Intracranial aneurysm is a serious and potentially life-threatening medical condition. Rupture of the aneurysm may cause fatal intracranial hemorrhage; therefore an accurate diagnosis and timely treatment are crucial. Endovascular embolization using electrochemically detachable platinum coils is the most recent technique for treating intracranial aneurysms.Reference Missler, Hundt, Wiesmann, Mayer and Brückmann 1 This method is less invasive than micro-neurosurgery; however it requires demanding imaging techniques to allow accurate assessment, guidance and verification during the procedure.Reference Bridcut, Murphy, Workman and Flynn 2 Traditionally, two-dimensional (2D) digital subtraction angiography (DSA) was the only technique that could show the critical anatomic features of an intracranial aneurysm. Typically it requires multiple oblique views to visualize the relationship of aneurysm with surrounding vessels. Three-dimensional (3D) rotational angiography (RA) is a relatively newer method which improves the analysis of an aneurysm compared with conventional DSA.Reference Anxionnat, Bracard and Ducrocq 3 – Reference Wong, Nawawi, Ramli and Abd Kadir 5 In this technique individual projections are acquired during the rotation of the C-arm around the patient over an arc using cone-beam computed tomography (CT) geometry. The 3D DSA image is reconstructed using the same maximum intensity projection (MIP), surface-shaded display (SSD), or multiplanar reconstruction (MPR) techniques as used in computed tomography angiography (CTA) and magnetic resonance angiography (MRA).Reference Sugahara, Korogi, Nakashima, Hamatake, Honda and Takahashi 6
Complex imaging guided examinations are typically associated with significant radiation exposure to the patient. It was reported that patient dose in neurointerventional procedures may cause radiation induced deterministic (skin injures) and stochastic (cancer risk) damage.Reference Gkanatsios, Huda and Peters 7 – Reference Raelson, Kanal and Vavilala 9 Therefore, radiation dose must be taken into consideration when comparing alternative techniques. Several previous publications have compared patient dose from these imaging methods used in interventional procedures. Schueler et al concluded that peak skin dose and cumulative incident dose are significantly lower for 3D cerebral angiography than for biplanar DSA.Reference Schueler, Kallmes and Cloft 10 Tsapaki et al reported substantial kerma-area product (KAP) reduction in 3D RA rotational angiography vs. 2D carotid arteriography.Reference Tsapaki, Vano and Muavrikou 11 On the contrary, Kothary et al demonstrated that routine use of C-arm can increase dose-area product (DAP) but decrease cumulative dose from DSA during transhepatic arterial chemoembolization.Reference Kothary, Abdelmaksoud and Tognolini 12 However, variations in 3D RA acquisition methods used by different manufacturers and modifications in DSA acquisition technique (e.g. the dose mode selection, different copper filtration thickness, the air gap between the patient and the image intensifier, etc.) may dramatically affect the resulting patient dose.Reference Wagner, Archer and Cohen 13 If the interventional unit is not equipped with a collimator-mounted dosimeter, then evaluation of patient radiation dose becomes even more challenging. Specific measurements are required to determine accurate DSA and 3D RA dose values for each unique configuration and procedure protocol.Reference Schueler, Kallmes and Cloft 10 Another consideration concerns dosimetric quantities. Entrance skin exposure or DAP values, which are primarily used to estimate deterministic radiation effect, do not provide an accurate dose assessment for intermodality comparison (e.g. conventional angiography vs. cone-beam CT scanning). Therefore, we needed to calculate the effective dose, representing the stochastic effect of radiation resulting from different procedures.
The purpose of this study was to compare patient effective dose resulting from two alternative imaging protocols for pre-coiling assessment of intracranial aneurysms: a series of 2D DSA projections and a 3D RA acquisition. It was recognized that the overall length of the aneurysm coiling procedure depends on the location and size of the aneurysm. Therefore, our investigation was limited to the planning stage of the procedure.
Methods
In a retrospective analysis we investigated the planning studies of forty four patients who underwent endovascular coiling in our institution between January and October 2012. One group of the patients was assessed using multiple DSA biplane views; another group was evaluated with RA technique.
Imaging protocols
All neurovascular studies in our institution were performed on a biplane Image Intensifier system Axiom Artis BA (Siemens Healthcare, Erlangen, Germany) with rotational capability of the C-arm (commercial name DynaCT). The rotational acquisition was performed using cone beam CT geometry. The unit was not equipped with a DAP meter, therefore no entrance dose information was provided. Most of the neuroradiologists used the conventional 2D DSA protocol that included 12 second cine runs with a rate of two frames per second. This acquisition included four standard views: antero-posterior (AP), lateral (LAT) and biplane oblique projections taken from multiple angles. The alternative 3D RA protocol was used by one neuroradiologist (JJSS); it included AP/LAT cine run followed by one rotational acquisition. Each spin involved the acquisition of 128 images during a 200° rotation of the C-arm around the patient’s head resulting in the 3D reconstruction; one rotational acquisition included two spins (mask and contrast). Details of both protocols are shown in the Table.
Table Summary of acquisition parameters and dosimetry results for both protocols: two-dimensional subtraction angiography (2D DSA) and three-dimensional rotational angiography (3D RA).
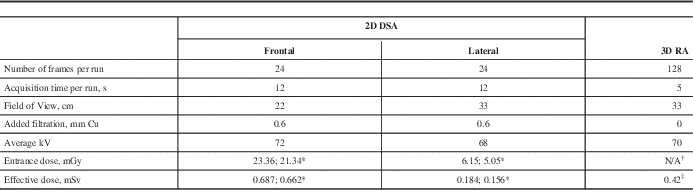
* Oblique projections
† Entrance dose was not measured during rotational angiography
‡ Total dose from 2 rotations
s=seconds, cm=centimetres, mm=millimetres, Cu=copper, kV=kilovolts, mGy=milligray, mSv=millisieverts
Dosimetry measurements and effective dose calculations
An anthropomorphic skull phantom was used for the simulation of conventional 2D DSA; entrance skin exposure was measured with the 60 cc ionization chamber (AccuPro, Radcal Corporation, Monrovia, CA, USA). Figure 1 shows the setup for entrance dose measurements during lateral DSA run using anthropomorphic skull phantom. A Monte Carlo simulation program PCXMC was used to calculate patient effective dose from each projection.Reference Servomaa and Tapiovaara 14 This software simulates the irradiation of a mathematical phantom and allows modeling of arbitrary projection geometries and exposure factors. The input data included the protocol parameters (e.g. field of view, peak kilovoltage (kVp), and x-ray beam filtration), projection angle, and measured entrance skin exposure. The program calculated organ doses and total effective dose using tissue weighting factors from the International Commission on Radiological Protection (ICRP) 103 report. 15 The air kerma from scattered radiation was measured with the 180 cc ion chamber at different locations in the room using the same anthropomorphic skull phantom.

Figure 1 Simulation of 2D DSA with anthropomorphic skull phantom; the 60 cc ion chamber is set up to measure entrance dose during lateral projection cine run.
During the 3D RA acquisition we measured CT Dose Index (CTDI) using an acrylic CT head dose phantom 16 cm diameter and a pencil-type 100 mm long CT ion chamber (Figure 2). The ImPACT (Imaging Performance Assessment of CT scanners) patient dose calculator, Version 1.0.4, was employed to estimate patient effective dose. 16 This software tool uses Monte Carlo data from a mathematical human phantom along with the measured CTDI values for the particular CT system. Data are entered through the graphic interface. For any particular scanner not included in the database (e.g. cone beam CT scanners), an empirically derived ImPACT factor can be calculated and used to match the scanner to one that is included within the database. Tissue weighting factors from the ICRP 103 report 15 were applied as well.

Figure 2 Dosimetry measurements during 3D RA with CT head phantom and a pencil-type 100 mm CT ionization chamber positioned at the center location.
Both imaging protocols, 2D DSA and 3D RA, employ Automated Exposure Control (AEC) technique. Based on the fact that the phantoms represent average patient head size, we made an assumption that the patient dose was the same as the dose obtained from the simulations. Effective dose was calculated for all patients in this study.
Statistical analysis
An unpaired two-tailed t-test was used to determine the significance of differences between the means of patient dose distributions in both groups (the 2D DSA and the 3D RA). A value of p<0.001 was considered significant.
Results
The calculated effective doses were 0.871 millisievert (mSv) for AP/LAT cine run, and 0.818 mSv for two oblique projections. The cumulative effective dose from one rotational acquisition (including two spins) was 0.42 mSv. The scattered radiation dose from the 2D DSA was two to three times higher, depending on the location: e.g. at 152 cm (5’) from the phantom the cumulative air kerma was 4.89 μGy during the biplane cine run and 2.18 μGy during the RA acquisition.
In the group of forty four patients who had a coiling procedure performed in our institution during the considered period, sixteen patients underwent the 2D projection biplane protocol; the mean number of cine runs was 5.1 (minimum 4, maximum 8). Twenty eight patients were assessed using the 3D RA protocol, which included an AP/LAT run and one rotational acquisition. Effective dose for the 3D RA protocol (1.29 mSv) was 39% lower compared to the mean dose in the 2D biplane group (2.11 mSv). The dose range for the 2D DSA protocol was from 1.69–3.43 mSv, based on the number of oblique projections acquired for different patients. Patient effective doses are shown in Figure 3. The lowest dose (first bar) corresponds to the 3D RA protocol. The second bar shows biplane protocol with the minimal number of projections: AP, LAT and two oblique. There were nine patients in this subgroup receiving effective dose of 1.69 mSv. The other patients within the 2D biplane protocol were assessed using the different numbers of runs acquired for them. An unpaired two-tailed t-test returned a p-value of 0.00028 (<0.001), demonstrating a significant difference between the means of two dose distributions (the 2D DSA and 3D RA protocols).

Figure 3 Patient effective dose distribution: the first bar corresponds to the 3D RA dose of 1.29 mSv (28 patients); the second bar (1.69 mSv) shows the most common AP/LAT + Oblique/Oblique projection 2D DSA (9 patients in this group). The other bars demonstrate doses from different numbers of projections for the remaining patients in the 2D DSA group.
Discussion
Three dimensional RA is being used for the assessment of intracranial aneurysms and has been used for their preoperative planning for a number of years.Reference Pedicelli, Rollo, Di Lella, Tartaglione, Colosimo and Bonomo 17 It has also been shown that the 3D RA is actually better than the conventional 2D biplane angiogram for the assessment of an intracranial aneurysm.Reference Hochmuth, Spetzger and Schumacher 18 However, many operators still do not use 3D RA in their routine practice for their presumed fear of higher radiation dose associated with 3D RA. Intuitively, 3D RA may appear to be associated with a higher radiation dose due to the higher number of frames required for image reconstruction comparing to the DSA biplane series.Reference Kothary, Abdelmaksoud and Tognolini 12 However, dose per frame is lower in RA acquisition and a comparison of these two methods is not straightforward as it is influenced by many technical factors. This pseudo-fear and thereby limited experience with 3D RA, has lead to a significant underuse of this new technique. Interventionists who are not using 3D RA in their practice usually do multiple oblique projections to assess the relationship of the aneurysm with its parent artery. Our study shows that, contrary to popular belief, 3D RA has lower radiation dose compared to 2D biplane angiogram. We hope this message will help many users to adopt 3D RA in their routine practice of coiling aneurysm and thereby will eventually result in a lowering of the overall radiation dose to patients undergoing endovascular coiling.
Effective dose is the most commonly used quantity to compare stochastic risk from different radiological procedures. Therefore, we needed to assess patient effective dose resulting from both imaging protocols. For the 2D DSA it was a straightforward method to measure entrance dose using phantom and calculate effective dose with the PCXMC software. However, we needed to define methodology for dosimetry measurements and calculations of the effective dose for the 3D RA acquisition. When the x-ray system is equipped with DAP meter, unlike our Axiom Artis BA, it is feasible to extract dose data for every frame of the rotational acquisition and to calculate corresponding effective doses for each projection using the PCXMC tool.Reference Bridcut, Murphy, Workman and Flynn 2 , Reference Wielandts, Smans, Ector and De Buck 19 Another approach to cone-beam CT dosimetry is based on traditional CTDI metrics, recognizing that the 100 mm ion chamber has limited utility due to a larger field of view in cone beam CT geometry. More accurate measurements could be done with the longer (250 mm) pencil ion chamberReference Kyriakou, Richter, Dorfler and Kalender 20 , or a smaller 0.6 cc Farmer ion chamber for point dose measurementsReference Fahrig, Dixon, Pane, Morin, Ganguly and Strobel 21 , Reference Dixon and Boon 22 However, routine Quality Control tests in CT are based on CTDI measurements with the 100 mm pencil ion chamber, and other dosimetry equipment might not be easily available in the diagnostic imaging department. The ImPACT dose calculator is based on the CTDI100 measurements as well. When the scanner is not included in the software package database it is possible to find the match using an empirically derived “ImPACT factor”, calculated from the ratios of the CTDI100 values at center and periphery to air measurements. The ImPACT factor allows matching the scanner to the nearest equivalent one, included with the calculator. Evaluation of the ImPACT utility for the cone beam CT applications has been reported in the literature where it was found that calculations were generally consistent with organ doses measured with thermoluminescent dosimeters (TLD) placed at various locations inside the Rando phantom.Reference Sawyer, Whittle, Matthews, Starritt and Jupp 23 , Reference Amer, Marchant, Sykes, Czajka and Moore 24 This method was adopted in our study for 3D RA patient dose calculations.
It was a limitation of our study that only specific equipment and protocols were investigated; however, our goal was to evaluate procedures performed in our institution to establish better patient practices. With the availability of DAP meter on newer DSA equipment, we may not have to do these complex dose calculations. However, since current DSA equipment used in practice do not have dose information available, this method is still relevant.
Conclusions
Our comparison of two alternative imaging protocols demonstrated that patient effective dose and scatter radiation to the staff were significantly lower from the 3D RA than that from the 2D projection DSA acquisitions used in the planning of cerebral aneurysm coiling. Based on the results of our study, the group of neuroradiologists in our institution was encouraged to implement rotational angiography acquisition for assessment of cerebral aneurysm. The use of rotational angiogram may potentially obviate the need for multiple oblique angiograms in this group of patients.
Disclosures
The authors have nothing to disclose.