Introduction
A mass-casualty incident (MCI) is defined as an overwhelming event which generates a large number of casualties that cannot be managed by the locally available resources. Reference Lomaglio, Ansaloni, Catena, Sartelli, Coccolini, Kluger, Coccolini, Catena and Ansaloni1 The definition of the MCI therefore depends on the available resources as well as the event itself. Many hospitals and emergency medical services (EMS) have set plans for managing MCIs. Unfortunately, despite its known vulnerability in such incidents, planning for the pediatric population is often omitted. Reference Dziuban, Peacock and Frogel2
A patient should be triaged multiple times during transit through the pre-hospital and hospital systems. On scene, primary triage is done for rapid assessment, reassessment, treatment, and transport decisions. If evacuation times are prolonged or after primary assessment in the prehospital setting, secondary triage, a more detailed assessment, is done in-hospital for resource allocation and disposition decisions (operation room, intensive care unit, etc.). 3 A patient’s triage category may change during their treatment journey. This may be related to evolution of disease/ injury or to evolution of the context (e.g., resource availability, ongoing MCI, etc.).
Although some triage systems stress keeping triage for both adults and pediatrics similar, to avoid confusion during infrequent and high-stake events, both primary and secondary triage systems in MCIs should differentiate between adult and pediatric patients because of different anatomy and physiology. As well, the different patterns of injury and causes of death in children and youth suggest the necessity of a pediatric-specific triage system. Reference Gilchrist and Simpson4 In fact, even when using the various adapted pediatric triage systems, healthcare providers tend to over-triage (i.e., overestimate the extent of the injury or exposure) in the pediatric patient. Reference Koziel, Meckler and Brown5 This may be related to emotional stress when dealing with pediatric patients in an MCI and to the unfamiliarity with assessing children. Reference Koziel, Meckler and Brown5 The lack of familiarity with pediatric injuries may lead to greater than 20% overtriage and unnecessary transfers which can have an important impact on resource allocation and utilization. Reference Goldstein, Van Arendonk and Aboagye6,Reference Nordin, Kenney, Thakkar and Diefenbach7
The recent increase in human-made MCIs involving children, particularly those involving firearms during the coronavirus disease 2019 (COVID-19) pandemic, in addition to the inevitability of pediatric casualties in any disaster, have raised concerns regarding the proper triage and management of children. Reference Cohen, Donnelly and Patel8,Reference Cicero and Baum9 Simulations that include children are increasingly being conducted around the world to help evaluate different triage systems. Reference Cicero, Auerbach, Zigmont, Riera, Ching and Baum10 However, there is still no consensus on the most accurate triage system for pediatric patients in MCIs. In fact, there is little consensus on the very definition of ‘accuracy’ of a triage algorithm, further complicating comparative evaluation.
Lerner et al. attempted to create a gold standard for both adult and pediatric triage systems by consensus in 2015. Reference Lerner, McKee and Cady11 However, the algorithm’s complexity and its use in-hospital delayed lab work, interventions, and disposition (e.g., use of vasopressors in the first 2 hours, discharge from the emergency department (ED) without work up, etc.) preclude it from being used in the field or on presentation to the ED. It may be more useful for comparison of different triage algorithms but cannot be used as an MCI triage system.
Objective
A scoping review was conducted to identify the most accurate triage system for pediatric patients in MCIs. Considering the heterogenicity of the studies, the vague nature of the subject and the large number of algorithms used, a scoping instead of a systematic review was chosen. The objective was not to create a new algorithm but to find the most accurate pediatric MCI algorithm.
Methods
The following databases were searched for relevant studies on February 3, 2021: MEDLINE (via Ovid, 1946 to February 3, 2021; via PubMed, January 22, 2021 to February 5, 2021); Embase (via Ovid 1974 to February 3, 2021); CINAHL (via EBSCOhost); The Cochrane CENTRAL Register of Controlled Trials (via Wiley, from Inception to Issue 2 of February 12, 2021); Scopus (via Elsevier); Global Health (via Ovid, 1973 to 2021 Week 4); Global Health Archive (via Ovid, Global Health Archive 1910 to 1972); and Global Index Medicus (via WHO). No language or publication year limits were applied.
In addition, clinical trials registries (ClinicalTrials.gov, International Clinical Trials Registry Platform, UK Clinical Trials Gateway) and grey literature sources (ProQuest Dissertations and Theses, ECRI Guidelines Trust, CMA Infobase, National Institute for Health, and Care Excellence, Canadian HTA Repository, Google Scholar, and Open Grey) were searched (February 19, 2021 for all save ICTRP and Google Scholar, searched February 24, 2021).
The search strategies were designed by an experienced librarian (AB) using text words and relevant indexing to identify articles on pediatric MCI triage systems. A second librarian peer-reviewed the initial Medline strategy, and a third librarian peer-reviewed the strategy adaptations to other databases. The final Medline strategy can be found in Appendix 1.
Further studies were identified in Web of Science and Scopus (March 24, 2021) by carrying out citation searches for the reference lists of included studies.
For all database records, deduplication was performed by the librarian with the bibliographic management software EndNote. 12 Deduplication settings were modified for each of the 12 rounds of iterative duplicate identification, followed by a manual verification for duplicates.
Screening was performed with the online software Rayyan QCRI. Reference Mourad Ouzzani, Fedorowicz and Elmagarmid13 The studies were then divided into 3 categories: accuracy of a single system, comparison of 2 or more primary triage systems, and comparison of secondary triage systems.
Inclusion criteria
Articles on pediatric MCI triage systems were screened by study type and study measures. Randomized Controlled Trials (RCTs), controlled studies, reviews, prospective observational studies, and retrospective chart review studies were included. Case reports and series, historical descriptive studies were excluded.
Studies evaluating the sensitivity, specificity, or accuracy (as per the author’s definition in each study) of different MCI triage systems were included. Studies comparing 2 or more MCI triage systems (primary or secondary) were also included.
Studies on exclusively adult data were excluded; however, from articles including both adult and pediatric data, the data concerning pediatric population was included.
Results
The searches identified 996 reports after deduplication. 972 were excluded after scanning the titles and abstracts. Upon full manuscript review of the remaining 24 articles, 6 were excluded. As a result, 18 articles were included in the study (Figure 1). These articles included 6 prospective observational studies, 5 retrospective studies, 4 conference abstracts, and 2 literature reviews, as well as 1 survey (Tables 1, 2 and 3). The articles were arranged into the 3 predefined categories:
1) Accuracy of a single pediatric triage system
2) Comparison of 2 or more primary triage systems: Simple Triage And Rapid Treatment (START) and its pediatric version JumpSTART (JS), CareFlight (CF), Pediatric Triage Tape (PTT), Sort, Assess, Lifesaving interventions, Treatment/ Transport (SALT), Sacco Triage Method (STM), Sieve Triage, Smart tape (Smart), New York Fire Department (NYFD), Glasgow Coma Scale (GCS) and No Algorithm (NA)
3) Comparison of secondary pediatric triage systems: Triage – Revised Trauma Score (T-RTS); Physiological and Anatomical Triage (PAT) and Pediatric Physiological and Anatomical Triage Score (PPATS)

Figure 1. Modified PRISMA Flow diagram.
Table 1. Accuracy of a single system

Table 2. Comparison of 2 or more primary triage systems
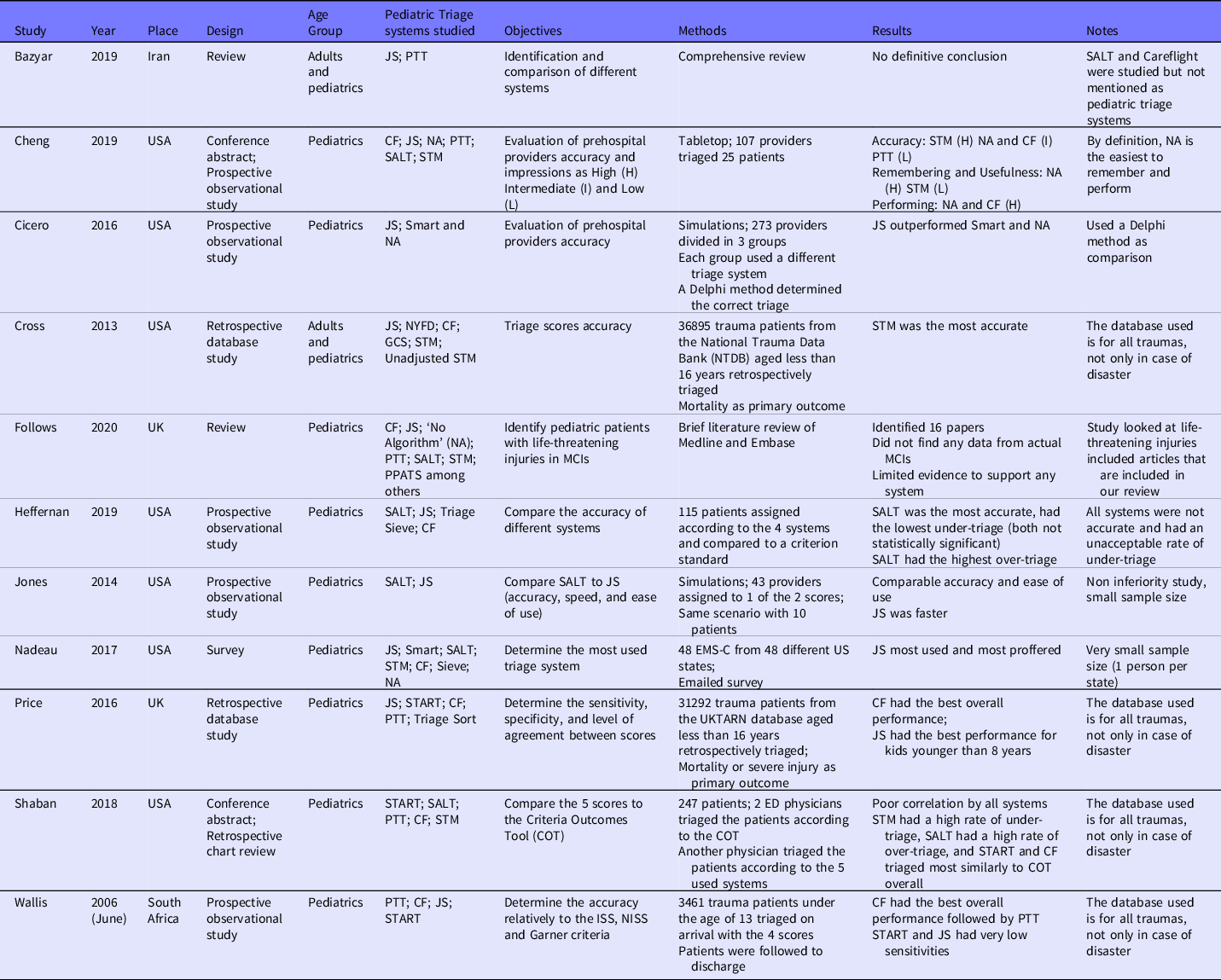
Table 3. Comparison of secondary triage systems

Accuracy of a single pediatric triage system
While the principal objective of this review was to identify the most accurate triage system for pediatric patients in MCIs, this review clearly demonstrates a lack of agreement in the literature on the most effective way of comparing triage algorithms. The difficulty in comparative evaluation stems from a lack of agreement on the definition of accuracy of a triage algorithm. Different articles reported different ways of looking at accuracy. 4 articles studied a single algorithm in different ways (Table 1). DeGeorge et al. found that providers who used JS had a low level of agreement concerning the triage category while McGlynn et al. tested the inter-rater reliability (IRR) of SALT and concluded that when applied to a large number of patients, SALT has a poor IRR with tendency to under-triage (increased proportion of lower acuity patients with increasing number of cases). Reference DeGeorge, Glick, Ackerman and Wu14,Reference McGlynn, Claudius and Kaji15 PTT was also studied by Wallis et al. and although it had high specificity when compared to the Injury Severity Score (ISS), it had very low sensitivity which means that PTT could miss critically ill patients. Reference Wallis and Carley16 Chayen et al. attempted to create an algorithm by combining primary (PTT) and secondary (T-RTS) scores to be applied in the hospital settings; however, this algorithm was not externally validated nor compared to other triage algorithms. Reference Chayen, O’Leary, Major, Dawson and Gosby17 It should be noted that 50% of the studies included are at least 10 years old, which reflects the need for more recent and updated studies especially with the increased MCIs in the pediatric population.
Comparison of different algorithms
A total of 11 studies compared pediatric MCI triage algorithms using different strategies (Table 2). Only 2 reviews tried to identify the most used algorithms but were unable to identify the best algorithm available and were not added to the total number of studies used for comparison. Reference Bazyar, Farrokhi and Khankeh18,Reference Follows and Vassallo19 JS was the most studied algorithm as it was mentioned in 8 out of 9 studies. The other well studied algorithms were CF (7 studies) and SALT (5 studies); STM and PTT were included in 4 studies while other algorithms were mentioned in less than 3 studies. Due to the heterogenicity of the studies, the most accurate algorithm was not identified. However, CF was found to have a good overall performance in multiple comparative studies, Reference Cheng, Staats and D’Arcy20–Reference Wallis and Carley23 while JS was the most used. Reference Nadeau and Cicero24 SALT was consistently found to have a high over-triage rate when compared to predefined scores meaning that it has high sensitivity but low specificity to high acuity cases and this means that although SALT is less likely to miss more critical patients, using it will probably lead to more resource utilization. Reference Shaban, Donofrio, Kaji and Cicero22,Reference Heffernan, Lerner and McKee25 Studies analyzing PTT and STM had very controversial and conflicting results: PTT was found to have a low accuracy in 1 study and high performance rate in another study; Reference Cheng, Staats and D’Arcy20,Reference Wallis and Carley23 STM on the other hand was found to be highly accurate in 2 studies and to have a high rate of under-triage in another study. Reference Cheng, Staats and D’Arcy20,Reference Shaban, Donofrio, Kaji and Cicero22,Reference Cross and Cicero26 These different and conflicting results highlight once again the issue of defining ‘accuracy.’
Comparison of secondary pediatric triage systems
A secondary triage system is defined as a second, more detailed, triage algorithm for patients who were already triaged with a primary triage algorithm. This helps identify patients in need of ICU admission or emergent intervention (Table 3). Reference Vassallo and Smith27 A triage algorithm, PPATS, was developed in 2018 specifically for pediatric patients and was found to better predict mortality than the previous T-RTS score. Reference Toida, Muguruma and Abe28 These results were externally validated using 2 retrospective database chart reviews that found the same results. Reference Muguruma, Toida, Furugori, Abe and Takeuchi29,Reference Lin, Lai and Hu30
Discussion
Almost 25% of the world population is less than 15 years of age and unfortunately, by 2016, shootings were the leading cause of death in the pediatric population in the United States surpassing motor vehicle collisions. 31–Reference Goldstick, Cunningham and Carter33 More often, this means that 25% of patients in MCIs may be pediatric patients. Multiple systems are used around the world to help prioritize care and efficiently allocate resources in such a way as to improve population outcomes overall. Although some systems are used more commonly than others, all MCI triage tools have the following principle in common: assignment of resources based on the initial patient assessment and consideration of available resources. 34
‘Children are not little adults.’ This well-known and used expression timelessly encapsulates the significant differences in anatomy, physiology, and clinical characteristics between children and adults. There exist numerous important variances in head and neck, thoracoabdominal and musculoskeletal injury patterns, as well as a child’s physiologic response to injury. Reference Figaji35 There is also a wide spectrum for categorizing pediatric trauma, based upon upper age limits (e.g., 12-21yr/ preadolescent), weight (e.g., ≥ 50kg) and/ or presence of secondary sex characteristics. Reference Hardin, Hackell, Committee On and Ambulatory36 Thus, while current algorithms are structured similarly regardless of age, these variances should be taken into consideration.
Another important and controversial point is the definition of accuracy and finding the ‘best’ triage system. Some authors like Shaban et al. and Wallis et al. compared different algorithms to a predefined score like Criteria Outcomes Tool (COT) for the former, and the ISS, NISS, and Garner criteria for the latter, with the triage algorithm that is most comparable to the predefined score being the most accurate. Reference Shaban, Donofrio, Kaji and Cicero22,Reference Wallis and Carley23 Other studies looked at the providers’ perspective and ease of use either in a tabletop or using a Delphi method during a simulation. Reference Cheng, Staats and D’Arcy20,Reference Cicero, Overly and Brown37 Also, Cross et al. retrospectively looked at trauma patients that were hospitalized and compared the outcome (mortality) while applying different triage algorithms upon first presentation to identify the best algorithm with the lowest mortality. Reference Cross and Cicero26
JS is the most commonly used system in the USA, Reference Nadeau and Cicero24 and this can be partially due to its derivation from an adult system, START, that is the most widely used system in the USA. Reference Bazyar, Farrokhi and Khankeh38 JS was designed for use in children 8 years of age and younger, but more recently, generally applied in all pre-adolescent patients. It was found to have the best outcomes in terms of mortality in this age group compared to other algorithms. However, JS can have low sensitivity when compared to the New Injury Severity Score (NISS) and the Injury Severity Score (ISS). These are 2 scores used to classify the severity of injury in individualized patients, which can lead to unacceptable rates of under-triage as missing patients with high NISS or ISS, means missing patients with higher risk of morbidity, and/or mortality. Reference Price, Brace-McDonnell, Stallard, Bleetman, Maconochie and Perkins21,Reference Wallis and Carley23 While over-triage means that resources will be overutilized, under-triage is by definition more serious and can lead to increased mortality or other adverse outcomes. Reference Peng and Xiang39 The American College of Surgeons Committee on Trauma (ACS-COT) has defined an under-triage rate of < 5% and over-triage rate of < 35% as acceptable. Reference Escobar and Morris40
SALT, a more detailed algorithm used in the USA, was developed in 2008 by the Centers for Disease Control and prevention (CDC) as a newer and more advanced triage system. Reference Lerner, Schwartz and Coule41 This review shows a higher over-triage rate in the pediatric population, which in fact, was an important limitation of the older START protocol. Reference Lee42 Another, more serious problem, arose when evaluating IRR with high numbers of casualties, where SALT had an unacceptable rate of under-triage, specifically in the pediatric population. Reference McGlynn, Claudius and Kaji15
In this review, CF, developed in Australia but currently replaced by Sieve and the PTT in the UK, was 1 of the best algorithms for the pediatric population. It is simple and was found to be accurate in multiple studies. However, as with most triage tools, CF was not adapted specifically to the pediatric population. Driven by the positive results in this review, CF could be used as the basis for development of a pediatric algorithm.
In the UK, the most frequently used algorithm is Sieve and its pediatric adaptation: the PTT. The PTT is a visual tape that is placed next to the patient, like the Broselow® tape. A Sieve algorithm is then applied according to the height of the patient. Although this method is the most adapted to the pediatric population, this review shows that it can also have a high rate of under-triage. Reference Wallis and Carley16 The combination of PTT and other methods (like T-RTS) has been explored but is yet to be validated. Reference Chayen, O’Leary, Major, Dawson and Gosby17
Worthy of note is the fact that most systems share a common weakness, and this is the first step of the algorithm. The assessment of a patient’s state based on their ability to walk has clear limitations when assessing infants and toddlers who are developmentally unable to ambulate independently. This also presents limitations for those who have baseline disabilities affecting their mobility. The assessment of developmentally appropriate mobility requires a certain degree of pediatric expertise. STM is the only system in the identified list which did not rely on the patient’s ability to walk as a method for initial sorting. Unfortunately, the data gathered on STM is conflicting. Furthermore, this algorithm is anchored on specific vital sign values that are adapted to the adult population. Reference Jenkins, McCarthy and Sauer43 Another consideration is that these algorithms are mainly trauma oriented. Pediatric patients may present in different ways in an MCI, being severely compromised but still able to walk. For example, an asthmatic pediatric patient could present walking in an MCI with severe shortness of breath and could be dangerously under-triaged.
On the other hand, the evaluation of secondary triage methods in this review found all 3 studies that determined that PPATS to be better than the more traditional algorithms like T-RTS in predicting mortality and patients needing ICU. This algorithm needs to be prospectively validated in a real-life MCI to further strengthen its external validation as an in-hospital triage system.
Limitations
This review has multiple limitations. This study was first intended to be a systematic review, however, due to the heterogenicity of the articles and the paucity of prospective data, a systematic review was not feasible and instead, a scoping review was done. Another limitation is the rarity of such pediatric MCIs; in fact, most of the articles used combined adult and pediatric data or simulation experience to draw conclusions. The paucity of real-life pediatric-only MCIs is probably a major obstacle in creating an accurate pediatric MCI triage algorithm system.
Conclusion
Many different pediatric MCI triage algorithms are used worldwide. Most primary triage algorithms are either adult algorithms or an adaptation from adult algorithms. Although some algorithms performed better than others in some respects, no primary triage algorithm was found to be superior to the others for the pediatric population. Further research should aim to determine the most important criteria for evaluation of a pediatric triage tool as well as acceptable limits of performance within those criteria. Only 1 secondary triage algorithm, the PPATS, was found to be superior for the pediatric population, because of its higher accuracy in predicting mortality. However, this triage system was compared to only 1 previous triage system and more studies comparing different secondary triage systems are needed. This review highlights a need for a new primary MCI pediatric triage tool that should be adaptable to the range of pediatric age groups.
Supplementary material
To view supplementary material for this article, please visit https://doi.org/10.1017/dmp.2022.287
Funding statement
The authors did not receive any funding for this work.
Conflict declaration
The authors report no conflict of interest. They also declare that this work is their own and every reference used is well cited. This work is not under review in any other journal.
Abbreviations
CDC, Centers for Disease Control and prevention; CF, CareFlight; COVID-19, Coronavirus Disease 2019; ED, Emergency Department; EMS, Emergency Medical Services; GCS, Glasgow Coma Scale; IRR, Inter-Rater Reliability; ISS, Injury Severity Score; JS, JumpStart; MCI, Mass-Casualty Incident; NA, No Algorithm; NISS, New Injury Severity Score; NYFD, New York Fire Department; PAT, Physiological and Anatomical Triage; PPATS, Pediatric Physiological and Anatomical Triage Score; PTT, Pediatric Triage Tape; RCTs, Randomized Controlled Trials; SALT, Sort, Assess, Lifesaving interventions, Treatment/ Transport; STM, Sacco Triage Method; T-RTS, Triage – Revised Trauma Score