Introduction
Cold winters represent a major barrier to the permanent establishment of invasive species such as Drosophila suzukii (Diptera: Drosophilidae) in cold-temperate regions such as Canada (Walsh et al. Reference Walsh, Bolda, Goodhue, Dreves, Lee and Bruck2011; Zerulla et al. Reference Zerulla, Schmidt, Streitberger, Zebitz and Zelger2015; Rossi-Stacconi et al. Reference Rossi-Stacconi, Kaur, Mazzoni, Ometto, Grassi and Gottardello2016; Shearer et al. Reference Shearer, West, Walton, Brown, Svetec and Chiu2016; Stockon et al. Reference Stockon, Wallingford, Rendon, Fanning, Green and Diepenbrock2019). In cold climates, to survive winters until the next season, D. suzukii should respond adaptively to cues signalling end of summer by expressing a true reproductive diapause (Danks Reference Danks2006; Denlinger Reference Denlinger2008; Salminen and Hoikkala Reference Salminen and Hoikkala2013; Zerulla et al. Reference Zerulla, Schmidt, Streitberger, Zebitz and Zelger2015; Rossi-Stacconi et al. Reference Rossi-Stacconi, Kaur, Mazzoni, Ometto, Grassi and Gottardello2016; Shearer et al. Reference Shearer, West, Walton, Brown, Svetec and Chiu2016; Zhai et al. Reference Zhai, Lin, Zhang, Zhang, Zheng and Yu2016). Then, having responded appropriately, diapausing flies must tolerate cold stress for at least six months at harmful temperatures well below their activity and metabolic thresholds (Rossi-Stacconi et al. Reference Rossi-Stacconi, Kaur, Mazzoni, Ometto, Grassi and Gottardello2016).
Overwintering in a cold-temperate region also implies that adult longevity should persist long after winter because D. suzukii’s oviposition and larval development depend on ripe fruits (e.g., Lee et al. Reference Lee, Bruck, Curry, Edwards, Haviland, Van Steenwyk and Yorgey2011; Winkler et al. Reference Winkler, Jung, Kleinhenz and Racca2020). Postdiapause reproductive maturity would not be recovered until temperatures reach thermal threshold for gametogenesis, estimated to be between 5 °C and 10 °C (Walsh et al. Reference Walsh, Bolda, Goodhue, Dreves, Lee and Bruck2011; Rota-Stabelli et al. Reference Rota-Stabelli, Blaxter and Anfora2013; Arnó et al. Reference Arnó, Solà, Riudavets and Gabarra2016; Gutierrez et al. Reference Gutierrez, Ponti and Dalton2016; Hamby et al. Reference Hamby, Bellamy, Chiu, Lee, Walton and Wiman2016; Ryan et al. Reference Ryan, Emiljanowicz, Wilkinson, Kornya and Newman2016; Little et al. Reference Little, Chapman, Moreau and Hillier2017; Alhmedi et al. Reference Alhmedi, Clymans, Van Kerckvoorde, Bylemans and Beliën2019; Winkler et al. Reference Winkler, Jung, Kleinhenz and Racca2020). Sufficient physiological time (effective heat units or degree-days above development threshold temperature) must then accumulate until prereproductive maturation, sexual encounter and mating, and ultimately egg laying by fertile females in early maturing fruits (Winkler et al. Reference Winkler, Jung, Kleinhenz and Racca2020).
In a three-year field study in the Saguenay–Lac-Saint-Jean region, Québec, Canada, Champagne-Cauchon et al. (Reference Champagne-Cauchon, Guay, Fournier and Cloutier2020) found D. suzukii to be a regular, minor pest on lowbush blueberry, where it had been trapped since 2012. In blueberry fields and surrounding areas, D. suzukii is widespread and abundant in late summer and fall, but no direct evidence of local overwintering was found. Nevertheless, high densities of dark morph, reproductively diapausing D. suzukii flies, occurred in late fall, suggesting the possibility of local overwintering (Champagne-Cauchon et al. Reference Champagne-Cauchon, Guay, Fournier and Cloutier2020). Studies to date in the invasive range of D. suzukii indicate that cold-acclimated flies are chilling intolerant (Tochen et al. Reference Tochen, Dalton, Wiman, Hamm, Shearer and Walton2014; Jakobs et al. Reference Jakobs, Gariepy and Sinclair2015; Ryan et al. Reference Ryan, Emiljanowicz, Wilkinson, Kornya and Newman2016; Langille et al. Reference Langille, Arteca and Newman2017; Nikolouli et al. Reference Nikolouli, Colinet, Renault, Enriquez, Mouto and Gibert2018; Winkler et al. Reference Winkler, Jung, Kleinhenz and Racca2020), which would preclude winter survival under natural conditions in Canada and in the northeastern United States of America, except in natural microhabitats protected from freezing or in heated anthropic environments (e.g., Zerulla et al. Reference Zerulla, Schmidt, Streitberger, Zebitz and Zelger2015; Stockon et al. Reference Stockon, Wallingford, Rendon, Fanning, Green and Diepenbrock2019).
Cloutier et al. (Reference Cloutier, Guay, Champagne-Cauchon and Fournier2021) studied D. suzukii’s winter survival by exposing field collected flies from Québec to realistically simulated winter regimes of cold stress that lasted six months, followed by a return to springtime conditions, while assuming that fall-acclimated flies select microhabitats with low risk of extensive freezing. Despite substantial mortality after 180 days of cold stress, numerous D. suzukii flies of both sexes survived. When transferred to springtime rising temperatures and increased daylength based on regional norms, life expectancy distributions indicated that winter survivors (10–30% of flies tested) would still be alive in June and up to July of next year (Cloutier et al. Reference Cloutier, Guay, Champagne-Cauchon and Fournier2021). The flies were from late-season, high-density populations (Champagne-Cauchon et al. Reference Champagne-Cauchon, Guay, Fournier and Cloutier2020), as previously reported (e.g., Thistlewood et al. Reference Thistlewood, Gill, Beers, Shearer, Walsh and Rozema2018). Despite the substantial mortality that was seen, it cannot be discounted that many D. suzukii might survive local winters to resume reproduction in spring. Even males survived the 180-day cold winter (Cloutier et al. Reference Cloutier, Guay, Champagne-Cauchon and Fournier2021), which suggests that both sexes of postdiapause flies could eventually mate after winter to reproduce in the next season. For survivors with no opportunity to mate before diapause, mating after winter would be essential to start a new generation in spring. The need to mate in spring would necessarily apply to males, as well as to females that might have mated before winter but are no longer fertile come spring, as is discussed in Ryan et al. (Reference Ryan, Emiljanowicz, Wilkinson, Kornya and Newman2016) and has been shown in D. montana (Ala-Honkola et al. Reference Ala-Honkola, Kauranen, Tukmaeva, Boetzl, Hoikkala and Schmitt2018).
Reproductive diapause is common among insects that overwinter in cold-temperate climates (Tauber et al. Reference Tauber, Tauber and Masaki1986; Danks Reference Danks1987; Denlinger Reference Denlinger2008). In multivoltine species such as D. suzukii, winter-diapausing flies probably experience fitness costs incurred by overwintering that nondiapausing flies of summer generations do not experience (Tauber et al. Reference Tauber, Tauber and Masaki1986; Danks Reference Danks1987; Sadakiyo and Ishihara Reference Sadakiyo and Ishihara2012a, Reference Sadakiyo and Ishihara2012b). Even at a low metabolic rate, the use of stored energy reserves for prolonged diapause likely negatively affects fitness in postdiapause versus nondiapause flies (Hahn and Denlinger Reference Hahn and Denlinger2007). Reproductive diapause also implies time and energy after dormancy to recover reproductive maturity, to mate at low population densities caused by winter mortality and among sex-biased conspecifics. For winter-diapausing females, the extra energy expenditures associated with diapause might reduce longevity and fertility compared to nondiapausing females. For males, the additional costs of diapause might be reflected in the reduced fertility of their female partners. Knowing the fitness costs of diapause in D. suzukii thus is of considerable interest, given that both sexes can survive cold stress periods equivalent to continental cold-temperate winters (Cloutier et al. Reference Cloutier, Guay, Champagne-Cauchon and Fournier2021). Documenting these fitness costs is possible by comparing lifetime fecundity of postdiapause and nondiapause flies in simulated, controlled springtime conditions. Knowing the species’ postdiapause reproductive potential would be useful for better predicting D. suzukii’s future expansion and potential to establish in temperate regions outside its native range (e.g., Ørsted and Ørsted Reference Ørsted and Ørsted2019).
The present study’s objective was to experimentally evaluate the early season reproductive dynamics of D. suzukii in simulated cold-temperate springtime conditions. We assessed the reproductive fitness of long-lived postdiapause flies that survived a cold winter that is typical of eastern Canada, as reported by Cloutier et al. (Reference Cloutier, Guay, Champagne-Cauchon and Fournier2021). Following cold-winter stress treatments that lasted six months, surviving male and female flies were monitored for reproductive potential in abiotic conditions that simulated spring and early summer. We expected that postwinter mating might be a critical requirement for postdiapause reproduction, even among females that may have mated the previous fall. We hypothesised that, due to the fitness costs of diapause, fitness would be reduced in postdiapause flies compared to that in nondiapause flies of summer generations. Our objectives were (1) to demonstrate that postdiapause cold-winter survivors of both sexes of D. suzukii can reproduce successfully in the next season; (2) to compare the reproductive potential of postdiapause D. suzukii to that of nondiapause D. suzukii from a laboratory colony (see Supplementary material for details) as a way of evaluating the fitness cost of overwintering in diapausing flies; and (3) to project potential springtime population growth from small numbers of flies, including both potential local winter survivors and nondiapause potential incoming migrant flies from elsewhere.
Methods
Insects
Male and female D. suzukii flies were live trapped in lowbush blueberry fields in late summer and fall 2017, in the Saguenay–Lac-Saint-Jean region, Québec, Canada. Postdiapause flies were springtime survivors of the second-year overwintering survival experiment of Cloutier et al. (Reference Cloutier, Guay, Champagne-Cauchon and Fournier2021). Flies had survived a cold-winter sine-wave fluctuating temperature regime of 3 ± 3 °C. Postdiapause male and female survivors were followed for the remainders of their lives to determine their reproductive potential in a realistic regime of improving abiotic conditions. In April and May 2018, at the end of the six-month cold-winter treatment period, survivors were transferred to a growth chamber that was programmed with daily fluctuating temperatures based on 10-year spring–summer data for Normandin, Saguenay–Lac-Saint-Jean region, Québec, Canada (48° 50' 30 000 N, 72° 32' 49 000 W; Fig. 1). The reproductive potential of postdiapause females was measured as lifetime fecundity and, for postdiapause males, as lifetime fecundity of female partners (see below). We took into account the results of an exploratory experiment conducted in 2017 (see Supplementary material 1) that showed that postdiapause winter survivors indeed could reproduce in simulated springtime conditions typical of the study region.

Fig. 1. Spring–early summer regime of daylength and fluctuating temperatures used to simulate temperate climatic conditions in monitoring lifetime fecundity of Drosophila suzukii. From first week of July, mean temperature of fluctuating temperature regime was fixed at 20 °C to avoid higher values beyond the optimal range of the species.
Observation units consisted either of isolated females or of couples of D. suzukii, for which female lifetime fecundity was measured while controlling for male presence. Dying males in couples were not replaced, but overall, a male was present during 73–85% of females’ reproductive lifetime, depending on the mating treatment. Having found previously (Supplementary material 1) that postdiapause mating was critical for postdiapause reproduction, mating treatments were added to examine postdiapause reproductive potential and to estimate the fitness costs of diapause by comparing fertility between diapause and nondiapause flies. Postdiapause females either were matched to a young male from a laboratory colony or remained isolated (no mate) to test the hypothesis that they might still retain viable sperm if they had mated the previous year (2017) before trapping. A colony was initiated in summer 2016 with flies trapped in blueberry fields, and it was maintained on a banana diet using a technique similar to Gonzalez-Cabrera et al. (Reference Gonzalez-Cabrera, Garcia-Cancino, Moreno-Carrillo, Sanchez-Gonzalez and Arredondo-Bernal2018), at 20 °C, 65% relative humidity, and a 16:8-hour light:dark photoperiod. The reproductive potential of postdiapause males, which were less numerous than females, was based on the lifetime fecundity of a virgin nondiapause female partner from the colony or of a postdiapause female. The experiment thus involved six mating treatments: (1) virgin nondiapause female from colony coupled with overwintered (postdiapause) male (CFOM); (2) both female and male from colony (nondiapause control treatment; CFCM); (3) virgin colony female without male (CF), testing for possible parthenogenesis; (4) overwintered (postdiapause) female without male (OF), testing for fertility based on viable sperm retention from mating in 2017; (5) postdiapause female coupled to nondiapause male (OFCM); and (6) couples formed by postdiapause females and postdiapause males (OFOM). The results of these treatments would allow us to determine if postdiapause females (OF) could reproduce with and without mating in spring and if they could mate effectively with either a colony male (CM) or a postdiapause male (OM). Similar reasoning applied in testing the reproductive potential of overwintered (postdiapause) males. Available females and males were randomly allocated to treatments, with the obvious restriction that, because the number of surviving males was lower than females, postdiapause male availability limited the number of replicates for treatments involving overwintered males. For treatments involving nondiapause colony flies (CF, CM), newly emerged (within 24–48 hours) colony flies that had been collected as pupae were used.
For consistency, we use the terminology of Henderson and Southwood (Reference Henderson and Southwood2016, section 11.4) when referring to the life table and reproductive rate of D. suzukii. We therefore refer to lifetime fertility of adult flies of known age and mating status – that is, control colony flies – reared in the laboratory or of flies of unknown age and mating status, trapped in nature and experimentally overwintered. Isolated females and couples were followed during their lifetimes in the predetermined regime of simulated springtime conditions (Fig. 1). Consequently, in the case of postdiapause flies, the term, “age-specific”, does not mean the true chronological age of the individual flies: it means their ageing-with-time during the experiment after they had emerged from winter dormancy upon being transferred to the springtime-simulated conditions.
Reproduction was monitored by counting larvae dissected from fruits exposed at three- to four-day intervals during each individual fly’s lifetime. Female fertility therefore represents hatched (larval) offspring born into the next generation – the net reproductive rate (R 0). For each female, R 0 also included the larvae found in blueberries that were dissected to monitor the results of ovipositor marking of exposed fruits by subsamples of females assigned to each treatment. Eggs laid on exposed fruits were not counted, but for up to five consecutive two-day periods, the skin of exposed blueberries was examined under a stereomicroscope to count small slits (ovipositor marks) left by females assigned to each mating treatment. We thus used egg-laying marks as a proxy for the number of eggs laid and compared the number of marks to the number of larvae found when we dissected the fruits after two-day exposure. We did not attempt to determine if eggs remained present in the ovipositor marks because fruits were examined and dissected for larvae within 24 hours of being exposed to flies. To calculate an “egg hatching ratio”, the number of ovipositor marks was compared to number of larvae found upon dissection of each fruit, with the assumption that one mark made on fruit skin corresponds to one egg laid. Low values of this ratio could reveal marks without oviposition – that is, marks made for, for example, feeding or for laying unfertile eggs (Atallah et al. Reference Atallah, Teixeira, Salazar, Zaragoza and Kopp2014). High values could represent more than one egg laid per mark or larval entry into the fruit without an apparent ovipositor stabbing.
Statistical analysis
With enough replicates available, life table data for this experiment were statistically analysed to compare reproductive performance between mating treatments. The normal distribution hypothesis could not be met for many life table variables (see, e.g., Supplementary material 2, Fig. S2-1), based on the Shapiro–Wilk statistic. We therefore used the distribution-free Kruskal–Wallis test, which is a nonparametric version of traditional one-way analysis of variance (Critchlow and Fligner Reference Critchlow and Fligner1991). When the Kruskal–Wallis chi-square statistic was significant (P ≤ 0.05), we used the dunnTest function of package FSA of R (Mangiafico Reference Mangiafico2021; R Studio Team 2021), which implements Dunn’s (Reference Dunn1964) method for multiple comparisons, with the Benjamini–Hochberg correction to control the experiment-wise error rate.
To estimate the population growth rates of postdiapause D. suzukii under the simulated springtime regime, we first used Henderson and Southwood’s method (Reference Henderson and Southwood2016, Chapter 11, Equation 11.30), based on “intrinsic capacity for increase” (r c), as generally used by insect ecologists. Capacity for increase was calculated using the equation: r c = log(R 0 /T c ), where R 0 is the mean net reproductive rate of the experimental cohorts of flies assigned to each treatment. R 0 was calculated as the sum of all daughter progenies born from females over lifetime, R 0 = ∑l x m x , where l x and m x represent age-specific mortality and fecundity, respectively. T c is the cohort “generation time”, or pivotal age – that is, the mean age of females at the time of offspring birth, when 0.5 R 0 under experimental conditions (springtime) had been realised, assuming a 1:1 sex ratio among offspring. Age 0 is female chronological age since the beginning of the experiment.
To model and predict springtime population growth based on the reproduction of postdiapause D. suzukii flies over lifetime, the cumulative fecundity data (i.e., cumulative larval offspring counts in dissected blueberries) were fitted to a modification of the Gompertz model. This model has been widely used to analyse population growth curves when the growth rate (r) varies in response to population density (Eberhardt et al. Reference Eberhardt, Breiwick and Demaster2008), as it similarly varied in the present study due to the ageing of females during lifetime. The model formulation used is

where F is the asymptotic value of lifetime fecundity, as adult females age with time, r is the maximum rate of population increase during lifetime due to natality, and t max is the time at the inflexion point of the population growth curve.
The model describes D. suzukii population growth resulting from reproduction of flies assigned to the different mating treatments as their reproductive rate changed with time due to ageing in springtime conditions. It is mathematically similar to a two-parameter classical Gompertz model (Eberhardt et al. Reference Eberhardt, Breiwick and Demaster2008). Asymptotic fecundity (F) is analogous to K in the Gompertz model, and instantaneous fecundity rate (r) is mathematically analogous to the density-dependent per capita growth rate. The third parameter, t max, is a location parameter that shifts the curve horizontally without changing it shape. It is the time at the inflexion point of the curve for which we have 36.8% of F, the upper asymptote (Tjørve and Tjørve Reference Tjørve and Tjørve2017). From this model, we can also estimate the time at which N(t) = 1, corresponding to time when fly reproduction started, allowing the estimate of the extent to which the starting time was affected by female diapause and mating status. By rearranging the terms in the model equation, we can estimate time when oviposition started as:

Parameter estimates for fecundity data obtained from different treatments were compared using Cochran’s chi-square test (Cochran Reference Cochran1952).
Results
In spring 2018, D. suzukii flies of both sexes were tested for reproductive fitness in a design involving six mating treatments comparing postdiapause and nondiapause flies under simulated springtime conditions. During testing, data on ovipositor marking of fruits by females were collected at two-day intervals for 14 days on average, which allowed us to relate fruit marking to fertility, as confirmed by number of larvae present in marked fruits. Survival data for treatments involving fly couples indicated that males generally died before their female mates. The mean proportion of a female’s lifetime when a live male partner was present varied from 37% (OFOM treatment) to 89% (CFOM), with differences being insignificant (χ 2 = 4.0152, P = 0.2735; data not shown).
Life table schedule
Isolated females without male partners (Table 1; CF treatment, N = 10) lived for 63.20 days on average and produced no progenies, as was expected (Table 1). By comparison, mean longevity of the (chronologically much older) postdiapause females without male partners (OF treatment, N = 10) was 45.90 days, and none produced progeny (Table 1). In control couples consisting of nondiapause male and female flies from the colony (Table 1; CFCM treatment, N = 13), females lived 64.15 days on average. They were fertile for 37.23 days and produced 58.46 offspring (Table 1). By comparison, the mean lifetime of the postdiapause females mated to nondiapause males was 48.27 days; they were fertile during 11.55 days (mean) and produced 9.50 progeny (mean; Table 1). Statistical analysis showed that differences in life statistics between similarly mated postdiapause females (OFCM) and nondiapause (CFCM) females mated to nondiapause males were significant for lifetime (P = 0.0311), reproductive period (P = 0.0017), and total offspring (P = 0.0036). Postdiapause female fertility was thus about six times less than for nondiapause females. Few replicates of treatments involved postdiapause males (OM; Table 1). Postdiapause males mated to postdiapause females (Table 1; OFOM treatment, N = 2) survived a modest fraction of her lifetime (0.37 ± 0.10 standard error) but did not reproduce, as their postdiapause partners left no progeny despite extensive mean longevity (67.00 days). The postdiapause males coupled to colony (nondiapause) females (Table 1; CFOM treatment, N = 3) survived over most of their female partners’ lifetimes (0.89 ± 0.05 standard error), averaging 51.67 days. Postdiapause males were shown to be fertile as their nondiapause female partners produced 17.67 progeny (mean) over a mean reproductive period of 24.67 days. Statistical analysis indicated that the reproductive period and total progenies of nondiapause females mated to postdiapause males did not significantly differ from those of females in the other treatments (Table 1); this is attributable to insufficient statistical power because of the small number of replicates (N = 3). Indeed, compared to the fertility of the nondiapause female partners of nondiapause males, postdiapause males appeared about 3.3 times less fertile (Table 1; progeny mean of 17.67 versus 58.46).
Table 1. Longevity and reproductive performance (mean ± standard error) of Drosophila suzukii in experiment involving mating treatments. Mated and unmated females were monitored for oviposition on blueberries until they died. Within columns, means sharing a letter are not statistically different based on Kruskal–Wallis analysis of variance.

1 Defined as female (F) of known diapause status (CF, nondiapause from colony; OF, postdiapause – i.e., having survived cold-winter temperatures), left unpaired or crossed with male (M) of similarly known diapause status (CM, OM).
2 Total number of females observed for each treatment.
3 Sample size for statistical analysis.
Monitoring fruit marking
The unmated postdiapause females left no marks on fruits (Table 2). Because of this, this treatment (OF) was excluded from statistical analysis. The total number of marks between other treatments varied markedly but with no significant differences (P > 0.05).
Table 2. Ovipositor marking by Drosophila suzukii on blueberry fruits in the 2018 experiment (see Supplementary material 2). Postdiapause female (OF) and male (OM) survivors to six months cold-winter treatment are compared to nondiapause females (CF) and males (CM) from a laboratory colony. Within columns, means sharing the same letter are not statistically different based on Kruskal–Wallis analysis of variance.

1 Defined as female (F) of known diapause status (CF, nondiapause from colony; OF, postdiapause, having survived cold winter), left unpaired or crossed with male (M) of similarly known diapause status (CM, OM).
2 Total number of females observed.
3 Sample size for statistical analysis (flies in OF treatment marked no fruits).
4 Daily number of ovipositor marks left on blueberry fruits.
5 Ratio of number of larvae in dissected fruits to ovipositor marks on fruits.
To account for the monitoring period of fruit marking not being strictly controlled, we calculated daily rate of fruit marking to allow direct comparison of marking frequency between treatments (Table 2). The resulting daily rate of marking ranged from 1.00 marks.d–1 to 3.01 marks.d–1 but had no significant treatments effect (Table 2). Unmated nondiapause (colony) females left abundant marks on fruits, but no offspring emerged from exposed blueberries (Table 2, CF). Nondiapause females coupled to nondiapause males left, on average, the same number of marks on fruit as the unmated nondiapause females did (Table 2, CF versus CFCM). The ratio of larvae to marks significantly differed between treatments (Table 2): the ratio was more than four times higher for nondiapause females than for postdiapause females when both were mated to nondiapause males (Table 2, CFCM versus OFCM), indicating a significant reduction of egg hatching in postdiapause females. In couples formed of postdiapause flies of both sexes (OFOM), only one of the two females left marks, producing high variance (Table 2). The ratio of larvae to marks for nondiapause females mated to a postdiapause male or to nondiapause male (OFOM versus CFCM) did not significantly differ, indicating similar egg-hatching success (Table 2).
Intrinsic population increase rate
Monitoring lifetime fecundity for flies in each treatment involved a maximum of 25 successive observation periods from mid-May to end of July 2018. We defined 25 consecutive age classes of adult flies experiencing similar conditions, for which age-specific mortality (l x) and age-specific fertility (m x) were calculated. The intrinsic capacity for increase (r c) over springtime varied, from 0.0726 d–1 for nondiapause female partners mated to postdiapause males (CFOM) to 0.0771 d–1 for postdiapause females mated to nondiapause males (OFCM; Table 3). By comparison, the (rc ) of nondiapause females mated to nondiapause males (CFCM) in the same conditions was 0.087 d–1; that is, 1.0–1.5% higher than for postdiapause females of nondiapause males and for nondiapause female partners of postdiapause males (CFOM; Table 3).
Table 3. Expected population growth rates of Drosophila suzukii in simulated cold-temperate springtime conditions, as affected by mating treatments in the 2018 experiment (see Supplementary material 2).

1 Defined as crosses of female (F) and male (M) of known diapause status: CF and CM, nondiapause colony flies; OF and OM, flies having survived cold-winter treatment (postdiapause).
2 Expected number of daughters produced during lifetime (cumulative l x m x).
3 Days since the beginning of simulated spring (true chronological age of postdiapause flies unknown).
Modelling population growth potential
The three-parameter Gompertz model provided good fit for the data on female fecundity as affected by age and mating treatments, with R-square values exceeding 98% (Fig. 2). The statistical analysis (Table 4) indicated that maximum instantaneous population growth rate of nondiapause-mated flies (CFCM; 0.0573 d–1) was significantly lower than that of the postdiapause flies in both the OFCM (0.1019 d–1) and CFOM (0.1435 d–1) treatments. Paired contrasts (Table 5) showed that all observed r differences were significant, with P-values ranging from 0.001 to 0.0168, with the r estimates for the OFCM and CFOM treatment being the least different. The asymptotic fecundity parameter (F) also varied strongly with treatments: its value for nondiapause flies (CFCM) was 2–3 times higher than for postdiapause flies (OFCM and CFOM; Table 4). Contrasts showed that F parameters for mating pairs involving each sex as the postdiapause individual (OFCM, CFOM) were highly significantly lower (P = 0.0018 and P < 0.001, respectively; Table 5) than the F parameter for nondiapause male and female mates (CFCM) was. Moreover, the difference between the types of partners for postdiapause males and postdiapause females was not significant (P = 0.2958; Table 5). The third parameter, time at inflexion (t max), also varied with treatments (Table 4), indicating that, for both treatments involving nondiapause females (CFCM and CFOM), the maximum rate of egg laying occurred earlier than it did for postdiapause females (OFCM). Paired contrasts showed that t max differences between both treatments involving nondiapause females (CFCM, CFOM) were not significant (P = 0.1014; Table 5). In contrast, both differences between treatments involving postdiapause females compared to nondiapause females (OFCM versus CFCM; OFCM versus CFOM) were highly significant (P < 0.0001; Table 5). The calculated time when reproduction started (t N(t) = 1) also varied between treatments (Table 4) and occurred much earlier in the treatment involving nondiapause mates (CFCM) compared to treatments involving a postdiapause mate of either the female or male sex (CFOM, OFCM). Paired contrasts showed that oviposition in treatments involving a postdiapause mate of either sex was significantly delayed compared to the treatment involving both nondiapause mates (P < 0.0001; Table 5), with no significant difference between treatments involving a postdiapause male or female (P = 0.5103; Table 5).

Fig. 2. Three-parameter Gompertz models fitted to data on Drosophila suzukii cumulative fecundity over time (t in days) as affected by mating treatments: A, fecundity of nondiapause females mated to nondiapause males; B, postdiapause females mated to nondiapause males; and C, postdiapause males mated to nondiapause females. Black curves illustrate fitted model with parameters specific to each treatment. Horizontal and vertical lines in each graph correspond to estimated parameters asymptotic fecundity (F), and delay before egg laying (t max) following transfer to springtime conditions, respectively.
Table 4. Gompertz model parameters fitted to 2018 data on Drosophila suzukii female reproduction to project population growth in the new season and compare time delay before egg laying (intersection with time axis).

1 Defined as crosses of female (F) and male (M) flies of known diapause status, either nondiapause from the colony (CF, CM) or having survived cold-winter treatment (postdiapause; OF, OM).
2 r, maximum reproductive rate; F, asymptotic cumulative net fecundity; t max, time to maximum reproductive rate; t N(t) = 1, time to the start of reproduction (intersection with X-axis).
Table 5. Paired contrasts between model parameters fitted to 2018 data on Drosophila suzukii female reproduction as affected by mating treatments.

1 Contrasted treatments defined as crosses of female (F) and male (M) flies of known diapause status, either undiapause from colony (CF, CM) or having survived cold-winter treatment (i.e., postdiapause; OF, OM).
r, maximum reproductive rate; F, asymptotic cumulative net fecundity; tmax, time to maximum reproductive rate; and tN(t) = 1, time to the start of reproduction (intersection with X-axis).
Ageing effects on female fecundity varied strongly between nondiapause and postdiapause flies (Fig. 3). The broadest, most-productive age-specific fecundity pattern was that of nondiapause mates (CFCM; Fig. 3); however, the highest peak fecundity was attained by nondiapause females mated to postdiapause males (CFOM; Fig. 3).

Fig. 3. Age-specific daily fecundity patterns of Drosophila suzukii in cold-temperate springtime conditions, as revealed by the instantaneous slope of Gompertz model fitted to larval production data for postdiapause and nondiapause flies from the 2018 experiment (see Supplementary material 2).
Springtime population growth and heat accumulation
Results of the above analysis are illustrated in Figure 4 to allow comparisons of initial population growth potential expected at low population density in spring in cold-temperate conditions. Cumulative degree-days in Figure 4 are based on historic climatic records for the region of fly origin, allowing the prediction of potential growth from postdiapause flies that survive winter locally and mate with potential migrant flies colonisers. Offspring production involving postdiapause flies (Fig. 4, trends CFOM and OFCM) started about two months after winter in early June, first with the females near 1 June, followed by nondiapause females mated to postdiapause males near 8 June. Cumulative physiological time (degree-days) since cold winter ended would then be 118 and 178 degree-days above the 5 °C low-temperature threshold, respectively. Per capita offspring contributed by postdiapause females reached a plateau of 18–19 offspring around 6 July, when heat units would cumulate 640 degree-days5 ˚C (Fig. 4). For nondiapause females sired by postdiapause males, a total of 10–11 offspring would be attained at the same time near 6 July, with 640 degree-days5 ˚C. Similar analysis for mating treatments involving nondiapause potential migrant flies (Fig. 4) shows that their per capita offspring contribution to a new generation could reach 60 offspring around 8 July, with 715 degree-days5˚C of cumulated heat units.

Fig. 4. Cumulative fecundity of postdiapause males (CFOM treatment) and females (OFCM treatment) of Drosophila suzukii compared to nondiapause fly mates (CFCM), in relation to cumulative physiological time in degree-days (DD) above the temperature threshold, 11.1 °C. Postdiapause flies of each sex were mated with a nondiapause partner from a colony of same geographic origin.
Figure 5 schematically summarises results of the 2018 experiment, expressed as per capita lifetime net contribution to population growth as female larval offspring in a new season, assuming a 1:1 sex ratio among the offspring of the flies initiating population growth. Postdiapause females could contribute about five daughters per capita if mated by a fertile nondiapause migrant male. Otherwise, postdiapause females mated by a postdiapause male could contribute a maximum of one daughter offspring, with little evidence they would remain fertile from mating in the previous season. On the other hand, fertile nondiapause females mating with a postdiapause male could contribute eight to nine daughters per capita. By comparison, young nondiapause migrant female flies mated to nondiapause males and arriving early to commence reproduction at about the same time could contribute as many as 30 daughters per capita (Fig. 5), a considerably larger number than that predicted for postdiapause females or for the nondiapause female partners of postdiapause males.
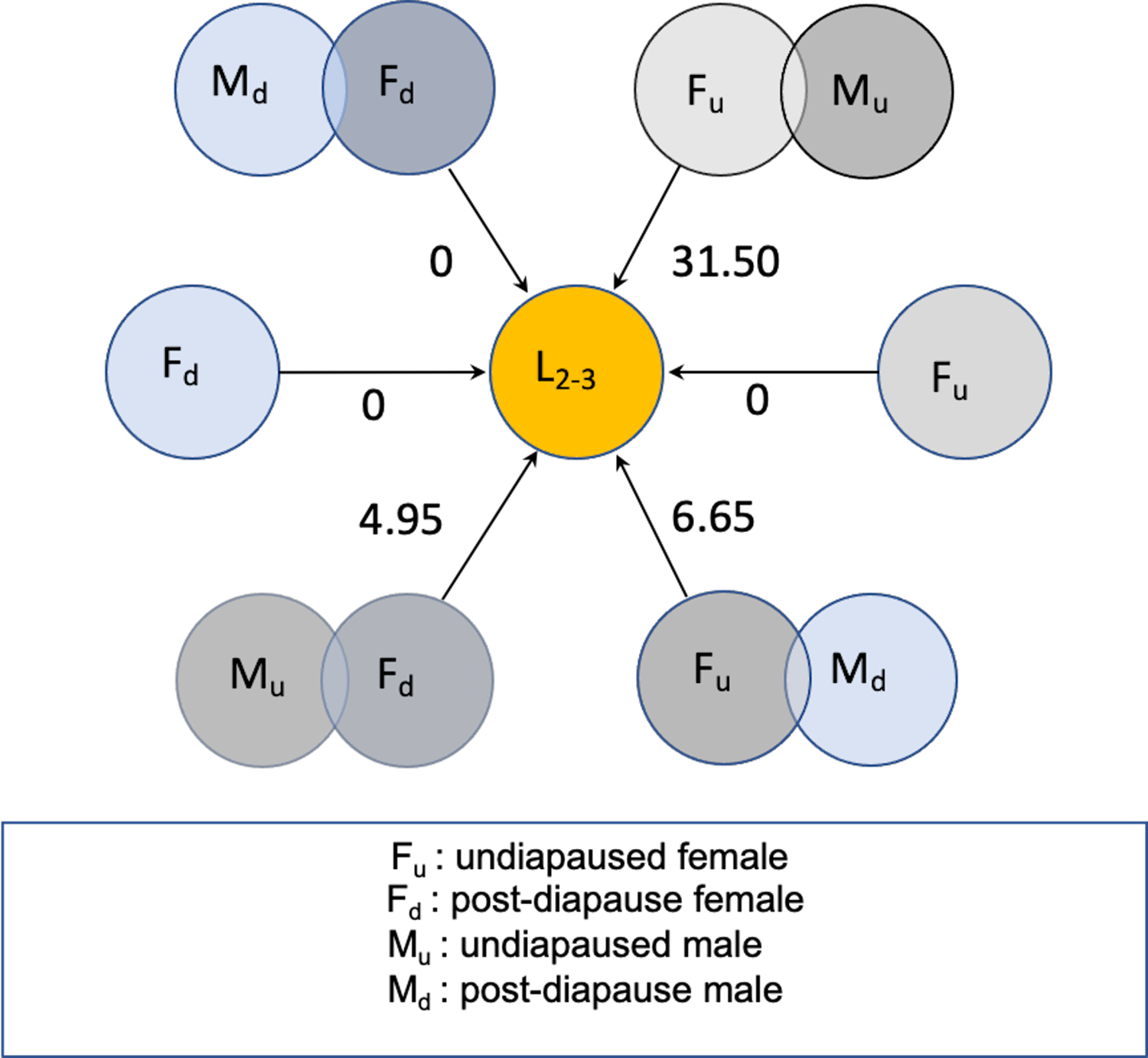
Fig. 5. Schematic representation of net reproductive rate (R 0) as number of daughter larvae (L 2–3), expected from postdiapause and nondiapause Drosophila suzukii in new season in cold-temperate conditions. Overlapping circles represent mating male and female flies according to diapause status. Unpaired circles represent unmated females, some of which might be fertile with sperm retained from previous mating.
Discussion
The mating behaviour and reproductive biology of D. suzukii in relation to winter diapause have been studied previously (e.g., Rossi-Stacconi et al. Reference Rossi-Stacconi, Kaur, Mazzoni, Ometto, Grassi and Gottardello2016; Grassi et al. Reference Grassi, Gottardello, Dalton, Tait, Rendon and Ioriatti2018; Stockon et al. Reference Stockon, Wallingford, Rendon, Fanning, Green and Diepenbrock2019), but more work is needed to evaluate the species’ potential establishment in cold-temperate regions such as Canada, where the adult survival of diapausing flies challenged by cold stress would need to be much longer. Based on our work in Québec (Cloutier et al. Reference Cloutier, Guay, Champagne-Cauchon and Fournier2021), D. suzukii flies from local populations can survive a 6-month cold winter despite substantial mortality, with female-biased survival as previously observed (e.g., Stockon et al. Reference Stockon, Wallingford, Rendon, Fanning, Green and Diepenbrock2019). The present study extends knowledge of the potential of D. suzukii to survive the harsh season and permanently establish in cold climates.
Cold tolerance and dormancy in D. suzukii have often been studied using laboratory strains (Stephens et al. Reference Stephens, Asplen, Hutchison and Venette2015; Ryan et al. Reference Ryan, Emiljanowicz, Wilkinson, Kornya and Newman2016; Shearer et al. Reference Shearer, West, Walton, Brown, Svetec and Chiu2016; Toxopeus et al. Reference Toxopeus, Jakobs, Ferguson, Gariepy and Sinclair2016; Wallingford and Loeb Reference Wallingford and Loeb2016). The D. suzukii individuals used in the present study were collected from Québec blueberry fields in late summer and fall and were possibly partially adapted to cold-climatic conditions. They had survived six months of cold stress near 0 °C in simulated winter, and their overall survival extended well into a realistic simulated springtime regime, allowing regeneration of reproductive function after diapause (Cloutier et al. Reference Cloutier, Guay, Champagne-Cauchon and Fournier2021). Finding mates in spring would be the most critical factor at the low fly densities that are typical in spring, due to the Allee principle (Jacobs Reference Jacobs2009), with decreasing available time and energy (Kramer et al. Reference Kramer, Dennis, Liehbold and Drake2009; Yamanaka and Liebhold Reference Yamanaka and Liebhold2009; Xu and Ma Reference Xu and Ma2020). This would apply mostly to male D. suzukii, for which the odds of winter mortality are nearly twice those of females (Cloutier et al. Reference Cloutier, Guay, Champagne-Cauchon and Fournier2021), and as indicated by the results of our 2017 experiment (Supplementary material 1).
Focusing on individuals and controlled mating, the 2018 experiment confirmed previous results, showing that field-collected and artificially overwintered postdiapause D. suzukii flies can reproduce in a realistic cold springtime regime many months after being captured in the field. However, except for a single female, postdiapause flies of both sexes were fertile only if experimentally mated with nondiapause partners from the laboratory colony (Table 3). Lifetime fertility of postdiapause females mated with nondiapause males (OFCM) was almost half that realised by postdiapause males via their nondiapause female mates. To summarise, our results showed that any contribution to population growth of postdiapause female and male flies would depend on the opportunity for mating with fertile partners, here provided as nondiapause flies from the colony. Ryan et al. (Reference Ryan, Emiljanowicz, Wilkinson, Kornya and Newman2016) suggested that mated D. suzukii might store sperm over winter to be used when conditions become favourable to laying eggs in the next growing season. We found evidence of sperm retention for at least six months in postdiapause D. suzukii females; however, this was observed in only one survivor tested (total N = 10; Table 1, OF treatment). Either most D. suzukii females were already in reproductive diapause when they emerged as adult flies in the field in late 2017 and were still virgin in spring 2018 or any sperm acquired from mating in the fall of 2017 was no longer available in spring 2018 to fertilise eggs matured in the spring.
Fitness costs of diapause
We refer here to fitness based on the adaptedness concept of Barker (Reference Barker, van der Werf, Graser, Frankham and Gondro2009, table 1) – that is, the degree to which postdiapause D. suzukii flies could contribute to the next generation, relative to the nondiapause adult phenotype of the species. Measured fertility losses imply substantial fitness costs of diapause (Sadakiyo and Ishihara Reference Sadakiyo and Ishihara2012b), inherent to D. suzukii’s persistence in cold-temperate regions. We found no evidence that postdiapause males could reproduce by mating with postdiapause females, which differs from our 2017 results (Supplementary material 1). A slightly different methodology (possible mate choice, multiple mating) might explain the few available postdiapause couples that were fertile in 2017.
In both sexes, fertility losses revealing fitness costs of diapause likely resulted from advanced ageing due to extensive survival under cold stress (Tatar and Yin Reference Tatar and Yin2001). Average chronological age during postwinter reproduction in spring 2018 was about seven to eight months older than the age of nondiapause flies used to scale fertility loss (Table 1, CFCM). Fitness trade-offs with prolonged survival are involved in genetically based clinal variation of reproductive diapause expression in D. melanogaster in the eastern United States of America (Schmidt et al. Reference Schmidt, Paaby and Shaneheschel2005). Schmidt and Conde (Reference Schmidt and Conde2006) reported that diapause genotypes of D. melanogaster had greater lifetime, lower age-specific mortality, and delayed reproduction in adult life when compared to nondiapause genotypes from the same latitudes. A similar trade-off between fitness and prolonged survival under cold stress could explain the reduced lifetime fertility of the longlived, fieldborn D. suzukii tested in the present study. What remains to be explained is why the negative effects of diapause on female fertility considerably exceed those on male fertility. A plausible explanation is that male fertility is mediated by their female mates (Margus and Lindström Reference Margus and Lindström2020): postdiapause D. suzukii’s male fertility was likely mitigated by the potentially high fertility of their nondiapause partners from the colony. It should also be clear that our estimates of fitness costs of diapause in D. suzukii are partial and neglect intergenerational effects on offspring quality (Hercus and Hoffmann Reference Hercus and Hoffmann2000; Priest et al. Reference Priest, Galloway and Roach2008).
Springtime population growth
Population growth-rate estimates of postdiapause D. suzukii in simulated spring conditions suggest that nondiapause immigrant flies of either sex could reproduce faster while mating among themselves rather than with any postdiapause heterosexual fly locally available. Analogous to r m (Henderson and Southwood Reference Henderson and Southwood2016), r c can measure initial growth potential after diapause in the absence of stage structure in winter survivor populations (Amarasake and Coutinho Reference Amarasake and Coutinho2013). Ability to recover from low densities after winter most likely depends on natality, assuming adult fly fertility and the presence of suitable fruits to lay eggs. Our r c estimate for nondiapause D. suzukii (circa 0.09 per day) is lower than those presented in other studies on summer-morph flies (circa 0.18, Emiljanowicz et al. Reference Emiljanowicz, Ryan, Langille and Newman2014, Ryan et al. Reference Ryan, Emiljanowicz, Wilkinson, Kornya and Newman2016; 0.22, Tochen et al. Reference Tochen, Dalton, Wiman, Hamm, Shearer and Walton2014), due mainly to the lower temperatures in our simulated springtime regime (Kindlmann et al. Reference Kindlmann, Dixon and Dostálková2001) and due in part to larval food quality (Tochen et al. Reference Tochen, Dalton, Wiman, Hamm, Shearer and Walton2014). Using our r c estimates to assess fitness costs of diapause indicates a reduction of 11% in postdiapause males, compared to a reduction of 18% in postdiapause females, which agrees with the above-described assessment based on lifetime offspring.
The three-parameter Gompertz population growth model showed how D. suzukii’s springtime population growth would be affected by a decreasing growth rate parameter (r), as females aged, according to the female’s diapause status or that of the mating partner. Ageing effects on fecundity varied strongly between nondiapause and postdiapause flies (Fig. 3). As expected, the age-specific fecundity pattern involving nondiapause mates (Fig. 3; CFCM) contributed most to spring population growth. However, this was because female fecundity was sustained over a much longer period of time not because of superior peak fecundity. Higher peak fecundity for nondiapause females mated to postdiapause males (CFOM) can be explained by constrained sperm availability from their postdiapause male mates whose age-specific fertility pattern was reduced.
Based on results, D. suzukii winter survivors would resume activity in mid to late April if the dynamics are driven mostly by physiological time (degree-days), regardless of other limitations. Wiman et al. (Reference Wiman, Dalton, Anfora, Biondi, Chiu and Daane2016) estimated that postdiapause D. suzukii requires 210 degree-days4 ˚C from 1 January to resume reproductive potential, but this ranges widely.
In 2017 (see Supplementary material 1), reproduction started at approximately 165 degree-days5 ˚C after cold winter ended among postdiapause females mated immediately upon transfer to simulated spring. In 2018 (see Supplementary material 2), oviposition started at 118 degree-days5 ˚C among postdiapause females (OFCM) and at 178 degree-days5 ˚C among nondiapause partners of postdiapause males (CFOM). The between-years difference could be due to field sampling (different years) and differing cold-winter regimes. Females in nondiapause control couples started laying eggs after only 35 degree-days5 ˚C, earlier than Wiman et al.’s (Reference Wiman, Dalton, Anfora, Biondi, Chiu and Daane2016) estimates. This can be explained by nondiapause females having already developed mature eggs in optimal rearing conditions when they were transitioned to the simulated springtime regime. Larval diet might also have played a role, as banana is superior to blueberry for rearing D. suzukii (Little et al. Reference Little, Chapman, Moreau and Hillier2017; Gonzalez-Cabrera et al. Reference Gonzalez-Cabrera, Garcia-Cancino, Moreno-Carrillo, Sanchez-Gonzalez and Arredondo-Bernal2018). In nature, to recover reproductive maturity, postdiapause flies would normally feed on noncrop protein sources, such as yeasts and other microorganisms in decaying fruits and vegetation (Hamby et al. Reference Hamby, Bellamy, Chiu, Lee, Walton and Wiman2016; Fountain et al. Reference Fountain, Bennett, Cobo-Medina, Conde Ruiz, Deakin and Delgado2018).
The heat requirements of postdiapause D. suzukii winter survivors suggest the flies experience phenological delays in their potential contribution to population growth come spring that potential nondiapause migrant flies colonising the region in early spring may not experience. This is especially true for postdiapause males, which seem unable to regenerate fertility as quickly as females do after diapause. Early reproduction is important in determining insect population growth (see, e.g., Price et al. Reference Price, Denno, Eubanks, Finke and Kaplan2011, Chapter 10). Heat-unit accumulation (physiological time) is far from being the sole determinant for insect population growth (Damos and Savopoulou-Soultani Reference Damos and Savopoulou-Soultani2012) but can be useful to project D. suzukii’s early season dynamics while considering mating opportunity and possible immigration. Indeed, springtime populations in a cold-temperate region would consist essentially of small numbers of adult flies that are in search of food, mates, and host fruits for female oviposition (Panel et al. Reference Panel, Zeeman, van der Sluis, van Elk, Pannebakker, Wertheim and Helsen2018).
Fruit-marking behaviour
Results from the present experiment include additional observations on the reproductive biology of D. suzukii. We did not attempt to determine the numbers of eggs laid, but we counted ovipositor marks left by females on fruit skins, which are signs associated with egg laying. We limited fruit exposure time to two days to help ensure that the fruit skins remained intact up until the time of counting marks and hatched larvae in dissected fruits (Walsh et al. Reference Walsh, Bolda, Goodhue, Dreves, Lee and Bruck2011). The unmated nondiapause virgin females that were used as controls left many ovipositor marks on fruits (CF treatment; Table 3), suggesting multiple attempts to lay eggs despite not being inseminated or courted by males. We do not know that all marks represented distinct egg-laying events because there was no evidence of larvae in the dissected fruits; however, we cannot discount that many infertile eggs may have been laid by virgins. The nondiapause virgin females were tested to examine possible facultative parthenogenesis. Tychoparthenogenesis characterises many Drosophila species, a lineage that hypothetically evolved it many times as an alternative reproductive strategy that allows host-niche and geographic range expansion (Chang et al. Reference Chang, Ting, Chang, Fang and Chang2014). We found no evidence that D. suzukii females deprived of mating can reproduce parthenogenetically. Despite that, their marking frequency equalled that of comparable mated females, suggesting that the virgin flies laid infertile eggs. Ryan et al. (Reference Ryan, Emiljanowicz, Wilkinson, Kornya and Newman2016) suggested that sperm-limited females can lay unfertilised eggs, possibly explaining the abundant marks left by nondiapause virgins in the present study. The fruit-marking frequency by females of control couples (CFCM) was similar but led to a high ratio of larvae to marks, thus confirming high oviposition success in blueberries (Atallah et al. Reference Atallah, Teixeira, Salazar, Zaragoza and Kopp2014). In contrast, postdiapause females deprived of mating (OF; N = 10) left no marks on fruits, indicating suppressed oviposition compared to nondiapause (CFCM) and postdiapause (OFCM, OFOM) mated females (Table 3). Mated postdiapause and nondiapause females marked fruits with equal frequency (Table 3). Fruit marking by mated postdiapause females and its absence by unmated ones indicate that marking was likely dependent on male contact – possibly mediated by male olfactory signals (Revadi et al. Reference Revadi, Lebreton, Witzgall, Anfora, Dekker and Becher2015) – as is also reported in D. montana in relation to reproductive diapause (Ala-Honkola et al. Reference Ala-Honkola, Kauranen, Tukmaeva, Boetzl, Hoikkala and Schmitt2018). A final point concerning mated postdiapause female fertility is the low ratio of hatched larvae to oviposition marks (Table 3), which suggests a reduced egg hatchability compared to eggs laid by nondiapause females. This is supported by the reduced offspring production (Table 1), hypothetically as a result of ageing during winter dormancy despite reduced physical and metabolic activity.
Conclusions
Knowledge of D. suzukii’s reproductive diapause is important to predict potential establishment in cold-temperate climates (reviewed in Nikolouli et al. Reference Nikolouli, Colinet, Renault, Enriquez, Mouto and Gibert2018). We showed that most D. suzukii that survived a six-month cold winter at temperatures close to 0 °C effectively reproduced after winter in simulated springtime conditions. We found negative effects of reproductive diapause on fitness in a sex-biased manner, despite both sexes surviving prolonged overwintering conditions. We also showed that cold stress is not the sole critical factor limiting postdiapause reproduction. Mating in spring with fertile heterosexual partners was critical for females as well as for males, as shown when we provided nondiapause fertile mates tested as potential migrants. Female winter survivors would only exceptionally be able to reproduce without copulating in spring, with little evidence that female flies active in the late prewinter season would continue to be fertile in the next spring as a result of prediapause mating. A population growth model with a variable growth rate parameter (r) determined mostly by ageing of first-generation flies in spring showed that early migrant flies would be strongly advantaged over locally overwintered postdiapause flies, with earlier reproductive maturity, a broader pattern of age-specific fecundity, and higher lifetime fecundity.
Local overwintering with high mortality, in combination with immigration from more favourable southern regions, could facilitate the spotted-wing drosophila’s local establishment in Québec and elsewhere in continental Canada.
Supplementary material
To view supplementary material for this article, please visit https://doi.org/10.4039/tce.2022.3.
Acknowledgements
The authors thank the Natural Sciences and Engineering Research Council of Canada Cooperative Research and Development programme and Discovery programme, the Syndicat des Producteurs de Bleuets du Québec, the Fonds de recherche du Québec-Nature et technologies, and the Fonds de la recherche agroalimentaire axé sur l’agriculture nordique du Saguenay–Lac-Saint-Jean for research grants to C.C. Special thanks are due to Dr. Valérie Fournier, Département de phytologie, Université Laval, Québec, Québec for useful comments on experimental work and to Gaétan Daigle, Service de consultation statistique, Département de mathématiques et statistiques, Université Laval. Finally, the authors are grateful to the blueberry producers from Saguenay–Lac-Saint-Jean who provided access to their lowbush blueberry fields.
Authors contributions
C.C. conceived the experiments and methodology with J.-F.G., and W.C.C. W.C.C., and J.-F.G. realised the experiments and recorded observations. C.C. statistically analysed the data, with help from J.-F.G. and a professional statistician. All authors contributed to the paper and authorised final publication.