Introduction
Conserving landscape characteristics, especially those that preserve habitat cohesiveness, is fundamental to ensuring the maintenance of ecological processes and biodiversity. Considering that habitat loss and fragmentation are universally recognized as the main direct threats to biodiversity, improving connectivity and habitat amount or quality stand out as the leading strategies in biodiversity conservation, although not without controversy as to which should be favoured (Wiens Reference Wiens2006, Hodgson et al. Reference Hodgson, Thomas, Wintle and Moilanen2009, Reference Hodgson, Moilanen, Wintle and Thomas2011, Doerr et al. Reference Doerr, Barrett and Doerr2011). This debate is particularly relevant for decision-makers, who need to balance these trade-offs in light of the widespread scarcity of funding resources (Wiens Reference Wiens2006, Bottrill et al. Reference Bottrill, Joseph, Carwardine, Bode, Cook, Game and Grantham2009).
Some habitat types are naturally separated within the landscape. Mountaintop rock outcrops represent a good case of this condition, often occurring as a string of distinct patches. Likely restrictions on gene flow and reduced connectivity between individuals, predominantly cross-species, suggest that outcrop plant communities may be comparable to vegetation on oceanic islands (Martinelli Reference Martinelli2007). Although rock outcrops are of very diverse geological origin, they share key characteristics, most of them related to the severity of the environment, and they are typically included among the old, climatically buffered, infertile landscapes (OCBILs; sensu Hopper Reference Hopper2009). Their plant communities are edaphically controlled (Strahler Reference Strahler1978, Kruckeberg Reference Kruckeberg2002) and are usually characterized by high species richness and habitat specialists (Gibson et al. Reference Gibson, Yates and Dillon2010, Reference Gibson, Meissner, Markey and Thompson2012, Messias et al. Reference Messias, Leite, Meira-Neto and Kozovits2012, Poot Reference Poot, Hopper and Van Diggelen2012). Plants are typically rupicolous, providing sparse and low vegetation cover and exposed to full sun, wind and rain (Jacobi et al. Reference Jacobi, Carmo, Vincent and Stehmann2007, Gibson et al. Reference Gibson, Yates and Dillon2010). It is within this setup that the Quadrilátero Ferrífero (Iron Quadrangle (IQ)) region in southeast Brazil calls for the application of connectivity analyses in order to conserve threatened montane habitats and their biodiversity. Its rock outcrops, particularly ironstone, harbour endemic-rich plant communities and contribute substantially to the biodiversity of the biomes they are embedded within – Atlantic Rainforest and Cerrado (Viana & Lombardi Reference Viana and Lombardi2007, Jacobi & Carmo Reference Jacobi and Carmo2008, Carmo & Jacobi Reference Carmo and Jacobi2013).
The IQ is contained in one of the UNESCO Biosphere Reserves (UNESCO 2016), and one of its main characteristics is the ubiquity on the mountaintops of cangas, ferruginous duricrusts rich in iron oxides and other metallic minerals, capping iron ore deposits. In this region, some canga outcrops belong to formations 50 Ma old, representing one of the oldest landscapes in Brazil (Monteiro et al. Reference Monteiro, Vasconcelos, Farley, Spier and Mello2014, Salgado & Carmo Reference Salgado and Carmo2015). The IQ is also one of the most important mineral provinces in the world, particularly of iron ore (Spier et al. Reference Spier, Barros and Rosière2003), and is currently under intense habitat-specific destruction by mining activities (Sonter et al. Reference Sonter, Moran, Barrett and Soares-Filho2014b). Until 1950, Brazil barely produced 1.5 million tons of iron ore annually (Machado & Figueroa Reference Machado and Figueirôa2001). At that time, the anthropic interventions in the mountains to extract iron ore were not significant, although they had been going on since the nineteenth century (Dorr II Reference Dorr1969). In 2014, however, the country produced around 400 million tons of iron ore, 51.6% of which being extracted from the IQ mountain ranges, and it was ranked second in world production (IBRAM 2015).
Landscape change simulations for 2010–2030 have related steel demand to far-reaching impacts on native vegetation, particularly in habitats removed by open pit mining (Sonter et al. Reference Sonter, Barrett, Soares-Filho and Moran2014a, Reference Sonter, Moran, Barrett and Soares-Filho2014b). The distribution of at least 116 plant species is restricted to the IQ and about a quarter of these habitat specialists occur exclusively in canga, the rock type that is most targeted by mining activities (Jacobi et al. Reference Jacobi, Carmo and Campos2011, Carmo & Jacobi Reference Carmo and Jacobi2012). Therefore, canga habitats may be characterized as areas with high vulnerability, high conservation value and biological irreplaceability, specifically in terms of plant species endemism (sensu Brooks et al. Reference Brooks, Mittermeier, Fonseca, Gerlach, Hoffmann and Lamoreux2006).
Connectivity analysis of both past and current configurations of patches, in this case canga outcrops, may contribute to better defining priority locations and conservation actions (Tischendorf & Fahrig Reference Tischendorf and Fahrig2000, Calabrese & Fagan Reference Calabrese and Fagan2004). Key to the application of connectivity analysis to conservation problems is the recognition that, besides connecting with other patches, an individual patch has itself internal connectivity (Pascual-Hortal & Saura Reference Pascual-Hortal and Saura2006, Rayfield et al. Reference Rayfield, Fortin and Fall2011). According to this notion, even an isolated patch has some connectivity value depending on the amount of resource (usually area). The overall amount of available (i.e., reachable) habitat for a particular species in a landscape is thus composed of ‘intrapatch connectivity’ (the area within an individual patch) and ‘interpatch connectivity’ (the links to other patches). Recent developments of the indices associated with the habitat availability and functional connectivity concepts (Saura & Rubio Reference Saura and Rubio2010) have allowed quantification and differentiation among these two types of connectivity. With this in mind, and with the ever-growing destruction of canga habitats, our aim was to evaluate the current habitat availability for a focal group of 32 plants endemic to ironstone outcrops. For this, we compared the change in habitat network properties of the historical and current canga patches in the IQ, focusing on functional connectivity in the ideal scenario where a certain species is present in all patches.
Methods
Study Area
The IQ lies in the transition of the two Brazilian biodiversity hotspots, Atlantic Rainforest and Cerrado (Myers et al. Reference Myers, Mittermeier, Mittermeier, Fonseca and Kent2000, Mittermeier Reference Mittermeier, Gil, Hoffman, Pilgrim, Brooks and Mittermeier2005, Joppa Reference Joppa, Roberts, Myers and Pimm2011). It is perhaps the location in eastern Brazil (Fig. 1) whose geology has been best studied, because of its peculiarly large amount of rock types and of historical economic interests represented by the mining industry (Dorr II Reference Dorr1969, Machado et al. Reference Machado, Noce, Ladeira, Belo de Oliveira and Carneiro1992). In contrast, much less was known of its biology until recently. Of the plant communities growing on rock outcrops, those on canga patches are the most affected by mining activities since they cover the reserves of iron ore. The canga duricrust is very heterogeneous and may harbour caves, swamps, cracks and escarpments. This bedrock contains high concentrations of metallic minerals, especially iron and manganese, is covered by very thin or no soil and water quickly runs off the surface. The most characteristic plant community over canga is composed of scattered shrubby or herbaceous species that grow by directly creeping along the surface or in association with crevices and gaps (Carmo & Jacobi Reference Carmo and Jacobi2016).
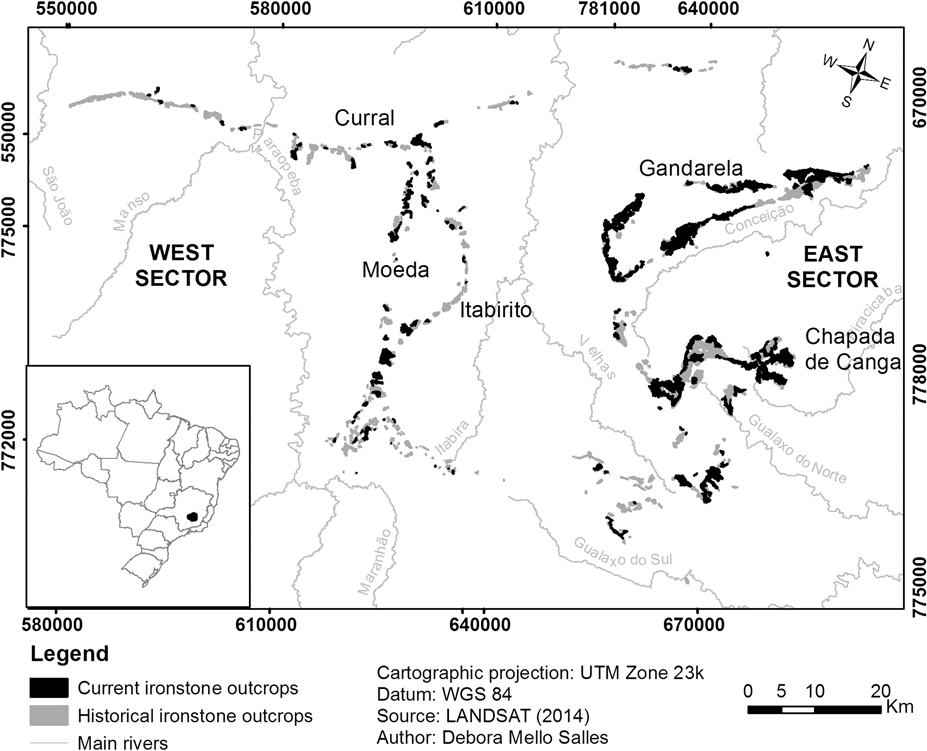
Fig. 1 Location of the Iron Quadrangle in southeast Brazil, with details of historical (grey) and current (black) ironstone outcrops.
The IQ cangas are found on the mountaintops (c. 900–1800 m) of the main regional ranges, which may be divided into east and west sectors, separated by the Velhas River valley. The main topographic features are the Serra do Gandarela and Chapada de Canga formation in the east sector and Serras da Moeda and Itabirito in the west sector. To the north, canga patches are found along the Serra do Curral, which extends west to east.
Data Sources
Polygons in vector format of the historical (1960s) IQ canga patches were obtained from Lobato et al. (Reference Lobato, Baltazar, Reis, Achtschin, Baars and Timbó2005), who georeferenced the cangas catalogued by the Brazilian National Department of Mineral Production (DNPM) and the United States Geological Survey (USGS) between 1946 and 1964 (Dorr II Reference Dorr1969) at a scale of 1:25 000. Current patches were mapped based on Landsat satellite images in August 2014 using Spring and ArcGIS software at a scale of 1:50 000. These new polygons excluded all canga areas that were no longer functional habitats, ranging from those subjected to small physical interventions, such as dirt roads, to predominantly large volumetric alterations resulting from opencast mining.
To evaluate functional connectivity, we adopted a maximum edge-to-edge dispersal distance of 500 m. This distance represents a very optimistic scenario based on pollination and seed dispersal syndromes of the 32 taxa (16 families; Supplementary Table S1, available online), known to be endemic to the IQ cangas (Jacobi & Carmo Reference Jacobi and Carmo2011, Carmo & Jacobi Reference Carmo and Jacobi2012). Because their records are distributed in an area smaller than 10 000 km2, all of these species may be classified as rare based on the criteria of Giulietti et al. (Reference Giulietti, Rapini, Andrade, Queiroz and Silva2009). Ten of these plants are already cited in the catalogue of Rare Plants of Brazil (Giulietti et al. Reference Giulietti, Rapini, Andrade, Queiroz and Silva2009). Their restricted distribution, associated with the magnitude of habitat loss and fragmentation, places them in the Critically Endangered category of the International Union for Conservation of Nature (IUCN) Red List of Threatened Species (IUCN 2012). Patches that were separated by 50 m or less were merged, under the assumption that narrow strips like roads would not be relevant deterrents for seed or pollen vectors.
Analyses
Among the connectivity metrics currently available, the graph-based metrics (Dale & Fortin Reference Dale and Fortin2010) developed by Pascual-Hortal and Saura (Reference Pascual-Hortal and Saura2006), within the conceptual framework of habitat availability or reachability, have the necessary attributes to evaluate the importance of individual patches as connectivity providers. We adopted the Integral Index of Connectivity (IIC), which is considered robust when compared to other indices based on graphs (Pascual-Hortal & Saura Reference Pascual-Hortal and Saura2006, Saura & Pascual-Hortal Reference Saura and Pascual-Hortal2007). The IIC relies on a binary connection model to determine whether two patches are connected, within an established maximum dispersal distance. The landscape was also set as binary (canga patches and matrix) because the focal species only develop over ironstone outcrops. After obtaining the edge-to-edge Euclidean distance for all pairs of patches, the importance of each individual patch was calculated as dIIC; that is, the relative decrease of the IIC value if that patch is removed from the landscape (see Pascual-Hortal & Saura Reference Pascual-Hortal and Saura2006 for details). Saura and Rubio (Reference Saura and Rubio2010) partitioned the dIIC into three fractions, dIICintra, dIICflux and dIICconnector, each of which calculates the contribution of a certain patch to complementary connectivity attributes. Thus, the ‘intra’ fraction relates to the amount of some patch attribute (area in our case) and ‘flux’ refers to how well connected the patch is, while the ‘connector’ fraction highlights the patch role as a stepping stone (Saura & Rubio Reference Saura and Rubio2010).
All index calculations were performed using version 2.6 of the Conefor Sensinode software (Saura & Torné Reference Saura and Torné2009, see http://www.conefor.org).
Results
Canga patches (canga duricrusts) already appear largely disconnected in their original configuration (Dorr II Reference Dorr1969) and are even more so today (Fig. 1).
Overall, the connectivity of cangas has been more impacted in the west sector. Formerly in the IQ there were 334 patches occupying 18 654 ha, and currently only 171 patches are found corresponding to 9608 ha, a decrease of approximately 50% in both the number and area of the outcrops (Table 1). Based on the 500-m maximum dispersal distance, the historical canga patches were assembled into 107 groups of connected patches, of which 47% consisted of a single patch (Fig. 2) and the total number of links was 319. The current configuration consists of only 81 groups and 127 links, and the proportion of single-patch groups increased to c. 62%. In the past, the east sector had a greater connectivity, encompassing almost 72% (13 395 ha) of the total area of the ironstone outcrops, and 109 patches (30%), 31 groups (25%) and 97 links (31%) (Table 1). Today, the east sector still houses almost 81% (7788 ha) of the total area of ironstone outcrops, 82 patches (48%), 28 groups (35%) and 80 links (62%).
Table 1 Connectivity attributes comparison between the Iron Quadrangle canga patch configuration and its east sector for the historical and current patches, adopting a maximum dispersal distance of 500 m

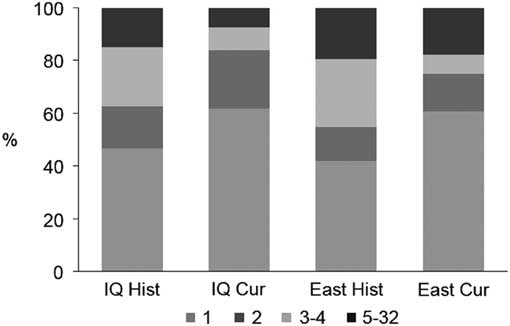
Fig. 2 Proportion of groups of patches in each configuration according to their size (i.e., number of connected patches). Cur = current cangas; East = east sector; Hist = original cangas; IQ = Iron Quadrangle.
Presently, all of the 20 highest-ranking patches based on their importance value of functional connectivity (dIIC) (Table 2) are located in the east sector. Except for four patches (including the first three), where the attribute that contributes most to the importance values is the amount of available area (i.e., dIICintra), in all of the other patches, the ‘flux’ fraction (dIICflux) is more relevant. Patches that serve mostly as relevant stepping stones (dIICconnector) are scarce. In general, in both the historical and current habitat patch configurations, the contribution of any single patch as a connector was close to zero. This low contribution of stepping-stone patches is a result of the large proportion of groups that are composed of only one or two patches, especially in the current configuration (Fig. 2).
Table 2 Importance value (dIIC) of the 20 highest-ranking canga patches in the Iron Quadrangle, all located in the east sector (see Fig. 2 for details on location). dIIC = dIICintra+dIICflux+dIICconnector

The five most important patches based on their values as habitat providers are located in Serra do Gandarela, Chapada de Canga, Serras do Tamanduá and Batatal, all in the east sector (Fig. 3). The relevance of the first three relies heavily on their large area, as they connect with very few patches and do not serve as connectors between others. The fourth and fifth top patches, on the other hand, are both large and well connected to other relevant patches, although they do not act as stepping stones.
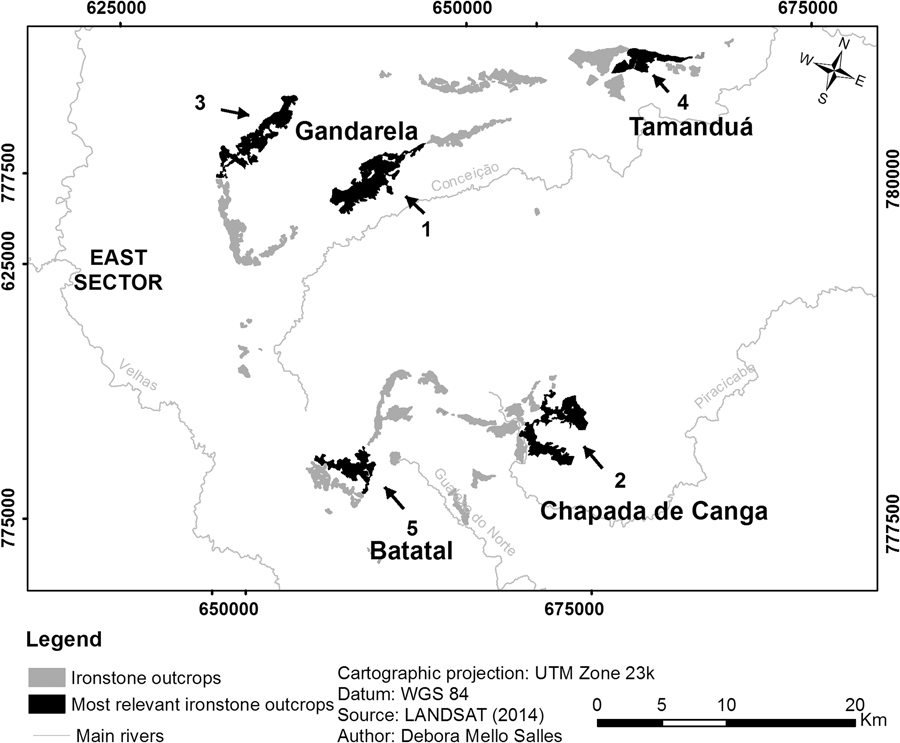
Fig. 3 Location in the east sector of the five most relevant current canga patches of the Iron Quadrangle, based on their importance value (dIIC) related to habitat availability for endemic plants.
Discussion
The assembly of ironstone outcrops in the IQ has suffered large transformations since its first systematic assessment (Dorr II Reference Dorr1969). The overall canga habitat loss until 2014 was 50%. This amount of habitat loss per se is enough to cause negative alterations in the canga plant communities. However, depending on the spatial configuration and topography, the same amount of area loss could lead to different types of connectivity reduction. In this respect, the IIC and related metrics not only allow the value of a given patch as a connectivity provider to be determined, but also indicate in what way it has value: either providing area or enhancing connectivity.
The connectivity analyses attested that canga habitats in the IQ are naturally disconnected in terms of their plant communities, and thus they are most likely already an evolutionary manifestation of the consequences of isolation, at least regarding groups of patches belonging to distant topographic features, such as Serra da Moeda and Chapada de Canga. There are few studies on the genetic impact of habitat loss in ironstone ranges. Butcher et al. (Reference Butcher, McNee and Krauss2009) centred on a micro-endemic, perennial, insect-pollinated Australian shrub of low genetic diversity and fine-scale spatial structure consistent with historically low gene flow. Thus, while it could be argued that the canga communities are adapted to these landscape conditions, today these outcrops are disappearing at an unprecedentedly high pace (Jacobi et al. Reference Jacobi, Carmo and Campos2011, Lavor et al. Reference Lavor, Van Den Berg, Jacobi, Carmo and Versieux2014). It is possible that there is still a large extinction debt for some species, chiefly those with large populations, while for other species with small populations the debt may have already been paid (Tilman Reference Tilman, May, Lehman and Nowak1994, Banks Reference Banks1997, Malanson Reference Malanson2008, Piqueray et al. Reference Piqueray, Bisteau, Cristofoli, Palm, Poschlod and Mahy2011). For example, in calcareous grasslands, the response to habitat loss and fragmentation can be relatively slow in some species (Helm et al. Reference Helm, Hanski and Pärtel2006) and relatively fast in others (Saar et al. Reference Saar, Takkis, Pärtel and Helm2012). In addition, variations in either resource quantity or distance among patches could negatively influence pollen or seed disperser behaviour, and this could in turn lead to high inbreeding, or at least to changes in population genetic structure (Frankham Reference Frankham, Ballou and Briscoe2004, Franceschinelli et al. Reference Franceschinelli, Jacobi, Drummond and Resende2006, Hughes Reference Hughes, Möller, Edwards, Bellstedt and Villiers2007).
Besides putting into evidence the canga habitat loss, our analyses served to quantify the degree to which canga habitats rely on conservation of large patches to maintain populations of plant species that are endemic to these habitats. Prioritizing the conservation of area is recommended in situations such as the one reported here, where it is clear that while small patches can contribute to enhancing connectivity, the risks of relying solely on this strategy are high (Wiens Reference Wiens2006, Rubio & Saura Reference Rubio and Saura2012, Rubio et al. Reference Rubio, Bodin, Brotons and Saura2015). In addition, since the ecological benefits of large patches go beyond these patches’ role as connectivity providers, some researchers strongly advocate that preserving the integrity of large areas should be put above all other strategies whenever feasible (e.g., Frankham 2004, Wiens Reference Wiens2006, Hodgson et al. Reference Hodgson, Moilanen, Wintle and Thomas2011).
In the IQ setting, two factors make connectivity by stepping stones (the ‘connector’ fraction sensu Saura & Rubio Reference Saura and Rubio2010) quite challenging and without good alternatives: the large percentage of groups formed by only one or two patches and the prevalent string-like array that results from the display along ridges as well as restrictive dispersal distances. This enhances the relevance of large individual patches as connectivity providers (the ‘intra’ fraction). Some patches could, nevertheless, still be considered meaningful for landscape connectivity, and efforts should be made for the conservation of these and their immediate environment, preferably as officially protected areas. The most promising groups of patches are those in the Gandarela and Chapada de Canga mountain ranges, particularly for the 22 taxa that occur in the east sector (Supplementary Table S1). All of them have well-connected, large patches and some stepping-stone patches. Unfortunately, the Gandarela National Park (Brasil 2014), which was recently created after intense public demand, had most of these areas excluded from its limits to comply with the interests of mining companies.
Despite the variation within the matrix vegetation types and intensity of change in land use (Sonter et al. Reference Sonter, Barrett, Soares-Filho and Moran2014a), we have pictured the distribution of canga patches as a simple binary matrix–habitat system, assuming edge effects are minor and where their position on mountaintops does not obstruct wind and volant dispersers. This simplification is justified because canga patches are well defined within the landscape and the focal plants for conservation purposes are habitat specialists (Carmo & Jacobi Reference Carmo and Jacobi2012). However, the ironstone plant community harbours many other plants that do not have such substrate restrictions. These ‘generalists’, which include invasive African grasses, are favoured by the stepping stones provided by other outcrop types (particularly quartzite) and even savannic surroundings. In addition, after mining, the substitution of canga habitat with less compact substrates (e.g., rubble) tends to benefit these and other generalists. This could lead to their dominance and directly or indirectly to local extinction of members of the focal group. Furthermore, there are indirect negative impacts associated with mining, such as those from refuse disposal in dumps or dams, which further compromise functional connectivity by degrading the environment and are frequent sources of exotic species used in rehabilitation that may become invaders (Durigan et al. Reference Durigan, Engel, Torezan, Melo, Marques, Martins and Scarano2010).
On-site restorations of ironstones are virtually impossible, given the substantial changes in topography and geological characteristics that result from opencast mining (Gibson et al. Reference Gibson, Coates, van Leeuwen and Yates2015, Jacobi et al. Reference Jacobi, Carmo and Campos2015). Compensating loss by creating patches, a strategy that in itself is only suitable for species with high dispersal abilities and that are not sensitive to patch turnover (Johst et al. Reference Johst, Dreschsler, Van Teefelen, Hartig, Vos and Wissel2011), is currently unrealistic, as it would imply relocating large slabs of substrate. Even so, mining companies are investing efforts into rehabilitating the surrounding areas or areas of lesser mining interest by spreading the previously removed topsoil layer and its seed bank. This method is highly biased towards species that are not habitat specific because the physical characteristics of the duricrust are not preserved. Furthermore, aside from the high beta-diversity among canga outcrops, there is also little floristic similarity between canga patches and other outcrop types, even when they are geographically close or adjacent (Messias et al. Reference Messias, Leite, Meira-Neto and Kozovits2012, Carmo & Jacobi Reference Carmo and Jacobi2013, Carmo & Jacobi Reference Carmo and Jacobi2016).
According to our analysis, the best alternative for the conservation of the plant species endemic to cangas is to preserve continuous large patches. Since these are usually economically targeted habitats, this is a solution that will demand considerable negotiation among parties.
Supplementary Material
For supplementary material accompanying this paper, visit www.cambridge.org/core/journals/environmental-conservation
Acknowledgements
We thank Márcia Felícia S Santos for her help with map-making. The insightful comments of Ricardo Ribeiro de Castro Solar, Fernando Augusto de Oliveira e Silveira, Fernando Figueiredo Goulart and two anonymous reviewers greatly improved previous versions of the manuscript.
Financial Support
This research was financed by CNPq and FAPEMIG. CMJ received a productivity fellowship (PQ ID) and a CNPq postdoctoral fellowship while on sabbatical at the University of Toronto. DMS and FFC received a FAPEMIG and a CNPq doctoral scholarship, respectively.
Conflict of Interest
None.
Ethical Standards
None