Introduction
The outbreak of the novel severe acute respiratory syndrome coronavirus 2 disease (SARS-CoV-2), the cause of coronavirus disease 2019 (COVID-19), has currently spread to almost all parts of the world. Available evidence indicates that the agent is transmitted via respiratory droplets and contact routes between humans [1]. Measures that hinder the spread of the virus include environmental control of indoor air flow [Reference Gola2]. However, relatively little attention has been paid to air-conditioning systems, which are one of the most common factors affecting indoor air flow. Some reports have implicated such systems in the transmission of SARS-CoV-2 [Reference Correia3,Reference Lu4], and norovirus [Reference Zhang5].
Air-conditioning systems play an important role in maintaining indoor air temperature and humidity in public buildings and hospitals. In the latter, particularly, intensive care units (ICUs) and operating rooms, the systems are fitted with high-efficiency particulate air (HEPA) filtration and laminar flow design to reduce the risk of air-borne infections. Installation of air-conditioning systems can help prevent hyperthermia in critically ill infected patients in a heat wave, and may reduce the cost of blood cultures requested since the number of cultures taken increases in such patients if a high ambient temperature is sustained [Reference Stephan6]. The systems have also proven effective in reducing mortality in heat-related illness in domestic homes [Reference O'Neill, Zanobetti and Schwartz7], and hospitals [Reference Lenzer8].
However, air-conditioning systems represent a potential source of microbial contamination in hospitals, as accumulated dust and moisture increase the risk of contamination and associated infections. Indeed, several fungal genera have been demonstrated in air-conditioned ICU [Reference Alves Simoes, Leite Junior and Hahn9], and mould colonisation has been observed in HEPA filters, and in air-conditioning systems [Reference Price10], as has the presence of SARS-CoV-2 on swab samples taken from surfaces of filters [Reference Mouchtouri11]. Likewise, contamination of air-conditioning systems has been implicated in some hospital-acquired infections [Reference Kumari12–Reference Stone14].
The risk of proliferation of microbes from air-conditioning systems and their transmission to high-risk patients, in hospitals is greatly reduced if strict management and control practices are followed [Reference Montagna15]. However, despite the several regulations covering the installation of these systems in hospitals, such as the HVAC Design Manual for Hospitals and Clinics published by the American Society of Heating, Refrigeration and Air-Conditioning Engineers, adherence to these standards is variable in routine practice. Indeed, epidemiological surveillance in a hospital in Paris found that only 32% of the patients diagnosed with invasive nosocomial aspergillosis were housed in rooms where an HEPA air filter system had been installed [Reference Bocquet16].
Studies on heating, ventilation and air-conditioning (HVAC) systems in hospitals have largely been conducted in restricted areas such as operating rooms and ICUs, and have focused on the impact of different airflow patterns, number of personnel, ventilation rates and other extrinsic factors [Reference Sadrizadeh17–Reference Lee19]. However, in the public areas of hospitals (wards, clinics etc.) airflow may be suboptimal and result in a higher risk of microbial contamination. This study therefore focused on these areas in which high-efficiency filters are rarely installed, and which have often been overlooked in other investigations.
Our aim was to clarify the presence and nature of potential risks associated with the use of air-conditioning systems, through the systematic assembly and analysis of published evidence on the effect of air-conditioning systems on the transmission of pathogens and related infectious diseases. Further, we explored effective measures for the protection of patients, staff and visitors from the potential risks of exposure to microorganisms related to air-conditioning systems, and application of measures with potential to combat such transmission in the COVID-19 pandemic.
Methods
The guidelines of the Preferred Reporting Items for Systematic Reviews and Meta-Analyses Extension for Scoping Reviews (PRISMA-ScR) [Reference Tricco20] were followed in this research study. The key stages of this framework [Reference Arksey and O'Malley21] were: identifying the research question, identifying relevant studies, study selection, charting of data and collating, summarising and reporting the results.
Research questions
The study addressed the following questions:
1. Is there any association between air-conditioning systems and the presence of pathogenic microorganisms in public areas of hospitals?
2. Do air-conditioning systems increase the risk of infection in such hospital areas?
Relevant studies
The complete PubMed, Embase and Web of Science databases were explored for relevant studies in December 2020. The search strategy is outlined in ‘Supplementary Material’. Papers published since the databases were established were included in the search, and relevant cited references.
Inclusion and exclusion criteria
All studies met the following criteria: published in English; intervention included different kinds of air-conditioning systems, such as unfiltered air or air-conditioning systems with HEPA filters; comparators were other areas without air-conditioning systems; assessment of the presence and measurement of pathogenic microorganisms in indoor air, ratios of viable microorganisms detected, incidence of infectious diseases, among others. The year of publication was not restricted in the literature search.
The exclusion criteria included the following: air-conditioning systems located only in operating rooms or other restricted areas; additional interventions (e.g. ultraviolet germicidal irradiation) combined with air-conditioning but focused on outcomes irrelevant to air-conditioning; and studies lacking specific data or comparators.
Study selection
All articles identified in the databases were exported into Endnote (Version 9.3), and duplicates were removed on initial screening. Study titles and abstracts and web searches of citations of relevant studies were screened by two independent researchers (Han-Ting Wu and Rong-Chen Dai) to assess their potential relevance for full review. The same researchers also independently reviewed the full texts of candidate articles against the inclusion and exclusion criteria. Any discrepancies were resolved through discussion with a third reviewer. The reasons for exclusion during the screening of the full texts were recorded.
Charting the data
Data were extracted independently by two review authors and discrepancies were identified and resolved as above. They comprised: names of the authors, year, type of study, outcome of interest, bacterial or fungal pathogens, hospital locations tested, air-conditioning systems used and relevant results and study conclusion.
Collating, summarising and reporting results
Due to the heterogeneity of studies and difficulty of quantifying the data, we tabulated key information i.e. the kinds of air-conditioning systems, tested areas and study designs and described relevant parameters in detail. Quantitative and qualitative findings were summarised within each grouping of air-conditioning systems and related quantitative data such as the concentrations of microorganisms found in samples of indoor air were recorded. Associations are presented using the summary measures reported in individual studies with P-values where available
Results
Selection of studies
Figure 1 presents the PRISMA diagram for the screening and selection of articles. A total of 1059 studies were retrieved, of which 299 duplicates and 688 irrelevant studies were excluded often because either they were not reported in English, did not meet the inclusion criteria, or their full texts were not available. As a consequence, 72 studies were assessed for eligibility; 51 were excluded as ancillary disinfection equipment was used along with air-conditioning systems, or only samples taken from air conditioners were tested, or evidence of the effect of air-conditioning systems or comparators was lacking. This process left 21 articles for analysis [Reference Perdelli22–Reference Jiamjarasrangsi42]. Seventeen were cross-sectional studies, three were cohort studies and one was a case−control study. All, but one, were published after 2000 and the other in 1975. Most of the articles were published in internationally recognised and specialised journals; an overview of the articles and their outcomes is presented in Table 1.

Fig. 1. PRISMA diagram of the screening and selection process.
Table 1. General overview of the studies
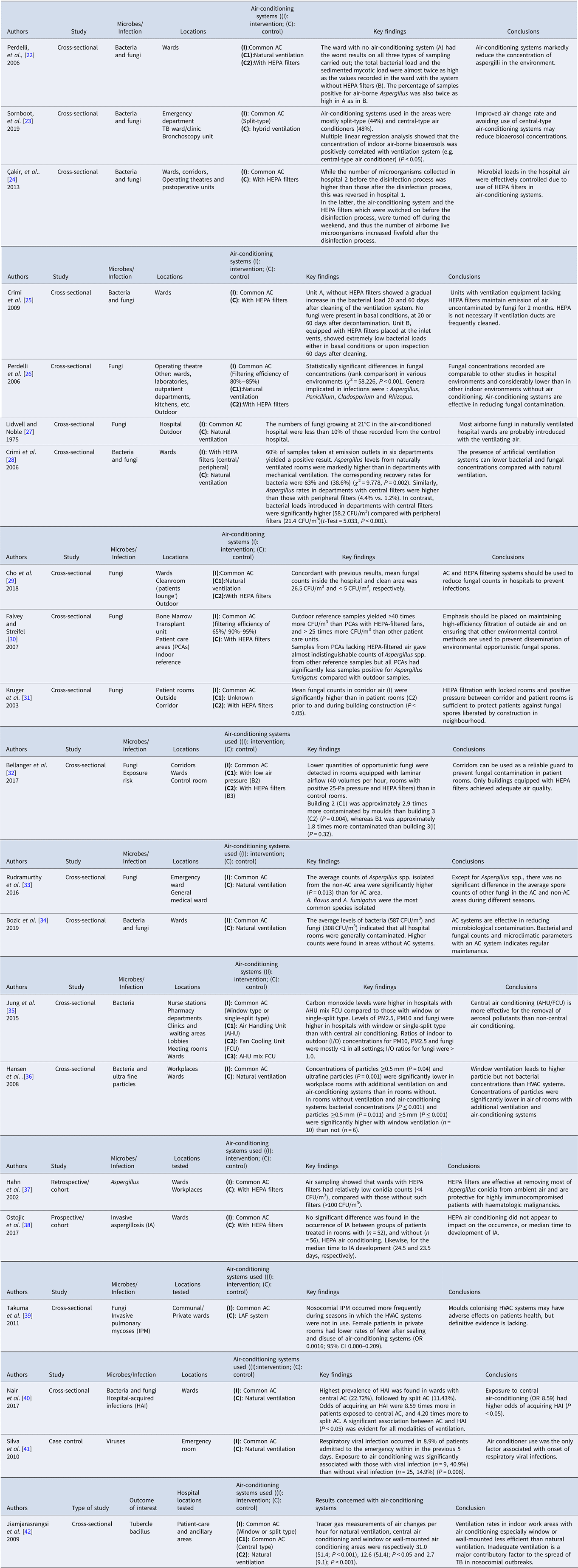
Air-conditioning systems (AC) used in each area were categorised as ‘Common AC,’ ‘Natural ventilation,’ or ‘With HEPA filters’ if other details of ventilation were not given. Specific parameter description such as the type of air conditioner was recorded if given.
Concentration of microorganisms in indoor air
Of the 21 studies included, 16 reported the concentrations of microorganisms in air samples from rooms (wards, corridors, laboratories) with different air-conditioning systems; five of the 16 also sampled outdoor hospital sites. Seven studies [Reference Perdelli22,Reference Perdelli26,Reference Lidwell and Noble27,Reference Cho29,Reference Rudramurthy33,Reference Bozic, Ilic and Ilic34,Reference Hansen36] reported results of microbial concentrations between naturally ventilated, and areas with common air-conditioning systems. In public areas of the hospitals, fungal loads in air-conditioned areas were considerably lower than those recorded in other indoor naturally ventilated environments [Reference Perdelli26,Reference Lidwell and Noble27,Reference Cho29,Reference Rudramurthy33,Reference Bozic, Ilic and Ilic34]. Moreover, the average levels of bacteria were similar to those recorded for fungi [Reference Perdelli22,Reference Bozic, Ilic and Ilic34,Reference Hansen36], the latter being most probably derived from the outdoor environment of the hospital [Reference Lidwell and Noble27].
Air-conditioning systems were further classified into those with, or without HEPA filters in 10 studies. Compared to rooms without air-conditioning or with natural ventilation, indoor airborne fungal and bacterial concentrations were the lowest in rooms with HEPA filters, thus demonstrating their effectiveness for the reduction of bioaerosols [Reference Perdelli22,Reference Cakir24,Reference Perdelli26,Reference Crimi28,Reference Cho29,Reference Kruger31,Reference Bellanger32,Reference Hahn37]. Furthermore, the type of air conditioner used was considered crucial as central air conditioners proved to be more effective than non-centrally sited systems such as window, or single-split types [Reference Jung35,Reference Hansen36]. Notably, one study identified that, compared with hybrid ventilation, the concentration of indoor bioaerosols was positively correlated with the type of ventilation system used (e.g., central air conditioners, P < 0.05) [Reference Sornboot23].
Ratios of viable microorganisms detected
Four studies analysed associations between air-conditioning and the rates of viable microorganisms, mainly Aspergillus, detected on sampling [Reference Perdelli22,Reference Crimi28,Reference Falvey and Streifel30,Reference Takuma39]. The proportion of air samples positive for Aspergillus was consistently much higher in rooms in which the air-conditioning systems were not in use at the time of sampling [Reference Perdelli22,Reference Crimi28,Reference Takuma39]. However, the lowest mean recovery rate, and percentage of samples positive for Aspergillus were recorded in another study in areas with HEPA-filtered air-conditioning systems. In contrast, the samples collected from patient care areas without HEPA-filtered systems and the other reference samples were almost indistinguishable in terms of mean counts of Aspergillus spp. [Reference Falvey and Streifel30].
Related infectious diseases
Five studies (two cross-sectional, two cohort and a case−control) reported outbreaks of related infectious diseases in air-conditioned hospitals. Exposure to central air-conditioning (OR 8.59) had a higher probability of causing hospital-acquired infections (P < 0.05) [Reference Nair40]. In addition, 8.9% of the patients admitted to the emergency room with onset of respiratory symptoms had viral infections, and exposure to air-conditioned air was the only linking factor [Reference Silva41]. Moreover, nosocomial invasive pulmonary mycoses occurred more frequently during seasons in which the HVAC systems were not in use than when they were used [Reference Takuma39]. Only one study reported that the occurrence of invasive aspergillosis (IA) and median time to onset of infection was not significantly different between groups of patients treated in areas with, and without, HEPA-filtered air-conditioning [Reference Ostojic38]. However, another study indicated that HEPA filters were protective for highly immunocompromised patients with haematologic malignancies and were effective for removing most Aspergillus conidia from the ambient air [Reference Hahn37].
Microbe species
Only five studies reported on specific identification of microbial species, mainly fungi, in air-conditioned hospitals. A cross-sectional study in a South Korean hospital [Reference Cho29] assessed the degree of fungal contamination in hospital air environments over the course of a year, and found that Aspergillus spp. were the most prevalent both inside (47.0%) and outside (62.0%) the hospital. Within the hospital, Penicillium spp. were the second most predominant fungi, accounting for 37.9% (n = 25) of the identified species and 8.9% (n = 14) of those found outside (P < 0.001). Overall, the third most common moulds were of the Alternaria genus [Reference Cho29]. Similar results were reported in another cross-sectional study in 10 hospitals by Perdelli et al. [Reference Perdelli26], which found that the mean concentrations of Aspergillus, Penicillium, Cladosporium and Rhizopus, which were implicated in patient's infections, were significantly higher in the kitchens than in other tested areas with HEPA filters in the air-conditioning systems.
In another study, samples of airborne fungi at a tertiary university hospital were collected monthly over 10 years, and all Aspergillus isolates were further categorised into different species, namely, A. fumigatus, A. niger and A. flavus; the latter two species were the most prevalent [Reference Falvey and Streifel30]. Likewise, in another study, A. flavus and A. fumigatus were the most common species isolated in rooms with or without air conditioners. The average number of Aspergillus spp. isolated from the non-air-conditioned rooms was significantly higher than from air-conditioned areas (P = 0.013) [Reference Rudramurthy33].
Indirect factors
Evidence of indirect factors influencing the effectiveness of air-conditioning was provided through a cross-sectional survey of 323 patient care, and ancillary areas, in hospitals of Thailand. This found that indoor ventilation rates (air changes per hour) of areas with central air-conditioning (median, 12.6) were consistently lower than those of work areas with natural ventilation (median, 31.0) (P < 0.001). Furthermore, the ventilation rates of areas with window or wall-mounted air conditioners (median, 2.7) were significantly less than in centrally air-conditioned areas (P < 0.001) [Reference Jiamjarasrangsi42]. Patients in rooms with low ventilation rates might have a higher risk of getting infected by the spread of Mycobacterium tuberculosis [Reference Ehrenkraz and Kicklighter43].
Discussion
Scoping reviews aim to show the primary resource and types of available evidence to provide key concepts for clinical practice, policy formulation and research, especially in an area which has not been reviewed systematically [Reference Arksey and O'Malley21].
In this study, we reviewed the relevant literature to assess how air-conditioning systems affect the incidence and impact of pathogenic microorganisms in the public indoor areas of hospitals. Air-conditioning systems play a more important role than heating or cooling the air in hospitals and other healthcare environments. A hospital is a public setting visited by various kinds of patients from different places. Thus, the issue of microbial contamination related to the use of air-conditioning systems cannot be underestimated, especially given the ongoing COVID-19 pandemic.
The review identified that, in public areas of hospitals, bacterial and fungal bioaerosol concentrations were generally higher in naturally ventilated rooms compared with the degerming effect of central air-conditioned systems which are proven to be effective in removing airborne microbes, although fungal spore levels may remain high in air-conditioned rooms [Reference Rudramurthy33]. The latter reinforces the need for periodical maintenance and disinfection of air-conditioning systems to prevent environmental colonisation and dissemination of fungi [Reference Rudramurthy33,Reference Bozic, Ilic and Ilic34]. Evidence suggests that patients exposed to air-conditioning systems had higher risks of acquiring a viral, or hospital-associated bacterial or fungal infection, the latter potentially causing invasive pulmonary mycoses. Moreover, when air-conditioning systems were in use, doors and windows were often closed to maintain a suitable temperature, which resulted in reduced ventilation rates [Reference Jiamjarasrangsi42]. Likewise, poor design and operation of air-conditioning systems can contribute to inadequate ventilation [Reference Park and Chang44,Reference Cheung and Jim45] and these factors may account for the increase in infection risks when exposed to air-conditioning systems in hospitals. Compared to window or split types of air-conditioning systems, often used in single-patient rooms, recycled central air-conditioning systems were more often installed in multiple-patients' room in a study conducted in a certain hospital in India [Reference Nair40]. Contact between patients and increased movement of personnel may also contribute to higher risk of acquiring hospital infections when exposed to central air-conditioning systems.
To the best of our knowledge, this is the first review in which the influence of high-efficiency filters in air-conditioning systems on the spread of microorganisms has been evaluated. Our key finding is that filters appear to be an indispensable part of air-conditioning systems. Ten of the studies addressed the benefits of HEPA filters in these systems and clearly showed that the concentration of airborne microorganisms in areas with HEPA filters was lower than the concentration in areas without them. However, the included studies did not focus on the non-HEPA filters that are commonly installed inside air conditioners, and few provided details of the operating system, such as pressurisation, humidity, temperature etc. Two studies reported on the efficiency of their non-HEPA filters used in the areas tested [Reference Falvey and Streifel30,Reference Hahn37]. Indeed, only one gave details of the mean temperatures and relative humidity of the natural ventilated areas and in the air-conditioned areas [Reference Cho29]. These factors may be the source of the heterogeneity of data noted in studies that simply classified areas based on the presence of an air conditioner or did not specify the type of air conditioners.
In a workshop summary of the Institute of Medicine (US) Forum on Microbial Threats, HEPA was defined as a pleated mechanical air filter composed of mats of randomly arranged glass fibres that collects and traps particles greater than 0.1 μm by diffusing, intercepting and impacting the passage of particles [Reference Schentag46]. A study conducted in two Wuhan hospitals showed that SARS-CoV-2 aerosols were mainly found in the submicrometer areas (aerosol size distributions between 0.25 and 1.0 μm) and supermicrometer areas (aerosol size distributions > 2.5 μm) [Reference Liu47]. Air filtration through HEPA can intercept most pathogens, including fungi, bacteria and encapsulated viruses, with an efficiency >99.97% [Reference Schentag46]. Although direct studies for SARS-CoV-2 have not as yet been performed, the current study on HEPA filter functionality, and prior CDC guidelines for SARS-CoV-1 together suggest a theoretical efficacy for HEPA filters in eliminating airborne SARS-CoV-2 [Reference Christopherson48].
HEPA filters in air-conditioning systems are widely acknowledged to be highly effective for the removal of microorganisms from the air and protective for high-risk patients. However, owing to their high costs of installation and maintenance, it may prove difficult for healthcare facilities to fit air-conditioning systems with HEPA filters in isolated areas, let alone in public areas. Even in the United Kingdom, only a quarter of 203 hospitals surveyed had isolation facilities available in their emergency departments [Reference Anathallee49]. This situation could only be worse in low-income and developing countries. Nevertheless, a cost-effectiveness incremental analysis showed that for prevention of invasive aspergillosis, rooms with HEPA-filtered systems were better cost-saving interventions than antifungal (posaconazole) prophylaxis and environmental protection measures ($2665 vs. $ 42 531 vs. $4073, respectively) [Reference Combariza50], and thus the economic benefits of such filters can exceed the costs of installation and maintenance.
For areas where HEPA filters are currently not available, possible substitutes to improve air hygiene are: lamps with germicidal ultraviolet irradiation, increasing room ventilation rates, and less widely applied, generation of hydrogen peroxide mists stabilised with silver ions [Reference Ryan51–Reference D'Orazio and D'Alessandro56]. Microbial contamination of room air and risks of transmission can be reduced to a minimum by regular implementation of disinfection measures. For hospitals in poor areas or with inadequate external air quality, mobile air-decontamination units and portable HEPA filtration units are alternative options and are easy to maintain [Reference Brenier-Pinchart57,Reference Boswell and Fox58].
This scoping review has some limitations. First, all the included studies reported different descriptions of the air-conditioning systems used, which may be responsible for differences in their conclusions. Second, although several studies provided seemingly detailed descriptions of the air sampling methods used, variables in the experimental set-up were not described. Details of the sampling time, and the position and height of the sampler when samples were taken, were generally imprecise or not reported. Third, locations of the hospitals, humidity, temperature and season have recognised impacts on microbial contamination of indoor air [Reference Cakir24,Reference Cho29,Reference Limaylla, Silva and Castelo Branco Fortaleza59]; these factors were considered in relatively few of the studies. Lastly, as standard deviations of microbe concentrations were reported inconsistently, the data presented may therefore be an underestimation of reality since the sampled areas were not randomly selected. Further, specific microorganisms in various settings were assessed based on selective sampling and reliance on existing techniques; thus, other microbes in the air and on surfaces might have been overlooked. Nevertheless, we consider that these limitations do not affect the validity and conclusions of the study.
In conclusion, this study focused on ventilation of hospital public areas, which are more likely to be overlooked relative to operating room and ICUs, and reviewed evidence regarding the risk of air-conditioning systems and hospital-acquired infections. The cleaning and maintenance of such systems should be done regularly according to existing standards as patients residing in contaminated air in rooms have a higher risk of exposure to pathogenic microorganisms. The universal installation of HEPA filters can effectively mitigate against microbial contamination and constitute a protective measure for patients. These findings may help improve management of air-conditioning systems during a pandemic. Future studies should attempt to assess multiple air-conditioning parameters during operational hours with quantitative and qualitative measurements of temperature, relative humidity and ventilation rates.
Strengths and limitations of this study
• Systematic methods were used to provide a comprehensive review of effects of air-conditioning systems and HEPA filters on the transmission of pathogenic microorganisms and related diseases.
• This study focused on hospital public areas, which are more likely to be overlooked relative to areas such as the operating room and ICU.
• Only articles published in English were included in this study.
Supplementary material
The supplementary material for this article can be found at https://doi.org/10.1017/S0950268821001990
Acknowledgments
We would like to express our gratitude to all authors who provided data for our scoping review. Moreover, we would like to thank Editage (www.editage.cn) for English language editing.
Author contributions
HTW and QSL contributed equally to this work and should be considered co-first authors; HTW and QSL did the conceptualisation,; CHJ, WM and SL contributed to methodology; investigation was conducted by HTW and QSL; supervision by RCD and LW; writing and original draft preparation by HTW and RCD and writing, review and editing by CHJ, HTW, QSL and SL. All authors read and approved the published version of the manuscript.
Financial support
This work was supported by the Key R & D projects from the Department of Science and Technology of Zhejiang Province (No. 2020C03126), the Health Commission of Zhejiang Province (No. 2017KY502), and the Administration of Traditional Chinese Medicine of Zhejiang Province (No. 2017ZZ007, 2020ZB095 and 2021ZA047), the People's Republic of China.
Conflict of interest
The authors declare no conflict of interest.
Informed consent statement
Not applicable.
Data availability statement
Data available on request due to restrictions.