the region containing the PCS has been associated with both AHs and reality-monitoring abilities [Reference Buda, Fornito, Bergström and Simons23–Reference Garrison, Fernyhough, McCarthy-Jones, Haggard and Simons25]. Moreover, a recent study has demonstrated that this region causally supports reality monitoring. In healthy subjects, active real-time fMRI neurofeedback training of the paracingulate cortex has been reported to improve the reality-monitoring accuracy for imagined items as well as the functional activity of the paracingulate cortex [Reference Garrison, Saviola, Morgenroth, Barker, Lührs and Simons36]. If the relationship between the PCS morphology and the functional role of the paracingulate cortex remains unclear, taken together these findings suggest that the PCS morphology may be the structural basis for the causal role of the paracingulate cortex in reality-monitoring abilities and hallucinations. Indeed, the PCS morphology is known to influence the topography of the medial PFC [Reference Vogt, Nimchinsky, Vogt and Hof21] and to generate a great interindividual variability on the location of the neural activity evoked in the medial PFC during a given cognitive task in healthy subjects [Reference Amiez, Neveu, Warrot, Petrides, Knoblauch and Procyk37]. Future fMRI studies should consider this morphological variability when reporting differences in brain activity in the medial PFC during reality-monitoring paradigms. The differences in medial PFC activity observed at the group level during a reality-monitoring task could reflect a different location of the neural activity due to intersubject differences in the PCS morphology. Taking into account this neuroanatomical feature when studying functional patterns of reality monitoring would provide more reliable evidence of a deficit in populations experiencing AHs.
It is noteworthy that the PCS is one of the latest sulci to develop in utero, appearing at the 36th week of ontogeny and maturing to the perinatal period for human [Reference Amiez, Wilson and Procyk22,Reference Armstrong, Schleicher, Omran, Curtis and Zilles38]. This sulcus is thus exposed to environmental factors able to interfere with its development. The reality-monitoring impairment found to be correlated with the PCS length may thus result from defective neurodevelopmental mechanisms. In this line, abnormal reality-monitoring performances have also been observed before the onset of frank psychotic episode in individuals at risk for schizophrenia within the continuum of psychosis [Reference Lavallé, Dondé, Gawęda, Brunelin and Mondino39]. A deepen investigation of the sulcal ontogeny, and even more of the developmental factors that may influence the PCS morphometry could improve the understanding of its relationship with reality-monitoring deficits.
Consistent with the right lateralization of our findings, a recent study found a reduction of the PCS length only in the right hemisphere of both psychotic and nonclinical voice hearers [Reference Powers, van Dyck, Garrison and Corlett40], suggesting the right PCS length reduction to be a specific marker of AHs whatever the clinical condition. By contrast, some studies identified bilateral PCS reductions in schizophrenia patients with AHs as compared with schizophrenia patients without AHs, nonclinical subjects with AHs, and healthy controls [Reference Garrison, Fernyhough, McCarthy-Jones, Simons and Sommer24,Reference Garrison, Fernyhough, McCarthy-Jones, Haggard and Simons25], and some others found specific left PCS reduction in schizophrenia patients with AHs as compared with those without AHs, and healthy controls [Reference Rollins, Garrison, Arribas, Seyedsalehi, Li and Chan41]. Further studies are thus needed to clarify if the length of the right PCS may be considered as a specific neuroanatomical marker of AHs or if the bilateral PCS is only reduced in schizophrenia patients with AHs.
Surprisingly, reality-monitoring performances did not correlate with GMV in medial frontal areas. Yet, the functional capacity of the medial PFC has been largely involved in the reality-monitoring process in both patients with schizophrenia and healthy individuals [Reference Garrison, Fernandez-Egea, Zaman, Agius and Simons13,Reference Metzak, Lavigne and Woodward42], and reduced GMV has been observed in these brain areas in patients with schizophrenia [Reference Chan, Di, GM and Gong43]. In addition, the presence/absence of PCS has been associated with GMV in the surrounding frontal regions, and these volumetric changes were related to reality-monitoring performances [Reference Buda, Fornito, Bergström and Simons23]. Further studies are now needed to investigate a potential relationship between the PCS variability and the surrounding prefrontal volume and its implication on the prefrontal functional capacity during reality monitoring.
As we hypothesized, most of the regions for which the GMV correlated with reality-monitoring performances correspond to the temporoparietal areas previously identified by functional imaging during reality-monitoring tasks. We found several brain structures whose GMVs negatively correlate with the externalization bias, indicating that schizophrenia patients with AHs with reduced GMV in these structures are more likely to misattribute internally generated information to an external source.
First, we observed a negative correlation between the externalization bias and a cluster encompassing the left supramarginal gyrus and the left superior temporal gyrus, which is considered as a part of Wernicke’s area (BA 40) involved in auditory and speech processing. Disruption to this system would induce an inadequate treatment of the verbal items presented in reality-monitoring tasks and participate to patients’ misattributions of source. In addition, a recent meta-analysis on motor agency specifically highlighted the left BA 40 as an integral part of the body-ownership network [Reference Seghezzi, Giannini and Zapparoli44]. This cluster can thus be considered as an element of both verbal and nonverbal self-production recognition, suggesting its modality-general implication in reality-monitoring processes. Consistently, the GMV and activity of this temporoparietal region have also been associated with AHs in schizophrenia patients [Reference Modinos, Costafreda, van Tol, McGuire, Aleman and Allen45–Reference Zmigrod, Garrison, Carr and Simons47]. The causal implication of temporoparietal regions in reality monitoring has finally been demonstrated by noninvasive stimulation over this region that modulated the externalization bias in both healthy subjects and schizophrenia patients and alleviated AHs in schizophrenia patients [Reference Mondino, Poulet, Suaud-Chagny and Brunelin16, Reference Brunelin, Poulet, Bediou, Kallel, Dalery and D’amato48–Reference Otani, Shiozawa, Cordeiro and Uchida50].
The VBM analysis also revealed negative correlations between the externalization bias and gray matter in several posteroinferior temporal regions. Considered as associative visual areas, these structures have mainly been associated with visual processing and visual hallucinations [Reference Zmigrod, Garrison, Carr and Simons47,Reference Rollins, Garrison, Simons, Rowe, O’Callaghan and Murray51,Reference Stephan-Otto, Siddi, Senior, Muñoz-Samons, Ochoa and Sánchez-Laforga52]. For now, the implications of the correlation between their GMV and externalization bias in our semantic task are unclear, and future studies should clarify the relationship between reduced GMV in these areas and the incorrect source attributions observed in schizophrenia patients with AHs. However, a substantial body of functional studies has already reported an activation of the right lingual gyrus during Theory-of-Mind tasks, involving among other things to make the distinction between internal and external space [Reference Jáni and Kašpárek53]. On its side, the left inferior temporal gyrus has been shown to specifically activate in the reality-monitoring contrast “correct attributions” versus “misattributions” in healthy participants [Reference Kensinger and Schacter54].
We identified a significant positive correlation between the reality-monitoring accuracy and the GMV of the right angular gyrus. This result replicates in a population of schizophrenia patients with AHs the results reported by Buda et al. [Reference Buda, Fornito, Bergström and Simons23] in a sample of healthy subjects. The right angular gyrus is engaged in a wide range of tasks reflecting our ability to discriminate the internal from external environment, such as Theory-of-Mind or agency attribution tasks [Reference Mar55,Reference Sperduti, Delaveau, Fossati and Nadel56]. Moreover, several case reports described its causal involvement in out-of-body experiences, a phenomenon referring to an autoscopic experience during which the subject perceive the world from an out-of-body position [Reference Blanke, Ortigue, Landis and Seeck57,Reference De Ridder, Van Laere, Dupont, Menovsky and Van de Heyning58]. In this way, our findings contribute to define the right angular gyrus as a pivotal neural locus for the distinction between the self and the external world. Its increased GMV may underlie its overactivity and in turn sustain decreased reality-monitoring performances in schizophrenia patients with AHs.
In addition to the sample size that could be considered as limited for correlation analyses (estimated post hoc power of 0.75), the main limitation of this study is the lack of comparison groups. Additional groups of healthy participants, healthy voice hearers, and patients with schizophrenia without AHs would had allowed us to determine if the structural correlates of reality monitoring are specific to schizophrenia or if they could be expanded to the global population. However, despite this limitation, our study has the advantage of investigating reality monitoring in a homogeneous sample of patients with severe daily treatment-resistant AHs, as compared with mixed samples of patients with heterogenous symptoms that are usually enrolled in the literature. The particularity of our patient sample in terms of treatment resistance and severity of AHs might also contribute to the differences in the right PCS length observed between our study and other studies including patients with AHs [Reference Garrison, Fernyhough, McCarthy-Jones, Simons and Sommer24,Reference Garrison, Fernyhough, McCarthy-Jones, Haggard and Simons25,Reference Rollins, Garrison, Arribas, Seyedsalehi, Li and Chan41]. Second, the question of the specificity of findings reported in the current study remains open. VBM findings suggested that reality-monitoring performances and item memory were linked to GMV changes in different brain regions. In addition, the PCS length seems to be specifically linked to reality-monitoring performances, that is, to reality-monitoring accuracy and externalization bias, but not to item memory or total positive symptoms. However, further investigations might assess whether reality monitoring might be related to other sulci. Third, one could question how the PCS, which can be considered as a static brain structure, could be related to a dynamic process such as reality monitoring. Although the PCS is expected to remain stable after its maturation during perinatal period, some PCS length changes over time have been described in a longitudinal study with adolescent onset psychosis [Reference Clark, Mackay, Davidson, Iversen, Collinson and James59]. Nevertheless, the observed correlation of reality-monitoring outcomes and PCS lengths does not necessarily imply that the PCS length is the only anatomical substrate for reality-monitoring deficits (and the emergence of AHs). Rather, we could hypothesize a two-hit process with a reality-monitoring deficit that predates the emergence of AHs, since reality-monitoring deficits are also reported in people with an at-risk mental state for psychosis and unaffected relatives of patients with schizophrenia [Reference Lavallé, Dondé, Gawęda, Brunelin and Mondino39], and which might be linked to the PCS length, and a second phase of aggravation of reality-monitoring deficits, together with other neuroanatomical features, such as GMV alterations.
In summary, this study demonstrated that reality-monitoring performances correlated with both the PCS morphology and the GMV in crucial brain regions engaged in the reality-monitoring neural network in patients with schizophrenia. If the exact relationship between the structural evidence that we have highlighted and their functional implications remains little known, these correlations propose some anatomical substrates for the observed reality-monitoring errors in schizophrenia patients with AHs. Such associations would lead future studies to clarify the relationship between the PCS and GMV variability and reality-monitoring abilities. Finally, further research work should investigate if similar structural features would be associated with AHs in nonclinical hallucinating individuals or if they specifically characterize AHs in schizophrenia.
Supplementary Materials
To view supplementary material for this article, please visit http://dx.doi.org/10.1192/j.eurpsy.2021.2234.
Data Availability Statement
The data that support the findings of this study are available from the corresponding author, M.M., upon reasonable request.
Acknowledgments
We would like to thank Caroline Damasceno for her help during participants recruitment and data collection, the CERMEP for their help in the MRI acquisitions, and all the participants of the study. We would also like to thank Laurine Paillet who achieved PCS measures allowing the assessment of inter-rater reliability.
Authorship Contributions
Conceptualization: J.B. and M.M.; Data curation: J.B. and M.M.; Formal analysis: M.P.; Investigation: F.H., J.B., and M.M.; Methodology: M.P., J.B., and M.M.; Resources: M.-F.S.-C., J.B., and M.M.; Supervision: M.M.; Validation: M.M.; Visualization: M.P.; Writing—original draft: M.P.; Writing—review & editing: all authors.
Financial Support
This research received no specific grant from any funding agency, commercial, or not-for-profit sectors. J. Brunelin was supported by a grant from the Neurodis Foundation (AO2018).
Conflicts of Interest
The authors declare none.
Comments
No Comments have been published for this article.