In line with the global predictions for the prevalence of Alzheimer's disease (AD), Australia declared AD as the ninth National health priority in 2012. Alzheimer's is a complex disease that progresses over many years, such as diabetes, heart disease and other chronic conditions. The gradual accumulation of the pathology of cerebral extracellular AD known as amyloid, which is mostly composed of aggregated amyloid-β (Aβ) peptides( Reference Chetelat, Villemagne and Bourgeat 1 ), as well as the accumulation of intracellular neurofibrillary tangles, appears to start up to 17–20 years before a clinically observable disease( Reference Villain, Chetelat and Grassiot 2 ). A number of factors may increase or decrease an individual's chances of developing the disease. These risk factors include age, genetics, environment, lifestyle and metabolic diseases.
Diet may play an important role in preventing AD. As many studies have linked AD risk to diet-modifiable conditions such as type 2 diabetes, hypertension and CVD, dietary approaches to AD prevention involving palatable, low risk, inexpensive substances are attracting great attention, as a method to ameliorating deficits concomitant with ageing and neurodegeneration. In particular, recent literature has suggested that the use of coconut oil (extra virgin/virgin), coconut water and coconut cream may have significant positive effects on the lowering of plasma cholesterol, blood pressure (BP) control and blood glucose levels, all of which are risk factors associated with AD. Coconut has also been identified as a potential cognitive strengthener( Reference Marina, Che Man and Nazimah 3 , Reference Gopala, Gaurav and Ajit 4 ) associated with AD. The present review reported the evidence for coconut oil consumption, with a particular emphasis on virgin coconut oil (VCO), outlining the potential risks and benefits in relation to AD prevention and/or management.
The scientific name for coconut is Cocos nucifera, and the plant is a member of the Arecaceae family( Reference Lopes and Larkins 5 – 10 ). Among the components of coconut, coconut oil is of the most interest related to human health. Of note, coconut oil is principally composed of SFA (about 92 %), with 62–70 % being medium-chain TAG (MCT)( Reference Gopala, Gaurav and Ajit 4 , Reference Bach and Babayan 11 , Reference Chandrashekar, Lokesh and Gopala 12 ), making coconut oil unique among dietary fats. A few clinical trials and animal studies using a formulation of MCT have reported significant improvement of cognition in AD patients. While research on Alzheimer's and MCT is still in its infancy, the science behind MCT is that MCT can be rapidly metabolised to induce metabolic ketosis and ketogenic, which could be employed as a therapy for a variety of brain disorders, including epilepsy and neurodegeneration. Anecdotes via the media and word-of-mouth have promoted great interest in the action of ketones and, thus, coconut oil.
Recent studies have investigated the possibility of using trans-zeatin and phyto-oestrogen and other sex hormone-like substances present in coconut water and young coconut juice in reducing the risk of AD( Reference Radenahmad, Vongvatcharanon and Withyachumnarnkul 13 , Reference Radenahmad, Saleh and Sawangjaroen 14 ). In contrast, experimental studies have suggested that coconut/coconut cream consumption can cause hyperlipidaemia and atherosclerosis, which are risk factors for AD. In contrast, several studies have reported that hyperlipidaemia and heart diseases are uncommon among high coconut consuming populations( Reference Kumar 15 , Reference Lindeberg and Lundh 16 ).
In view of the interest in the potential of coconut oil, coconut water and coconut cream as a dietary supplement that could ameliorate the symptoms of neurodegeneration, we analysed the literature to understand the influence of coconut on the pathology of AD and risk factors for AD. Adding complexity to this discussion is the various forms of coconut available, and also the method of extraction used to produce the end product.
Coconut oil
Coconut oil is extracted by either hot or cold pressed techniques, and the method used is reported to influence the quality and grade of the oil, although agreement on which method is best has not been achieved. Both wet and dry methods are used, and some approaches also involve solvents for the final extraction, if using coconut expeller cake( Reference Gopala, Gaurav and Ajit 4 ). VCO, manufactured using controlled temperature (hot or cold) methods are thought to be the most effective methods if aiming to retain the highest levels of biologically active components such as tocotrienols, squalene (hydrocarbon, important for animal steroid formation), tocopherols and sterols (phytosterols)( Reference Marina, Che Man and Nazimah 3 ). This is in contrast to copra oil (derived from the dried coconut meat or kernel) that is processed with no temperature control( Reference Marina, Che Man and Nazimah 3 , Reference Villarino, Dy and Lizada 17 ). VCO is natural, chemically unrefined and considered safe for human consumption( Reference Gopala, Gaurav and Ajit 4 ). Thus, in addition to being used as a cooking oil, VCO can also be considered as a functional food supplement. The total phenolic content of VCO (7·78–29·18 mg gallic acid equivalents/100 g oil) is significantly higher than that of refined coconut oil (6·14 mg gallic acid equivalents/100 g oil)( Reference Gopala, Gaurav and Ajit 4 , Reference Dauqan, Sani and Abdullah 18 ). However, there is no significant difference in fatty acid content among VCO, Copra and refined coconut oil, all containing 92 % SFA, 6 % MUFA and 2 % PUFA. However, VCO has shown greater beneficial effects than copra oil in lowering lipid levels in serum and tissues and in reducing LDL oxidation by physiological oxidants. This property of VCO may be attributed to the biologically active polyphenol components present in the oil( Reference Nevin and Rajamohan 19 ) .
Not surprisingly, the high levels of saturated fat have generally deterred those who are more health conscious from using coconut oils, cream or milk. Furthermore, low-fat diets have been considered to be the best approach to reduce the risk of AD, in particular the Mediterranean diet( Reference Scarmeas, Stern and Tang 20 , Reference Gu, Luchsinger and Stern 21 ). Therefore, promoting coconut oil as a food would appear counter-intuitive. However, closer scrutiny of the chemical properties, digestion and uptake may suggest that this concern may not be well founded. Coconut oil is rich in medium-chain fatty acids (MCFA), which are metabolised differently to the long-chain fatty acids (LCFA) commonly found in human diets. In addition, coconut oil offers anti-ageing and antioxidant properties( Reference Marina, Man and Nazimah 22 , Reference Müller, Lindman and Blomfeldt 23 ). Coconut oil in food has a long history, and is very popular in South Asia and has a prominent place in Ayurvedic medicine.
Coconut oil consists mostly of medium-chain fatty acids
Coconut oil is principally composed of SFA (about 92 %), with 62–70 % being MCFA( Reference Gopala, Gaurav and Ajit 4 , Reference Bach and Babayan 11 , Reference Chandrashekar, Lokesh and Gopala 12 ) (Table 1), making coconut oil unique among dietary fats( Reference Marina, Che Man and Nazimah 3 , Reference Bach and Babayan 11 ). The difference between MCFA and LCFA is the length of the fatty acid carbon chain. MCFA have a chain length of six to twelve carbons( Reference Marina, Che Man and Nazimah 3 , Reference Traul, Driedger and Ingle 24 ) (Table 1), whereas LCFA contain fourteen or more carbons( Reference Bach and Babayan 11 , Reference Traul, Driedger and Ingle 24 ). The length of the carbon chain determines the physical and chemical properties of the fats as well as their metabolism in the human body( Reference Traul, Driedger and Ingle 24 ). Soya oil contains 60 % PUFA, 24 % MUFA and 16 % SFA( Reference Warner 25 ). In contrast, palm oil contains 50 % MUFA and 50 % SFA. This high level of PUFA of soya oil can improve the blood lipid profile status( Reference Adam, Das and Faizah 26 ). In addition, with its high content of tocopherols, soya oil is known to exhibit various antioxidant actions against lipid peroxidation.
Table 1 Fatty acid composition of coconut oil, showing percentage of total fat
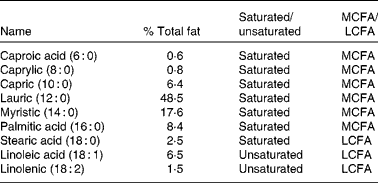
MCFA, medium-chain fatty acids; LCFA, long-chain fatty acids.
Metabolism of medium-chain fatty acids
MCFA are broken down almost immediately by enzymes in the saliva and gastric juices, without the need for pancreatic fat-digesting enzymes( Reference Ruppin and Middleton 27 ); furthermore, this process involves relatively moderate energy consumption. Therefore, the metabolism of coconut oil is significantly different from that of other fatty acids commonly found in the diet( Reference Dauqan, Sani and Abdullah 18 ).
Medium-chain fatty acid absorption
In the case of most other fatty acids and cholesterol, the intestines play a major role in absorption( Reference Masson, Plat and Mensink 28 ); yet unfortunately, pancreatic function declines with age, and therefore malabsorption problems can occur in patients who suffer from digestive and metabolic conditions. In other words, as the pancreatic output of digestive enzymes reduces, the efficiency of the small intestine in the absorption of nutrients diminishes( Reference Ruppin and Middleton 27 – Reference Holt 30 ). This is important, as vitamin and mineral deficiencies are recognised as a global health issue( Reference Mandelbaum-Schmid 31 ), and age-related changes and select disease processes exacerbate this problem( Reference Mishkin, Stein and Gatmaitan 29 , Reference McCann and Ames 32 , Reference Ockner, Manning and Poppenhausen 33 ). As weight loss and malnutrition are recognised as frequent companions and contributors to AD, dietary supplementation with coconut oil may help prevent weight loss and increase the intake of certain vitamins and minerals.
The concern that coconut oil may increase plasma lipid levels and adversely affect health is a point of contention. MCFA are partially hydrolysed from dietary TAG by lingual lipase in the stomach and completely digested by pancreatic lipase within the intestinal lumen( Reference Ruppin and Middleton 27 ). Therefore, MCFA are absorbed directly from the intestines into the portal vein and sent straight to the liver( Reference Ruppin and Middleton 27 , Reference Ockner, Manning and Poppenhausen 33 ). Unlike MCFA, other fats such as cholesterol, as well as saturated fat, monounsaturated fat and polyunsaturated fat containing LCFA, combine with proteins and form lipoproteins( Reference Traul, Driedger and Ingle 24 , Reference Ruppin and Middleton 27 , Reference Ockner, Manning and Poppenhausen 33 , Reference Tholstrup, Ehnholm and Jauhiainen 34 ). These lipoproteins enter the bloodstream via the lymphatic system, thus mostly bypassing the liver( Reference Ruppin and Middleton 27 , Reference Ockner, Manning and Poppenhausen 33 ). As lipoproteins circulate in the blood, their fatty components are dispersed to tissues( Reference Tsuji, Kasai and Takeuchi 35 ), therefore contributing to the accumulation of fat in such body tissues, as part of normal fat storage. However, in the process, some of these fats congeal within the artery walls, increasing the risk of hypertension and adding to the cardiovascular risk factors, and both known to increase AD risk( Reference Gu, Luchsinger and Stern 21 ). In contrast to LCFA that are easily esterified and bind strongly to fatty acid binding proteins( Reference Valdivieso 36 , Reference Ippagunta, Hadenfeldt and Miner 37 ), MCFA are not easily esterified and resist binding. Thus, MCFA are less likely to contribute to such fat deposits, and thus have reduced impact on the cardiovascular system, including BP( Reference Tholstrup, Ehnholm and Jauhiainen 34 , Reference Tsuji, Kasai and Takeuchi 35 , Reference Agnew and Holdsworth 38 , Reference Tantibhedhyangkul and Hashim 39 ). However, recent research with VCO has demonstrated that repeatedly heated VCO causes an elevation in BP. BP elevation has been associated with a significant increase in the inflammatory biomarkers (vascular cell adhesion molecule-1, intercellular adhesion molecule-1 and C-reactive protein), thromboxane A2 and a significant reduction in the plasma PGI2 level( Reference Hamsi, Othman and Das 40 ). Repeatedly heated soya oil and palm oil also elevated BP( Reference Leong, Najib and Das 41 , Reference Adam, Das and Soelaiman 42 ).
Medium-chain fatty acid breakdown
Similar to the absorption differences mentioned above, the human body metabolises MCFA and LCFA via different pathways( Reference Ockner, Manning and Poppenhausen 33 , Reference Paul and Concetta 43 ). SCFA are transported in the blood as NEFA, while longer-chain NEFA are combined with albumin( Reference Bach and Babayan 11 ). The metabolism of fatty acids is initiated on the outer mitochondrial membrane and is catalysed by acyl-CoA synthetase( Reference Paul and Concetta 43 ) as shown in Fig. 1. This step is required partly to enable the transport of the fatty acids into the mitochondrial matrix. First, the fatty acid forms an acyl-adenylate; then while still tightly bound to the enzyme, acyl-adenylate is converted to acyl-CoA (medium-chain acyl-CoA or long-chain acyl-CoA) and AMP( Reference Paul and Concetta 43 ). Acyl-CoA can then be transported into the mitochondria using different pathways depending on the fatty acid chain length( Reference Paul and Concetta 43 ). Long-chain acyl-CoA molecules conjugate with carnitine (l-3-hydroxy-4-aminobutyrobetaine or l-3-hydroxy-4-N-trimethylaminobutanoic acid, and its acyl-esters (acylcarnitines)( Reference Paul and Concetta 43 , Reference Hoppel 44 ) to form acylcarnitine, and this reaction is catalysed by carnitine acyltransferase I( Reference Paul and Concetta 43 ). In contrast, MCFA enter the mitochondria independently of the carnitine transport system( Reference Papamandjaris, Macdougall and Jones 45 ), and therefore do not depend on the activity of the carnitine acyltransferase-1 enzyme, as with LCFA( Reference Paul and Concetta 43 ). Medium-chain fatty acyl-CoA molecules easily transfer into the mitochondria and can then be converted into acetoacetate (AcAc) and β-hydroxybutyrate, mainly by medium-chain fatty acyl-CoA-dehydrogenase( Reference Papamandjaris, Macdougall and Jones 45 ). These two products can be metabolised further in the liver to produce CO2, H2O and energy( Reference Mishkin, Stein and Gatmaitan 29 , Reference Ockner, Manning and Poppenhausen 33 , Reference Papamandjaris, Macdougall and Jones 45 ).

Fig. 1 Formation of acyl-CoA.
Benefits of medium-chain fatty acids compared with long-chain fatty acids
The result of the quicker metabolic conversion of MCFA is that instead of being deposited as fat, the energy generated from MCFA is very competently converted into fuel for immediate use by organs and muscles. Furthermore, MCFA produce 34·7 kJ/g (8·3 kcal/g) ingested, whereas LCFA will produce 38·5 kJ/g (9·2 kcal/g) ingested( Reference Gopala, Gaurav and Ajit 4 ). Thus, MCFA provide about 10 % less energy than LCFA. Although the difference sounds insignificant, this is just one of the many advantages of MCFA, as it will reduce obesity to some degree, and obesity is an independent risk factor for hypertension, hyperlipidaemia and diabetes, which are, in turn, the risk factors associated with AD( Reference Dara, Jessica and Hannah 46 ).
Differences between LCFA and MCFA metabolism may also help in other more indirect ways in controlling obesity, and differences in the metabolism as well as the metabolic effects of LCFA and MCFA have been demonstrated in both animal and human studies( Reference St-Onge and Jones 47 – Reference Xue, Liu and Wang 49 ): for example, increases in postprandial energy expenditure (EE) as well as the attenuation of weight accretion have been demonstrated, after short- or longer-term MCFA consumption, and these are discussed below.
In early clinical studies, Flatt et al. ( Reference Flatt, Ravussin and Acheson 50 ) compared diets rich in either MCFA, LCFA or low in fats, and found that low-fat diets were most efficient for weight loss; however, they also found that MCFA-rich diets may be better than LCFA-rich diets; this was supported by Hill et al. ( Reference Hill, Peters and Yang 51 ) who reported that higher EE was achieved through MCFA intake over 7 d when consumed in liquid formulation. This study demonstrated that excess dietary energy as MCFA motivated thermogenesis to a higher degree than did excess energy as LCFA. This higher EE induced by MCFA is most likely due to increased metabolic rates and thermogenesis. In a trial involving six participants, Scalfi et al. ( Reference Scalfi, Coltorti and Contaldo 52 ) introduced meals containing 30 % fat, in the form of maize oil and animal fat, or MCFA oil (56 % octanoate and 40 % decanoate), to evaluate EE. They found that EE after consumption of MCFA (compared with LCFA) was 48 % greater in lean individuals, and 65 % greater in obese individuals. Dulloo et al. ( Reference Dulloo, Fathi and Mensi 53 ) compared the effects of low-to-moderate amounts of MCFA and LCFA consumption in eight healthy adult men. Subjects were given MCFA and LCFA (30 g total) at (g:g) ratios of 0:30, 5:25, 15:15 and 30:0, and their EE were measured. Increases in EE of 45, 135 and 265 kJ were reported following 5, 15 and 30 g of MCFA in the diet, respectively, suggesting an approach to altering body fat composition and metabolism. White et al. ( Reference White, Papamandjaris and Jones 54 ), however, cautioned that the anti-obesity effect of MCFA results could be transient, as they found that short-term feeding of MCFA-enriched diets increased temporary EE, yet with longer intake, this benefit was reduced. Encouragingly, however, a double-blind controlled trial in men and women (n 78) over a 12-week period demonstrated a greater reduction in body weight and fat following the daily ingestion of 60 g/d of MCFA compared with 60 g/d LCFA( Reference Tsuji, Kasai and Takeuchi 35 ), with other major dietary parameters not being significantly different. Furthermore, several studies have now shown that EE is higher when diets contain MCFA rather than LCFA; thus, MCFA are more conducive to weight loss( Reference St-Onge and Jones 47 , Reference Noguchi, Takeuchi and Kubota 55 – Reference Baba, Bracco and Hashim 58 ). The above studies are encouraging, yet may need to be repeated in larger cohorts to give the results further validation. However, a recent study in 2010 has concluded that there is no evidence that fatty acid chain length has an effect on the measures of appetite and food intake when assessed following a single high-fat test meal in lean participants. This study failed to observe any differences between SCFA (dairy fat), MCFA (coconut oil) and LCFA (beef tallow) when energy is held constant at a test meal( Reference Poppitt, Strik and MacGibbon 59 ). Hamsi et al. ( Reference Hamsi, Othman and Das 40 ) showed that heating of VCO repeatedly, which is a common practise in order to save the cost, could have detrimental effects on the body weight. This study demonstrated that rats fed with VCO, repeatedly heated one, five and ten times, resulted in higher weight gain than the non-heated oil-fed group. This finding is not unique to coconut oil, but is in line with earlier animal studies, which showed that heated palm oil and soya oil resulted in greater body weight gain compared with the control group( Reference Leong, Najib and Das 41 , Reference Adam, Das and Soelaiman 42 ).
PUFA play wide range of roles in cell metabolism, signalling and inflammation. Of the PUFA, very-long-chain EPA and DHA found principally in fish play key roles in metabolism and inflammation. Some studies have suggested that MCFA can enhance the positive effects of other dietary lipids such as PUFA. Conjugated linoleic acid, such as fish oil, is a popular dietary supplement marketed for its role in enhancing fat metabolism( Reference Li, Huang and Xie 60 ). Conjugated linoleic acid is purported to have several physiological functions, including appetite suppression, increased fat mobilisation and increased fatty acid oxidation( Reference Vemuri, Kelley and Mackey 61 ), and in one study( Reference Ippagunta, Hadenfeldt and Miner 37 ) of mice fed conjugated linoleic acid, it has been found that the addition of MCFA (through dietary coconut oil)is associated with improved lipolysis (breakdown of TAG into glycerol and NEFA) compared with diets containing conjugated linoleic acid supplemented with soya oil. However, discrepancies exist across publications; for example, a number of studies have linked coconut oil to higher levels of LDL( Reference Radenahmad, Vongvatcharanon and Withyachumnarnkul 13 , Reference Dauqan, Sani and Abdullah 18 , Reference Nevin and Rajamohan 19 ), higher risks for CVD( Reference Dauqan, Sani and Abdullah 18 , Reference Nevin and Rajamohan 19 ) and impairments in memory( Reference Kumar 15 , Reference Lindeberg and Lundh 16 , Reference Dauqan, Sani and Abdullah 18 , Reference Nevin and Rajamohan 19 ) as well as in hippocampus morphology( Reference Lindeberg and Lundh 16 , Reference Dauqan, Sani and Abdullah 18 ).
Medium-chain fatty acids can be converted to ketone bodies
MCT or MCFA can act as a non-carbohydrate fuel source by enhancing the formation of ketones or ketone bodies in the body which are AcAc, 3-β-hydroxybutyrate (3HB) and acetone( Reference Traul, Driedger and Ingle 24 ) (see Fig. 2). The first two molecules are used for energy production, whereas acetone is a breakdown product of AcAc. Fatty acids cannot pass the blood–brain barrier (BBB); thus, the human brain primarily depends on glucose. However, it can utilise alternative fuels such as monocarboxylic acids, lactate and ketones to maintain energy homeostasis( Reference Hasselbalch, Knudsen and Jakobsen 62 , Reference Page, Williamson and Yu 63 ), and ketone bodies are used extensively as an energy source during glucose deficiency (ketosis)( Reference Morris 64 , Reference Sumithran, Prendergas and Delbridge 65 ). AcAc and 3HB are short-chain (four-carbon) organic acids (ketone bodies) that can cross cell membranes freely( Reference Morris 64 ), and cross the BBB through proton-linked, monocarboxylic acid transporters( Reference Morris 64 ).

Fig. 2 Ketone bodies.
Ketone bodies are absorbed by cells and converted back to acetyl-CoA, which enters the citric acid cycle (Krebs cycle) and is oxidised in the mitochondria to provide ATP( Reference Sato, Yoshihiro and Keon 66 ) and also precursors of acetylcholine( Reference Hasselbalch, Knudsen and Jakobsen 62 ) in neurons. Alternatively, ketone bodies can be converted to acetyl-CoA in the brain for the purpose of synthesising LCFA( Reference Hasselbalch, Knudsen and Jakobsen 62 , Reference Morris 64 , Reference Serra, Casals and Asins 67 ).
Ketogenic diets
Diets that comprise very low carbohydrate levels, substantial amounts of protein and high fat levels have a capacity to result in the production of high levels of ketone bodies (3HB, AcAc and acetone) and are often known as ketogenic diets (KD)( Reference Freeman and Kossoff 68 ). A KD has been found to be one of the most effective therapies for drug-resistant epilepsy; it has also provided specific benefits in conditions such as GLUT protein I (GLUT-I) deficiency, pyruvate dehydrogenase deficiency, myoclonic astatic epilepsy (Doose syndrome), tuberous sclerosis complex, Rett syndrome and severe myoclonic epilepsy in infancy (Dravet syndrome)( Reference Kashiwaya, Takeshima and Mori 69 – Reference Imamura, Takeshima and Kashiwaya 74 ). Despite being used for many decades, the mechanism whereby a KD can reduce epilepsy is not understood. Recent research has suggested ketosis, reduced glucose, elevated fatty acid levels and enhanced bioenergetics reserves, as well as neuron-specific effects such as modulation of ATP-sensitive potassium channels, enhanced neurotransmission, increased brain-derived neurotrophic factor expression due to glycolytic restriction and reduced neuroinflammation may be involved.
Rats maintained on a KD display an altered influx of nutrients to the brain, due to the up-regulation of both ketone transporters and GLUT type 1( Reference Puchowicz, Xu and Sun 75 – Reference Patel, Pyzik and Turner 77 ). However, in early studies, it has also been found that the classic KD leads to a higher risk of atherosclerosis( Reference Klag, Ford and Mead 78 ), a condition known to increase the risk of AD. More recent studies have indicated that the fatty acid content of the KD influences this risk of atherosclerosis: the classic KD contains a 4:1 or 3:1 ratio (by weight) of fat to combined protein and carbohydrate( Reference Alexander, Rogovik and Ran 79 ), with most of this fat being LCFA. Later studies have found that altered KD that are rich in MCFA, sometimes known as the MCT-KD, are more nutritionally adequate than classic KD, and are still effective in treating epilepsy disorders yet reduce cardiac risk( Reference Liu, Williams and Basualdo-Hammond 80 , Reference Liu 81 ). The MCT-KD countenances more fruits and vegetables, more food choices and causes lesser incidence of kidney stones, hypoglycaemia, constipation, low bone density and growth retardation( Reference Liu 81 ).
The MCT-KD contains less fat overall, as it includes MCFA (from coconut oil) that can provide a greater amount of ketone bodies per gram of fat and thus allows more carbohydrate and protein in the diet, making the diet more palatable than the classic KD. KD rich in MCFA have significant effects on lowering the cholesterol:HDL ratio compared with the classic KD( Reference Liu, Williams and Basualdo-Hammond 80 ).
The use of glucose for energy is vital in the brain; yet, this system is impaired in AD, partly due to disruption of the insulin signalling mechanism( Reference Steen, Terry and Rivera 82 ). Low glucose utilisation has been demonstrated in many studies by fluoro-2-deoxy-d-glucose positron emission tomography imaging in AD subjects. Importantly, this has also been detected in elderly people who later develop AD( Reference Mosconi 83 ). In fact, the strikingly reduced expression in the central nervous system of genes encoding insulin, insulin like growth factor I (IGF-I) and insulin like growth factor II (IGF-II), as well as the insulin and IGF-I receptors, suggests that AD may represent a neuroendocrine disorder, which has been termed ‘Type 3 diabetes’. Since energy provision via glucose appears to be inadequate in emergent (pre-clinical) AD as well as established AD, it has been suggested that an enhanced supply of ketone bodies may be beneficial due to the resultant enhanced ATP output of mitochondria( Reference Kashiwaya, Takeshima and Mori 69 , Reference Kwiterovich, Vining and Pyzik 76 , Reference Barañano and Hartman 84 ). In type 1 diabetic patients, who would also benefit considerably from sources of energy other than glucose to maintain brain energy homeostasis, an elevation in 3HB levels in plasma( Reference Page, Williamson and Yu 63 ) has been observed when coconut oil has been consumed.
The effectiveness of KD diets in raising ketone body levels is measurable in plasma, as has been shown, for example, by measuring increased 3HB levels in rat plasma( Reference Puchowicz, Xu and Sun 75 ). Significantly, some clinical studies of AD or mild cognitive impairment patients( Reference Page, Williamson and Yu 63 , Reference Puchowicz, Xu and Sun 75 , Reference Reger, Henderson and Hale 85 , Reference Newport 86 ) have reported positive effects on cognitive performance after consuming MCFA-rich foods, while also observing significant increases in blood 3HB levels after treatment (P = 0·007)( Reference Reger, Henderson and Hale 85 ). However, in this last study, the cognitive improvement has not been seen in ApoE-ɛ4 allele carriers (carriage of ApoE-ɛ4 alleles increases AD risk). Later studies investigating KD diets in AD patients have shown that KD diets raised mean serum 3HB levels from about 0·1 mmol/l to about 0·4 mmol/l in these patients( Reference Henderson, Vogel and Barr 87 ). In this trial, AD patients have demonstrated improvement in cognition when measured at 45 and 90 d post ketone supplementation. However, the benefits were seen only in ApoE4-ɛ4 allele-negative patients and resulted in adverse events including diarrhoea, flatulence and dyspepsia. Additional research is important to determine the therapeutic benefits of MCT for patients with AD and how ApoE-ɛ4 status may mediate β-OHB efficacy.
Barañano & Hartman( Reference Barañano and Hartman 84 ) supported the concept that KD can enhance the mitochondrial production of ATP, and prevent the development of AD via numerous other pathways. Together with ATP production, mechanisms proposed include altered brain pH affecting neuronal excitability, direct inhibitory effects on ion channels, increasing levels of both ketone transporters and GLUT-1, increasing capillary density or improving the regulation of sirtuins, a family of proteins that play a major role in mediating anti-ageing effects of energy restriction.
In AD, the deposition of aggregated Aβ peptides in the brain is recognised as a hallmark feature of AD, and while it is known that Aβ is formed by proteolytic cleavage of the amyloid precursor protein (APP) by various proteases, the mechanisms that cause the peptide to accumulate in the brain, aggregate and cause neuronal toxicity are not fully understood( Reference Castellani, Lee and Zhu 88 ). By providing an alternative energy source to glucose, ketones may be able to sustain neuronal viability. In support of this, a dual-tracer positron emission tomography imaging study of rats on a KD showed that the diet caused increases in brain uptake of the two tracers 11C-AcAc and 18F-fluorodeoxyglucose S( Reference Pifferi, Tremblay and Croteau 89 ). Later studies by the same group have shown that a 14-d KD could increase the cerebral metabolic rate of AcAc and glucose by 28 and 44 %, respectively, in aged (24-month) rats( Reference Roy, Nugent and Tremblay-Mercier 90 ). Another recent pilot study( Reference Nafar and Mearow 91 ), which investigated the effects of coconut oil supplementation directly on cortical neurons treated with amyloid-(A) peptide in vitro, has indicated that neuron survival in cultures co-treated with coconut oil and Aβ is rescued compared with cultures exposed only to Aβ. Coconut oil co-treatment also attenuated Aβ-induced mitochondrial alterations. The results of this pilot study have provided a basis for further investigation of the effects of coconut oil, or its constituents, on neuronal survival, focusing on the mechanisms that may be involved( Reference Nafar and Mearow 91 ). There are some contrasting results among the Animal studies. Van der Auwera et al. ( Reference Van Der Auwera, Wera and Van Leuven 92 ) reported a decrease of Aβ in the brain of young transgenic AD mice over expressing the London APP mutation fed with KD for 1·5 months, while study with aged dogs that has reported the effect of KD on Aβ is restricted to the parietal lobe of the brain( Reference Studzinski, MacKay and Beckett 93 ). Kashiwaya et al. ( Reference Kashiwaya, Bergman and Lee 94 ) observed that long-term (8 months) feeding of a ketone ester in middle-aged mice (8·5 months old) improved cognition and reduced Aβ and τ pathology. Another study( Reference Beckett, Studzinski and Keller 95 ) has demonstrated that AD mice model fed with a high-fat, low-carbohydrate KD shows improved motor function but without changes in Aβ. Providing further support for the benefits of high dietary MCFA levels against AD, an in vitro study demonstrated that the addition of ketone bodies (β-hydroxybutyrate) protects the hippocampal neurons from Aβ toxicity, thus suggesting possible therapeutic roles for KD on mitochondrial defects related to AD( Reference Kashiwaya, Takeshima and Mori 69 ). Few studies have demonstrated that KD could significantly improve glucose homeostasis, reducing metabolic dysregulation and insulin resistance (IR), which is important to reduce the pathology of AD( Reference Dashti, Mathew and Khadada 96 – Reference Paoli, Bianco and Grimaldi 98 ).
Morris et al. ( Reference Morris, Evans and Bienias 99 ) suggested that a high intake of unsaturated, unhydrogenated fats may be protective against AD, proposing that coconut oil may also be protective against AD. Despite the positive effect of KD, how the KD affects β-amyloid levels and whether this effect could be disease modifying in AD requires further study.
Adverse effects of ketones
There is a paucity of data on the adverse effects of ketone administration in the literature. A study has reported significant rise in the mean blood cholesterol level to over 2500 mg/l following a prolonged intake of a KD( Reference Freeman, Vining and Pillas 100 ). This effect, in turn, may be atherogenic, leading to lipid deposition in blood vessels. Some researchers have observed dilated cardiomyopathy in patients on the KD, due to the toxic effects of elevated plasma NEFA. Further, an increased incidence in nephrolithiasis as well as increases in serum uric acid levels has been reported( Reference Hall, Andrus and Yonkers 101 , Reference Kielb, Koo and Bloom 102 ). Some side effects are common following administration of ketone bodies, such as dehydration and hypoglycaemia. However, growth retardation, obesity, nutrient deficiency, decreased bone density, hepatic failure and immune dysfunction are also observed, but not frequently( Reference Liu 81 , Reference Henderson, Vogel and Barr 87 ).
Hiraide et al. ( Reference Hiraide, Katayama and Sugimoto 103 ) reported a significant increase in pH and Na concentrations following the administration of a 20 % solution of Na β-hydroxyl butyrate (BHB) to severe trauma patients. Also, reduction in glucose cerebral metabolism and the increase in cerebral blood flow were observed by Hasselbalch et al. ( Reference Hasselbalch, Madsen and Hageman 104 ) during the administration of intravenous BHB. The long-term consequences of these deviations are not yet known.
KD with high-protein diets may cause possible kidney damage due to high levels of N excretion during protein metabolism( Reference Westerterp-Plantenga, Nieuwenhuizen and Tome 105 ). However, several researches have reported that even high levels of protein in the diet do not damage renal function( Reference Skov, Haulrik and Toubro 106 ). KD with very low carbohydrate can cause a regression of diabetic nephropathy due to acidosis( Reference Poplawski, Mastaitis and Isoda 107 ). As the concentration of ketone bodies never rises above 8 mmol/l, this risk is minimum with normal insulin function subjects( Reference Cahill 108 ).
Coconut oil as a source of antioxidants
Antioxidants are substances of natural and synthetic origin that have a high potential to scavenge free radicals( Reference Kim, Park and Kim 109 – Reference Tepe, Sokmen and Sokmen 111 ). The development of AD has been linked to oxidative stress, and studies have suggested that antioxidant-rich natural diets may protect against AD. Although studies on the benefits for AD have not been conclusive( Reference Kim, Park and Kim 109 , Reference Park and Kim 110 , Reference Necula, Kayed and Milton 112 ), many suggest that combinations of (rather than individual) antioxidants are beneficial( Reference Shah 113 ). Coconut oil has a high percentage of phenolic acids, and these are phytochemicals, sometimes also referred to as a polyphenols. Phenolic acids are recognised for their antioxidant properties. p-Coumaric acid, ferulic acid, caffeic acid and catechin acid are the major phenolic acids found in coconut oil( Reference Marina, Man and Nazimah 22 ). The hydroxyl group of phenolic compounds may be able to reduce the toxicity of the Alzheimer's Aβ peptide( Reference Hirohata, Hasegawa and Tsutsumi-Yasuhara 114 – Reference Bastianetto and Quirion 118 ). In vitro studies that have investigated flavonoids indicate that the hydroxyl groups could trap hydrogen bonds of Aβ, which is important as this may reduce Aβ aggregation( Reference Hirohata, Hasegawa and Tsutsumi-Yasuhara 114 ). It has also been shown that phenolic compounds can bind Aβ fibrils with their long axis parallel to the long axis of Aβ fibrils( Reference Krebs, Bromley and Donald 119 ). Several other phenolic compounds have been shown to prevent Aβ aggregation and/or toxicity such as resveratrol, catechin and curcumin( Reference Ono, Hasegawa and Naiki 120 , Reference Porat, Abramowitz and Gazit 121 ). However, despite the encouraging studies mentioned above, the exact mechanisms by which the phenolic group affects Aβ toxicity is not currently clear. While data from AD studies( Reference Liu, Williams and Basualdo-Hammond 80 – Reference Mosconi 83 ) have suggested the beneficial effects of phenolic compounds on Aβ-related pathology, some discrepancies still exist. For example, recent work has demonstrated a significant inhibition of Aβ oligomers as well as higher growth of Aβ fibrils( Reference Wang, Ho and Zhao 122 ) by phenolic compounds. These controversial results should be investigated further( Reference Singh, Arseneault and Sanderson 123 ).
Ferulic acid (4-hydroxy-3-methoxycinnamic acid) is a phenolic compound that has potent antioxidant and anti-inflammatory activities( Reference Ono, Hirohata and Yamada 124 , Reference Zhao and Moghadasian 125 ). Ferulic acid, in particular, is one of the phenolic compounds demonstrated to have strong anti-Aβ aggregation properties( Reference Ono, Condron and Ho 126 ). Researchers have found that the chronic administration of ferulic acid can reduce cortical levels of Aβ1-40 and Aβ1-42 as well as IL-1β levels in APP/PSI AD-model transgenic mice( Reference Ji-Jing, Jun-Sub and Taek-Keun 127 ). Ferulic acid has also been shown to inhibit Aβ deposition in the brain( Reference Ji-Jing, Jun-Sub and Taek-Keun 127 ). However, another study has found that ferulic acid could not prevent the formation of Aβ fibrils, but could reduce the length of the fibrils( Reference Seema and Jayakumar 128 ). It appears that ferulic acid may be able to interrupt the elongation process by binding to the Aβ fibrils( Reference McLaurin, Kierstead and Brown 129 ). In other mouse studies, the long-term administration of ferulic acids could suppress the increase in glial fibrillary acidic protein and IL-1β immunoreactivity in the hippocampus that is induced by Aβ 1–42 treatment( Reference Ji-Jing, Jae-Young and Hee-Sung 130 ).
p-Coumaric acid is another compound found in coconut oil that has high antioxidant capacity( Reference Konishi, Hitomi and Yoshioka 131 ). Maltolyl p-coumarate had been found to attenuate cognitive deficits in rat models and to cause a reduction in apoptotic cell death in the hippocampus of Aβ1–42-infused rats. All these studies have suggested that coconut oil contains many antioxidants with the potential to reduce the development of AD pathology.
Coconut oil in insulin resistance and control of plasma lipids
There are currently no effective AD treatments, and there is currently no cure on the immediate horizon. As mentioned earlier in this review, health issues, such as IR and obesity, similar to CVD, disrupted cholesterol metabolism, type 2 diabetes and hypertension, are all risk factors for AD( Reference Martins, Hone and Foster 132 , Reference Martins, Berger and Sharman 133 ). Recent studies, many of which have already been mentioned, have shown that including coconut oil in the diet can reduce the risk of these factors, and due to the major disruption in insulin function that appears to happen early in the pathogenesis of AD, this aspect has gained particular attention.
IR is a condition where cells fail to respond to the normal actions of the hormone insulin( Reference Ali, Ferris and Naran 134 ). This results in hyperinsulinaemia that can eventually be diagnosed as type 2 diabetes. Insulin and insulin receptors have been reported to be enriched in brain areas where memory functions take place( Reference Ali, Ferris and Naran 134 ). Therefore, impaired insulin regulation results in cognitive and memory shortfalls, such as those observed in AD patients as well as people with mild cognitive impairment( Reference Fernández-Real, López-Bermejo and Vendrell 135 ). Insulin is also an important regulator of proteins involved in the pathology of AD, namely the APP, and τ( Reference Behl, Davis and Klier 136 ). Poor insulin action leads to poor regulation of brain glucose levels, which, in turn, can lead to an acceleration of neurodegeneration, due to oxidative stress and increased Aβ production from APP, both of which are key steps in the pathogenesis of AD( Reference Castellani, Lee and Zhu 88 ).
A higher rate of diabetes has developed in India and South Pacific Islands following dietary changes from traditional fats such as ghee and coconut oil to polyunsaturated fats such as sunflower or safflower oils( Reference Sircar and Kansra 137 ). Conversely, researchers have observed that a diet rich in coconut oil shields against IR in diabetic rats( Reference Kochikuzhyil, Devi and Fattepur 138 ). Furthermore, a more recent study has found that rats fed with LCFA and n-6 PUFA for 8 weeks induce IR, and increased the expression of liver X-receptors (LXRα), carbohydrate response element binding protein and LCFA elongase-6 in the liver and white adipose tissue( Reference Sun, Jiang and Wang 139 ). In contrast, the rats fed MCFA (from coconut oil) had reduced LXRα, carbohydrate response element binding protein and LCFA elongase-6 expression as well as improved insulin signalling and less IR. In an in vitro study that compared LCFA and MCFA effects in myotubes, it has been found that MCFA-treated cells displayed less lipid accumulation, and MCFA increased the intrinsic respiratory capacity of mitochondria without increasing oxidative stress (less reactive oxygen species generation)( Reference Montgomery, Osborne and Brown 140 ). Furthermore, in studies of thiazolidinediones, ligands that increase insulin sensitivity in type 2 diabetes via the PPARγ, it has been found that certain MCFA such as those in coconut oil (C8–C10) are low-potency agonists, yet without the deleterious side effects( Reference Liberato, Nascimento and Ayers 141 ). Such studies are beginning to characterise the mechanisms involved in the insulin signalling-protective effects of MCFA-containing diets. However, not all studies agree; for example, one study of male rats on a MCFA-rich diet has found that the diet causes increases in body adiposity and hyperinsulinaemia and reduces insulin-mediated glucose uptake in the skeletal muscle( Reference Marçal, Camporez and Lima-Salgado 142 ), indicating that further research is required to understand the metabolism and effects of different MCFA.
The major components in coconut oil that are believed to be involved in reducing IR are fatty acids (such as lauric acid (45–50 %) and capric acid) and phenolic compounds (such as ferulic acid and p-coumaric acid)( Reference Sykes and Margaret 143 , Reference Nomura, Kashiwada and Hosoda 144 ). Levels of the beneficial components are believed to be higher in VCO, which, as mentioned earlier, is prepared via a cold or low-heat-based extraction method. This oil contains higher levels of phenolic acids than copra or refined coconut oil( Reference Gopala, Gaurav and Ajit 4 ).
Coconut oil and lipid metabolism
The addition of VCO to the diet has also been associated with a decrease in plasma LDL-cholesterol (LDL-C) and TAG levels and an increase in HDL-cholesterol levels( Reference Nevin and Rajamohan 19 ). In this rat study, Nevin & Rajamohan( Reference Nevin and Rajamohan 19 ) demonstrated that VCO has a higher capacity to reduce serum LDL levels than copra oil, and to reduce LDL oxidation by physiological oxidants. Another study has concluded that coconut oil can lower cholesterol synthesis in human subjects, possibly due to lower production rates of apoB-containing lipoproteins( Reference Cox, Sutherland and Mann 145 ).
Abnormal metabolism of lipoproteins such as lipoprotein (a)/Lp(a) and their variants has been associated with peripheral artery disease, stroke, atherosclerosis, cerebrovascular disease as well as AD( Reference Iwamoto, Watanabe and Umahara 146 , Reference Matsuzaki, Sasaki and Hata 147 ). Coconut oil has been shown to help reduce Lp (a) levels, and the addition of coconut oil to the diet may improve cholesterol metabolism. In a study of twenty-five women, it has been observed that lipoprotein(a) levels are 13 % lower after the women had consumed a high-fat diet containing coconut oil (38·4 % of energy from fat)( Reference Müller, Lindman and Blomfeldt 23 ). The same study has found that the postprandial plasma concentration of tissue plasminogen activator antigen (tPA antigen, often abnormally high in diabetes/IR)( Reference Eliasson, Jansson and Lindahl 148 ), has dwindled when the women consumed the high-fat coconut diet, when compared with women who had consumed a diet high in unsaturated fat. Another study of women has noted that dietary coconut oil intake has been positively associated with HDL-cholesterol levels, especially among pre-menopausal women; the study has also found that coconut oil consumption did not cause a significant increase in LDL-C or TAG levels( Reference Feranil, Duazo and Kuzawa 149 ). A meta-analysis( Reference Siri-Tarino, Sun and Hu 150 ) of prospective epidemiological studies has demonstrated that dietary saturated fat is not associated with an increased risk of CHD or CVD. In contrast to these positive studies, Tsai et al. ( Reference Tsai, Park and Kovacic 151 ) reported that both MCT and lauric acid raised serum LDL-C concentrations compared with the more polyunsaturated baseline diet. Cater et al. ( Reference Cater, Heller and Denke 152 ) also showed that MCT have one-half the potency that palmitic acid has at raising total and LDL-C concentrations. Interestingly, in 2004, Tholstrup et al. ( Reference Tholstrup, Ehnholm and Jauhiainen 34 ) observed that MCFA had a hypercholesterolaemic effect. One study has noted that soya oil reduces cholesterol to a greater degree than coconut oil with no influence on HDL-cholesterol( Reference Ganji and Kies 153 ), and addition of Psyllium fibre supplementation lowers serum cholesterol regardless of saturation level of dietary fat( Reference Ganji and Kies 153 ). As cholesterol metabolism and AD pathology have been shown to be linked( Reference Matsuzaki, Sasaki and Hata 147 ), further clinical research is required to understand the contribution of coconut oil to cholesterol metabolism and AD. Nevertheless, due to the many likely benefits of VCO, most researchers would recommend the inclusion of coconut oil in the diet; however, researchers are yet to decide how much coconut oil is required for optimal health. Two studies have recommended a daily intake of 3·5 tablespoons of VCO for a 72 kg man( Reference Hayatullina, Norliza and Norazlina 154 , Reference Isaacs and Thormar 155 ). This was based on the quantity of MCFA present in human breast milk. Interestingly, VCO and human breast milk have more saturated fats than mono- or poly-unsaturated fats, and in both cases, the main fat is lauric acid, with VCO containing the most, at about 50 %( Reference Isaacs and Thormar 155 ). However, coconut dosage to enhance the memory of impaired people has not been concluded.
Apart from the benefits already mentioned above, both lauric acid, the main fatty acid in coconut, and phenolic compounds have anti-microbial or anti-bacterial properties. Thus, these compounds are considered to be protective against low-grade infections often associated with IR( Reference Ji-Jing, Jun-Sub and Taek-Keun 127 , Reference Ji-Jing, Jae-Young and Hee-Sung 130 ). Interestingly, specific fractions of coconut oil, extracted under hot conditions, have been shown to reduce blood glucose, cholesterol and lipid peroxidation, and some polyphenolic compounds appear to reduce liver lipid peroxidation( Reference Seneviratnea, HapuarachchIa and Ekanayake 156 , Reference Mahadevappa, Arunchand and Farhath 157 ).
Coconut oil and blood–brain barrier
The blood–brain barrier (BBB) is a brain endothelial structure of the fully differentiated neurovascular system( Reference Zlokovic 158 ) that protects the brain from foreign substances. It is noted that more than 98 % of all small-molecule drugs, and approximately 100 % of all large-molecule drugs or genes, do not cross the BBB( Reference Pardridge 159 ). Thus, it is very difficult to develop effective new neurotherapeutics for AD that permeate the BBB. However, there is literature that indicates that circulating D-β-3hydroxybutyrate ketone body, which is formed out of MCFA, crosses the BBB and enters the mitochondria where it is metabolised to AcAc and converted to acetyl-CoA, which enters into the Krebs cycle( Reference Laffel 160 ). One in vivo study with mice has identified the capacity of caprylic acid, a constituent of coconut oil, to cross the BBB. This study indicates that as a result of crossing the BBB, caprylic acid demonstrated anti-convulsant and a neuroprotective effect( Reference Wlaź, Socała and Nieoczym 161 ).
Coconut water
In countries where coconuts are a primary produce, coconut water is a common beverage. Coconut water contains a range of beneficial ingredients, including vitamins, minerals, antioxidants, amino acids, enzymes, growth factors and other nutrients( Reference Adams and Bralt 162 ). Cytokinins, a class of plant growth hormones (phytohormones) present in coconut water, influence plant cell division, and are considered to have anti-ageing properties( Reference Letham 163 , Reference Huan, Takamura and Tanaka 164 ). There are two types of cytokinins: adenine-type cytokinins (kinetin, zeatin and 6-benzylaminopurine) and phenylurea-type cytokinins (diphenylurea and thidiazuron). Recent studies have investigated the possibility of using trans-zeatin as a treatment drug for neuronal diseases including AD. Zeatin has demonstrated antioxidant and cell protective effects against Aβ-induced neurotoxicity in cultures of neuronal PC12 cells, and in experiments of mice treated with scopolamine to induce amnesia, pretreatment of the mice with zeatin caused a reduction in the level of induced amnesia, according to the passive avoidance test and Y maze test( Reference Choi, Jeong and Choi 165 ). Interestingly, another study has found that trans-zeatin could inhibit acetylcholinesterase( Reference Heo, Hong and Cho 166 , Reference Mirjana, Danijela and Tamara 167 ). This indicates that cytokinin could have therapeutic value, as levels of the neurotransmitter acetylcholine are reduced in AD, and acetylcholinesterase inhibitors are currently used to ameliorate the symptoms of AD.
Coconut water has also been shown to have beneficial effects on serum and tissue lipid parameters, when given to rats concurrently fed a high-cholesterol containing diet( Reference Sandhya and Rajamohan 168 ). Another study has investigated the positive effect of regular consumption of two tropical food drinks, coconut (C. nucifera) water and mauby (Colubrina arborescens), on the control of hypertension( Reference Alleyne, Roache and Thomas 169 ). The combined products were found to be almost twice as effective as the products in isolation.
Other coconut food products
Apart from coconut water and extracted coconut oil, the coconut has a number of other culinary uses. The fleshy part of the seed, the coconut meat, can be used fresh or dried in cooking. Coconut cream and coconut milk are made by pressing the flesh to extract fluid, and these are used in many countries in cooking; for example, coconut milk is a component of many curries in India, Sri Lanka and other Asian countries. Desiccated coconut and coconut flour are also used in cooking and baking. Other products include coconut chips and flakes. Each of these products has a lipid (MCFA) component, and may also contain high levels of both soluble and insoluble fibre, as well as varying levels of the antioxidants and other beneficial components already mentioned above. Research has shown that many of these coconut products can improve lipid profiles as well as provide other benefits. For example, one study( Reference Chukwunonso, Obioma and Ifeoma 170 ) has shown that the consumption of coconut milk does not elevate serum lipid levels, and another study( Reference Ekanayaka, Ekanayaka and Perera 171 ) has found that a coconut milk porridge fed to sixty healthy people 5 d a week for 8 weeks caused a decrease in LDL levels and an increase in HDL levels. Further studies should be carried out to help validate these significant benefits of consuming coconut milk and cream, and to determine whether such benefits are counteracted by any unfavourable changes to serum lipid profiles. In another study( Reference Trinidad, Anacleta and Aida 172 ), coconut flakes have been shown to reduce total cholesterol as well as LDL-C and serum TAG levels. Coconut residue after fluid extraction has a high percentage of soluble (3·41 g/100 g) and insoluble (34·0 g/100 g) dietary fibre( Reference Ng, Tan and Lai 173 ), and such high fibre content has been suggested to contribute to many of coconut's health benefits as in the coconut flake study mentioned above.
Salil et al. ( Reference Salil, Nevin and Rajamohan 174 ) demonstrated an improvement in diabetic indicators following the consumption of coconut flesh; in this case, it is believed to be due to the protein content of coconut( Reference Nwangwa and Chukwuemeka 175 ). The coconut kernel protein is rich in arginine, and the observed anti-diabetic activity of coconut flesh has been suggested to be due to the provision of arginine, which has been shown to influence pancreatic β cell regeneration( Reference Salil, Nevin and Rajamohan 174 , Reference Nwangwa and Chukwuemeka 175 ). Similarly, another study has found that coconut water has a blood glucose-lowering effect and that coconut milk has a regenerative effect on the pancreatic cells damaged by diabetes( Reference Henderson, Vogel and Barr 87 ). Arginine is a precursor of NO, produced by the endothelial isoform of NO synthase, and NO is a signalling molecule that has a direct influence on insulin sensitivity. Maintaining NO production is also thought to be important in reducing cardiovascular complications of diabetes: arginine availability impacts on NO production, which can expand the blood vessels, allowing for the BP in the patients to be reduced( Reference Hoang, Padgham and Meininger 176 ).
Conclusions
The consumption and use of coconut in its various forms has a long and established history in medicinal, scientific and nutritional arenas. While consumed prolifically in regions engaged in coconut primary production, Western cultures have tended to highlight the fatty acid content, particularly the saturated fat, and therefore limited its culinary usage.
The lipid content of coconut, being mostly MCFA, offers an energy source that bypasses the usual glucose pathway, in the form of ketone bodies, and without the associated fat deposition often caused by LCFA. Despite the positive effect of a KD, whether the KD influences β-amyloid levels and protects against AD requires further study. The dosage of ketones and the duration relevant to the AD also needs to be investigated. At this time, it is not clear whether ketone bodies produced from coconut oil has a direct effect on AD, specifically in relation to slowing or clearance of Aβ and τ pathologies – and if so, under what conditions. Furthermore, research needs to be conducted to quantify the yield of ketones from VCO, and support the ability of coconut derivatives to cross the BBB, to establish likely efficacy.
However, evidence to suggest that coconut may lower total and LDL-C, reduce systolic BP and ameliorate IR is of particular interest, in relation to AD risk reduction. A small number of clinical trials and animal studies using a formulation of MCT have reported significant improvement of cognition in AD patients. At the same time, studies in which the diet has been supplemented with SFA, particularly hydrogenated coconut oil, have reported deleterious effects on hippocampal morphology and behaviour, and increased plasma LDL levels.
Evidence suggests that despite coconut being a saturated fat, it may not pose the usual negative effects on lipid profiles; however, the influence on neuronal function and survival, as well as cardiovascular effects remains unknown. While the nutritional components of coconut are well accepted, inconsistencies in the data, it is suggested that further research needs to be undertaken before broadly advocating the use of coconut oil in addition to existing fat consumption or in substitution.
Coconut is, however, widely available, inexpensive, non-toxic and highly palatable, and consuming a regular intake of good quality coconut oil or another coconut product may become a simple yet important dietary change that may be shown in the future to reduce the risk of AD. However, research has suggested that the extraction method used to obtain VCO appears to affect the quality of coconut oil and may directly affect the efficacy. If specific extraction methods are essential to achieve efficacy, only particular preparations may confer benefit. Once this is known, further analysis needs to be undertaken regarding the absorption process, recommended dose, and whether it should be taken in combination with other food groups or in isolation.
It must be emphasised that the use of coconut oil to treat or prevent AD is not supported by any peer-reviewed large cohort clinical data; any positive findings are based on small clinical trials and on anecdotal evidence; however, coconut remains a compound of interest requiring further investigation.
Acknowledgements
The authors gratefully acknowledge the support from the McCusker Alzheimer's Research Foundation and Edith Cowan University. W. M. A. D. B. F. is supported by the McCusker Alzheimer's Research Foundation and a grant from the CSIRO for the AIBL (The Australian Imaging, Biomarkers and Lifestyle) study. K. G. G. is supported by a grant from the Anglican Retirement Villages, Foundation for Aged Care and a grant from the CRC-Mental Health Limited. All authors contributed to the literature search, analysis of the data published, manuscript writing and revisions of the article. The authors declare no conflicts of interest arising from the conclusions of this research.