Introduction
Non-native species are being introduced into many countries with increasing frequency. These exotic organisms can threaten and damage ecosystems, biodiversity, agriculture and even human health (Wittenberg and Cock, Reference Wittenberg and Cock2001; Pimentel et al., Reference Pimentel, Zuniga and Morrison2005; Pejchar and Mooney, Reference Pejchar and Mooney2009). Biological invasions present important problems in different facets of human society, such as environmental, human health and agricultural production issues. Biological invasions lead to pest problems that can cause considerable economic damage due to losses of agricultural and forestry crops. Additionally, exotic species can disturb ecosystems and trophic networks through the displacement and extinction of native species (Gurevitch and Padilla, Reference Gurevitch and Padilla2004), causing environmental damage and biodiversity loss (Vitousek et al., Reference Vitousek, D'Antonio, Loope and Westbrooksw1996; Pimentel et al., Reference Pimentel, Zuniga and Morrison2005). The damage to biodiversity and the economy produced by non-native species are due to their high propensity for dispersal and explosive production of large populations during the first years of an invasion resulting from their establishment in an environment without their natural enemies; the ‘enemy-release hypothesis’ (Williamson, Reference Williamson1996; Crawley, Reference Crawley1997). In the case of arthropodan biological invasions, one of the most important groups related to natural enemies is the parasitoids (Quicke, Reference Quicke1997; Schönrogge et al., Reference Schönrogge, Moriya, Melika, Randle, Begg, Aebi, Stone, Ozaki, Yukawa, Ohgushi and Price2006), mostly belonging to the order Hymenoptera that have theoretically co-speciated with their hosts through adaptations to overcome their hosts' defences (top-down relations), and the hosts modifying their defences to avoid being parasitized (bottom-up relations) (Stone et al., Reference Stone, Schönrogge, Atkinson, Bellido and Pujade-Villar2002).
Invasions by gall-inducing insects such as gall wasps (Hymenoptera: Cynipidae) present difficulties for pest control because these organisms spend most of their life cycle concealed inside a vegetal structure (the gall) which is part of the host plant (Rohfritsch and Shorthouse, Reference Rohfritsch, Shorthouse, Kahl and Schell1982). As cynipids are in their larval and pupal stages inside galls, many types of pest control, such as chemical biocides, are ineffective (Cooper and Rieske, Reference Cooper and Rieske2007). Therefore, knowledge of their association with natural parasitoid enemies, most of which belong to the superfamily Chalcidoidea (Askew et al., Reference Askew, Plantard, Gómez, Hernández-Nieves and Nieves-Aldrey2006, Reference Askew, Melika, Pujade-Villar, Schönrogge, Stone and Nieves-Aldrey2013), can indicate which of the parasitoid species have potential as biological control agents and would be candidates for intentional introductions to new regions.
An example of an invasive gall wasp biological invasion is Andricus quercuscalicis Burgsdorf 1783 (Collins et al., Reference Collins, Crawley and McGavin1983; Stone and Sunnucks, Reference Stone and Sunnucks1993; Schönrogge et al., Reference Schönrogge, Stone and Crawley1995; Schönrogge et al., Reference Schönrogge, Moriya, Melika, Randle, Begg, Aebi, Stone, Ozaki, Yukawa, Ohgushi and Price2006), which was introduced to western Europe from south-eastern Europe through the planting and human transport of Quercus cerris. In its invaded range, An. quercuscalicis impedes the development and production of Quercus acorns.
Another example, addressed in this paper, is provided by the Asian chestnut gall wasp, Dryocosmus kuriphilus Yasumatsu, 1951, a parthenogenetic cynipid from China that has colonized Castanea Mill. trees in North America (Payne et al., Reference Payne, Menke and Schroeder1975), Japan (Oho and Umeya, Reference Oho and Umeya1975) and Europe (Brussino et al., Reference Brussino, Bosio, Baudino, Giordano, Ramello and Melika2002) and is an economic pest of chestnut forests in these newly colonized territories (Payne et al., Reference Payne, Menke and Schroeder1975; EFSA, 2010; Gehring et al., Reference Gehring, Bellosi, Quacchia and Conedera2017). D. kuriphilus is the only taxon of Cynipidae to attack chestnuts in Europe and North America, and there was, therefore, no native parasitoid fauna associated with cynipids on Castanea trees when it was introduced (Stone et al., Reference Stone, Schönrogge, Atkinson, Bellido and Pujade-Villar2002), unlike the situation in China, where this cynipid has natural enemies (Moriya et al., Reference Moriya, Inoue, Otake, Shiga and Mabuchi1989). The best-studied natural enemy of D. kuriphilus is Torymus sinensis Kamijo, 1982 (Hymenoptera: Torymidae), a chalcid parasitoid that is also native to China and which has been used as a biological control agent in many countries (Moriya et al., Reference Moriya, Shiga, Adachi and Van Driesche2003; Cooper and Rieske, Reference Cooper and Rieske2007; Quacchia et al., Reference Quacchia, Moriya and Bosio2013; Matošević et al., Reference Matošević, Lacković, Melika, Kos, Franić, Kriston and Rot2016; Borowiec et al., Reference Borowiec, Thaon, Brancaccio, Cailleret, Ris and Vercken2018; Avtzis et al., Reference Avtzis, Melika, Matošević and Coyle2019). This torymid appears to be a host-specific parasitoid of D. kuriphilus, and its life cycle is synchronized with that of its host, although there is also evidence that T. sinensis can parasitize native oak galls (Ferracini et al., Reference Ferracini, Ferrari, Saladini, Pontini, Corradetti and Alma2015, Reference Ferracini, Bertolino, Bernardo, Bonsignore, Faccoli, Ferrari, Lupi, Maini, Mazzon, Nugnes, Rocco, Santi and Tavella2018). As the total attack rates of native parasitoid species remained low for many years after the arrival of D. kuriphilus (Quacchia et al., Reference Quacchia, Ferracini, Nicholls, Piazza, Saladini, Tota, Melika and Alma2012), biological control programmes involving T. sinensis appeared to be the most promising method of controlling the pest in chestnut forests worldwide (Moriya et al., Reference Moriya, Inoue and Mabuchi1990; Quacchia et al., Reference Quacchia, Moriya, Bosio, Scapin and Alma2008). However, the use of T. sinensis in biocontrol involves introducing an exotic species, and this could potentially lead, in the absence of adequate screening, to environmental problems, especially non-target impacts (Gibbs et al., Reference Gibbs, Schönrogge, Alma, Melika, Quacchia, Stone and Aebi2011; Ferracini et al., Reference Ferracini, Ferrari, Saladini, Pontini, Corradetti and Alma2015, Reference Ferracini, Bertolino, Bernardo, Bonsignore, Faccoli, Ferrari, Lupi, Maini, Mazzon, Nugnes, Rocco, Santi and Tavella2018).
The studied D. kuriphilus communities showed high parasitoid species recruitment in a short time, and new biological communities were formed in many territories, though parasitism rates were low. This contrasts with the invasion of Western Europe by An. quercuscalicis and other Andricus cynipid species, in which effective parasitoid recruitment did not begin until after more than 20 years (Schönrogge et al., Reference Schönrogge, Moriya, Melika, Randle, Begg, Aebi, Stone, Ozaki, Yukawa, Ohgushi and Price2006). The parasitoid communities recruited by D. kuriphilus have been studied in many countries such as USA (Cooper and Rieske-Kinney, Reference Cooper and Rieske-Kinney2006), Italy (Aebi et al., Reference Aebi, Schönrogge, Melika, Quacchia, Alma and Stone2007; Quacchia et al., Reference Quacchia, Ferracini, Nicholls, Piazza, Saladini, Tota, Melika and Alma2012; Panzavolta et al., Reference Panzavolta, Bernardo, Bracalini, Cascone, Croci, Gebiola, Iodice, Tiberi and Guerrieri2013), Croatia (Matošević and Melika, Reference Matošević and Melika2013) and Slovenia (Kos et al., Reference Kos, Kriston and Melika2015). In Spain, the first native recruited parasitoids of D. kuriphilus were reported from Catalonia (Jara-Chiquito et al., Reference Jara-Chiquito, Heras and Pujade-Villar2016), and preliminary lists of parasitoids of D. kuriphilus in Galicia have also been published (Pérez-Otero et al., Reference Pérez-Otero, Crespo and Mansilla2017; Santolamazza-Carbone et al., Reference Santolamazza-Carbone, Luna-Grande, Cordero-Rivera, Nieves-Aldrey and Cuenca Valera2018). However, there have been no reports from another important chestnut-growing region of Spain where the chestnut crop economy has been badly affected in recent years by D. kuriphilus: Málaga Province (Andalusia, southern Spain).
On the Iberian Peninsula, D. kuriphilus was first detected in Catalonia in 2012 (EPPO, 2012; Pujade-Villar et al., Reference Pujade-Villar, Torrell and Rojo2013), and new D. kuriphilus hotspots then appeared rapidly in other Spanish regions (Gil-Tapetado et al., Reference Gil-Tapetado, Gómez, Cabrero-Sañudo and Nieves-Aldrey2018). The species was recorded in Galicia (north-western Spain) and Andalusia (southern Spain) in 2014, and it was detected in the northwest of Portugal in 2014 (EPPO, 2014).
The Iberian Peninsula is a heterogeneous territory incorporating different climate and with marked variations in temperature and precipitation across a latitudinal gradient (Hawkins et al., Reference Hawkins, Porter and Diniz-Filho2003a, Reference Hawkins, Field, Cornell, Currie, Guégan, Kaufman, Kerr, Mittelbach, Oberdorff, O'Brien, Porter and Turner2003b; Cabrero-Sañudo and Lobo, Reference Cabrero-Sañudo and Lobo2006; Mittelbach et al., Reference Mittelbach, Schemske, Cornell, Allen, Brown, Bush, Harrison, Hurlbert, Knowlton, Lessios, McCain, McCune, McDade, McPeek, Near, Price, Ricklefs, Roy, Sax, Schluter, Sobel and Turelli2007; Vetaas and Ferrer-Castán, Reference Vetaas and Ferrer-Castán2008). Considering this climatic diversity, and despite the consistent worldwide patterns of D. kuriphilus communities (Aebi et al., Reference Aebi, Schönrogge, Melika, Alma, Bosio, Quacchia, Picciau, Abe, Moriya, Yara, Seljak, Stone, Ozaki, Yukawa, Ohgushi and Price2006), regional differences were expected to be found in the D. kuriphilus parasitoid communities in Spain. Differing environmental conditions lead to differences in vegetation, and Quercus species diversity close to stands of chestnut trees infested by D. kuriphilus is one of the most important sources of dissimilarity between native gall wasp parasitoid communities (Nieves-Aldrey, Reference Nieves-Aldrey2001; Askew et al., Reference Askew, Melika, Pujade-Villar, Schönrogge, Stone and Nieves-Aldrey2013). Different oak tree species support different native cynipid species, and these in turn support different chalcid parasitoid species. This could explain the differing composition of parasitoid communities recruited by D. kuriphilus in different geographical sites in Spain.
Here, we describe and analyse the chalcid parasitoid communities recruited by D. kuriphilus in two geographically distant areas of Spain: (1) Málaga province (Andalusia, southern Spain) and (2) the autonomous community of Galicia (northwest Spain). Both geographical areas harbour important Castanea forests which have been invaded by D. kuriphilus in recent years, causing severe chestnut yield losses. Consequently, controlled releases of T. sinensis have been authorized by the Spanish government and implemented in recent years (Nieves-Aldrey et al., Reference Nieves-Aldrey, Gil-Tapetado, Gavira, Boyero, Polidori, Lombardero, Blanco, Rey Del Castillo, Rodríguez-Rojo, Vela and Wong2019). These parasitoid communities are characterized by differences in the abundance and composition of species and by their emergence dates. Our study provides the first information on the species communities formed de novo in 2014, which is of great economic interest, given the status of D. kuriphilus as an important pest of Castanea trees. We examine the role that T. sinensis is playing in these novel communities in the two areas where this torymid has been released, and we reveal apparent differences. Finally, we lay the groundwork for future studies on the interactions of the recruited D. kuriphilus community with the native parasitoid community associated with cynipids inducing galls on oak hosts (Quercus spp., Fagaceae).
Materials and methods
Sampling area
To perform standardized sampling, 15 localities were selected according to the D. kuriphilus infestation data across the zones provided by the Spanish regional public administration of Andalusia and Galicia. These localities are in two different regions: (1) Sierra de las Nieves and Valle del Genal (Málaga, south Spain) and (2) Galicia (northwest Spain), the latter divided into two sub-regions: (i) Mediterranean Galicia (southern Galicia) and (ii) Eurosiberian Galicia (northern Galicia) are chosen to represent zones of D. kuriphilus hotspots (Gil-Tapetado et al., Reference Gil-Tapetado, Gómez, Cabrero-Sañudo and Nieves-Aldrey2018). Coordinates of the sampling areas are recorded in table 1, and their geographical locations are indicated in fig. 1.

Figure 1. Maps of the locations of the sampling points on the IP. (a) The region of Galicia and (b) the region of Málaga. The names of the localities of each sampling point appear in table 1.
Table 1. Dryocosmus kuriphilus localities sampled in this survey
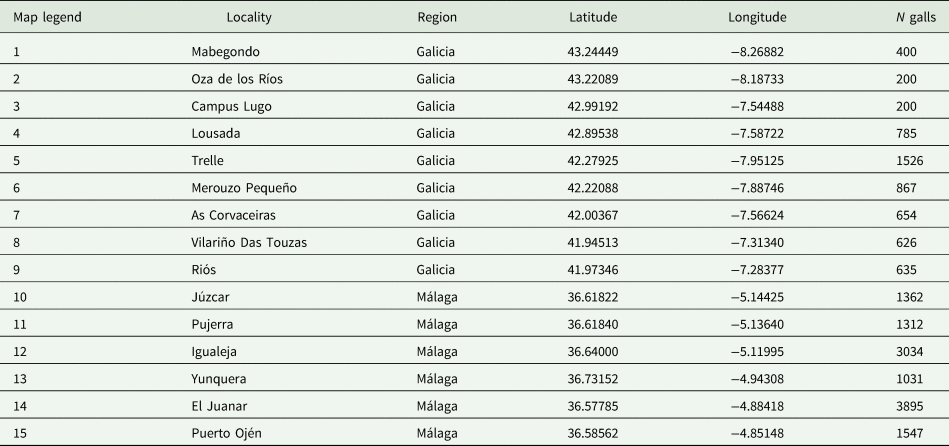
The total number of sampled galls is indicated in the N galls column. The map legend shows the relationships of the sampling points that appear in fig. 1 and their corresponding locality.
The Málaga region studied in this survey refers only to two different areas: (1) Sierra de las Nieves and Sierra Blanca (localities of Yunquera, El Juanar and Puerto Ojén), with areas of 202 and 70 km2, respectively, and an average altitude of 1000 m a.s.l.; and (2) Valle del Genal (localities of Pujerra, Igualeja and Júzcar), with an area of 485 km2 and an average altitude of 700 m a.s.l. In the sampling sites of the Málaga region, the predominant vegetation is composed of oak forests of Quercus suber L. and Quercus canariensis Willd., with Quercus faginea Lam., Quercus ilex L., and Quercus coccifera L. in more xeric areas. The presence of chestnut trees at higher altitudes (up to 1700 m a.s.l.) in the Sierra de las Nieves, in a relict forest of Quercus alpestris Boiss., is remarkable. These mixed forests of Quercus and Castanea nestle in these mountains and valleys, but they are always at an altitude of ~800 m a.s.l. In the Galicia region (with an area of 29,575 km2), chestnut trees are distributed more widely, from sea level to a high altitude, because of the more humid conditions and high rainfall throughout the entire territory, but especially in the Eurosiberian subregion. Eurosiberian Galicia is a colder and rainier environment that favours the dominance of Quercus robur L. over other Quercus species in this area (Amaral Franco, Reference Amaral Franco, Castroviejo, Laínz, López González, Montserrat, Muñoz Garmendia, Paiva and Villar1990). In Mediterranean Galicia, oak forests of Quercus pyrenaica Willd. and Q. suber are predominant, while Q. ilex is restricted to only a small area of Sierra da Enciña Lastra close to the community of Castilla y León.
Gall collection and rearing
At each sampling site, a minimum of 200 galls was collected (a total of 18,074 galls, 5893 in Galicia and 12,181 in Málaga) (table 1). D. kuriphilus galls were collected from the trees between ground level and a height of 2 m. Galls were collected during multiple sampling campaigns between March 2017 and January 2019 in both regions. Fresh galls were collected in spring and summer samplings, and dry ones were collected in autumn and winter.
D. kuriphilus galls were stored for rearing in cardboard boxes equipped with skylight extractors and were maintained indoors in the Museo Nacional de Ciencias Naturales of Madrid (MNCN-CSIC). The boxes were checked daily to collect emerged parasitoids. Specimens were preserved in vials with 99% ethanol and labelled with their emergence date and collection location.
Parasitoid identification
Parasitoids were identified to species using available morphological taxonomic keys (Nieves-Aldrey, Reference Nieves-Aldrey1983, Reference Nieves-Aldrey1984a, Reference Nieves-Aldrey1984b, unpublished) and unpublished keys from Askew and Thúroczy for an update in some parasitoid families. Some of the reared parasitoid species, such as Eupelmus urozonus Dalman, 1820, Ormyrus pomaceus (Geoffroy, 1785), and Bootanomyia dorsalis (Fabricius, 1798), include complex cryptic lineages that are difficult to distinguish morphologically, as has been revealed in studies with molecular markers and other tools (Al-Khatib et al., Reference Al Khatib, Fusu, Cruaud, Gibson, Borowiec, Rasplus, Ris and Delvare2014; Nicholls et al., Reference Nicholls, Preuss, Hayward, Melika, Csóka, Nieves-Aldrey, Askew, Tavakoli, Schönrogge and Stone2010; Goméz et al., Reference Goméz, Hernández-Nieves, Gayubo and Nieves-Aldrey2017). A conservative approach to the identification of species belonging to these cryptic species aggregates was chosen, and they were assigned to the binominal combination used for the aggregate or complex (table 2). In addition, all published information was compiled (table 3) to show the absolute numbers of parasitoids sampled from galls of D. kuriphilus in Spain.
Table 2. List of inquilines and native and non-native chalcidoid parasitoids reared from galls of D. kuriphilus from sampling sites in Galicia and Málaga

Ig, Igualeja; Pu, Pujerra; Jz, Júzcar; Ju, Juánar; PO, Puerto Ojén; Yu, Yunquera; Ri, Riós; VT, Vilariño das Touzas; AC, As Corvaceiras; MP, Merouzo Pequeño; Tr, Trelle; CL, Campus Lugo; Lo, Lousada; Ma, Mabegondo; OR, Oza de los Rios.
The inquiline species is underlined, and the released non-native species is indicated in bold. The sum columns show the total individuals of a species, indicating the most abundant species in blue and the rarest species from the sampling campaigns in red.
a Probably related to secondary fauna that inhabits D. kuriphilus galls.
Table 3. Compilation of the native parasitoids and inquilines (*) reared in all reports addressing the D. kuriphilus communities of Spain
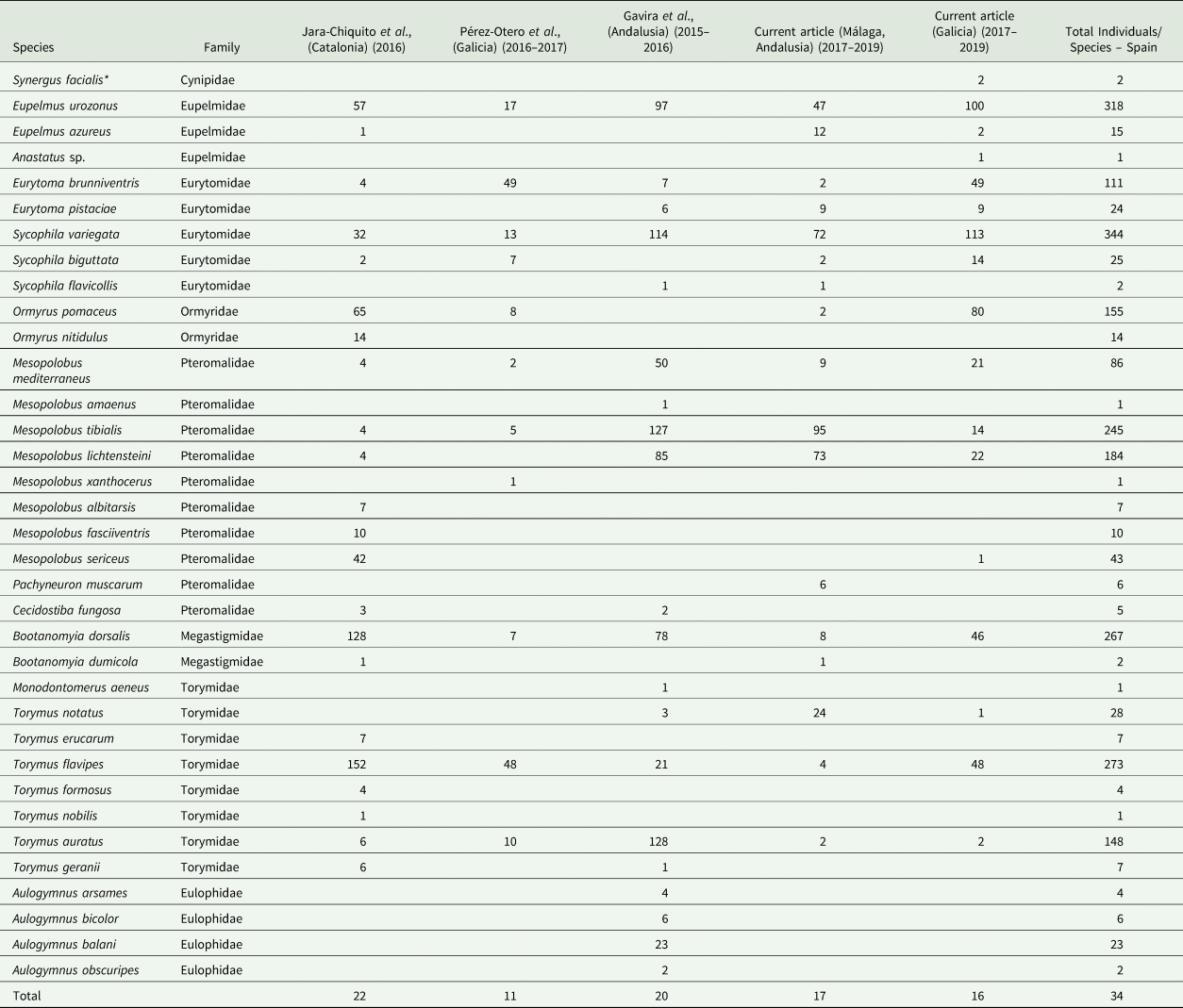
Sampling effort curves
To calibrate the completeness of the inventory of D. kuriphilus parasitoid communities, an evaluation of the sampling of the 15 studied areas was performed with sampling effort curves (SECs). SECs can estimate the degree of sampling of the different D. kuriphilus communities by sampling area and locality, to observe any possible bias that exists in the description of the community according to the data obtained, and to indicate the strength of the analyses.
To perform this evaluation, the software EstimateS (Colwell, Reference Colwell2018) was used to calculate the Chao2 estimator of species incidences and CurveExpert (Hyams, Reference Hyams2007) to obtain the Clench adjustment curve (Jiménez-Valverde and Hortal, Reference Jiménez-Valverde and Hortal2003). The parameters of the curve slope (slope), the percent of registered species (%rs) and the percent of realized effort (%re) were calculated using the a and b parameters of the Clench curve. These last analyses were performed in R Studio (RStudio Team, 2019) (table 4).
Table 4. Results of sampling effort curves (SECs) by locality, region and subregion of Málaga and Galicia
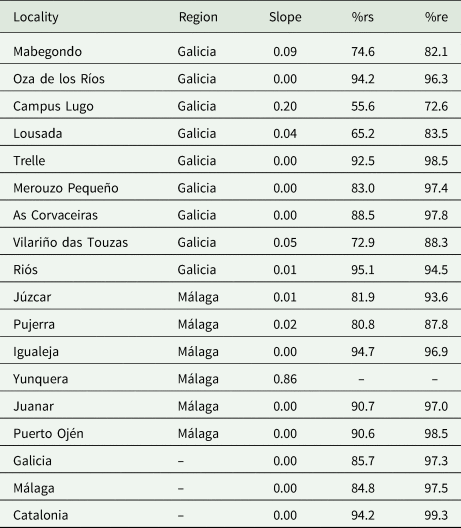
The slope represents the Clench curve slope of the SEC; %rs is the percentage of species observed; and %re is the percentage of the realized sampling effort by area. Localities with insufficient data are indicated by – for each unfulfilled parameter. Data from the Catalonia region were reported by Jara-Chiquito et al., (2017) to show the communities studied in detail on the Iberian Peninsula.
Analyses of communities
To describe biodiversity patterns in D. kuriphilus communities, three different parameters were analysed: (1) species richness (sS), (2) parasitoid emergence dates, and (3) species nestedness and structure.
To calculate sS (1), the identified individuals from each locality were counted by species, as shown in table 2. The numbers of the Hill series or true diversity (Jost, Reference Jost2006) of predicted sS (q 0), effective sS (q 1), dominant sS (q 2) and accessory sS (q 0−q 1) were calculated using R Studio (RStudio Team, 2019) with the Spade R library (Chao et al., Reference Chao, Ma, Hsieh and Chiu2016). The ChaoJost Hill series of sS indicates a predicted sS according to the data for each biological community, showing the theoretical number of possible species that could constitute each community (table 5).
Table 5. Species richness (sS) diversity of parasitoids of D. kuriphilus communities of Málaga and Galicia by locality and region

Empirical sS indicates the sampled species diversity in each area, while ChaoJosts S provides a prediction of possible diversity in each zone. The sS diversity of the Catalonia region reported by Jara-Chiquito et al. (2017) is presented to show all the communities studied in detail on the IP. q 0 = total sS; in empirical q 0 = observed sS; q 1 = sS of effective species; q 2 = sS of dominant species; and q 0–q 1 = sS of accessory species.
Emergence date graphs of the parasitoid species (2) were generated using the relative abundance of species, considering the total abundance per parasitoid divided by the number of D. kuriphilus galls per sample (table 1). In this way, the differences among the absolute numbers of parasitoids are reduced and standardized, producing a graph that is better adjusted to the biological community. These graphs were generated for different Chalcidoidea families associated with D. kuriphilus, separated by region, and were created using R Studio with the library ggplot2 (Wickham, Reference Wickham2018). The graphs show the presence of parasitoid adults between May 2017 and March 2018 in both regions (fig. 2).
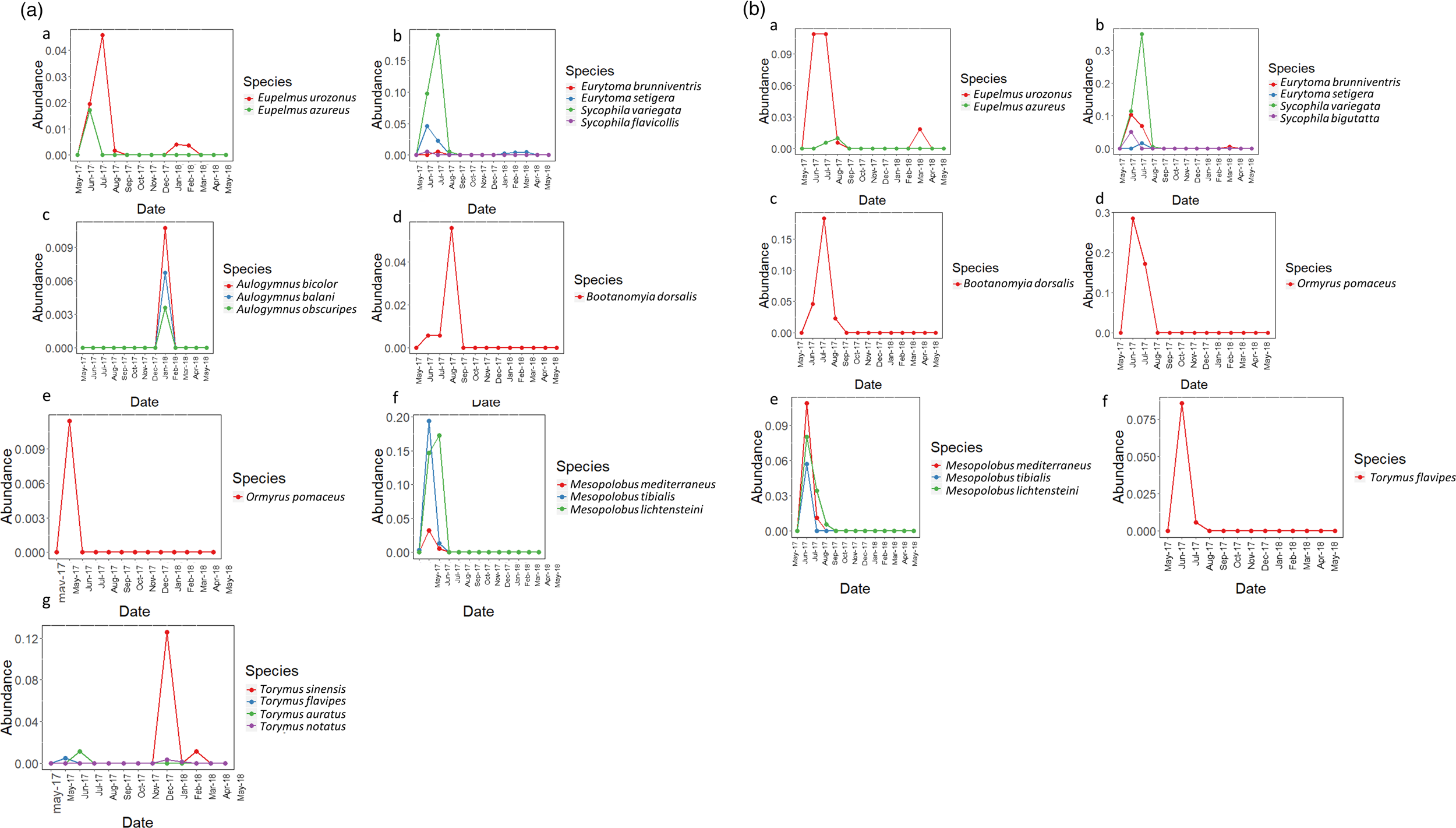
Figure 2. Emergence date charts of parasitoid species of D. kuriphilus obtained in this survey, grouped by family. The y-axis represents the relative abundance of each species of the corresponding family, and the x-axis represents their emergence date from May 2017 to March 2018. (A) The Málaga region. (a) Eupelmidae; (b) Eurytomidae; (c) Eulophidae; (d) Megastigmidae; (e) Ormyridae; (f) Pteromalidae; (g) Torymidae; and, (B) represents the region of Galicia (a) Eupelmidae; (b) Eurytomidae; (c) Megastigmidae; (d) Ormyridae; (e) Pteromalidae; (f) Torymidae.
Nestedness analyses of D. kuriphilus community (3) showed the structure and species evenness of both communities. These analyses were performed with the Nestedness Temperature Calculator v. 2.0 software (Ulrich, Reference Ulrich2006; Ulrich et al., Reference Ulrich, Almeida-Neto and Gotelli2009) using 10,000 random runs. Additionally, system temperatures were compared with temperatures obtained at random, while Monte Carlo-derived probabilities were generated to confirm the nestedness of communities, and the ordering of the species in the locality richness matrix was obtained together with the state occupancy percent (fig. 3). Differences between the 2017–2018 and 2018–2019 were not calculated due to the difference in sampling numbers between these periods. The probability of state occupancy probability for each species was also calculated by the program. Nested distributions imply that the most ubiquitous species will virtually always appear on the most hospitable locality, whereas the most marginal species will appear on the least hospitable locality; the other species vary in a specifiable manner between these two extremes, permitting us to calculate the probability of their occupancy in the other localities (Atmar and Patterson, Reference Atmar and Patterson1993). This is represented by colours, with black being a 100% occupancy percentage and the other colours in fig. 3 being a lower percentage, with red being the lowest percentage between 0 and 10%.
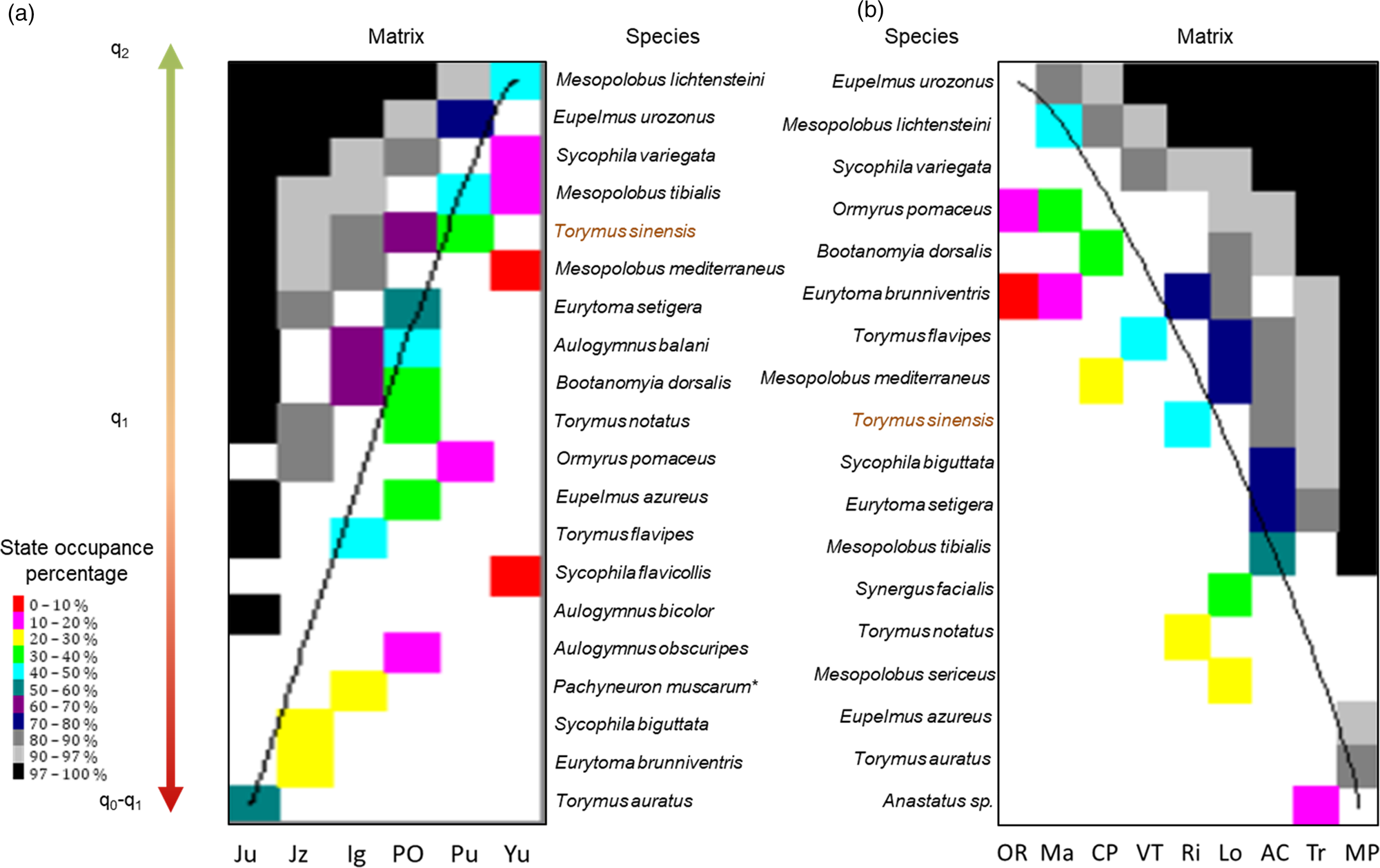
Figure 3. Nestedness diagram and structure of both communities of D. kuriphilus. (a) The D. kuriphilus community of Málaga and (b) the community of Galicia. Rows indicate the parasitoid species, and columns represent each sampling locality. The order of species from top to bottom is related to their corresponding strong or weak role in the community, as related to q 2, q 1 and q 1–q 0 diversity. Species at the top are related to dominant species and species at the bottom to the accessory species of the community. The coloured squares of the matrix indicate the state occupancy percentage or the abundance degree of each parasitoid in each locality. A high state occupancy percentage indicates high abundances and stronger roles in each locality and the whole community, while low percentages indicate low abundances and weaker roles in each locality and community.
Results
Native parasitoid species recruited by D. kuriphilus on the Iberian Peninsula
The parasitoids identified in this survey included a total of 22 species of Chalcidoidea, 17 of which were present in the Galicia region and 19 in the Málaga region (table 2). Almost all obtained species exhibit a generalist biology with a wide host range (Askew et al., Reference Askew, Melika, Pujade-Villar, Schönrogge, Stone and Nieves-Aldrey2013; Noyes, Reference Noyes2019), and many of them are multivoltine. The absence of the family Eulophidae in the samples from the Galicia region can be highlighted. Pachyneuron muscarum (Linnaeus, 1758), reared in the Málaga samples, was probably present due to the secondary fauna of Homoptera that use D. kuriphilus galls as a refuge. This species almost certainly plays no part in the D. kuriphilus parasitoid community as a natural enemy of the gall insect.
Four species, Torymus notatus (Walker, 1833), Aulogymnus bicolor (Askew, Reference Askew, Vinson and Price1975), Aulogymnus balani (Pujade-Villar, Reference Pujade-Villar1991) and Aulogymnus obscuripes (Mayr, 1877) stand out among the identified native parasitoids because of their biological characteristics. The three species of Aulogymnus are recorded for the first time from the D. kuriphilus parasitoid community in Spain. Unlike most of the native parasitoids recruited by D. kuriphilus, these four chalcids are similar to T. sinensis in being univoltine species that develop only on the cynipid host and hibernate as pharate adults inside old galls remaining on the tree in winter. Their early spring emergence dates are synchronized with the early development of their host galls.
A total of 1153 chalcid parasitoids and two inquiline cynipid individuals was reared from the galls collected in all standardized sampling campaigns in Málaga and Galicia (table 2). The total relative abundances of the recruited parasitoids (total parasitoids divided by the total collected galls by region) were similar in the Mediterranean areas of Galicia and Málaga (0.09 and 0.05, respectively). However, despite the number of galls collected in both regions, the relative abundance was greater in Galicia. The most abundant native parasitoid species in both regions were Mesopolobus tibialis (Westwood, 1833) (109), Mesopolobus lichtensteini (Mayr, 1903) (95), Eup. urozonus (147), Sycophila variegata (Curtis, 1831) (185) and O. pomaceus (82). However, while M. tibialis was a dominant species in Málaga and an accesory species in Galicia, O. pomaceus was dominant in Galicia and accesory in Málaga (fig. 3). If both native and non-native parasitoids are considered within the recruited parasitoid community of D. kuriphilus in the two study areas, then the dominant species is the alien released species T. sinensis, of which a total of 231 individuals were recovered, including 212 from Málaga and 19 from galls collected in Galicia.
Sampling effort curves
The sampling effort was sufficient at all the collecting sites except for two, Yunquera and Campus Lugo (table 4). On average, excepting for these two localities, the SECs per locality showed a slope of 0.03, 85%rs and 93.2%re. Considering the results for the Galicia and Málaga regions, the SECs showed a mean slope of 0.00 (the SECs have reached the asymptote), with 85.7 and 84.8%rs and 97.3 and 97.5%re, respectively. As the values of these parameters met the usual evaluation criteria (slope <0.1, %rs>75 and %re>75), the sample collected was theoretically representative of the real biological community, thus possible bias was minimized and the data were sufficient to support the following analyses.
Species richness
sS varied between the regions, being higher in Málaga, with an observed or empirical q 0 = 19, ChaoJost q 0 ≈ 19.1, and a mean per locality of q 0 = 9.2 (table 5). The Galicia region exhibited an empirical q 0 = 17, ChaoJost q 0 ≈ 17.5 and a mean per locality q 0 = 7.4. The empirical effective species q 1 was higher in Galicia than in Málaga (9.094 and 7.212, respectively). The dominant species values of the communities in the Galicia and Málaga regions were different (7.333 and 4.916, respectively), and the q 2 value of the Málaga community was very similar to that reported in Catalonia (5.184) (Jara-Chiquito et al., Reference Jara-Chiquito, Heras and Pujade-Villar2016). The number of accessory species (q 0–q 1) also varied between the regions, being much higher in Málaga (11.79) than in Galicia (7.91).
Emergence date and relative abundance of native and non-native parasitoids
The emergence date charts showed a high relative abundance of T. sinensis in the Málaga region and low representation in Galicia (fig. 2A, B). They also emphasized the abundance difference between T. sinensis and the native Torymus species in the Málaga region (212 vs. 30 total individuals, respectively) (fig. 2A.g). Together with T. sinensis, other species that constitute a biological sub-community with an emergence date at the beginning of the year (February–March) appear when new galls are starting to develop. This overwintering sub-community includes three species of the genus Aulogymnus (fig. 2A.c) and T. notatus (figs 2A.g and 4). The emergence date charts also show the existence of multi- or bivoltine species, such as Eup. urozonus (fig. 2A.a and B.a) and Eurytoma brunniventris Ratzeburg, 1852, only in the Galicia region (fig. 2B.a). The emergence date of B. dorsalis (fig. 2A.d and B.c) is later than that of other native parasitoids.
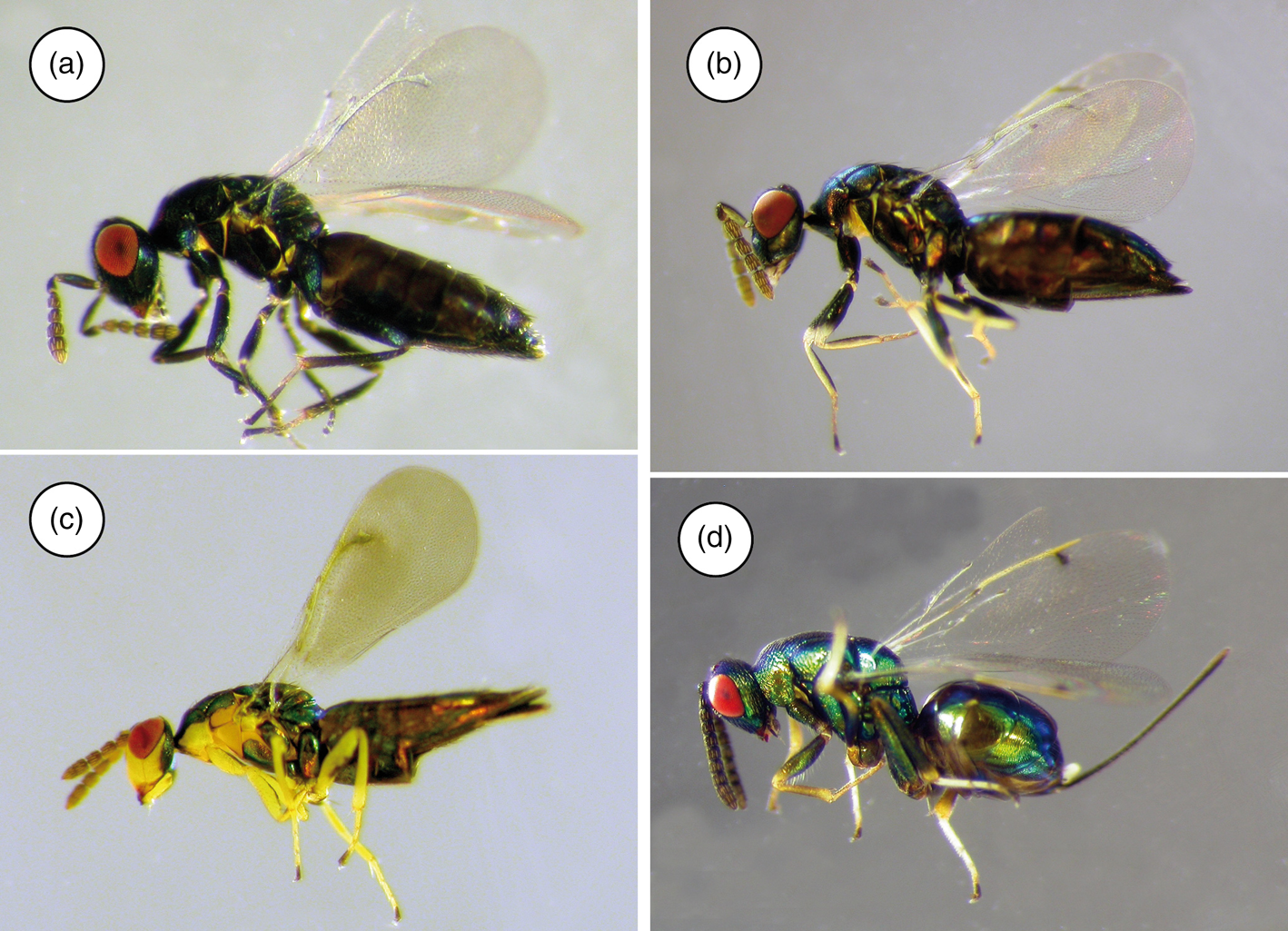
Figure 4. Habitus of native species of Chalcidoidea representatives of the overwintering sub-community associated with D. kuriphilus in Spain. Females of (a) Aulogymnus obscuripes; (b) Aulogymnus balani; (c) Aulogymnus bicolor; (d) Torymus notatus.
There are a relatively large number of accessory species in the Málaga community (fig. 3), but they are present in small numbers of individuals and their impact in the recruited community is probably insignificant. The highest relative abundance among all parasitoids in the sample was found for Syc. variegata in the locality of Trelle, in the Mediterranean subregion of Galicia, at 0.14%. The relative abundance of T. sinensis reared from all the sampled D. kuriphilus galls was very low (<0.1%).
Species nestedness and community structure
Comparison of the nestedness diagram of both communities together with sS (fig. 3) estimates how many species were categorized as the most dominant species (q 2), effective species (q 1) and most-accessory species (q 0–q 1) of each D. kuriphilus parasitoid community. In the Málaga region, the most abundant species were M. lichtensteini, Eup. urozonus, Syc. variegata, M. tibialis and T. sinensis, while in Galicia, the dominant species were Eup. urozonus, M. lichtensteini, Syc. variegata and O. pomaceus. The state occupancy percentage indicated the most-accessory species of each community. These accessory species were Sycophila flavicollis (Walker, 1834), Au. obscuripes, Sycophila biguttata (Swederus, 1795) and Eur. brunniventris in Málaga and T. notatus, Eupelmus azureus Ratzeburg, 1844 (=Eupelmus spongipartus Förster, 1860 (Al Khatib et al., Reference Al Khatib, Fusu, Cruaud, Gibson, Borowiec, Rasplus, Ris and Delvare2014)), Mesopolobus sericeus (Förster, 1770), and Anastatus sp. in Galicia, with the addition of the cynipid inquiline (Synergini) Synergus facialis Hartig, 1840.
In both cases, tests show that the system nesting temperature was different from the mean nesting temperature generated at random (Galicia 17.26° vs. 49.31°(±7.72°) and Málaga 27.68° vs. 46.19° (±8.33°), respectively; Monte Carlo-derived bootstrap results: Galicia: P < 0.001 and Málaga: P = 0.01). The system nesting temperatures were colder than those generated at random, confirming the presence of a pattern that can be studied and analysed in detail.
Discussion
Cynipid inquiline species
Together with identified species from previously reported D. kuriphilus community surveys (table 3), the total number of native parasitoids associated with this alien gall wasp in Spain is 34 species (Jara-Chiquito et al., Reference Jara-Chiquito, Heras and Pujade-Villar2016, Reference Jara-Chiquito, Askew and Pujade-Villar2020; Pérez-Otero et al., Reference Pérez-Otero, Crespo and Mansilla2017), in addition to one inquiline Cynipidae species.
This cynipid inquiline species, Syn. facialis, appeared in the D. kuriphilus community of Galicia. This is the second record in Europe of an inquiline species recruited by D. kuriphilus; the first came from Greece, where a new species of Saphonecrus Dalla Torre and Kieffer was described by Melika et al. (Reference Melika, Memtsas, Nicholls and Avtzis2018). Prior to these records, only one inquiline species, Synergus castaneus Pujade-Villar, Bernardo and Viggiani, 2013, was recorded from cynipid galls on Castanea in China, although these were not galls of D. kuriphilus (Bernardo et al., Reference Bernardo, Iodice, Sasso, Tutore, Cascone and Guerrieri2013). We found Syn. facialis in a single locality (Lousada), and it should be emphasized that we failed to rear additional individuals either in the second year of the sampling campaigns at this locality or at other collection sites in Galicia. Given that Syn. facialis is one of the cynipid inquiline species associated with the widest range of host galls (Nieves-Aldrey, Reference Nieves-Aldrey2001; Askew et al., Reference Askew, Melika, Pujade-Villar, Schönrogge, Stone and Nieves-Aldrey2013), it is quite possible that its rearing from D. kuriphilus represents only an incidental presence and that this species is not established as a stable component of the associated community of this invasive species in Galicia.
Parasitoid overwintering sub-community
While most of the recruited parasitoids by D. kuriphilus (table 2) exhibit generalist behaviour and phenologies with two or more emergence dates, we found four species with similar life cycles to that of T. sinensis in Málaga: T. notatus, Au. bicolor, Au. obscuripes and Au. balani (fig. 4). In contrast to the generalist species, these four parasitoids are specialist species exhibiting univoltine life cycles, and they are more synchronized with the life cycle of D. kuriphilus, attacking the early spring stage of the host gall. For this reason, these species might regulate D. kuriphilus more efficiently than other native parasitoids and may represent a possible alternative as a biocontrol agent to the alien T. sinensis. However, it should be emphasized that the low numbers of these species, at least in the first years of recruitment, suggest that these native chalcids may not be effective biological control agents of D. kuriphilus (Quacchia et al., Reference Quacchia, Ferracini, Nicholls, Piazza, Saladini, Tota, Melika and Alma2012). Because of their possible potential to control D. kuriphilus, relevant biological information is discussed below.
T. notatus is a native species that is morphologically closest to T. sinensis and it has a univoltine life cycle synchronized with the phenology of its host galls. Given this closeness to T. sinensis, T. notatus could be a candidate for hybridization with T. sinensis (Pogolotti et al., Reference Pogolotti, Cuesta-Porta, Pujade-Villar and Ferracini2018). T. notatus has been reared in Europe from a limited number of galls of the genera Andricus, Neuroterus and Plagiotrochus (Askew et al., Reference Askew, Melika, Pujade-Villar, Schönrogge, Stone and Nieves-Aldrey2013, but see also Nieves-Aldrey, Reference Nieves-Aldrey2001 and Gómez et al., Reference Gómez, Hernández-Nieves, Garrido Torres, Askew and Nieves-Aldrey2006, for Spanish records), but its most common hosts in Spain are galls of the sexual generations of Andricus curvator Hartig, 1840 (in the United Kingdom this is the only known host) and Plagiotrochus australis (Mayr, 1882). Since these two species (T. notatus and T. sinensis) emerge from the galls at the same time and given their morphological similarity, much care is necessary to avoid confusing them.
From the sampling sites in Spain (table 2), 23 individual T. notatus (10 males and 13 females) were identified from three sites in the Málaga region: El Juanar, Júzcar and Puerto Ojén. One female of T. notatus was also found in Riós in the Mediterranean Region of Galicia. In addition, one male and four females were reared from galls collected at one of our sampling sites in Catalonia (Sant Hilari). In all cases, T. sinensis was also reared from the same D. kuriphilus gall samples. Our data indicate similar establishment rates for the two species in at least one sampling site in the Málaga region (Puerto Ojén). At this site, we reared 18 T. notatus and 15 T. sinensis individuals, suggesting that, given their similar life cycle and taxonomic closeness, the native T. notatus might supplement the biocontrol effect of T. sinensis, at least at this site.
Six females of Au. bicolor were reared from samples collected in El Juanar (Málaga). This is a specialist parasitoid species only associated with galls of Plagiotrochus species on Mediterranean evergreen Quercus (Q. ilex and Q. coccifera). It is recorded only from Spain and Jordan where it is associated mainly with Plagiotrochus quercusilicis (Fabricius, 1798) and P. australis (Nieves-Aldrey, Reference Nieves-Aldrey1983; Pujade-Villar and Ros-Farré, Reference Pujade-Villar and Ros-Farré1998; Gómez et al., Reference Gómez, Hernández-Nieves, Garrido Torres, Askew and Nieves-Aldrey2006; Rizzo and Askew, Reference Rizzo and Askew2008; Askew et al., Reference Askew, Melika, Pujade-Villar, Schönrogge, Stone and Nieves-Aldrey2013).
Ten females of Au. balani were recovered from galls collected in El Juanar and Puerto Ojén (Málaga). Previously this species was known only from the Catalonia region (northeast Spain), where it has been reared from galls of the sexual generation of Pseudoneuroterus saliens (Kollar, 1857) (Pujade-Villar, Reference Pujade-Villar1991), and from Tunisia, where it has been reared from Synophrus olivieri Kieffer, 1898 (Pujade-Villar et al., Reference Pujade-Villar, Askew, Grami and Jamâa2010), both cynipid hosts being associated with cork oak (Q. suber). This is its first record from the parasitoid community of D. kuriphilus. It should be noted that Au. balani has been recovered from D. kuriphilus galls at a site in southern Spain where chestnut species share habitat with natural Mediterranean forests of evergreen oaks such as Q. suber and Q. ilex, among other species of Quercus. These evergreen Quercus are the host plants of the cynipid species recorded as hosts of the above-mentioned Aulogymnus species. This is the first time that they have been recorded as parasitoids of D. kuriphilus.
Two females of Au. obscuripes were reared in March from D. kuriphilus galls collected in winter in Puerto Ojén (Málaga). This species has been recorded as a parasitoid of ten oak gall wasp species of the genera Andricus, Chilaspis, Cynips and Pseudoneuroterus. However, the more common host species galls are those of the sexual generation of An. quercuscalicis (Burgsdorf, 1783) (Askew et al., Reference Askew, Melika, Pujade-Villar, Schönrogge, Stone and Nieves-Aldrey2013). There is only one previously available record of this Aulogymnus on the Iberian Peninsula; it was identified as a parasitoid in sexual galls of Andricus pseudoinflator Tavares, 1902 in the Catalonia region (Pujade-Villar, Reference Pujade-Villar1991). This is the first time that Au. obscuripes has been recorded in the native parasitoid community of D. kuriphilus.
The four species mentioned above belong to a native parasitoid sub-community that can be referred to as the ‘overwintering’ parasitoid community, in contrast to the so-called ‘summer’ parasitoid community referred to in most other published studies (Quacchia et al., Reference Quacchia, Ferracini, Nicholls, Piazza, Saladini, Tota, Melika and Alma2012; Kos et al., Reference Kos, Kriston and Melika2015). The Aulogymnus species recorded in this study have historically been associated with Q. suber cynipid gall inducers (Askew et al., Reference Askew, Melika, Pujade-Villar, Schönrogge, Stone and Nieves-Aldrey2013), proximity or the presence of cork oaks probably dictating whether they enter D. kuriphilus communities. In Eurosiberian Galicia, Q. suber is scarce, preferring more thermophilic areas such as the Mediterranean (Gil and Varela, Reference Gil and Varela2008), and the mentioned Aulogymnus species have not been found in the D. kuriphilus community. A similar case is provided by T. notatus, a species that in Spain is predominantly associated with galls of species of Plagiotrochus on Q. ilex, an evergreen oak that is very scarce in the predominantly Eurosiberian Galicia. The different roles of T. notatus in the two regions are noteworthy (fig. 3), as this parasitoid is a potentially effective species for the control of D. kuriphilus in Málaga, whereas it may be an accessory or marginal species in Galicia.
Studies addressing the overwintering D. kuriphilus parasitoid sub-community are uncommon compared with works focused on the summer sub-community (Aebi et al., Reference Aebi, Schönrogge, Melika, Alma, Bosio, Quacchia, Picciau, Abe, Moriya, Yara, Seljak, Stone, Ozaki, Yukawa, Ohgushi and Price2006; Matošević and Melika, Reference Matošević and Melika2013; Jara-Chiquito et al., Reference Jara-Chiquito, Heras and Pujade-Villar2016, Reference Jara-Chiquito, Askew and Pujade-Villar2020; Bonsignore and Bernardo, Reference Bonsignore and Bernardo2018). The summer community has higher species richness, is easier and more accessible to study and is related to the collection and observation of green and fresh D. kuriphilus galls.
The role of T. sinensis in the Iberian communities
As T. sinensis was found to be a well-established, dominant species in the Málaga community (fig. 3) and was the most abundant reared parasitoid, it is highly probable that this species can also influence the native parasitoid fauna in the D. kuriphilus community. This effect was not quantified in this study; however, it has been measured in other works, such as that of Ferracini et al. (Reference Ferracini, Bertolino, Bernardo, Bonsignore, Faccoli, Ferrari, Lupi, Maini, Mazzon, Nugnes, Rocco, Santi and Tavella2018) or Bonsignore and Bernardo (Reference Bonsignore and Bernardo2018). In these studies, the authors found a negative relationship between the entry of T. sinensis into the community and the species richness of native parasitoids in D. kuriphilus galls. It is possible that highly abundant T. sinensis, linked to the overwintering sub-community, will parasitize galls in the early stages of formation, and thus decrease the number of available non-parasitized galls for the summer sub-community. However, the summer sub-community is composed mainly of polyphagous parasitoids that can act as hyperparasitoids, such as Eup. urozonus and Eur. brunniventris, and these would not be affected. Considering the abundance of native parasitoids and T. sinensis that was found in this study (table 2), no negative direct effect of this foreign parasitoid on the indigenous fauna has been detected. Considering the region of Galicia and the relatively low number of individuals in the T. sinensis population, it does not seem that T. sinensis can have an effect as strong as indicated by Ferracini et al. (Reference Ferracini, Bertolino, Bernardo, Bonsignore, Faccoli, Ferrari, Lupi, Maini, Mazzon, Nugnes, Rocco, Santi and Tavella2018). However, in the case of the Málaga region, given the relatively large and growing population of T. sinensis in proportion to the native parasitoids, it is possible that this foreign parasitoid could greatly affect the native species if it were to invade the native oak cynipid communities in the future. These differences could be considered an effect and bias of the sampling, since less galls were collected in Galicia than in Malaga. However, the species accumulation curves (table 4) support that the samplings by region are correctly carried out and that both areas are comparable.
No specimens of T. sinensis were collected in the sampling campaigns of 2017–2018 in Galicia, and only 19 individuals were collected in 2018–2019, indicating a low level of establishment in this territory, confirming preliminary data from Nieves-Aldrey et al. (Reference Nieves-Aldrey, Gil-Tapetado, Gavira, Boyero, Polidori, Lombardero, Blanco, Rey Del Castillo, Rodríguez-Rojo, Vela and Wong2019). However, in later years, the number of T. sinensis will probably increase, as has occurred in other countries such as Italy (Quacchia et al., Reference Quacchia, Moriya, Bosio, Scapin and Alma2008).
D. kuriphilus communities' composition and structure
Considering the nestedness analysis (fig. 3) and the community composition together with the theory of island biogeography (MacArthur and Wilson, Reference MacArthur and Wilson2001; Lomolino et al., Reference Lomolino, Riddle and Whittaker2016), D. kuriphilus communities can be considered to act as sink or ‘insular communities’ including fewer species than the source or ‘mainland communities’. These D. kuriphilus communities in their early stages are composed almost exclusively of generalist species (table 2), exhibiting high plasticity and adaptability, and a greater colonization potential (Cornell and Hawkins, Reference Cornell and Hawkins1993). The mature ‘mainland communities’ from which the D. kuriphilus communities would be nourished are the native cynipid communities found on Quercus species, which harbour the native parasitoid fauna (Askew and Shaw, Reference Askew, Shaw, Waage and Greathead1986; Nieves-Aldrey, Reference Nieves-Aldrey2001) and constitute a relatively well-organized and structured biological system (Askew, Reference Askew, Vinson and Price1975). Thus, generalist species from the mature Quercus cynipid communities are the first species to conform to a new biological community (‘insular communities’ in which D. kuriphilus enters) since they exhibit a wider ecological plasticity, greater adaptability and an easier fit to a newly available niche (Cornell and Hawkins, Reference Cornell and Hawkins1993), with a greater host diversity, and adaptability to more potential hosts (Askew, Reference Askew, Vinson and Price1975).
Theoretically, as there are no apparently known physical barriers between ‘insular’ and ‘mainland communities’ (Castanea and Quercus trees form mixed forests in Málaga and Galicia regions), the colonization, recruitment of parasitoids and ecological succession in these two communities, both being initiated in 2014 with the arrival of D. kuriphilus, should be rapid (Ferracini et al., Reference Ferracini, Bertolino, Bernardo, Bonsignore, Faccoli, Ferrari, Lupi, Maini, Mazzon, Nugnes, Rocco, Santi and Tavella2018). The recruitment to the communities of a specialist species such as T. notatus (table 2) during the study period, a species not detected in previous years could represent a step in the development of the biological community to a state of greater maturity. In Málaga, T. notatus was detected in 2017–2018 in three localities together with the three species of the genus Aulogymnus, while in the Galician communities only one individual of T. notatus was found at a single locality in 2018–2019. This is the first difference in D. kuriphilus community composition that was identified between Málaga and Galicia; ecological succession in the first region may a priori be more advanced than in the second.
The composition differences in beta diversity between the Málaga and Galicia D. kuriphilus communities showed a similarity of Jaccard = 0.65 and Sorensen = 0.79, indicating high similarity between them. The composition differences between the Galicia and Málaga D. kuriphilus communities were probably due to the different distributions and abundances of the native chalcid species on the Iberian Peninsula. For example, Mesopolobus species appear to be more abundant in Málaga than in the Galicia region (table 2), appearing as dominant species in the community of Málaga. This situation is especially noticeable for M. tibialis, which is a dominant species in the Málaga region but nearly an accessory species in Galicia. The species M. lichtensteini and Mesopolobus mediterraneus (Mayr, 1903) seem to represent a peculiarity of the Iberian D. kuriphilus communities (tables 2 and 3), as they are scarce or absent in other published European surveys of recruited parasitoids (Aebi et al., Reference Aebi, Schönrogge, Melika, Quacchia, Alma and Stone2007; Matošević and Melika, Reference Matošević and Melika2013; Panzavolta et al., Reference Panzavolta, Bernardo, Bracalini, Cascone, Croci, Gebiola, Iodice, Tiberi and Guerrieri2013; Quacchia et al., Reference Quacchia, Moriya and Bosio2013; Francati et al., Reference Francati, Alma, Ferracini, Pollini and Dindo2015; Kos et al., Reference Kos, Kriston and Melika2015; Bonsignore and Bernardo, Reference Bonsignore and Bernardo2018). The presence and abundance of reared M. lichtensteini and M. mediterraneus in the Spanish D. kuriphilus communities (95 and 30, respectively, in this study (table 2) and 184 and 86 for the whole Iberian Peninsula) (table 3) could be due to their abundance generally on the Iberian Peninsula, contrasting with just a few records from other European countries, especially in northern Europe (Askew, Reference Askew2013; Askew et al., Reference Askew, Melika, Pujade-Villar, Schönrogge, Stone and Nieves-Aldrey2013). This finding could reinforce the idea that these species exhibit an Iberian distribution affinity.
In contrast, O. pomaceus appears to be dominant in the community of Galicia, replacing Mesopolobus species (fig. 3b). In fact, O. pomaceus occurs as one of the few effective or even accessory species in the Málaga community (fig. 3a) where it is rare (two individuals) with a mean occupancy percentage (45–55) and appearing in only two localities (Júzcar and Pujerra). The scarcity of O. pomaceus in the Málaga region (table 2) is difficult to explain, considering a large number of potential cynipid native hosts available here. However, as O. pomaceus is a cryptic species complex (Goméz et al., Reference Goméz, Hernández-Nieves, Gayubo and Nieves-Aldrey2017), it is possible that there are two different biological entities from this complex, in Galicia and Málaga, and that these have different roles in the native cynipid communities and, by extension, their recruitment by the new host D. kuriphilus. This may also be the case for other cryptic species complexes, such as Torymus flavipes (Walker, 1833) (Kaartinen et al., Reference Kaartinen, Stone, Hearn, Lohse and Roslin2010), Eup. urozonus (Askew and Nieves-Aldrey, Reference Askew and Nieves-Aldrey2000; Al Khatib et al., Reference Al Khatib, Fusu, Cruaud, Gibson, Borowiec, Rasplus, Ris and Delvare2014) and Eur. brunniventris (Lotfalizadeh et al., Reference Lotfalizadeh, Delvare and Rasplus2007). Together with Eurytoma pistaciae Rondani 1877 (=Eurytoma setigera Mayr, 1878), these species are reported to have multivoltine cycles that could actually mask different biological units with the same or very similar morphology. Eur. pistaciae also seems to represent a peculiarity of the Iberian D. kuriphilus communities because, like M. lichtensteini and M. mediterraneus, more reared individuals were found in Spain (table 3) than were reported in previous papers on D. kuriphilus parasitoids in Europe. However, unlike the previously noted Mesopolobus species, Eur. pistaciae seems to exhibit a primarily circum-Mediterranean distribution (Askew et al., Reference Askew, Melika, Pujade-Villar, Schönrogge, Stone and Nieves-Aldrey2013; Delvare et al., Reference Delvare, Stojanova, Benoit, Lecomte and Askew2019).
The nestedness diagram (fig. 3) and sS indices (table 5) show that the D. kuriphilus communities in Málaga and Galicia have different structures. The Málaga community exhibits the highest q 0 sS index (total richness of species), although the Galicia region has the highest q 1 and q 2sS indices (effective and dominant richness). Additionally, the relative abundance is higher in this region than in Málaga. The higher q 0 diversity in Málaga might be explained by the proximity of the D. kuriphilus community to a more diverse community of native cynipids and their Quercus hosts, given that 5–7 Quercus species are present at most collection sites in Malaga, while 2–3 were the typical numbers in the Galicia communities (Stone et al., Reference Stone, Lohse, Nicholls, Fuentes-Utrilla, Sinclair, Schönrogge, Csóka, Melika, Nieves-Aldrey, Pujade-Villar, Tavakoli, Askew and Hickerson2012; Ferracini et al., Reference Ferracini, Bertolino, Bernardo, Bonsignore, Faccoli, Ferrari, Lupi, Maini, Mazzon, Nugnes, Rocco, Santi and Tavella2018). Overall, the region having the most disparate cynipid tree hosts was Eurosiberian Galicia, with a predominance of Q. robur and scarcity or the absence of Q. ilex and Q. suber, which is present in both Mediterranean Galicia and the Málaga region. The only cynipids associated with these Quercus species on the Iberian Peninsula are Plagiotrochus or the sexual generations of heteroecic Andricus (associated with Q. suber). In Central Europe, they are associated with Quercus cerris L. (Nieves-Aldrey, Reference Nieves-Aldrey2001). The co-occurrence of Q. suber and Quercus species of the Quercus section could possibly contribute to the success of heteroecic species of Andricus, making the rich parasitoid communities associated with native cynipids more stable and favouring the recruitment of more parasitoids by the D. kuriphilus community. The native cynipids, Quercus hosts and parasitoid diversity of native gall wasps are three factors that should be studied to better understand these ‘insular communities’ derived from components of the ‘mainland community’. In addition, the distances between the donor and sink communities and, more specifically, between the native cynipids and D. kuriphilus galls, are likely to also modulate community composition and species abundance associated with this invasive species.
Accessory species in D. kuriphilus communities (q 0–q 1, fig. 3) are probably incidental species that are irregularly associated with D. kuriphilus galls and play no important role in these communities. These accessory species are more abundant in the Málaga region than in Galicia, probably due to the high global parasitoid richness in the former region, which is also related to the high diversity of Quercus species, which can harbour more diverse cynipid hosts.
The results of this study can be compared with previously published studies from Spain (table 3). For example, the most abundant species in Catalonia (data of 2013–2014) were B. dorsalis and T. flavipes, and these species played an important, though not dominant, role in the communities of Galicia and Málaga. The scarcity of B. dorsalis in the Galicia region was also indicated by Pérez-Otero et al. (Reference Pérez-Otero, Crespo and Mansilla2017). However, T. flavipes and B. dorsalis are among the most abundant species in the D. kuriphilus parasitoid community in other European territories (Aebi et al., Reference Aebi, Schönrogge, Melika, Alma, Bosio, Quacchia, Picciau, Abe, Moriya, Yara, Seljak, Stone, Ozaki, Yukawa, Ohgushi and Price2006; Quacchia et al., Reference Quacchia, Ferracini, Nicholls, Piazza, Saladini, Tota, Melika and Alma2012; Matošević and Melika, Reference Matošević and Melika2013; Bonsignore and Bernardo, Reference Bonsignore and Bernardo2018). It is possible that the roles of these parasitoids are very plastic and vary over time as these biological communities evolve into a more mature state. After all, these community analyses provide a current record of the state of these D. kuriphilus parasitoid communities which will undoubtedly change in the future. The realization of biological community studies and their monitoring over the years can show how de novo communities, such as the D. kuriphilus communities on the Iberian Peninsula evolve. Nevertheless, it will probably take many years for ecological succession to attain maturation. In fact, following the estimate of Cornell and Hawkins (Reference Cornell and Hawkins1993), it will take more than 150 years for a de novo community to reach its equilibrium; this is too long to wait for a solution to the problem imposed by D. kuriphilus on the Iberian Peninsula and in other parts of the world.
Acknowledgements
This study was financed by an Encomienda de Gestión from MAPAMA to Agencia Estatal CSIC, 16MNES003 and by research project from MEC, AGL2016-76262-R (AEI/FEDER, UE) awarded to JLNA. We thank María J. Lombardero and Juan R. Boyero for their help and collaboration with this project. Special thanks to Oscar N. Gavira and M. Pilar Rodríguez-Rojo for assistance in sampling campaigns and field work.