Introduction
Protected areas (PAs) are crucial for ecosystem conservation and resilience to climate-related impacts. Reducing carbon dioxide emissions, building carbon stocks, buffering environmental changes (Walker et al. Reference Walker, Moore, Arima, Perz, Simmons and Caldas2009, Jantz et al. Reference Jantz, Goetz and Laporte2014) and maintaining ecosystem productivity for water and food provision are among the main contributions of PAs to climate change adaptation (Dudley et al. Reference Dudley, Stolton, Belokurov, Krueger, Lopoukhine and MacKinnon2010). Furthermore, Chapter 3 of the Intergovernmental Panel on Climate Change (IPCC) fifth assessment report highlighted the importance of protecting and restoring freshwater habitats as adaptive measures that benefit climate change adaptation planning (Jiménez Cisneros et al. Reference Jiménez Cisneros, Oki, Arnell, Benito, Cogley, Döll, Field, Barros, Dokken, Mach, Mastrandrea and Bilir2014). Nevertheless, PAs such as national parks have been based principally on the needs of terrestrial ecosystems and much less on those of freshwater ecosystems (Azevedo-Santos et al. Reference Azevedo-Santos, Frederico, Fagundes, Pompeu, Pelicice and Padial2019, Acreman et al. Reference Acreman, Hughes, Arthington, Tickner and Dueñas2020). The absence of a watershed approach, the lack of large rivers representation and the absence of river connectivity protection for migratory species are among the main reasons for the weak effectiveness of freshwater ecosystem conservation by PAs (Acreman et al. Reference Acreman, Hughes, Arthington, Tickner and Dueñas2020). Although the Convention on Biological Diversity (CBD) included a conservation target of 17% of terrestrial and inland water areas in the Aichi Biodiversity Targets (Convention on Biological Diversity 2010), at the global scale, c. 70% of river reaches have no PAs in their upstream catchments, only 11% achieve full integrated protection and the average surface area of protection of the largest basins remains below 10% (Abell et al. Reference Abell, Lehner, Thieme and Linke2017). Furthermore, other actions such as the Convention on Wetlands have emerged due to the need for wetland conservation; currently, there are 2404 wetlands (total area 2 543 391.59 km2) with Ramsar designation worldwide (Ramsar 2020).
Approximately 40% of mountain ranges worldwide do not contain any PAs, and 75% of mountain ranges have less than half of their elevational gradients covered by the strict International Union for Conservation of Nature (IUCN) categories of PAs (Elsen et al. Reference Elsen, Monahan and Merenlender2018). This highlights the need to increase protection across elevational gradients, especially in sites where high impacts of warming are predicted. The tropical Andes are highly sensitive to climate change, as it is predicted that temperature will increase by more than 4°C in the tropics, especially at high-elevation sites, due to their geographical position and steep elevational gradients (Bradley et al. Reference Bradley, Vuille, Diaz and Vergara2006, Pepin et al. Reference Pepin, Bradley, Diaz, Baraer, Caceres and Forsythe2015). Increasing warming has negative impacts on freshwater resources. For instance, rapid mountain glacier retreat and changes in precipitation patterns have resulted in hydrological changes – droughts in some places and floods in others – affecting millions of people in the tropical Andes (Baraer et al. Reference Baraer, Mark, McKenzie, Condom, Bury and Huh2012, Satgé et al. Reference Satgé, Espinoza, Zolá, Roig, Timouk and Molina2017, Vuille et al. Reference Vuille, Carey, Huggel, Buytaert, Rabatel and Jacobsen2018). Furthermore, species distribution shifts to upper elevations of mountains due to increasing warming and/or new habitats created by glacier retreat (Seimon et al. Reference Seimon, Seimon, Yager, Reider, Delgado and Sowell2017, Zimmer et al. Reference Zimmer, Meneses, Rabatel, Soruco, Dangles and Anthelme2018) call for ecosystem protection across different elevational gradients. Therefore, assessing the role of PAs in freshwater ecosystem conservation in the tropical Andes will provide an important benchmark and offer insights into climate change adaptation and remaining gaps in understanding.
We address this issue by reviewing the literature and analysing maps related to freshwater ecosystems covered by PAs in the tropical Andes. We have three research questions: (1) Do the objectives of PAs consider freshwater ecosystems conservation in the tropical Andes? (2) To what extent are mountain freshwater ecosystems protected by PAs in the tropical Andes? (3) To what extent are ecosystem services and biodiversity safeguarded by current protection efforts? The meaning of ‘protection’ can be complex, and for freshwater ecosystems protection can include a variety of actions, such as establishing new PAs and sustainable management measures to maintain river connectivity or water quality, among others. Here, the meaning of ‘protection’ is based on the PA definition of the IUCN: a PA is a clearly defined geographical space, recognized, dedicated and managed, through legal or other effective means, to achieve the long-term conservation of nature with associated ecosystem services and cultural values (Dudley et al. Reference Dudley, Shadie and Stolton2013).
Methods
We conducted a literature review and assembled novel databases to analyse and produce maps of freshwater protection by PAs (including lakes, streams and watersheds with glaciers). For the literature review, we applied a systematic method by employing eligibility criteria and a protocol to strengthen the transparency, accuracy and completeness of reports (Moher et al. Reference Moher, Liberati, Tetzlaff and Altman2009), as in some other environmental studies (McDowell et al. Reference McDowell, Huggel, Frey, Wang, Cramer and Ricciardi2019, Acreman et al. Reference Acreman, Hughes, Arthington, Tickner and Dueñas2020). The map analysis drew on databases of leading institutions: the IUCN, the World Wildlife Fund (WWF), the Critical Ecosystem Partnership Fund (CEPF) and the National Snow and Ice Data Center (NSIDC).
Literature review
First, we designed a protocol considering our research questions, spatial and temporal scopes and languages. The study was limited to the tropical Andes hotspot, a defined area with exceptional concentrations of endemic species and habitat loss, whose boundaries have been determined by biological commonalities (Myers et al. Reference Myers, Mittermeier, Mittermeier, da Fonseca and Kent2000). For question (1), related to the objectives of the creation of PAs, we reviewed literature published since 1990. For questions (2) and (3), we reviewed literature published since 2014. We considered mainly articles written in English obtained from Web of Science and Scopus, as well as research articles and reports from non-governmental organizations (NGOs) and governmental institutions in Spanish (e.g., national systems of PAs) available online. The criteria for choosing literature items were: research articles and reports of leading institutions; documents that answered each research question in part or in full (e.g., papers that assessed the role of PAs in freshwater protection directly and/or papers that quantified freshwater ecosystems within a PA); and information on current issues regarding freshwater ecosystems and biodiversity conservation, such as climate change and habitat disruption, which highlighted the importance of the role of PAs in addressing these issues.
We conducted the literature review in a stepwise fashion. First, we produced 24 string codes with keywords on the topic for the literature search (Supplementary Table S1, available online), resulting in 1341 selected articles. Second, we verified the criteria in the titles, then in the abstracts and finally in the full documents, resulting in 359, 269 and 130 articles without duplicates, respectively. Third, we summarized each document in order to separate the information directly or indirectly related to the topic, resulting in 32 research articles that were finally selected. In addition, we included grey literature from Google Scholar and governmental institutions and NGOs in order to better answer the research questions. The grey literature review resulted in 15 documents being selected (13 management plans of PAs and 2 reports). We cross-checked from selected research articles to ensure that relevant research articles were considered in the analysis. This resulted in the inclusion of 5 research papers, leading to a grand total of 52 documents (37 research articles, 13 management plans and 2 NGO reports; Fig. S1).
Maps of freshwater ecosystems and PAs
We overlaid PA coverages on freshwater systems layers (i.e., lakes, glacial lakes, streams and watersheds) to quantify freshwater ecosystem protection using Geographic Information System Tools. The tropical Andes hotspot layer was obtained from the CEPF (Hoffman et al. Reference Hoffman, Koenig, Bunting, Costanza and Williams2016). The PA information was obtained from the World Database on Protected Areas (WDPA; https://www.protectedplanet.net), which includes shapefiles and a robust attribute tables for each PA with IUCN categories and national levels of protection. Shapefiles of freshwater ecosystems (i.e., lakes, rivers and watersheds) were obtained from the Hydrological data and maps based on SHuttle Elevation Derivatives at multiple Scales (HydroSHEDS; Lehner et al. Reference Lehner, Verdin and Jarvis2008; http://www.hydrosheds.org). The HydroLAKES database provides information on global lakes and reservoirs and was designed as a digital map repository to include all lakes with a surface area of at least 0.1 km2 (Messager et al. Reference Messager, Lehner, Grill, Nedeva and Schmitt2016). In our study, we considered only lakes for analysing lake protection. Layers of glaciers and glacial lakes came from the NSIDC: Global Land Ice Measurements from Space (GLIMS & NSIDC 2018) and the High Mountain Asia Near-Global Multi-Decadal Glacial Lake Inventory, Version 1 (Shugar et al. Reference Shugar, Burr, Haritashya, Kargel, Watson and Kennedy2020). We selected glacier coverages of the Randolph Glacier Inventory from the GLIMS database. Furthermore, we verified Argentinean glaciers from the Argentinian Glacier Inventory (Zalazar et al. Reference Zalazar, Ferri, Castro, Gargantini, Gimenez and Pitte2020) and the Atlas of Argentinean Glaciers (http://www.glaciaresargentinos.gob.ar), which are also available in the GLIMS database. We also quantified Ramsar sites (data from https://rsis.ramsar.org), which are PAs that receive the Ramsar designation after meeting criteria such as sites containing representative, rare or unique wetland types. They are compatible with all IUCN categories of PAs (Dudley et al. Reference Dudley, Shadie and Stolton2013).
Before quantifying the freshwater ecosystem protection, we selected PAs based on the IUCN classification because it facilitates comparisons among countries (Dudley et al. Reference Dudley, Shadie and Stolton2013). Definitions of each IUCN category are available in Table S4. The preliminary assessment of the maps showed a total of 1330 PAs that cover 33% of the total surface area of the tropical Andes (1 550 539 km2). Of the total area, 0.01%, 8.20%, 0.33%, 0.58%, 3.18% and 5.03% were protected by the IUCN categories Ia, II, III, IV, V and VI, respectively, while 4.24%, 0.08% and 11.15% of the total area were under no category of protection (the not applicable, assigned and not reported categories, respectively; Fig. S2). For our study, we selected only IUCN categories from Ia to IV, as they represent the highest levels of protection from national governments. All PAs from Ecuador and Bolivia were classified as ‘Not Reported’ (149 PAs; Fig. S2c), meaning that either the IUCN category was unknown and/or the data provider had not provided any related information. Thus, for these countries, we selected PAs under the national systems of PAs: Servicio Nacional de Áreas Protegidas (SERNAP) for Bolivia and the Sistema Nacional de Áreas Protegidas (SNAP) for Ecuador (Fig. S3). Then, protected freshwater ecosystems were quantified by overlapping the layers of selected PAs onto lakes and streams. We analysed stream orders using the Strahler stream order to provide an indication of the stream-type protection (i.e., ephemeral, perennial or intermittent), as well as about how much of the headwater and lowland streams had been covered by PAs (Hansen Reference Hansen2001, Biggs et al. Reference Biggs, von Fumetti and Kelly-Quinn2017). Moreover, for quantifying the number and surface area of glacier system protection (i.e., lakes, watersheds with glaciers and glaciers), we overlaid PA layers onto the layers of glaciers, glacial lakes and watersheds. For quantifying the watershed protection covered by glaciers, we calculated the number of watersheds covered by glaciers and PAs.
Results
Of the 52 documents selected for the literature review, 44.2% (13 management plans and 10 research articles) were related to the inclusion of water resources conservation in the objectives of the PAs’ creation and related to the creation of local PAs. Moreover, 25.0% (13) and 17.3% (9) of the documents were related to freshwater ecosystem inventories and aquatic biodiversity in PAs and climate-related impacts, respectively. Furthermore, 13.5% (7) of the documents were related to ecosystem services in PAs (Table S2).
Do the objectives of PAs consider freshwater ecosystem conservation in the tropical Andes?
Creation of PAs and water resources conservation
Most PAs worldwide were not created for freshwater conservation (Dudley et al. Reference Dudley, Shadie and Stolton2013). In the Andean region, Messerli et al. (Reference Messerli, Grosjean and Vuille1997) published the first study about the importance of including water resources protection in policy to avoid conflicts related to water scarcity. They presented a map with 60 PAs and suggested that more water PAs should be created, especially in arid places with less than 500 mm of annual precipitation. In the grey literature, we found 7 national parks out of the 16 assessed with biodiversity and water resources conservation among the main objectives of their creation (Table S3). For instance, Tunari National Park in Bolivia was created for hydrological protection and forest conservation (Table S3). In Ecuador, Podocarpus National Park was created for forest and freshwater ecosystem protection (Table S3). In the same country, the Pro-Cuencas Podocarpus Fund aimed to conserve water quantity and quality by reducing pollution by 25% and reforesting 10% of the watersheds (Redondo-Brenes Reference Redondo-Brenes2009). In Peru, the Río Abiseo National Park was created to protect humid forest protection and to maintain the hydrological stability of catchments (Young Reference Young1993). In Chile, Lauca National Park covers three volcanic cones, lakes and peatlands. This park and its neighbours, Las Vicuñas National Reserve and the National Monument Salar de Surire, form the Lauca Biosphere Reserve (Rundel & Palma Reference Rundel and Palma2000). Furthermore, we found four PAs with unclear objectives and/or that were not created for freshwater protection but the management plans highlighted the need for water resources protection. Additionally, we found five PAs with high values for glacier and freshwater conservation (Table S3). Tuni Condoriri National Park in Bolivia was the most relevant case because no management plan has yet been initiated, despite the fact that the park includes high-value glaciated mountains that provide drinking water to millions of people in the cities of La Paz and El Alto (Hoffmann & Oetting Reference Hoffmann, Oetting and Leal Filho2011).
Creation of local PAs
We found six studies on the creation of local PAs for water resources. In Peru, the regional Government of San Martin created the Rumialba Ecological Recovery and Conservation Zone (ZOCRE) of 23.96 km2 covering the upper watersheds of the Rumiyacu, Mishquiyacu and Almendra rivers, which provide drinking water to c. 50 000 people (Montoya-Zumaeta et al. Reference Montoya-Zumaeta, Rojas and Wunder2019). In the same country, stakeholders of the Piura community agreed to a programme to protect a large watershed spanning from the Andes to the Pacific coast through the Regional Fund for Water and Sanitation (FORASAN). Among their main activities were the establishment of private PAs such as Cuyas and Samanga (Ostovar Reference Ostovar2019). In Ecuador, Iñiguez Gallardo et al. (Reference Iñiguez Gallardo, Helsley, Pinel, Ammon, Lopez Rodriguez and Wendland2013) assessed the governance of a proposed Ramsar wetland (Saraguro–Oña–Yacuambi) containing several lakes (e.g., Laguna Grande and Tres Lagunas) and located within the PA of Shincata Protected Forest and the municipal Yacuambi Natural Reserve. In Ecuador as well, payment for ecosystem services has been one of the ways by which to address water resources protection. For instance, citizens and NGOs initiated payment to rural communities of the Antisana and Cayambe–Coca ecological reserves to guarantee water provision, estimated to be c. 80% of the supply for Quito (Joslin Reference Joslin2020). In Colombia and Venezuela, Leroy (Reference Leroy2019) assessed the perceptions of two local communities regarding water scarcity and climate change indicators (e.g., disturbance of the seasons); Venezuelan farmers adopted irrigation systems and actions to protect wetlands by establishing protection boundaries, especially after a severe drought occurred.
To what extent are mountain freshwater ecosystems protected by PAs in the tropical Andes?
PAs, lake and glacial lake inventories
We found that 4% (581 km2) of the total lake area (13 716 km2), representing a volume of 4.4 km3, was covered by PAs in the tropical Andes (Fig. 1a–c), and the majority of lakes (2188 lakes, 88%) were not covered by PAs (Fig. 1d). Additionally, 31% (45) of glacial lakes were covered by PAs (Fig. 3d), representing 29% (15 km2) of the total surface area of glacial lakes.
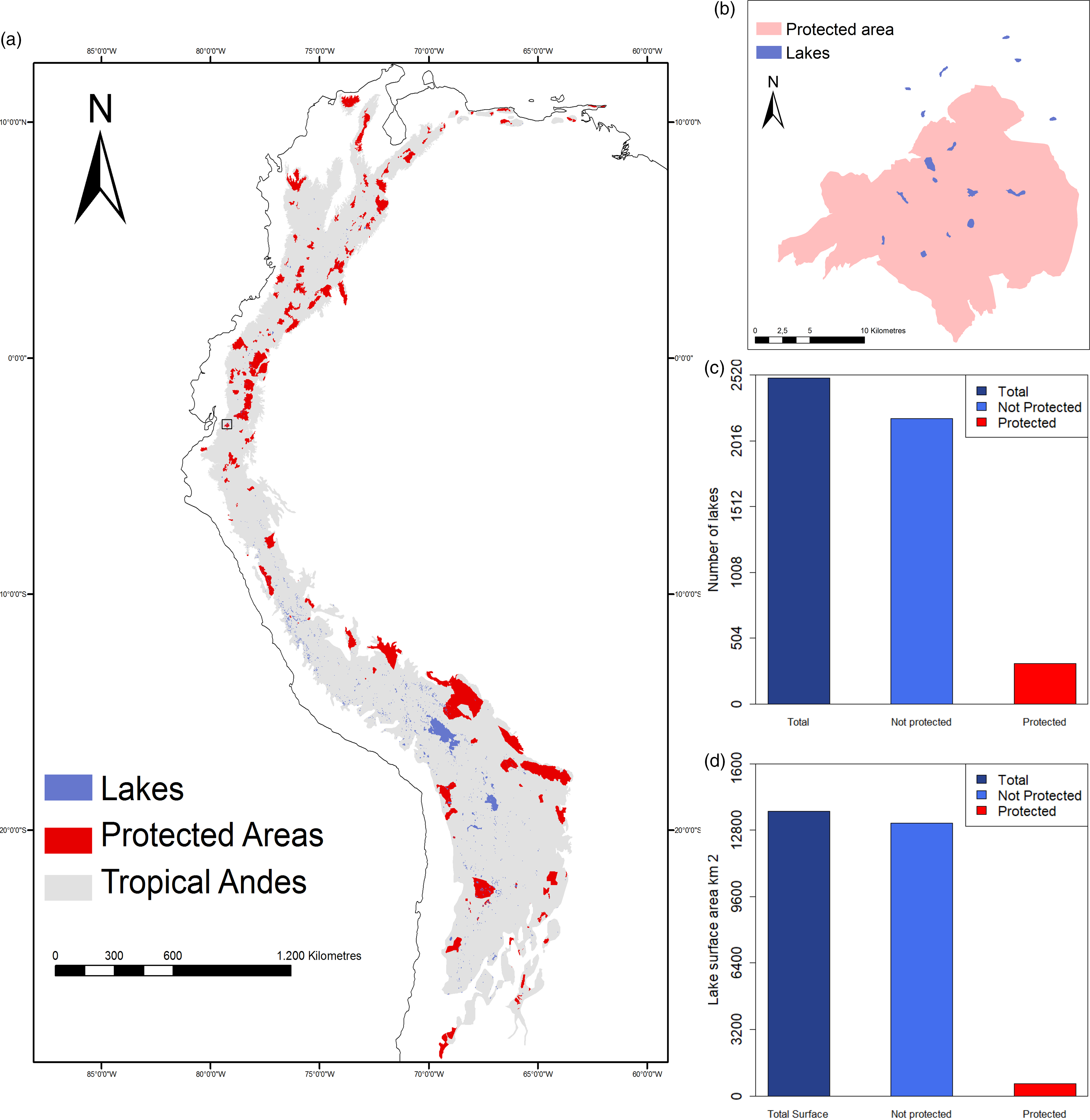
Fig. 1. (a) Protected areas (PAs) and lakes in the tropical Andes. The PA in the black rectangle is Cajas National Park, which is depicted in (b) for visualization of the lake distribution inside of the park. (c) Total number of PA protected and unprotected lakes. (d) Total surface area of PA protected and unprotected lakes.

Fig. 2. (a) Protected areas (PAs) and streams in the tropical Andes. Only a portion of the tropical Andes is shown, and only streams of fourth order and higher are depicted for better visualization. Stream orders are based on the Strahler stream order. The PA within the black rectangle is the Llanganates National Park, which is depicted in (b). (c) Total number of PA protected streams sorted by stream order. (d) Stream length in relation to stream order. Error bars show standard errors.

Fig. 3. (a) Protected areas (PAs), glacial lakes, glacier cover and watershed number covered by glaciers and PAs in circles at the national scale (watershed average surface area = 631 km2). Only 110 watersheds covered with glaciers are shown as the remainder were shared among different countries. The PA within the black rectangle is the Cocuy National Park, which is depicted in (b). (c) Total surface area of PA protected and unprotected glacier cover. (d) Total number of PA protected and unprotected glacier lakes in the tropical Andes.
In our literature review, we found two national park inventories of lake areas. A more detailed inventory reported 202 lakes and 5955 water bodies in the Cajas National Park, Ecuador. Lakes larger than 104 m2 constituted 85% of superficial water in the park, and 10 lakes deeper than 18 m contained 50% of the water sources (Mosquera et al. Reference Mosquera, Hampel, Vázquez, Alonso and Catalan2017). Similarly, Polk et al. (Reference Polk, Young, Baraer, Mark, McKenzie, Bury and Carey2017) reported lakes ranging in area from 0.66 to 16.29 km2 between 1987 and 1995 in the Huascaran National Park, Peru.
We did not find studies on the role of PAs in addressing climate-related impacts on freshwater ecosystems, only studies related to water losses by evaporation and the increase in the number of lakes due to glacier retreat in the tropical Andes. For instance, higher evaporation rates (1700 mm year–1) over Lake Titicaca were reported (Pillco Zolá et al. Reference Pillco Zolá, Bengtsson, Berndtsson, Martí-Cardona, Satgé and Timouk2019). Moreover, 201 sites might become lakes in the future due to glacier retreat in Peruvian cordilleras (Colonia et al. Reference Colonia, Torres, Haeberli, Schauwecker, Braendle, Giraldez and Cochachin2017). Similarly, glacier recession between 1986 and 2014 in the Bolivian cordilleras increased the number of proglacial lakes by 47% in the Cordillera Real (from 92 to 135 lakes) and by 72% in the Cordillera Apolobamba (from 29 to 50 lakes; Cook et al. Reference Cook, Kougkoulos, Edwards, Dortch and Hoffmann2016). Similarly, glacier retreat in the Peruvian Vilcanota–Urubamba basins augmented the lake area and number from 23.3 km2 (460 lakes) in 1988 to 26.9 km2 (544 lakes) in 2016, and future lake areas could grow by between 3.2% and 6.0% under IPCC scenarios Representative Concentration Pathway (RCP) 2.6 and RCP 8.5, respectively (Drenkhan et al. Reference Drenkhan, Guardamino, Huggel and Frey2018).
PAs, streams and watersheds with and without glaciers
Based on our map analysis, the total stream length covered by PAs was 55 229 km, representing only 12% of the total length (Fig. 2a). Stream orders from 1 to 7 were covered by PAs, with lengths ranging from 2.5 to 4.0 km (Fig. 2a & b). We observed a high number of first-order streams, of which 14% were covered by PAs. Seventh-order streams were even less covered by PAs (6%; Fig. 2c). Thus, small streams such as headwaters and ephemeral streams seem to be better protected by PAs than larger streams and rivers, but the percentages remain low.
One study quantified the protection of streams and catchments by PAs (Thieme et al. Reference Thieme, Lehner, Abell, Hamilton, Kellndorfer, Powell and Riveros2007), including the longitudinal and lateral connectivity of drainage basins, ranging from high elevations in the Andes to lowland sites in the Amazon. PAs covered 10% (1259 km2) of the high-elevation streams within a buffer area of 10 km2, but without representation of longitudinal and lateral connectivity.
We did not find studies on glacier streams and catchments protected by PAs. In our maps, we observed that 40% of the total glacier surface area (2407 km2) was covered by PAs (Fig. 3c), which resulted in 120 watersheds with glaciers (average surface area of 631 km2) covered by PAs, representing 29% of watersheds with glaciers in the tropical Andes (Fig. 3a & b).
Ramsar sites in the tropical Andes
We found four research articles assessing the extent of and future changes to wetlands. Ramsar sites are increasing in number and surface area, resulting in 113 sites with a total area of c. 373 000 km2 in South America, but more specific national policies and monitoring are needed (Wittmann et al. Reference Wittmann, Householder, Wittmann, Lopes, Junk and Piedade2015). We quantified 31 Ramsar sites that occupy 60 232.6 km2 (4%) of the tropical Andes (Table 1).
Table 1. Ramsar sites and surface areas (km2) in the tropical Andes based on the Ramsar sites information databases (https://rsis.ramsar.org).

The bofedales (peatlands) in Peru were designated as a Ramsar site in 2003 (Ramsar Site No. 1317). In Ecuador, bofedales cover 205.18 km2 (39%) of the total area of the Chimborazo Fauna Production Preserve, at 3800–6268 m altitude (Jara et al. Reference Jara, Delegido, Ayala, Lozano, Armas and Flores2019). In Peru, a total peatland area of 384.44 km2 represents 11% of the area of the Huascarán National Park (Chimner et al. Reference Chimner, Bourgeau-Chavez, Grelik, Hribljan, Clarke and Polk2019). Moreover, Otto and Gibbons (Reference Otto and Gibbons2017) assessed peatland area responses under 2 precipitation scenarios in 17 watersheds located inside and outside of the Salinas y Agua Blanca National Reserve. Projections indicate that annual rainfall decrease will result in total wetland loss by the end of the twenty-first century for watersheds with 200–500 mm of annual mean rainfall and mainly inside of the PA. In Sajama National Park of Bolivia, bofedales have declined in area from 34 km2 in 1986 to 22 km2 in 2016, whereas dry mixed grasses increased from 5.1 to 20.3 km2 (Yager et al. Reference Yager, Valdivia, Slayback, Jimenez, Isela Meneses and Palabral2019). Stakeholders also perceived a likely reduction in ecosystem services provision by peatlands (e.g., water storage) over the next 20 years in Salinas y Aguada Blanca National Reserve, Peru (Ibáñez Blancas et al. Reference Ibáñez Blancas, La Torre-Cuadros and Mallma Carrera2018).
To what extent are ecosystem services and biodiversity safeguarded by current protection efforts?
PAs, freshwater biodiversity and impacts
One of the most comprehensive reports on the distribution, extinction risk and climate change vulnerability of freshwater biodiversity in the tropical Andes and Amazon is that of Tognelli et al. (Reference Tognelli, Lasso, Bota-Sierra, Jiménez-Segura and Cox2016). It includes 9 freshwater ecoregions, from Bolivia to Colombia, and 967 species in 4 taxonomic groups: fishes, molluscs, dragonflies and aquatic plants. The study identified 86 key biodiversity areas within 22 PAs and 39 catchment management zones, and it proposed 25 new freshwater PAs to include 151 threatened species. Furthermore, Tognelli et al. (Reference Tognelli, Anderson, Jiménez-Segura, Chuctay, Chocano and Maldonado-Ocampo2019) reported that 571 endemic fish species (88%) were not within any PA, and they identified c. 475 catchments as very high priority for biodiversity conservation, but only 2% were covered by PAs. Furthermore, Miranda et al. (Reference Miranda, Tobes, Gaspar and Peláez-Rodríguez2018) reported fish distribution in clusters at the Suaza River (Colombia), with a clear distinction in the communities at the headwater sites (within the Cueva de los Guácharos National Park) due to the low level of human intervention. Based on the distribution of 481 vertebrates (including fishes) and 54 invertebrates across elevational gradients in an Ecuadorian Andean–Amazon basin and covering 6 PAs, Lessmann et al. (Reference Lessmann, Guayasamin, Casner, Flecker, Funk and Ghalambor2016) reported that lowlands (<600 m) are diverse in vertebrate species, whereas mid-elevations (600–1600 m) are diverse in invertebrates, suggesting the need for fluvial corridors for sustaining species migration. On the other hand, an analysis of the conservation of 132 aquatic macroinvertebrate species within PAs from the tropical Andes to the dry Chaco reported that only 0.009% of the macroinvertebrate distribution was within the PAs (Nieto et al. Reference Nieto, Ovando, Loyola, Izquierdo, Romero and Molineri2017).
Regarding climate-related impacts, Tognelli et al. (Reference Tognelli, Lasso, Bota-Sierra, Jiménez-Segura and Cox2016) reported aquatic plants as the most vulnerable to climate change, followed by fishes, under the IPCC scenario RCP 4.5. Climate change will likely reduce the dispersion of most of the Andean–Amazon fish species, especially those inhabiting the highlands due to the additional effects of fragmentation (Herrera et al. Reference Herrera, Oberdorff, Anderson, Brosse, Carvajal-Vallejos and Frederico2020). Currently, 142 dams have impacted the connectivity of six major Andean–Amazon river basins, and future new dams will likely impact the connectivity of 5 basins and 671 fish species (Anderson et al. Reference Anderson, Jenkins, Heilpern, Maldonado-Ocampo, Carvajal-Vallejos and Encalada2018). On the other hand, lakes in Cajas National Park (Ecuador) have started to become thermally stratified, resulting in plankton community shifts and with likely impacts on higher trophic levels such as fishes (Labaj et al. Reference Labaj, Michelutti and Smol2017, Reference Labaj, Michelutti and Smol2018a, Reference Labaj, Michelutti and Smol2018b).
PAs and ecosystem services
Fisheries and organic matter decomposition
One study assessed a PA role in organic matter decomposition by aquatic invertebrates, where leaf litter decomposition inside of Cajas National Park (Ecuador) was three times faster than outside of the PA (Rincon et al. Reference Rincon, Merchan, Sparer, Rojas and Zarate2017). One study on fishery resources in Ramsar sites of the Andes reported a biomass production of 49 631 tonnes by the year 2000 of the native species Orestias ispi (Vila et al. Reference Vila, Pardo and Scott2007).
Carbon fluxes
Carbon flux studies have been conducted mainly in bofedales, which are carbon dioxide sinks because organic matter production is greater than decomposition (Hribljan et al. Reference Hribljan, Suárez, Heckman, Lilleskov and Chimner2016). Carbon stocks of 1 040 000 and 572 000 kg ha–1 were reported for the bofedales of Sajama National Park and Tuni in Bolivia, respectively (Hribljan et al. Reference Hribljan, Cooper, Sueltenfuss, Wolf, Heckman, Lilleskov and Chimner2015). Across hydrological gradients, undrained bofedales within the Huascaran National Park in Peru are likely to be carbon sinks, and highly drained peatlands are carbon sources (Planas-Clarke et al. Reference Planas-Clarke, Chimner, Hribljan, Lilleskov and Fuentealba2020).
Water supply
In the grey literature, we found that the city of Tarija (Bolivia) gets 70% and 30% of its surface water and groundwater, respectively, from the La Vitoria watershed, which is protected by Cordillera de Sama National Park (Brown Reference Brown2005). Other studies have assessed the water supply by forests in PAs (Ramos Franco & Armenteras Pascual Reference Ramos Franco and Armenteras Pascual2019, Fastré et al. Reference Fastré, Possingham, Strubbe and Matthysen2020). However, we did not find studies that assessed the direct role of PAs in water supply.
Discussion
Knowledge gaps and future studies
Our review has focused on PAs because they are key instruments in the creation of environmental policies for ecosystem conservation and climate change adaptation. We synthesized evidence of the role of PAs in freshwater ecosystem conservation in the tropical Andes, including ecosystems at very high elevation. Addressing the following knowledge gaps would contribute to freshwater conservation and climate change adaptation.
The creation of PAs
PAs have not been designed for the protection of large rivers and watersheds, and they mainly protect small freshwater ecosystems (e.g., small headwater catchments and streams). Furthermore, the creation of local PAs highlights the need to maintain the water supply. Therefore, it is important to know more about the creation of specific PAs for freshwater conservation, which may have been based on very local decisions (e.g., by rural communities and private sectors; Hora et al. Reference Hora, Marchant and Borsdorf2018). Mapping these local PAs and other adaptation measures that explore nature-based solutions, including the protection and restoration of freshwater ecosystems to prevent climate-related risks such as hydrological changes (Hartman et al. Reference Hartman, Bookhagen and Chadwick2016), could provide a well-rounded assessment of current climate change adaptation and water provision efforts. Additionally, more effective efforts are needed for PAs without management plans and with high values for glacier protection.
Freshwater ecosystems covered by PAs
To our knowledge, this review is the first to combine information about freshwater systems and PAs, including glaciated systems, in the tropical Andes. Our mapping quantified lower percentages of lakes, glacier lakes, streams and glaciers covered by PAs than the 17% of the Aichi protection target (Convention on Biological Diversity 2010). However, we might have underestimated or overestimated the real conditions as we used global inventories and because freshwater ecosystems and glaciers are dynamic and changing in response to climatic conditions (Baraer et al. Reference Baraer, Mark, McKenzie, Condom, Bury and Huh2012, Vuille et al. Reference Vuille, Carey, Huggel, Buytaert, Rabatel and Jacobsen2018). Therefore, more detailed inventories within and around PAs are needed for the better design of freshwater conservation. Future lake inventories might include climate-related impacts such as the increase in the number of lakes due to glacier retreat (Drenkhan et al. Reference Drenkhan, Guardamino, Huggel and Frey2018) and water evaporation (Satgé et al. Reference Satgé, Espinoza, Zolá, Roig, Timouk and Molina2017). However, PAs alone or the increase in the area of PAs might not be sufficient to guarantee freshwater ecosystem conservation and to cope with climate change as in terrestrial ecosystems (Possingham et al. Reference Possingham, Bode and Klein2015, Pringle et al. Reference Pringle2017). The achievement of long-term freshwater conservation and climate change adaptation requires strong cooperation among stakeholders and local and regional governments in order to put in place effective and sustainable management measures of freshwater ecosystems within PAs.
PAs, aquatic biodiversity and ecosystem services
Biodiversity conservation within PAs has been assessed mainly for fishes across the Andean–Amazon region, while few studies have been performed on the western Andean slope and at high-elevation sites (>4000 m altitude). We have provided a benchmark of the current situation, but more research is needed for the habitat conservation planning of other aquatic groups (e.g., benthic invertebrates; Crespo-Pérez et al. Reference Crespo-Pérez, Dangles, Ibarra, Espinosa, Andino, Jacobsen and Cauvy-Fraunié2020) and for supporting species’ upward migration in mountains due to increasing warming or the new habitats that have been opened up by glacier retreat (Milner et al. Reference Milner, Robertson, Monaghan, Veal and Flory2008, Seimon et al. Reference Seimon, Seimon, Yager, Reider, Delgado and Sowell2017). Regarding river connectivity, future studies on the protection of free-flowing rivers by PAs across the Andean–Amazon region could provide relevant information about fluvial connectivity, conservation of river ecological function and ecosystem services provision (Opperman et al. Reference Opperman, Shahbol, Maynard, Grill, Higgins, Tracey and Thieme2021). Furthermore, we evidenced how glacier coverages have been protected by PAs as they represent important water sources in the region. Nevertheless, polar and alpine sites that include ice sheets, glaciers and perennial snowfields have been categorized as a new biome by the IUCN due to their high concentrations of microbial life (Keith et al. Reference Keith, Ferrer-Paris, Nicholson and Kingsford2020). Investigations of the biodiversity inhabiting glaciers are needed in order to better understand their implications for stream and lake functioning in the tropical Andes, as has been reported for other regions (Fellman et al. Reference Fellman, Hood, Raymond, Hudson, Bozeman and Arimitsu2015).
On the other hand, studies of PAs as constant providers of ecosystem services were limited to specific cases of fisheries and organic matter decomposition. Studies on the role of organic matter decomposition by aquatic invertebrates for maintaining water quality inside and outside PAs are required. Furthermore, we did not find any studies about the economic implications of water supply by PAs. Specifically, we did not find studies that have assessed the economic value of PAs in protecting significant water sources such as glaciers, which are of great importance for the millions of people inhabiting the region (Soruco et al. Reference Soruco, Vincent, Rabatel, Francou, Thibert, Sicart and Condom2015, Kinouchi et al. Reference Kinouchi, Nakajima, Mendoza, Fuchs and Asaoka2019). Thus, future studies focusing on these issues will provide relevant information for freshwater conservation in the tropical Andes.
Supplementary material
To view supplementary material for this article, please visit https://doi.org/10.1017/S0376892921000382.
Acknowledgements
We thank the anonymous reviewer and editor for their helpful suggestions and corrections regarding a previous version of the manuscript. We thank Gabrielle Vance from the Mountain Research Initiative (MRI) for the English revision.
Financial support
Writing this review article was an activity of the Mentoring and Training Program in IPCC Processes for Early Career Mountain Researchers supported by the Swiss Agency for Development and Cooperation (SDC) within the programme of SDC contributions to the 6th Assessment Cycle of the IPCC, and organized by MRI, University of Zurich, Helvetas and ICIMOD. E. Quenta-Herrera conceptualized the review article and was selected to participate in the programme.
Conflict of interest
None.
Ethical standards
None.