Impact statement
This review investigates the potential formation of plastic-like residues from superabsorbent polymers (SAPs) in soil. SAPs are used extensively in various industries worldwide, and their market share is projected to grow significantly. Considering the global scale of plastic pollution, a better understanding of the fate and environmental impacts of this lesser-studied class of plastic pollutants is crucial for developing effective management strategies throughout the SAPs’ life cycle. Our findings provide first insights into the mechanisms and conditions that may promote solid SAP residue formation, offering solutions to mitigate their potential negative impacts on soil health and functioning. Understanding the presence of SAP residues in soil contributes to the broader field of plastic pollution research, raising awareness of an emerging class of plastic pollutants. Moreover, this research underscores the importance of responsible SAP usage and disposal, highlighting the need for stakeholder education and sustainable approaches for application, disposal and remediation. Overall, these findings have both local and global implications, promoting the development of environment-friendly practices and contributing to the long-term sustainability of ecosystems and society.
Introduction
Synthetic polymers are ubiquitous in our daily lives and the global economy. About 350 million tons are produced each year, with expectations of exceeding 900 million tons by 2050 (Borrelle et al., Reference Borrelle, Ringma, Law, Monnahan, Lebreton, McGivern, Murphy, Jambeck, Leonard, Hilleary, Eriksen, Possingham, De Frond, Gerber, Polidoro, Tahir, Bernard, Mallos, Barnes and Rochman2020). Besides the consumption of petrochemical and natural resources, these materials generate massive amounts of plastic waste. Currently, only 20% of plastic waste is recycled or incinerated, while 80% are assumed to end up in the environment, primarily in soil and water (Lebreton and Andrady, Reference Lebreton and Andrady2019; Kosior and Mitchell, Reference Kosior, Mitchell and Letcher2020).
In addition to microplastics (<5 mm) and nanoplastics (<1 μm), SAPs represent another largely overlooked class of potential plastic pollutants (Chen et al., Reference Chen, Wu, Raffa, Picchioni and Koning2022; Nawalage et al., Reference Nawalage, Kushara, Kushan and Bellanthudawa2022). SAPs are synthetic polymers that can absorb and retain large amounts of liquid by forming a highly interconnected three-dimensional network (Zohourian and Kabiri, Reference Zohourian and Kabiri2008). SAPs are used in various fields, including hygiene and biomedical products, agriculture, construction, wastewater treatment and remediation (Ma and Wen, Reference Ma and Wen2020; Saha et al., Reference Saha, Sekharan and Manna2020; Venkatachalam and Kaliappa, Reference Venkatachalam and Kaliappa2023). Most commercially used SAPs are based on polyacrylates (PAA), polymethacrylates (PMA) and polyacrylamides (PAM). The global annual production of sodium polyacrylate, used in disposable diapers, already exceeds 2 million tons. With a rising global population and the expanding application of SAPs, their revenue is projected to grow from 9 billion dollars in 2019 to 13 billion dollars by 2024 (Ullah et al., Reference Ullah, Othman, Javed, Ahmad and Akil2015; Chazovachii et al., Reference Chazovachii, Somers, Robo, Collias, James, Marsh, Zimmerman, Alfaro and McNeil2021; Rizwan et al., Reference Rizwan, Gilani, Durani and Naseem2021; Chen et al., Reference Chen, Wu, Raffa, Picchioni and Koning2022).
Upon entering the soil, SAPs have been shown to gradually lose their intended properties despite their designated durability (Buchmann et al., Reference Buchmann, Bentz and Schaumann2015; Buchmann and Schaumann, Reference Buchmann and Schaumann2017; Almeida and Klemm, Reference Almeida and Klemm2018; Jung et al., Reference Jung, Endres and Weichold2020; Saha et al., Reference Saha, Rattan, Sekharan and Manna2021). Prolonged weathering and elevated residence times may trigger the formation of (micro)plastic-like solid SAP residues, as indicated by Bai et al. (Reference Bai, Song and Zhang2013), Dehkordi (Reference Dehkordi2016) and Kumar et al. (Reference Kumar, Kumar and Vennela2018). However, evidence on the formation of solid SAP residues in soil has been merely anecdotal until now. Comprehensive fate studies elucidating the physicochemical processes and environmental conditions promoting such a formation are missing. This might be attributed to the relatively limited attention given to SAPs in soil within (micro)plastics and polymer research (Cutié et al., Reference Cutié, Buzanowski and Berdasco1990; Nagaraj and Rajan, Reference Nagaraj, Rajan, AlMaadeed, Ponnamma and Carignano2020; Thomas et al., Reference Thomas, Schütze, Heinze and Steinmetz2020; Chen et al., Reference Chen, Wu, Raffa, Picchioni and Koning2022).
This review examines the potential formation of solid SAP residues in soil and its relevance for plastic pollution research and soil science. We first provide a brief overview of the historical development of SAPs, their application areas (see Section “History and areas of application”) and SAP classification (see Section “Classification and properties of SAPs”). Subsequently, we discuss the conditions under which soil SAP residues may form (see Section “SAPs as new source of microplastics in soil”) and describe analytical methods for their identification and characterization (see Section “Analytical techniques for investigating SAPs and the formation of solid SAP residues in soil”).
History and areas of application
In the 1940s, the first water-absorbent polymer was synthesized, using divinylbenzene and acrylic acid (Buchholz and Graham, Reference Buchholz and Graham1998). Since then, continuous research and development have propelled the widespread adoption of SAPs in everyday life, medical standards and industrial processes (see Chen et al., Reference Chen, Wu, Raffa, Picchioni and Koning2022, for a comprehensive overview on the history of SAPs).
Due to their hydrophilic properties, selective permeability and mechanical, chemical and biological stability, SAPs are used in personal care products, cosmetics and biomedical applications (Wang and Wang, Reference Wang, Wang, Kirk and Othmer2000; Kulawik-Pióro and Miastkowska, Reference Kulawik-Pióro and Miastkowska2021). They are contained in diapers, sanitary napkins and panty liners for their absorbing properties, while further acting as preservatives and moisturizers in cosmetics (Venkatachalam and Kaliappa, Reference Venkatachalam and Kaliappa2023; Chen et al., Reference Chen, Wu, Raffa, Picchioni and Koning2022). In biomedical applications, SAPs are applied in cell cultures and drug delivery (Bordbar-Khiabani and Gasik, Reference Bordbar-Khiabani and Gasik2022; Ho et al., Reference Ho, Chang, Chan, Chung, Shu, Chuang, Duh, Yang and Tyan2022). The amount of SAP used varies greatly depending on the intended use and product design: for instance, 2–7 g of SAPs is added per diaper, whereas less than 1 mg of SAP is added per gram of skin cream (Medina-Torres et al., Reference Medina-Torres, Calderas, Sanchez-Olivares and Nuñez-Ramirez2014; Kulawik-Pióro et al., Reference Kulawik-Pióro, Kurpiewska and Kułaszka2017; Bachra et al., Reference Bachra, Grouli, Damiri, Bennamara and Berrada2020).
Moreover, SAPs have been used as soil amendments since the 1950s due to their ability to improve soil properties and crop productivity (Bai et al., Reference Bai, Song and Zhang2013; Xi and Zhang, Reference Xi and Zhang2021; Zheng et al., Reference Zheng, Mei, Wang, Yin, Li, Zheng, Ou and Cui2023). PAA and PAM, for instance, can be applied to modulate the uptake and release of water, nutrients, fertilizers or pesticides and to improve soil aggregation and structural stability (Venkatachalam and Kaliappa, Reference Venkatachalam and Kaliappa2023; Liu et al., Reference Liu, Zhu, Mu, Wang, Quan and Wang2022). Typical soil application rates range from 10 to 225 kg ha−1, depending on the polymer type, environmental conditions, application depths and soil properties (Rostampour et al., Reference Rostampour, Yarnia, Khoee, Seghatoleslami and Moosavi2013; Grabiński and Wyzińska, Reference Grabiński and Wyzińska2018). In this context, there is currently an absence of dedicated regulations or policies that provide guidance on their safe and appropriate usage in soil management.
SAPs are further used for soil remediation and wastewater treatment due to their metal-chelating properties. For wastewater purification, modified PAM hydrogels are applied (Kaşgöz et al., Reference Kaşgöz, Özgümüş and Orbay2005; Lebkiri et al., Reference Lebkiri, Abbou, Hsissou, Safi, Sadiku, Berisha, Amri, Essaadaoui, Kadiri, Lebkiri and Rifi2023), while natural and synthetic SAPs have been used for soil remediation to stabilize toxic metals and reduce their bioavailability (Yang et al., Reference Yang, Cen, Feng, Liu, Qu and Miao2020; Toan et al., Reference Toan, Hue, Dung, Tung, Duc, Van Khoi, Van Thanh and Linh2023; Zhang et al., Reference Zhang, He, Gao, Mady, Filipović, Dyck, Lv and Liu2023).
In the construction industry, SAPs improve the properties of concrete, including their self-sealing and curing, water control and durability (Lokeshwari et al., Reference Lokeshwari, Pavan Bandakli, Tarun, Sachin and Kumar2021; Schröfl et al., Reference Schröfl, Erk, Siriwatwechakul, Wyrzykowski and Snoeck2022). They also enhance the workability of concrete and increase its resistance to shrinkage and cracking through freeze–thaw cycles (Liu et al., Reference Liu, Khayat and Shi2020; Chahar and Pal, Reference Chahar and Pal2022; Xu et al., Reference Xu, Harvey, Begiristain, Domínguez, Sánchez-Abella, Browne and Cook2022).
Classification and properties of SAPs
Depending on synthesis methods and the resulting material properties, various classification schemes are common for SAPs (Figure 1). For a detailed overview, we refer to the review by Chen et al. (Reference Chen, Wu, Raffa, Picchioni and Koning2022).
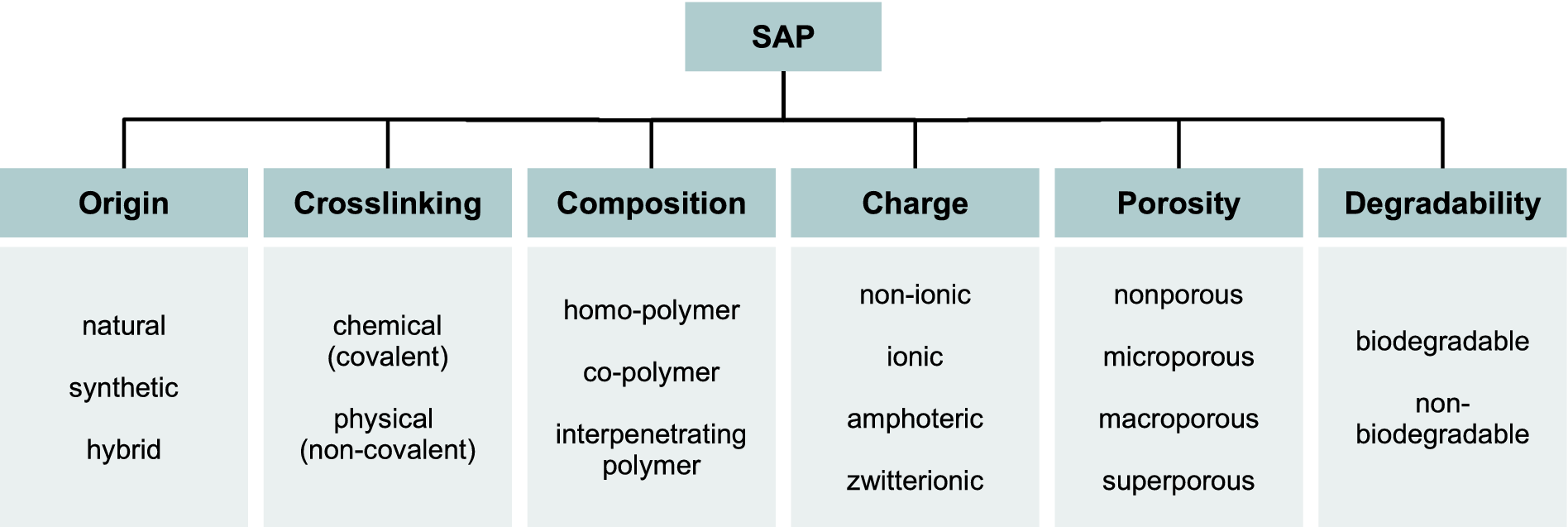
Figure 1. SAP classification based on different properties (Ahmed, Reference Ahmed2015; Saha et al., Reference Saha, Sekharan and Manna2020; Venkatachalam and Kaliappa, Reference Venkatachalam and Kaliappa2023).
Based on their origin, SAPs can be categorized into natural, synthetic and hybrid polymers. Synthetic SAPs are produced from monomers like acrylic acid, acrylamide and vinyl alcohol, while natural SAPs originate from biological sources like plant excretions, earthworm mucus or microorganisms. Hybrid SAPs are copolymers of natural and synthetic SAPs. Cross-linking, which connects polymer chains through ionic or covalent bonds, plays an important role in SAP formation: physical cross-linking is reversible, while chemical cross-linking is permanent and more resistant to environmental dynamics and degradation (Xu et al., Reference Xu, Liu, Ren and Gao2018). Consequently, the SAP network not only exhibits insolubility in water and transforms into a gel when it absorbs water, but it also has the capacity to retain water and solutes based on its cross-linking density (Ahmed, Reference Ahmed2015). Depending on the functional groups and their charge within the polymer network, SAPs can be non-ionic, ionic, amphoteric and zwitterionic (Grabowska-Polanowska et al., Reference Grabowska-Polanowska, Garbowski, Bar-Michalczyk and Kowalczyk2021). SAPs are further distinguished by their monomeric composition and porosity. The latter is controlled by the reaction conditions, polymerization aids like foaming agents and cross-linking agents such as polyvalent cations (Coelho et al., Reference Coelho, Ferreira, Alves, Cordeiro, Fonseca, Góis and Gil2010; Saha et al., Reference Saha, Sekharan and Manna2020). Degradability is another property for characterizing SAPs, with nonbiodegradable, often synthetic SAPs persisting in soil for extended periods. Recent research focuses on developing copolymers with enhanced water absorption capacity and higher biodegradability (Durpekova et al., Reference Durpekova, Di Martino, Dusankova, Drohsler and Sedlarik2021; Russo et al., Reference Russo, Fucile, Giacometti and Sannino2021). However, the lack of a standardized method for measuring the rate and extent of biodegradation of both biobased and synthetic hydrogels presents a challenge in prescribing the ideal hydrogel as well as its application approach (Adjuik et al., Reference Adjuik, Nokes and Montross2023).
So far, SAP hydrogels have been mostly characterized for their application performance as reviewed by Mahinroosta et al. (Reference Mahinroosta, Farsangi, Allahverdi and Shakoori2018) and Bashir et al. (Reference Bashir, Hina, Javed Iqbal, Mujtaba, Alghamdi, Wageh, Ramesh and Ramesh2020). The basic properties considered include swelling degree, swelling ratio, gel strength, network porosity and (bio)degradability. The network porosity influences the transport of water, solutes and cellular components through the three-dimensional polymer network, whereas the swelling degree and swelling ratio are important for the water absorption capacity of SAP hydrogels (Kazanskii and Dubrovskii, Reference Kazanskii and Dubrovskii1992). The type and degree of cross-linking influence various properties of SAP hydrogels, especially the swelling ratio and mechanical stability, as increased cross-linking improves the mechanical stability but reduces the swelling capacity and vice versa (Gupta and Shivakumar, Reference Gupta and Shivakumar2012; Kadhim et al., Reference Kadhim, Hameed and Rasheed2022). However, overall SAP properties in soil depend not only on the chemical composition of the SAP and the respective synthesis method but also on the given environmental conditions, that is, the soil pH, soil temperature and ionic strength of the soil solution. The impact of basic SAP properties on their environmental fate is far less researched and further explored in the following section.
SAPs as new source of microplastics in soil
Entry, pathways and fate of SAPs in soil
SAPs enter the soil intendedly or unintendedly. Unintended entry occurs through accidental spillage, improper disposal of SAP-containing products and surface runoff (Hamilton et al., Reference Hamilton, Reinert, Hagan and Lord1995; Jesca and Junior, Reference Jesca and Junior2015). SAPs used in personal care products like shampoo, sunscreen or toothpaste may enter aquatic systems through insufficient wastewater treatment or by bathing in natural waters (Rozman and Kalčíková, Reference Rozman and Kalčíková2021).
By contrast, SAPs are intentionally mixed into the soil for agricultural purposes, erosion control and construction (Venkatachalam and Kaliappa, Reference Venkatachalam and Kaliappa2023; Almajed et al., Reference Almajed, Lemboye and Moghal2022). Various application methods are used, including (1) tilling SAPs into the topsoil (0–20 cm), (2) spraying SAP powder onto foliage or surface soil, (3) applying SAPs to the seed burial or root zone and (4) coating or soaking seeds into SAP hydrogels before planting (Li et al., Reference Li, He, Hughes, Liu and Zheng2014; Oladosu et al., Reference Oladosu, Rafii, Arolu, Chukwu, Salisu, Fagbohun, Muftaudeen, Swaray and Haliru2022; Zheng et al., Reference Zheng, Mei, Wang, Yin, Li, Zheng, Ou and Cui2023). The rate, frequency and methods of SAP application are not standardized and can result in varying quantities and distribution patterns of SAPs in soil.
Once entered the soil, various environmental dynamics may affect the properties and fate of SAPs. Several studies have shown a direct link between SAP swellability and effects on soil properties as an overall function of moisture dynamics, agricultural management practices, soil texture, soil solution composition and polymer–mineral interactions (Buchmann et al., Reference Buchmann, Bentz and Schaumann2015; Chang et al., Reference Chang, Im, Prasidhi and Cho2015; Ayeldeen et al., Reference Ayeldeen, Negm and Sawwaf2016; Brax et al., Reference Brax, Buchmann and Schaumann2017). Other scholars highlighted a lack of scientific knowledge of the fate of SAPs in soil, particularly in the long term (Sojka et al., Reference Sojka, Bjorneberg, Entry, Lentz and Orts2007; Nyyssölä and Ahlgren, Reference Nyyssölä and Ahlgren2019; Ostrand et al., Reference Ostrand, De Sutter, Aaron, Limb and Steele2020; Adjuik et al., Reference Adjuik, Nokes and Montross2023).
Although microorganisms are known to break down SAPs in soil, biodegradation rates vary between 0.2–0.5% y−1 for PAA and 4.5–9.8% y−1 for PAM. The biodegradation of other SAPs like polydiallyldimethylammonium chloride (pDADMAC) has not been investigated yet (Entry et al., Reference Entry, Sojka and Hicks2008; Wilske et al., Reference Wilske, Bai, Lindenstruth, Bach, Rezaie, Frede and Breuer2013). Various factors influence the rate and extent of SAP biodegradation, including SAP composition and application depth, as well as soil temperature and soil moisture (Smagin et al., Reference Smagin, Sadovnikova and Smagina2014). The carbon backbone of SAPs like PAA is particularly recalcitrant against microbial activity (Nyyssölä and Ahlgren, Reference Nyyssölä and Ahlgren2019). Longer polymer chains and higher hydrophobicity may further hinder biodegradation (Siracusa, Reference Siracusa2019). Low oxygen and moisture levels, high salinity, low temperatures and low nutrient availability additionally reduce SAP biodegradation (Gu, Reference Gu2003; Adjuik et al., Reference Adjuik, Nokes and Montross2023). Current literature reviews by Nyyssölä and Ahlgren (Reference Nyyssölä and Ahlgren2019) and Adjuik et al. (Reference Adjuik, Nokes and Montross2023) thus conclude that biodegradation of various SAPs is a rather slow process.
Besides biodegradation, chemical, photolytic and mechanical processes are being discussed to fragment SAP macromolecules into smaller units (Sojka et al., Reference Sojka, Bjorneberg, Entry, Lentz and Orts2007). Fragmentation may increase SAP mobility and make them more susceptible to translocation into deeper soil or via surface runoff, exposing them to friction and abrasion, and thereby promoting further fragmentation. Soil texture and soil organic matter (SOM) may significantly influence SAP mobility in terms of decreasing SAP mobility through adsorption, complexation and precipitation (Theng, Reference Theng2012), but this needs to be corroborated by (semi)field studies.
Irrespective of potential degradation or aging processes, SAPs employed in (semi)arid climates to enhance the soil water-holding capacity were found to lose their efficacy within 3 months after application (Banedjschafie and Durner, Reference Banedjschafie and Durner2015). Consequently, repeated applications at short intervals are necessary to sustain their intended effect. Given effective concentrations of up to 10 g kg−1 soil (Banedjschafie and Durner, Reference Banedjschafie and Durner2015), SAP accumulation in soil is likely (Hennecke et al., Reference Hennecke, Bauer, Herrchen, Wischerhoff and Gores2018; Nyyssölä and Ahlgren, Reference Nyyssölä and Ahlgren2019; Adjuik et al., Reference Adjuik, Nokes and Montross2023). Moreover, recommended application rates do not always yield the desired effect as indicated by Volkmar and Chang (Reference Volkmar and Chang1995) who reported instances of farmers exceeding manufacturer recommendations by a factor of 4. In this regard, the anticipated effects of climate change, such as increased frequency and severity of droughts, may incite even higher application rates and shorter application intervals in affected regions (Saha et al., Reference Saha, Sekharan and Manna2020). Therefore, the potential for successive SAP accumulation in soil increases significantly.
Given the ongoing application of SAPs and their continued entry into the environment, particularly in soil, it is essential for future research to investigate the long-term fate of hardly biodegradable SAPs. These SAPs are likely to persist in soil and appear challenging to remove, often necessitating labor-intensive and costly remediation methods (Puoci et al., Reference Puoci, Iemma, Spizzirri, Cirillo, Curcio and Picci2008). As our current understanding of SAP fragmentation in soil is limited and often based on theoretical considerations (Adjuik et al., Reference Adjuik, Nokes and Montross2023), it is crucial to investigate the specific environmental conditions and processes that drive SAP fate, the potential formation of solid SAP residues and implications on ecosystem functioning.
Main processes contributing to the potential formation of solid SAP residues in soil
Various physicochemical parameters may influence SAP transformation and solid SAP residue formation in soil. Chemical factors include soil pH and ionic strength. Physical factors include changes in soil temperature, soil moisture dynamics, soil texture and agricultural influences like tillage and soil compaction (Figure 2). The limited biodegradability of SAPs enables them to persist in soil for an extended period, providing enough time for potentially relevant processes to act and promote the formation of solid SAP residues.

Figure 2. Factors and processes contributing to the potential formation of solid SAP residues in soil; red dots indicate cross-links within the SAP network.
Chemical processes, such as cross-linking between SAP chains and soil constituents like clay minerals, SOM and polyvalent cations, can create strong and hardly detachable clay mineral–polymer complexes, depending on the pH and electrostatic interactions (Buchmann and Schaumann, Reference Buchmann and Schaumann2017; Buchmann et al., Reference Buchmann, Bentz and Schaumann2015; B. K. G. Theng, Reference Theng1982). Cross-linking with polyvalent cations like Ca2+ or Mg2+ results in insoluble and dense polymer networks (Adjuik et al., Reference Adjuik, Nokes and Montross2023). For example, a study conducted by Banedjschafie and Durner (Reference Banedjschafie and Durner2015) showed that Ca2+ strongly reduced SAP hydrogel swelling compared with Mg2+. SAP hydrogels can also bind to soil particles, enhancing SAP sequestration and thus its persistence in soil (Buchmann and Schaumann, Reference Buchmann and Schaumann2017; Zareie et al., Reference Zareie, Bahramian, Sefti and Salehi2019). Although there are no studies available on the pH dependency of solid SAP residue formation to date, pH changes will most likely influence the SAPs’ macromolecular structure. Polymer research has shown that a low pH decreases the sorption capacity of SAPs like PAA (Bakass et al., Reference Bakass, Mokhlisse and Lallemant2001; Elliott et al., Reference Elliott, Macdonald, Nie and Bowman2004). Under natural environmental conditions, the hydrophilic moieties of the SAP are typically deprotonated, which induces repulsion between the polymer chains and enables swelling (Figure 2). Upon increasing protonation, the hydrogel shrinks (Rather et al., Reference Rather, Bhat and Shalla2022). The pH may further modulate the sorption of other surface-active substances like natural (nano)particles or organic matter to SAPs, affecting soil wettability, diffusivity and mechanical stability (Alvarez-Lorenzo and Concheiro, Reference Alvarez-Lorenzo and Concheiro2003; Zhao et al., Reference Zhao, Cheng, Ji, Kang and Chen2009; Li et al., Reference Li, Jia, Sun, Xu, Burcu Baykal Minsky, Cölfen, von Klitzing and Guo2018; Degen et al., Reference Degen, Paulus, Zwar, Jakobi, Dogan, Tolan and Rehage2019). For instance, Li et al. (Reference Li, He, Liu and Zheng2013) suggested that SAPs contribute to the accumulation of stable SOM fractions in soil through SOM–SAP interactions. However, no studies are available on SOM–SAP interactions and their potential role in solid SAP residue formation. This is probably because typical SAP applications tend to take place in regions with poor water supplies and in soils with low SOM content (Zheng et al., Reference Zheng, Mei, Wang, Yin, Li, Zheng, Ou and Cui2023). Therefore, it remains unclear and subject to future research to elucidate how and to what extent SOM–SAP interactions influence the effect of SAPs on water and nutrient retention, their degradation and spatiotemporal fate.
Physical factors like temperature and moisture dynamics, for instance, during freezing–thawing and drying–rewetting, as well as tillage and soil compaction probably, contribute to the formation of solid SAP residues in soil. Drying–rewetting cycles could promote solid SAP residue formation as the SAP network collapses during dehydration, forming condensed structures (Ostrand et al., Reference Ostrand, De Sutter, Aaron, Limb and Steele2020). Temperatures close to or above the lower critical solution temperature can alter the hydrophilic and hydrophobic states of the polymer network, reducing the overall water uptake and swellability (Xu et al., Reference Xu, Kang and Neoh2006; Andry et al., Reference Andry, Yamamoto, Irie, Moritani, Inoue and Fujiyama2009). Climate change increases the likelihood of high temperatures and prolonged dry periods, which may trigger the formation of condensed, less rewettable structures or solid SAP residues that are both resistant to biodegradation in the short term and long term. Freeze–thaw cycles may further trigger solid SAP residue formation in soil through network rupturing during ice formation and subsequent structural changes, for instance, by additional cross-linking or increased stiffness and crystallinity of the hydrogel network (Ma and Wen, Reference Ma and Wen2020). Soil compaction can cause SAP hydrogels to form less swellable condensed structures that are harder for microorganisms to access and degrade, while soil texture can constrain SAP hydrogel swelling due to water competition and mutual swelling restriction with clay particles or SOM (Buchmann and Schaumann, Reference Buchmann and Schaumann2017; Lejcuś et al., Reference Lejcuś, Śpitalniak and Dąbrowska2018). The impact of agricultural practices like tillage on SAPs and solid SAP residue formation in soil is unstudied (Malik et al., Reference Malik, Chaudhary, Malik, Punia, Sewhag, Berkesia, Nagora, Kalia, Malik, Kumar, Kumar, Kamboj, Ahlawat, Kumar and Boora2022).
Since only a few targeted studies on the transformation potential of SAPs have been published to date, the actual relevance of the subsequently discussed mechanisms, processes and factors can be neither clarified nor quantified. In addition, the quantity, frequency and methods of SAP application are likely to affect the strength and likelihood of processes resulting in the formation of solid SAP residues. This is because the application depth, the method of mixing and the rate of application will influence the contact time and distribution and consequently the degree and duration of interaction with soil constituents and environmental dynamics. However, it remains unclear to what extent the discussed processes and conditions in terms of the direction, strength and duration of their interaction could favor the potential formation of solid SAP residues in soil.
Implications on soil functioning and environmental friendliness
Solid SAP residues in soil are assumed to influence soil functioning and thus its provision of ecosystem services. Like other (micro)plastics, solid SAP residues potentially redistribute and accumulate in soil and consequently alter physicochemical soil properties such as porosity, water infiltration and aeration (F. Wang et al., Reference Wang, Wang, Adams, Sun and Zhang2022; Zheng et al., Reference Zheng, Mei, Wang, Yin, Li, Zheng, Ou and Cui2023). Excessive amounts of plastic residues in soil (>240 kg ha−1) were shown to reduce agricultural yields because of reduced nutrient availability and microbial diversity, deteriorated soil structure and hampered root development (Gao et al., Reference Gao, Yan, Liu, Ding, Chen and Li2019). Similarly, solid SAP residues might affect agricultural productivity due to reduced water infiltration and poor aeration, especially in regions with limited water availability. Reduced soil porosity and water infiltration are well-known to cause erosion, exacerbating soil losses and degradation (Ferreira et al., Reference Ferreira, Samaneh Seifollahi-Aghmiuni, Destouni, Ghajarnia and Kalantari2022). Furthermore, it remains unclear whether the potential sorption of nutrients by solid SAP residues could limit nutrient availability for plants and soil microorganisms and thereby reduce soil fertility and productivity (Han et al., Reference Han, Yu, Yang, Li, Xu and Wang2012; Banedjschafie and Durner, Reference Banedjschafie and Durner2015; Buchmann et al., Reference Buchmann, Bentz and Schaumann2015; Nyyssölä and Ahlgren, Reference Nyyssölä and Ahlgren2019; Ji et al., Reference Ji, Zhao, Wu, Han, J-q and Bai2022). Similar to microplastics, solid SAP residues could sorb toxic substances and undergo transport through the soil column by bioturbation and agricultural practices (Zhu et al., Reference Zhu, Zhu, Wang and Gu2019; Xu et al., Reference Xu, Liu, Cryder, Huang, Lu, He, Wang, Lu, Brookes, Tang, Gan and Xu2019).
Regarding the effects of SAPs on the fate of agrochemicals and other soil contaminants, current studies indicate a SAP-induced reduction in contaminant mobility and toxicity. The sorption behavior of SAP hydrogels is generally well-researched for metals and dyes (Hüttermann et al., Reference Hüttermann, Lawrence and Agaba2009; Bhattacharya and Shunmugam, Reference Bhattacharya and Shunmugam2020; Venkatachalam and Kaliappa, Reference Venkatachalam and Kaliappa2023). For instance, Dhiman et al. (Reference Dhiman, Prasher, ElSayed, Patel, Nzediegwu and Mawof2020) observed a higher retention and reduced plant uptake of Cd, Cu and Zn in soil after PAM amendments. Yang et al. (Reference Yang, Wang, Huang, Zhang, He, Gao and Ye2017) and Y. Yang et al. (Reference Yang, Zhang, Yang, Bai, Tang, Ye and Wang2018) found exposure concentrations of the fungicide carbendazim in soil reduced by sorption to PAM hydrogels. However, structural and functional changes in SAPs, for instance, during their transformation to solid SAP residues, could remobilize agrochemicals or pollutants.
The formation and the spatiotemporal effects of solid SAP residue accumulation on soil biota remain largely unclear as no literature is currently available. Assuming that solid SAP residues behave like conventional (micro)plastics, they might be taken up by soil organisms or sediment-dwelling organisms such as annelids, potentially interfering with nutrients and other substances during gut passage (Khoshmanesh et al., Reference Khoshmanesh, Sanati and Ramavandi2023). Consequently, solid SAP residues could be a potential hotspot and vector for toxic substances and facilitate toxic substances entering the human food chain via groundwater or plant uptake. The current knowledge of the potential ecotoxicological side effects of SAPs and solid SAP residues in soil is fairly limited, indicating major gaps in our scientific understanding of their environmental risks (Saha et al., Reference Saha, Sekharan and Manna2020). As of yet, the available literature on SAPs points toward no or only limited effects in aquatic and terrestrial ecosystems (Sousa et al., Reference Sousa, Lima, Neris, Silva, Nascimento, Araújo, Ratke, Silva, Osajima, Bezerra and Silva-Filho2021; Wang et al., Reference Wang, Wang, Zhao and Wang2018; Basanta et al., Reference Basanta, Díaz-Raviña, González-Prieto and Carballas2002), whereas no information is available for solid SAP residues.
All in all, the effects of SAPs and solid SAP residues on soil physicochemical properties, functions and the availability and fate of water, nutrients and agrochemicals are still incompletely understood. It is essential to consider the potential unintended consequences of SAP usage, particularly in the long term and after the formation of solid SAP residues with properties distinct from the SAP hydrogel. Alternative sustainable solutions, such as biodegradable soil amendments, should be explored to support crop productivity and soil health. However, little research has compared the biodegradability of synthetic and biobased or biodegradable SAPs in agricultural soils (Adjuik et al., Reference Adjuik, Nokes and Montross2023).
Analytical techniques for investigating SAPs and the formation of solid SAP residues in soil
Current challenges and limitations
SAP hydrogels and solid SAP residues are amenable to a wide range of analytical techniques including mechanical, spectroscopic and thermoanalytical approaches (Brax et al., Reference Brax, Buchmann and Schaumann2017; Huang et al., Reference Huang, Hu and Wang2022; Tian et al., Reference Tian, Cheng, Zhen and Lei2022; Rosso et al., Reference Rosso, Gregoris, Litti, Zorzi, Fiorini, Bravo, Barbante, Gambaro and Corami2023). In soil, these methods may be used either for examining changes in the entire soil system, which is bulk analysis, or for the targeted qualitative and quantitative analysis of SAPs and their potential transformation to solid residues (Figure 3). However, each technique involves certain challenges and limitations, such as difficulties in the detection and quantification of trace concentrations, lack of specificity, time-consuming sample preparation or insufficient spatiotemporal resolution. Moreover, standardization is missing for most methods (Fojt et al., Reference Fojt, David, Přikryl, Řezáčová and Kučerík2020; Jin et al., Reference Jin, Liu, Yu, Zhou, Wu, Fu, Yin, Fernandez and Karimi-Maleh2022).

Figure 3. Analytical techniques for the investigation of various SAP properties in soil.
Bulk analysis of SAPs and solid SAP residues in soil
Rheometry analyzes the mechanical properties of materials like hydrogels and soils by applying controlled deformation or stress and measuring the response. It can detect hydrogel properties, such as the elastic and viscous modulus, yield stress, strain–stress relationship and time-dependent mechanical properties. Soil rheology is commonly measured with oscillatory tests to assess microstructural stability, particle–particle interactions and viscoelasticity (Brax et al., Reference Brax, Buchmann and Schaumann2017; Holthusen et al., Reference Holthusen, Batistão and Reichert2020). Rheometry primarily provides mechanistic and process-level information because it measures the entire soil–SAP system. Despite the recent use of rheometry to study the mutual effects of SAPs, polymer–clay interactions and environmental factors on soil microstructural stability (Buchmann et al., Reference Buchmann, Bentz and Schaumann2015; Zareie et al., Reference Zareie, Bahramian, Sefti and Salehi2019; Knott et al., Reference Knott, Ani, Kroener and Diehl2022), the effect of solid SAP residue formation is still unclear and can only be assumed to be more particle-like than glue-like (Wen et al., Reference Wen, Li, Huang, Armwood, Amini and Li2019; Buchmann et al., Reference Buchmann, Steinmetz, Brax, Peth and Schaumann2020; Cui et al., Reference Cui, Wang, Zehnder and Hui2021). Thus, further research is needed to identify suitable indicators for determining the formation of solid SAP residues in soil.
While rheological measurements provide information about the mechanical behavior of samples, nuclear magnetic resonance (NMR) techniques, namely 13C- and solid-state NMR spectroscopy, diffusion-ordered spectroscopy and 1H-NMR relaxometry, provide information on the chemical composition, molecular structure, dynamics, solvent interactions and conformational changes of samples (Vandersypen and Chuang, Reference Vandersypen and Chuang2005; Brax et al., Reference Brax, Buchmann and Schaumann2017; El Nokab et al., Reference El Nokab, El, Lasorsa, Sebakhy, Picchioni and van der Wel2022; Plante et al., Reference Plante, Sanderman, Asanopoulos, Bell and Baldock2023). 1H-NMR relaxometry uses nuclear spin relaxation times, two-dimensional correlation spectra and water diffusivity to measure structural features and dynamics between sample structures and water molecules in situ (Demco and Pich, Reference Demco and Pich2023). It is commonly used for hydrogels and soil to assess polymer–water interactions and water molecule mobility and binding (Romero-Zeron et al., Reference Romero-Zeron, Manalo and Kantzas2004; Buchmann and Schaumann, Reference Buchmann and Schaumann2017; Brax et al., Reference Brax, Schaumann and Diehl2018; Arens et al., Reference Arens, Barther, Landsgesell, Holm and Wilhelm2019; Buchmann et al., Reference Buchmann, Steinmetz, Brax, Peth and Schaumann2020). However, research on the impact of SAP hydrogels on soil using 1H-NMR relaxometry is limited. No studies have been conducted on solid SAP residues so far. Despite challenges in differentiating water populations or hydrogel phases in soil, 1H-NMR relaxometry, especially two-dimensional or pulsed-field gradient 1H-NMR relaxometry (Brax et al., Reference Brax, Buchmann and Schaumann2017), could provide valuable insights into the impact of solid SAP residues on soil–water interactions and their interactions with various soil components, such as clay particles and SOM.
In addition to water dynamics and mechanical properties measured by 1H-NMR relaxometry and rheometry, respectively, differential scanning calorimetry (DSC) assesses the heat flow during thermal transitions, providing information on thermal stability and thermodynamic parameters like enthalpy and entropy. This helps to understand the physical and chemical properties of materials such as hydrogels, plastic and soil, including glass transitions, melting or crystallization events, drying dynamics, water binding and interactions with SOM (Ondruch et al., Reference Ondruch, Kucerik, Steinmetz and Schaumann2017; Sorolla-Rosario et al., Reference Sorolla-Rosario, Llorca-Porcel, Pérez-Martínez, Lozano-Castelló and Bueno-López2022; Sulaeman et al., Reference Sulaeman, Putro and Nikmatin2022). DSC has so far mostly been used for studying the thermal properties of pure SAPs for quality control and material testing (Drzeżdżon et al., Reference Drzeżdżon, Jacewicz, Sielicka and Chmurzyński2019) but may as well be applied for characterizing changes in the thermal profile of soil after SAP addition. As DSC provides information on the melting and crystallization behavior of soil water, it may help understand the role of water as an SAP plasticizer at different transformation stages to solid SAP residues (Buchmann et al., Reference Buchmann, Steinmetz, Brax, Peth and Schaumann2020).
Techniques to assess SAPs and their transformation
Imaging techniques can contribute to directly identifying and characterizing the structural features and dynamics of SAPs and solid SAP residues in soil. Various imaging techniques are available and include optical (light) microscopy, scanning electron microscopy (SEM), transmission electron microscopy (TEM), atomic force microscopy (AFM), X-ray computed tomography (CT) and magnetic resonance imaging (MRI) (Haber-Pohlmeier et al., Reference Haber-Pohlmeier, Tötzke, Lehmann, Kardjilov, Pohlmeier and Oswald2019; Joshi et al., Reference Joshi, Homburg and Ehrmann2022; Moud et al., Reference Moud, Kamkar, Sanati-Nezhad and Hejazi2022; Zhang et al., Reference Zhang, He, Gao, Mady, Filipović, Dyck, Lv and Liu2023).
SEM is a common and powerful technique that provides valuable information about sample surface morphology, microstructural features and elemental composition (Bo et al., Reference Bo, Renkuan, Yunkai, Tao, Peiling, Ji, Weimin and Zhichao2012; Li et al., Reference Li, Wufuer, Duo, Wang, Luo, Zhang and Pan2020; Huang et al., Reference Huang, Xu, Lei, Yu, Ouyang, Chen, Jia and Guo2021; Sarkar et al., Reference Sarkar, Diab and Thompson2023). Although SEM has been widely used to investigate SAPs and (micro)plastics in environmental research (Bo et al., Reference Bo, Renkuan, Yunkai, Tao, Peiling, Ji, Weimin and Zhichao2012; Buchmann and Schaumann, Reference Buchmann and Schaumann2017; Brax et al., Reference Brax, Köhne, Kroener and Schaumann2019; Malik et al., Reference Malik, Warkar and Saxena2023), it involves several limitations. These include altering structures during sample preparation and the restrictions of in situ measurements. Despite its successful application to SAPs and SAP–soil mixtures, sample preparation such as drying and coating can alter sample structures, limiting visual interpretation. Here, shock freezing and freeze drying can be suitable alternatives (Aston et al., Reference Aston, Sewell, Klein, Lawrie and Grøndahl2016; Brax et al., Reference Brax, Buchmann and Schaumann2017). In contrast to traditional SEM, environmental SEM (ESEM) can measure hydrated or wet samples without additional dehydration or coating with conductive materials (Qin et al., Reference Qin, Barsotti and Piri2021). Since such measures typically involve reduced sensitivity, further research is needed to make them fit for SAP characterizations.
In contrast to SEM, CT can be used to create three-dimensional images of the internal structure of samples. Especially in combination with advanced image processing, CT offers a noninvasive and nondestructive visualization of both living and nonliving media (Ferreira et al., Reference Ferreira, Pires and Reichardt2022; Zhang et al., Reference Zhang, Xi and Wei2023). CT works by irradiating a sample with X-rays, which are absorbed differently by its components, and the resulting images are reconstructed into three dimensions (Hampel, Reference Hampel and Wang2022). This technique provides information on sample porosity, structure and distribution of water and air (Ferreira et al., Reference Ferreira, Pires and Reichardt2022; Gong et al., Reference Gong, Nie, Xu, Ji and Liu2022; Young et al., Reference Young, Mooney, Heck, Peth, Mooney, Young, Heck and Peth2022). However, distinguishing SAP hydrogels from soil pore water is challenging as the density of SAP hydrogel-associated water is close to that of soil pore water (Brax et al., Reference Brax, Buchmann and Schaumann2017). Recent studies have shown that CT is suitable for (micro)plastic research, for example, for investigating their behavior and distribution in porous media (Tötzke et al., Reference Tötzke, Oswald, Hilger and Kardjilov2021; Ranjan et al., Reference Ranjan, Joseph, Sharma and Goel2023; Wang et al., Reference Wang, Li, Li, Yang and Jing2023). Thus, CT seems suitable for identifying solid SAP residues in soil together with their spatiotemporal distribution and structural characteristics.
AFM is a widely used technique for investigating environmental matrices, including SAPs, bacteria and soils (Hasan et al., Reference Hasan, Mohamed Keshawy and Abdel-Raouf2022). AFM creates high-resolution topographic maps of sample surfaces by measuring the forces between a probe and the sample at atomic resolution (Joshi et al., Reference Joshi, Homburg and Ehrmann2022). AFM can also measure the mechanical properties of hydrogels and plastics (Shi et al., Reference Shi, Dong, Ji, Fan, Yu, Xia and Sui2022; Xu et al., Reference Xu, Qin, Huang, Lin, Li and Xie2022; Muir et al., Reference Muir, Weintraub, Dhand, Fallahi, Han and Burdick2023). Although AFM offers several advantages over light microscopy and SEM, investigations of SAPs in soil are limited, mainly due to challenges in sample preparation, imaging speed and distinguishing between dehydrated hydrogels and solid SAP residues (Joshi et al., Reference Joshi, Homburg and Ehrmann2022). Quantifying the mechanical properties of solid SAP residues could further be challenged by spatiotemporal variations in cross-linking, composition and particle density. Complementary techniques such as SEM are necessary to obtain a comprehensive understanding of the transformation process.
MRI is an imaging technique used to investigate water distribution, diffusivity, swelling–shrinkage behavior and flow velocity in porous media such as soil and hydrogels (Dwihapsari et al., Reference Dwihapsari and Zakiah2019; Ranzinger et al., Reference Ranzinger, Hille-Reichel, Zehe, Guthausen and Horn2020; Haber-Pohlmeier et al., Reference Haber-Pohlmeier, Galvosas, Wang, Pohlmeier, Haber-Pohlmeier, Blümich and Ciobanu2022; Mirbod and Shapley, Reference Mirbod and Shapley2023). It can also provide information on soil structural characteristics, water content, nutrient and solute transport, network structure, swelling behavior and mechanical properties of hydrogels (Prior-Cabanillas et al., Reference Prior-Cabanillas, Barrales-Rienda, Frutos and Quijada-Garrido2007; Haber-Pohlmeier et al., Reference Haber-Pohlmeier, Tötzke, Lehmann, Kardjilov, Pohlmeier and Oswald2019, Reference Haber-Pohlmeier, Galvosas, Wang, Pohlmeier, Haber-Pohlmeier, Blümich and Ciobanu2022; Corradini et al., Reference Corradini, Pajewski, Di Censo, Alecci and Galante2022). Currently, only a few studies exist on the application of MRI in (micro)plastic research due to their small particle sizes, different magnetic properties and high background noise (Aghaei et al., Reference Aghaei, Mercer, Schneider, Sled, Macgowan, Baschat, Kingdom, Helm, Simpson, Simpson, Jobst and Cahill2022; Harvey et al., Reference Harvey, Mercer, Stapleton, Steeves, Hanrahan, Cui, Aghaei, Spring, Helm, Simpson, Simpson, Macgowan, Baschat, Kingdom, Sled, Jobst and Cahill2023). Furthermore, detecting solid SAP residues in soil can be challenging due to their overall low concentrations. MRI is time-consuming and expensive, and artifacts from ferromagnetic particles and soil drying can affect image quality (Merz et al., Reference Merz, Pohlmeier, Balcom, Enjilela and Vereecken2015; Oswald et al., Reference Oswald, Tötzke, Haber-Pohlmeier, Pohlmeier, Kaestner and Lehmann2015; Pflugfelder et al., Reference Pflugfelder, Metzner, van Dusschoten, Reichel, Jahnke and Koller2017). Despite these limitations, MRI has the potential to provide valuable insights into the behavior of SAP residues in soil and their interactions with various soil components.
Thermogravimetric analysis (TGA) can complement the already demonstrated techniques by providing information on the thermal stability and decomposition behavior of samples. TGA with optional MS coupling analyzes the mass losses and evolving gases induced by gradually heating a sample from room temperature to up to 1,000°C (David et al., Reference David, Steinmetz, Kučerík and Schaumann2018). Differences in the cross-linked structure and stabilization of SAP hydrogels and solid SAP residues by SOM, polyvalent cations and residual water are likely to result in different thermal stability of SAP hydrogels and solid SAP residues. Although this has not yet been demonstrated for SAPs, a recent TGA study by Fernández et al. (Reference Fernández, Redondo, Martín-de-León and Cantero2023) indicated clear differences in the thermogravimetric properties of PMA at different stages of polymer stabilization by water and at various pH levels. On this assumption, TGA may be a particularly promising tool for the discrimination of SAP hydrogels and solid SAP residues and for characterizing their transformation in soil. For more information on the application of TGA in soil and on polymers, see Kučerík et al. (Reference Kučerík, Svatoň, Malý, Brtnický, Doležalová-Weismannová, Demyan, Siewert and Tokarski2019) and Zaharescu et al. (Reference Zaharescu, Predoana, Pandele-Cusu, Klein, Aparicio and Jitianu2018), respectively.
Trace analysis of SAPs in environmental matrices
Classical analytical techniques for the quantification of SAPs in environmental matrices include ultraviolet/visible (UV/Vis) or fluorescence spectrometry and size exclusion chromatography (SEC) (Lu and Wu, Reference Lu and Wu2003). With recent advances in microplastic research, methods like Fourier transform infrared (FTIR) and Raman spectroscopy or pyrolysis–gas chromatography/mass spectrometry (GC/MS) have become increasingly popular (Thomas et al., Reference Thomas, Schütze, Heinze and Steinmetz2020; Steinmetz and Schröder, Reference Steinmetz and Schröder2022) and will probably be adapted to SAPs and other hydrophilic polymers in the near future. All these methods have in common that they are well suited for the quantification of SAP traces in the environment, and their ability to discriminate SAP hydrogels from microplastic-like solid SAP residues is, however, limited. To this end, adequate extraction methods tailored to existing instrumental techniques are to be developed. These could, for instance, exploit differences in density or solubility of SAP hydrogels and solid SAP residues in different solvents as it is common practice in microplastic analyses (Steinmetz et al., Reference Steinmetz, Kintzi, Muñoz and Schaumann2020; Thomas et al., Reference Thomas, Schütze, Heinze and Steinmetz2020).
UV/Vis photometry may be applied directly for quantifying PAM (Momani et al., Reference Momani, Al and Örmeci2014) or pDADMAC (Gumbi et al., Reference Gumbi, Ngila and Ndungu2014) in aqueous soil extracts at detection limits in the low ppm range. Prior iodine complexation of PAM (Lu and Wu, Reference Lu and Wu2003) and polyvinyl alcohol (Macias et al., Reference Macias, Bodugoz-Senturk and Muratoglu2013) or the addition of an azo dye for pDADMAC quantification (Mwangi et al., Reference Mwangi, Ngila and Ndungu2012) further increases specificity and sensitivity. Yet, functional groups like amides present both in SAPs and in soil will probably cause interferences and false-positive detections if not removed during sample cleanup.
SEC coupled with UV or refractive index (RI) detectors is capable of reaching similar detection limits to photometric methods but provides additional information on the molecular weight of the analyzed SAP (Lu and Wu, Reference Lu and Wu2003). The SAP’s molecular weight could be an important factor in predicting the SAP’s tendencity toward the formation of solid residues. A recent proof of principle further explored the potential of SEC coupled with a mass spectrometer (MS) for the trace analysis of polyethylene glycol (PEG) in aqueous samples at 10–100 μg L−1 (Huppertsberg et al., Reference Huppertsberg, Zahn, Pauelsen, Reemtsma and Knepper2020). Besides considerably lower detection limits, an MS is inherently more specific and less prone to false-positive detections compared to UV or RI detectors. Detection limits as low as 2 ng L−1 are common to liquid chromatography mass spectrometry applications, which are, however, mostly used for the quantification of SAP monomers like acrylamide in mushrooms (Castle, Reference Castle1993) or drinking water (Backe et al., Reference Backe, Yingling and Johnson2014; Mnif et al., Reference Mnif, Hurel and Marmier2014). Similarly, GC/MS may be applied for the analysis of PEG oligomers (Onigbinde et al., Reference Onigbinde, Nicol and Munson2001).
Pyrolysis–GC/MS has already been successfully applied for the quantification of poly(N-vinyl)caprolactam in wastewater effluents at 70 μg L−1 (Vidovic et al., Reference Vidovic, Krauskopf, Jovancicevic, Antic and Schwarzbauer2022) or PAM in sewage sludge with a limit of detection of 250 mg kg−1 (Kronimus and Schwarzbauer, Reference Kronimus and Schwarzbauer2007). Since SAPs are typically less thermally stable than other thermoplastic polymers, sample pyrolysis is conducted at temperatures below 500 °C and combined with thermally assisted hydrolysis and methylation agents like N,O-bis(trimethylsilyl)trifluoroacetamide and trimethylchlorosilane (Kumooka, Reference Kumooka2004) or tetramethylammonium hydroxide (Kronimus and Schwarzbauer, Reference Kronimus and Schwarzbauer2007).
FTIR and Raman spectroscopy, both established techniques for the identification of plastic particles, may as well be applied to SAP hydrogels or solid SAP residues. SAP hydrogels could be analyzed as gel, after (freeze)drying or after flocculation triggered by polyvalent ions or pH changes (Moud, Reference Moud2022; Anwar et al., Reference Anwar, Asrafuzzaman, Amin, Hoque, Thomas, Sharma, Jain and Shekhar2023). In addition to identifying the polymer itself, FTIR would be further capable of characterizing the degree of polymer degradation (Liang et al., Reference Liang, Du, Ma, Shen, Wu and Zhou2018; Freitas et al., Reference Freitas, de Oliveira, de Faria Abreu and da Silva Almeida2019) or cation exchange of the polymer framework (Rodionov et al., Reference Rodionov, Nii-Annang, Fischer, Gattinger, Bens, Raab and Hüttl2014). Similarly, near- and mid-IR imaging may be applied. Both methods are common for rapid and low-cost microplastic analyses (Wander et al., Reference Wander, Lommel, Meyer, Braun and Paul2022; Faltynkova and Wagner, Reference Faltynkova and Wagner2023), but have not been adapted to SAPs yet.
Handling perspectives and future trends
The increasing use of SAPs, their manifold entry routes into the soil and their overall low biodegradability underscore the need to further elucidate their potential to transform into solid SAP residues. There is particularly little research on the extent to which SOM–SAP interactions may influence this formation and whether or how the resulting changes in SAP and soil properties could affect the fate of environmental chemicals in soil. These aspects urge plastic pollution research to understand both the short- and long-term implications of SAPs on soil properties and soil functioning better.
Identifying the mechanisms and conditions of SAP aging and transformation in soil is crucial for effective management strategies throughout the life cycle of SAPs. Moreover, the impact of climate change and associated extreme weather events must be further investigated, as they could significantly influence the amount and frequency of SAP input and fate in the soil. Since targeted studies are generally missing, we suggest the following roadmap for future investigations:
-
1. Identifying the most relevant processes and their mutual interactions that trigger solid SAP residue formation in soil.
-
2. Assessing to what extent the quantity, frequency and methods of SAP application modulate solid SAP residue formation in soil.
-
3. Developing and applying suitable techniques to assess the formation and spatiotemporal distribution of solid SAP residues in soil and their effect on soil properties and soil functioning.
-
4. Establishing monitoring and quantification methods that will help to evaluate potential environmental impacts and mitigation measures, particularly in the field.
Considering that solid SAP residues are found to form in the short term and long term, several measures for SAP producers, consumers and disposers can be considered. These include developing eco-friendly and biodegradable SAPs, ensuring proper usage and disposal of SAP-containing products, educating all stakeholders about associated risks and exploring sustainable approaches for application, disposal and remediation.
Open peer review
To view the open peer review materials for this article, please visit http://doi.org/10.1017/plc.2024.2.
Data availability statement
Data availability is not applicable to this article as no new data were created or analyzed in this study.
Acknowledgments
We kindly thank Gabriele E. Schaumann for her valuable feedback on the manuscript as well as Allan Philippe for providing the idea for Figure 3.
Author contribution
C.B. and Z.S. conceptualized the study; C.B., J.N., M.M., M.B. and Z.S. conducted the literature research, wrote, reviewed and edited the manuscript; and Z.S. supervised the study. All authors have read and agreed to the published version of the manuscript.
Financial support
This research was financially supported by the Deutsche Forschungsgemeinschaft (Grant No. BU 3763/1–1).
Competing interest
The authors declare none.
Comments
Dear Prof. Fletcher, dear Dr. Oluwasanya,
Thank you very much for your kind invitation to contribute a review article to the first issue of Cambridge Prisms: Plastics.
We are pleased to submit our manuscript entitled “Superabsorbent polymers in soil—the new microplastics?” which evaluates the current scientific understanding of the application and fate of superabsorbent polymers in soil, with particular focus on their potential to form (micro)plastic-like solid residues.
Given the limited attention that superabsorbent polymers have received in (micro)plastics and polymer research, we believe this review will be of significant interest to the scientific community.
Sincerely,
Zacharias Steinmetz on behalf of all authors