Nutrition is essential for the maintenance of optimal health. Both under- and overnutrition lead to disease development and increase in the rate of mortality. Both phenomena are still present in human society, and it is rather difficult to overcome them, despite the unprecedented technological advances in food science in the past few decades. In addition, we witness the occurrence of obesity accompanied by deficit of essential nutrients and plant food bioactives in low- and middle-income countries, as well as in low-income subgroups in developed countries(Reference Anderson1,Reference Dammann and Smith2) . Other than economic status, it has also been suggested that the educational status has a significant influence on food choices(Reference Barker, Lawrence and Crozier3).
Plant food bioactives are important constituents of the human diet. Although not classified as essential nutrients, they may have beneficial effects on human health. Unlike vitamins, their inadequate intake will not result in specific syndrome of deficit, but rather in increased risk of chronic diseases. The plant food bioactives include terpenoids (isoprenoids), phenolic compounds (including polyphenols), glucosinolates, betalains and others. In this review, we will focus on polyphenols and their effects on human cardiometabolic health. Indeed, there is growing evidence about the preventive effects of polyphenols on cardiometabolic diseases, such as type 2 diabetes mellitus(Reference Grosso, Stepaniak and Micek4) and atherosclerosis(Reference Medina-Remon, Casas and Tressserra-Rimbau5). There is also evidence that polyphenols exert beneficial effects on neurodegenerative diseases(Reference Sawikr, Yarla and Peluso6) and some forms of cancer(Reference Costa, Tsatsakis and Mamoulakis7), which will not be addressed on this occasion.
Because of their remarkable in vitro free radical-scavenging properties, early investigators attributed the beneficial effects of polyphenols on human health exclusively to their capacity to scavenge free radicals(Reference Salah, Miller and Paganga8,Reference Visioli, Bellomo and Galli9) . Regarding their cardioprotective effects, many studies focused on LDL oxidation, which plays central role in atherogenesis(Reference Vinson10). Indeed, it has been demonstrated that upon consumption of foods and beverages rich in polyphenols, the resistance of LDL to oxidation increases(Reference Miura, Chiba and Miura11). Notably, LDL oxidisability is still of interest(Reference Hernaez, Remaley and Farras12). Following these observations, polyphenols became an integral part of the theory of oxidative stress.
Therefore, in the first part of this review, we will give an overview of the theory of oxidative stress and a summary of the results of antioxidant supplementation studies. In the second part, we will focus on polyphenols and their antioxidant properties, which will be followed by an overview of their effects on cardiometabolic health, and our present understanding of the inter-individual variability in the response of their consumption.
Oxidative stress and antioxidant supplementation
Definition of oxidative stress and mechanism of lipid peroxidation
The term ‘free radical’ refers to the chemical species containing one or more unpaired electrons, which encompass various O-, C-, N- or S-centred radicals. Among them, the O-centred radicals are the most prevalent in the biological systems(Reference Kehrer and Klotz13), including the superoxide radical and the highly reactive hydroxyl radical. These, along with the non-radical hydrogen peroxide, are the most common reactive oxygen species (ROS). Following Harman’s free radical theory of aging (1956)(Reference Harman14), the term ‘oxidative stress’ was first mentioned in 1970, referring to the oxidative challenge of erythrocytes by hydrogen peroxide(Reference Paniker, Srivastava and Beutler15). In 1985, oxidative stress was defined as an imbalance between oxidants and antioxidants, in favour of the former(Reference Sies and Sies16), focusing on the oxidative damage of cells, tissues and organs(Reference Sies and Cadenas17). Since then, the concept has evolved substantially, leading to redefinition of oxidative stress as a dysfunction of cellular redox signalling and redox control(Reference Jones18,Reference Jones19) . Most recently, a distinction has been made between oxidative eustress, as a physiological condition that is essential for redox signalling, and oxidative distress, as a supraphysiological condition that causes damage to biomolecules(Reference Sies and Fink20). So far, hundreds of thousands of papers have been published demonstrating and confirming the association of oxidative stress with various human diseases, including cardiometabolic ones. Indeed, nowadays, it is almost impossible to find a human disease or condition that is not associated with the oxidative stress.
Classical theory describes the oxidative stress as an overall imbalance between the ROS from one side and the cellular antioxidants from the other, of course in favour of ROS. This imbalance occurs because of an excessive production of ROS, or an inadequate concentration and/or activity of the cellular antioxidants (non-enzymatic or enzymatic), or because of both(Reference Birben, Sahiner and Sackesen21). The classical understanding of the oxidative stress was a leitmotif of the early attempts to translate the immense amount of scientific evidence into clinical practice. Within these efforts, it seems that the chain reaction of lipid peroxidation attracted most attention. Hydroxyl radical (HO·), as the most reactive ROS, reacts in a high rate reaction with PUFA, in the first place linoleic or arachidonic acid of membrane phospholipids. Hydroxyl radical abstracts hydrogen atom from PUFA, which starts the autocatalytic chain reaction of lipid peroxidation. In this initiating reaction, a carbon-centred lipid radical is formed (L·), which further reacts with molecular oxygen to generate an oxygen-centred lipid peroxyl radical (LOO·). The lipid peroxyl radical can attack another PUFA to generate lipid hydroperoxide (LOOH) and a new carbon-centred lipid radical, which propagates the chain reaction(Reference Zimniak22). Lipid hydroperoxides have the ability to react with trace metals to generate lipid alkoxyl radicals (LO·). Both lipid peroxyl radicals and lipid alkoxyl radicals undergo cyclisation and/or degradation, generating a plethora of reactive aldehydes such as malondialdehyde (MDA), trans-4-hydroxy-2-nonenal, trans-4-oxo-2-nonenal, acrolein, glyoxal and many others. What reactive aldehydes will be generated depends on the type of PUFA subjected to lipid peroxidation(Reference Cipak Gasparovic, Zarkovic and Zarkovic23). Importantly, these reactive aldehydes have the ability to oxidise proteins(Reference Ruskovska and Bernlohr24) and DNA(Reference Gueraud, Atalay and Bresgen25). Therefore, if present in excess and/or for a longer period, they can induce cellular damage and consequently induce disease development.
Lipid peroxyl radicals can be scavenged not only by vitamin E but also by other nutrients such as polyphenol or β-carotene(Reference Tsuchihashi, Kigoshi and Iwatsuki26), which terminates the chain reaction of lipid peroxidation. Notably, the chemistry behind the scavenging of lipid peroxyl radicals by polyphenols and vitamin E is very similar(Reference Spiteller27). Following the reaction of termination by vitamin E, a relatively stable α-tocopheryl radical is formed (α-tocopherol is the predominant form of vitamin E in human tissues(Reference Jiang, Christen and Shigenaga28)). The α-tocopheryl radical can be oxidised to a stable non-radical quinone or reduced back (recycled) to hydroquinone by vitamin C(Reference Kurutas29). However, the biological relevance of the later reaction is not completely clear(Reference Niki30). Other possibilities for termination of the chain reaction of lipid peroxidation are the following: substrate unavailability, collision of two LOO· molecules or reaction of LOO· with other radicals.
Antioxidant supplementation studies – hopes and pitfalls
Undoubtedly, if present at high concentrations, ROS, free radicals and reactive aldehydes will cause cellular damage and eventually disease. Therefore, for early investigators, it seemed logical that supplementation with low molecular weight antioxidants would prevent the cellular damage, resulting in beneficial effects on human health and decreased mortality. Following this assumption, numerous randomised controlled trials (RCT) have been conducted with the aim to study the effects of supplementation with vitamin C, vitamin E, β-carotene, vitamin A and Se, alone or in various combinations, on oxidative stress and health maintenance. Some of these studies included patients with various chronic diseases. In others, the relevance of antioxidant supplementation in primary prevention was studied on healthy subjects. A meta-analysis of sixty-seven RCT was conducted and published in 2008(Reference Bjelakovic, Nikolova and Gluud31), the results of which were discouraging. The same authors repeated the meta-analysis, including the papers that were published subsequently and the updated meta-analysis of seventy-eight RCT was published in 2012(Reference Bjelakovic, Nikolova and Gluud32). The results were similar, that is, showing ‘no evidence to support health effects of supplementation with low molecular weight antioxidants in both primary and secondary prevention’. Moreover, it has been demonstrated that supplementation with vitamin E, β-carotene and vitamin A may slightly increase mortality. After the initial disappointment, a quest for rational explanation has been initiated and several important issues have been raised, which will be presented in the following paragraphs.
Over-simplification of the theory of oxidative stress has been identified as an important cause of the disappointing results of the antioxidant supplementation studies, which were an attempt to translate the accumulated scientific evidence into practice. This over-simplification has been referred as a ‘translational shortcut’(Reference Ghezzi, Jaquet and Marcucci33). Indeed, behind the simplified representation of the oxidative stress as an overall imbalance between ROS and antioxidants, there is a complex network of cellular redox regulators. These include antioxidant enzymes and low molecular weight antioxidants that work together, and along with other cellular factors, to establish and maintain the cellular redox balance. Obviously, an over-simplification of this complex network down to unselective supplementation with high doses of free radical scavengers was not effective. Moreover, the finding of increased mortality points out that ‘natural’, which refers to both vitamin E and β-carotene, is not equal to ‘safe in high doses’. Another potential issue might be the composition and/or quality of supplements, which would be extremely difficult to address from our perspective.
In the antioxidant supplementation studies, an important factor that should have been taken into account is the role of reactive aldehydes as second messengers of free radicals. Indeed, there is evidence that, in its physiological concentrations, trans-4-hydroxy-2-nonenal acts as a signalling molecule, and as such is involved in many biological processes(Reference Milkovic, Gasparovic and Zarkovic34), including adaptive response to other oxidative stressors(Reference Chen, Saito and Yoshida35). Therefore, supplementation with high doses of vitamin E may impair the physiological role of trans-4-hydroxy-2-nonenal, potentially leading to adverse effects. It is indicative that an increased mortality was observed in supplementation with vitamin E, β-carotene and vitamin A, all of them liposoluble compounds, but not with vitamin C. Indeed, liposolubility aggravates elimination, which may be an important element for development and manifestation of adverse effects.
Vitamin E(Reference Azzi36) and β-carotene(Reference von Lintig37) have multiple biological functions. Currently, it is not completely understood how these functions were implicated in the results of the RCT of antioxidant supplementation. However, some aspects have been discussed in a recent review(Reference Vrolijk, Opperhuizen and Jansen38). Namely, it has been demonstrated that β-carotene inhibits the enzymatic activity of glutathione-S-transferase π, which provides protection against benzo(a)pyrene diol epoxide, a toxic metabolite of tobacco smoke. The decreased enzymatic activity of glutathione-S-transferase π has been proposed as the underlying mechanism of the increased incidence of lung cancer in smokers supplemented with β-carotene(39). On the other hand, it has been demonstrated that vitamin E supplementation has beneficial health effects in specific groups of patients(Reference Niki40), such as patients with non-alcoholic steatohepatitis(Reference Sanyal, Chalasani and Kowdley41), diabetic patients with haptoglobin 2-2 genotype(Reference Vardi, Levy and Levy42), patients on haemodialysis(Reference Ruskovska, Pop-Kostova and Jansen43) and patients with oxidative stress-induced hypertension(Reference Ahmad, Yuan Yuan and Nawaz44). Moreover, some meta-analyses did not confirm the finding regarding the increased mortality in persons supplemented with vitamin E(Reference Abner, Schmitt and Mendiondo45,Reference Curtis, Bullen and Piccenna46) . These examples illustrate the need to identify population groups and individuals who will most benefit from supplementation with antioxidant vitamins, as precisely as possible, including the dose and duration of supplementation. To the best of our knowledge, the inter-individual variability of response to low molecular weight antioxidants has not been explored in detail so far. Biomarkers of oxidative stress(Reference Jansen and Ruskovska47) shall be used for identification of patients who will most benefit from supplementation with antioxidant vitamins. However, the shortcomings of the currently available biomarkers(Reference Frijhoff, Winyard and Zarkovic48) do not allow their effective use in clinic. Therefore, a development of better, biologically much more specific and analytically more precise biomarkers of oxidative stress is of utmost importance.
Polyphenols and their antioxidant properties
Polyphenols in the human diet
Polyphenols are plant food bioactives that possess remarkable in vitro antioxidant capacity(Reference Kurin, Mucaji and Nagy49). They have the ability to scavenge lipid peroxyl radicals(Reference Erben-Russ, Bors and Saran50). As such, they are an integral part of the theory of oxidative stress(Reference Siti, Kamisah and Kamsiah51). Polyphenols are present at relatively high concentrations in the human diet. However, depending on individual(Reference Gonzalez, Fernandez and Cuervo52) and national nutrition habits, there is a large difference in daily consumption, ranging from 378 (se 15)(Reference Miranda, Steluti and Fisberg53) to 1757 (sd 696)(Reference Grosso, Stepaniak and Topor-Mądry54) mg/d. Dietary polyphenols originate mainly from plant-based foods including fruits (mainly berries and apples), vegetables (onions, spinach, asparagus and broccoli), spices (black pepper, curcuma and saffron), whole grains, coffee, cocoa, tea, olive and various kinds of nuts(Reference Scalbert and Williamson55). Many of them are also administered as food supplements or herbal remedies. Polyphenols are well studied and classified. They are widespread in the plants, encompassing over 8000 different compounds(Reference Pandey and Rizvi56). However, the number of polyphenols that are relevant to human nutrition is much smaller. Two databases containing comprehensive information on nutritional polyphenols have been developed and are freely accessible: http://phenol-explorer.eu/ (Reference Neveu, Perez-Jimenez and Vos57) and https://www.ars.usda.gov/ARSUserFiles/80400525/Data/Flav/Flav_R03-1.pdf (Reference Bhagwat, Haytowitz and Holden58).
Regarding the health benefits of consumption of foods and beverages rich in polyphenols, the first epidemiological study demonstrating an inverse association between flavonoid intake and mortality from CHD was published in 1993(Reference Hertog, Feskens and Hollman59). Unfortunately, another translational shortcut was taken here. Since polyphenols demonstrate high in vitro antioxidant capacity, it was assumed that the in vitro antioxidant capacity of any food or plant extract can be used as a direct determinant (or, in other words, a measure) of their beneficial health effects in humans. Following this concept, a list of in vitro antioxidant capacities of foods and beverages (measured with Oxygen Radical Absorbance Capacity assay) was published(60). Besides the Oxygen Radical Absorbance Capacity assay(Reference Prior, Hoang and Gu61,Reference Gillespie, Chae and Ainsworth62) , several other methods were also developed, such as trolox equivalent antioxidant capacity, 2,2-diphenyl-1-picrylhydrazyl and ferric reducing antioxidant power assay(Reference Litescu, Eremia and Radu63). However, growing scientific evidence started to elucidate some of the numerous and complex mechanisms of absorption, distribution, metabolism and excretion (ADME) of polyphenols, as molecular basis of their low bioavailability. It also became clear that the in vivo antioxidant activity of polyphenols involves much more complex mechanisms than the free radical scavenging and that molecular mechanisms that are not directly associated with the antioxidant enzymes are also involved in their health promoting effects. At the same time, the Oxygen Radical Absorbance Capacity list was misused by food and dietary supplement manufacturing companies to promote their products(Reference Cunningham64). All this together led to withdrawal of the Oxygen Radical Absorbance Capacity list.
Metabolism of nutritional polyphenols in the human body
Bioavailability of nutritional polyphenols is low and far from uniform. Their plasma concentrations are at range of nm to low µm (Reference Manach, Williamson and Morand65). A notable exception is the gastrointestinal tract(Reference Halliwell, Zhao and Whiteman66), where the intestinal cells are in contact with high concentrations of polyphenols and other plant food bioactives. In the human body, polyphenols are subjected to complex and extensive metabolic transformations(Reference Del Rio, Rodriguez-Mateos and Spencer67), which significantly alter their chemical properties and sometimes diminish their antioxidant activity(Reference Lotito, Zhang and Yang68). Notably, lack of association between the antioxidant activity and some of the biological effects of polyphenol metabolites has been clearly observed(Reference Loke, Proudfoot and Stewart69). Therefore, the in vitro total antioxidant capacity of foods, beverages or plant extracts cannot be a direct determinant of their biological effects.
Polyphenols are a heterogeneous family of plant food bioactives, encompassing several classes of bioactive compounds, such as flavonoids, phenolic acids, lignans, stilbenes and other polyphenols(Reference Neveu, Perez-Jimenez and Vos57). Because of the heterogeneity in their chemical structures, the metabolism of compounds that belong to different classes and subclasses, or even the metabolism of individual compounds, is different, which has been extensively reviewed elsewhere(Reference Del Rio, Rodriguez-Mateos and Spencer67,Reference Williamson and Clifford70) . Here, we will give only a general overview of the metabolism of polyphenols, pinpointing the factors that are relevant for the inter-individual variability in response to their consumption. In the human diet, most of the polyphenols are found as glycosides. Hydrolysis of glycoside conjugates is a critical step in absorption of polyphenols in the small intestine, following which corresponding aglycones appear in the intestinal cells. In the intestinal cells, these aglycones are further subjected to an initial phase II metabolism, resulting in appearance of sulphate, glucuronide or methyl metabolites. These metabolites enter the circulation and reach the liver, where their phase II metabolism continues; only a small amount is transported back to the lumen of the small intestine, eventually reaching the large intestine. Therefore, after the absorption in the small intestine, polyphenol phase II metabolites are predominantly found in the circulation(Reference Mullen, Edwards and Crozier71), whereas the appearance of parent compounds, such as (-)-epicatechin-3-O-gallate and (-)-epigallocatechin-3-O-gallate, is practically an exception of the general rule. Post-absorption plasma concentrations of phase II metabolites are low, reflecting the low bioavailability of polyphenols, which is further emphasised by their rapid urinary excretion. A plethora of enzymes and transporters is involved in the processes of hydrolysis, absorption, phase II metabolism and renal excretion of polyphenols(Reference Del Rio, Rodriguez-Mateos and Spencer67). Theoretically, polymorphisms in the genes that code these proteins may be involved in the absorption, distribution, metabolism and excretion component of the overall inter-individual variability in response to consumption of nutritional polyphenols. Indeed, it has been demonstrated that catechol-O-methyltransferase (enzyme that catalyses methylation of nutritional polyphenols in the human body) genotype (rs4680) may determine the health benefits from green tea intake(Reference Inoue-Choi, Yuan and Yang72). However, the existing data are limited, and genetic factors, along with the influence of gut microbiota, should be addressed in more detail in future studies(Reference Gibney, Milenkovic and Combet73).
Indeed, only a small portion of the nutritional polyphenols is absorbed in the small intestine, while most of them reach the large intestine where they are subjected to extensive metabolism. Gut microbiota plays an important role in the metabolism of nutritional polyphenols, which results in the appearance of a plethora of gut microbiota metabolites, such as aglycones, small phenolic acids and valerolactones. Some of these molecules are absorbed in the large intestine and are further metabolised in the liver. There is strong scientific evidence that gut microbiota metabolites are among the key mediators of the (cardiometabolic) health promoting effects of polyphenols(Reference Kawabata, Yoshioka and Terao74,Reference Fernandez-Millan, Ramos and Alvarez75) . In addition, with identification of different metabotypes(Reference Gonzalez-Sarrias, Garcia-Villalba and Romo-Vaquero76,Reference Hazim, Curtis and Schar77) , gut microbiota has been pinpointed as one of the determinants of inter-individual variability in response to consumption of polyphenols.
Molecular mechanisms involved in the antioxidant effects of polyphenols and beyond
With the evolution of our understanding of oxidative stress (see section ‘Definition of oxidative stress and mechanism of lipid peroxidation’) and elucidation of the complex metabolism of polyphenols in the human body (section ‘Metabolism of nutritional polyphenols in the human body’), the concept that health promoting effects of polyphenols are mediated solely by their ability to scavenge free radicals in vivo has been abandoned. Indeed, it has been clarified that polyphenol metabolites do not scavenge substantial amount of free radicals in vivo and that α-tocopherol is the key compound that terminates the chain reaction of lipid peroxidation. Hence, summarising the latest scientific evidence from both fields, the major mechanism by which polyphenols contribute to the cellular antioxidant defence has been proposed recently(Reference Forman, Davies and Ursini78). Namely, after consumption, low concentrations of mainly metabolised polyphenols reach the cells. Some of them, such as catechin, epicatechin, hydroxytyrosol, delphinidin and carnosic acid, are oxidised by free radicals, in free radical-scavenging reactions. An important target of the oxidised polyphenols is Keap1 (Kelch like ECH associated protein 1), a cytosolic protein whose function is to regulate the activity of transcription factor Nrf2 (nuclear factor, erythroid 2 like 2). Some polyphenols, such as resveratrol and curcumin, bind with Keap1 directly, without prior oxidation. The regulatory function of Keap1 consists of assistance in ubiquitination of Nrf2, which results in its degradation by proteasome. After binding a polyphenolic compound, Keap1 is inactivated and the half-life of Nrf2 is extended. Therefore, Nrf2 migrates to the nucleus, binds to the antioxidant response element (also known as electrophile response element) and initiates the transcription of genes that are involved in the antioxidant defence and detoxification. Besides Nrf2, other transcription factors, such as c-Maf, c-Jun, c-Fos, Fra1, Bach1, Nrf1 or c-Myc, are also involved in the antioxidant response element-mediated transcription in a complex interplay. In addition, there is evidence that protein kinases, such as protein kinase C or phosphoinositide-3-kinase, play role in the phosphorylation of Nrf2, which is a critical step for its translocation to the nucleus. Recent findings that polyphenol metabolites have potential to modulate kinases’ activities are indicative of multilevel regulation of Nrf2-mediated transcription.
Besides the Keap1/Nrf2/antioxidant response element pathway, nutritional polyphenols also modulate a plethora of cellular functions that are not directly connected with the enzymatic antioxidant defence, but are still very closely associated with the subtle regulation of cellular redox balance. Related to the cardiometabolic health, the effects of polyphenols on cellular processes that are involved in chronic inflammation, endothelial dysfunction, impaired insulin signalling, adipose tissue remodelling or mitochondrial dysfunction have been reviewed in detail(Reference Krga, Milenkovic and Morand79–Reference Kerimi and Williamson82). Similar to what has been demonstrated for Keap1, there is growing body of scientific evidence that molecular mechanisms of health promoting effects of polyphenols involve their binding to specific proteins, and subsequent effects on cell signalling. For instance, in silico docking analyses revealed that curcumin also has the ability to bind to some kinases, such as TGF-β-activated kinase 1 (mitogen-activated protein kinase kinase kinase 7), 3-phosphoinositide-dependent protein kinase 1, serine/threonine kinase (AKT) 1 and AKT2, which are involved in regulation of the redox-sensitive pro-inflammatory transcription factor NF-κB. Indeed, NF-κB is established as a key mediator of inflammation, which is a common underlying mechanism of cardiometabolic diseases. Accordingly, a pre-exposure of human umbilical vein endothelial cells to curcumin (1 µm) prior to TNFα stimulation attenuates the activation of NF-κB. In parallel, curcumin decreases monocyte adhesion and transendothelial migration and modulates the expression of genes involved in antioxidant defence, metabolism, cell signalling, focal adhesion, intercellular junction and cytoskeleton organisation(Reference Monfoulet, Mercier and Bayle83), which is altogether pertinent to an improved endothelial function. Similarly, it has been demonstrated that pre-exposure of human umbilical vein endothelial cells to physiologically relevant concentrations of plasma anthocyanins’ metabolites (including their gut microbiota metabolites) also decreases the TNFα-induced monocyte cell adhesion. Gene expression analysis demonstrated modulation of genes that are involved in cell adhesion, leucocyte transendothelial migration, regulation of actin cytoskeleton or NF-κB signalling. Subsequent bioinformatic analysis and in silico docking demonstrated that anthocyanins’ metabolites can bind to several cell signalling proteins, including dual specificity mitogen-activated protein kinase kinase 2 and NF-κB inhibitor kinase alpha, and potentially modulate their activity. The results of in silico docking were confirmed with Western blot analysis, demonstrating a decreased phosphorylation of mitogen-activated protein kinase and p65 unit of NF-κB, pertinent to the modulation of upstream kinases, and the degreased leucocyte transendothelial migration and inflammation(Reference Krga, Tamaian and Mercier84). In addition, it has been demonstrated that flavanol colonic metabolites activate endothelial nitric oxide synthase in human endothelial cells via AKT and AMP-activated protein kinase signalling and increase intracellular nitric oxide, which plays a pivotal role in regulation of endothelial function(Reference Alvarez-Cilleros, Ramos and Goya85). An integrated systems’ biology approach has been taken recently to elucidate the multi-target and multi-layer cellular effects of circulating epicatechin metabolites on endothelial cells, demonstrating modulation in gene and microRNA expressions and DNA methylation. In silico docking demonstrated favourable binding of epicatechin metabolites to p38 mitogen-activated protein kinase, which regulates several transcription factors such as NF-κB, CREB1, c-MYC, c-JUN, STAT3 or SP1(Reference Milenkovic, Berghe and Morand86).
Molecular mechanisms of the effects of polyphenols on cardiometabolic health have also been studied in other relevant cell models, such as immune cells, adipocytes, hepatocytes, smooth muscle cells and β-cells. Aiming to systematise the present scientific evidence, we recently extracted and analysed the nutrigenomic data related to the effects of flavanols or their circulating metabolites in these cell models (paper under submission). Thereby, it was noticed that in large number of studies, the cells were exposed to plant extracts or high concentrations of pure compounds, which are not physiologically relevant(Reference Gibney, Milenkovic and Combet73). This knowledge gap needs to be addressed in future, in order to better understand the molecular mechanisms of health promoting effects of polyphenols.
Regarding the contribution of nutritional polyphenols to the cellular antioxidant defence, an important consideration is that they are present in the cells at low concentrations. Therefore, they cannot scavenge a significant amount of free radicals. Instead, they modulate transcription of the genes involved in antioxidant defence and detoxification. On the other hand, if present at high concentrations, polyphenols may induce toxicity. These considerations are integrated in the concept of hormesis(Reference Rattan87), which also applies to nutritional polyphenols(Reference Son, Camandola and Mattson88). Potential toxicity of high doses of polyphenols should be always kept in mind, as over-the-counter supplements (such as resveratrol, curcumin, quercetin or various plant extracts) are widely available. The lesson learned from the antioxidant supplementation studies that ‘natural’ does not always mean ‘safe in high doses’ should be well remembered. As new strategies to increase the bioavailability of polyphenols are developing rapidly(Reference Lewandowska, Szewczyk and Hrabec89), safety and toxicity should be carefully investigated. The topic of safety and toxicity(Reference Yates, Erdman and Shao90) becomes even more important when we take into account the inter-individual variability in response, which will be discussed in the following sections.
Effects of polyphenols on cardiometabolic health and cardiometabolic risk factors
Human intervention studies
Following the pioneering study of Hertog et al. (Reference Hertog, Feskens and Hollman59), which demonstrated an inverse association between flavonoid intake and mortality from CHD, numerous human studies have been conducted to explore the impact of consumption of polyphenols on cardiometabolic health. In this type of studies, specific biomarkers should be clearly identified and precisely measured. It is above the scope of this review to analyse in detail this large body of scientific evidence. Therefore, in this paper, we present only several relevant human studies (Table 1). ‘Cardiometabolic’ is a relatively new term that encompasses a wide spectrum of cardiovascular and metabolic diseases, including CHD, non-alcoholic fatty liver disease, the metabolic syndrome and type 2 diabetes. These diseases are the leading cause of morbidity and mortality worldwide. They all share common risk factors such as overweight/obesity, inflammation, increased blood pressure and dyslipidaemia(Reference Guo, Moellering and Garvey91). Knowing that diet and lifestyle largely determine the risk for cardiometabolic diseases, one of the main goals in promoting the cardiometabolic health is the development of preventive health programmes, such as adoption of healthy dietary habits by the majority of population. Moreover, personalised nutrition could be an effective strategy for prevention and treatment of cardiometabolic diseases in future, either at larger population groups or at individual level.
Table 1. Overview of the protective effects of polyphenols on cardiometabolic health – evidence from human studies

Cardioprotective effects of polyphenols have been extensively studied. In an acute study(Reference Bondonno, Yang and Croft92), it has been demonstrated that flavonoid-rich apple improves plasma nitric oxide status and endothelial function (measured as flow-mediated dilatation) and lowers systolic blood pressure. These outcomes are among the most important determinants of cardiovascular health. One of the most convincing evidence for cardioprotective effects of dietary flavonoids has also been related to their beneficial effects on endothelial function, as demonstrated in a meta-analysis conducted in 2008(Reference Hooper, Kroon and Rimm105). In that sense, the most convincing effect was observed for cocoa flavonoids. Polyphenols have been pointed out as a promising nutritional approach for prevention and treatment of hypertension. Indeed, a large cross-sectional study demonstrated a negative correlation between total polyphenol intake and blood pressure, both systolic and diastolic(Reference Medina-Remon, Zamora-Ros and Rotches-Ribalta93). Similarly, regular consumption of cocoa product rich in cocoa fibre significantly decreased the systolic and diastolic blood pressure in moderately hypercholesterolaemic humans(Reference Sarria, Mateos and Sierra-Cinos94). Another important determinant of cardiovascular (and more broadly, cardiometabolic) health is the level of systemic inflammation of low intensity, which is clinically assessed using plasma C-reactive protein as a biomarker, preferably with a method of high sensitivity (hs-CRP). In that sense, a recent meta-analysis of RCT demonstrated that resveratrol significantly decreases serum CRP levels(Reference Haghighatdoost and Hariri106), thus potentially decreasing the cardiovascular risk.
Cardioprotective effects of nutritional polyphenols are also mediated through their beneficial influence on circulating lipoproteins. As such, nutritional polyphenols exhibit a potential to decrease the atherogenic risk. The effect of epigallocatechin gallate on LDL-cholesterol was summarised in a recent systematic review, demonstrating a significant reduction of plasma LDL-cholesterol in healthy individuals with epigallocatechin gallate intake between 107 and 856 mg/d(Reference Momose, Maeda-Yamamoto and Nabetani107). It has also been demonstrated that regular consumption of cocoa increases HDL-cholesterol and decreases oxidised LDL in elderly at high risk for CVD(Reference Khan, Monagas and Andres-Lacueva95). Similarly, regular consumption of flavanol-rich cocoa products has been found to increase HDL-cholesterol in both normocholesterolaemic and moderately hypercholesterolaemic free-living subjects(Reference Sarria, Martinez-Lopez and Sierra-Cinos96,Reference Martinez-Lopez, Sarria and Sierra-Cinos97) . In addition, increase in HDL-cholesterol and decrease in plasma-oxidised LDL are linear with the phenolic content of olive oil(Reference Covas, Nyyssonen and Poulsen98). Dyslipidaemia and oxidative stress, which are well-defined risk factors for atherosclerosis, are also decreased by diet containing products naturally rich in polyphenols(Reference Annuzzi, Bozzetto and Costabile99).
Effects of dietary polyphenols on the metabolic component of the cardiometabolic risk factors have also been explored by many researchers. Indeed, the beneficial effects of dietary polyphenols on insulin sensitivity, glucose tolerance and insulin secretion may potentially decrease the risk of type 2 diabetes. In that sense, it has been demonstrated that strawberry and cranberry polyphenols improve insulin sensitivity in overweight and obese non-diabetic, insulin-resistant persons(Reference Paquette, Medina Larque and Weisnagel100) and that polyphenols in general decrease blood glucose response during the oral glucose tolerance test in individuals at high cardiometabolic risk(Reference Bozzetto, Annuzzi and Pacini101). Consumption of flavanol-rich dark chocolate(Reference Grassi, Lippi and Necozione102) and supplementation with epicatechin(Reference Dower, Geleijnse and Gijsbers103) improve insulin sensitivity in healthy subjects. Importantly, numerous epidemiological studies and RCT have demonstrated that consumption of green tea, red wine and cocoa lowers the risk of type 2 diabetes(Reference Martin, Goya and Ramos108). In addition, higher consumption of anthocyanins and anthocyanin-rich fruit has also been associated with lower risk of type 2 diabetes(Reference Wedick, Pan and Cassidy104).
Accumulated scientific evidence about the effects of cocoa flavanols and polyphenols in olive on specific aspects of human health was analysed by European Food Safety Authority Panel on Dietetic Products, Nutrition and Allergies. Regarding the cocoa flavanols(109,110) , the Panel endorses the health claim that they ‘help maintain endothelium-dependent vasodilatation which contributes to healthy blood flow’(110), and regarding the polyphenols in olive, the Panel endorses that ‘consumption of olive oil polyphenols contributes to the protection of blood lipids from oxidative damage’(111). Notably, although many human intervention studies demonstrated beneficial effects of polyphenols on cardiometabolic health, and predominantly on cardiometabolic risk factors, there are also studies that reported lack of expected outcomes. For example, red wine polyphenols did not improve obesity-associated insulin resistance(Reference Woerdeman, Del Rio and Calani112), and regular consumption of polyphenol-rich apple failed to influence endothelial function in hypercholesterolaemic adults(Reference Auclair, Chironi and Milenkovic113). Considering these and other similar findings, it is essential to understand that many aspects should be taken into account in order to predict the effects of dietary polyphenols on cardiometabolic health. The chemical variety of polyphenols, as well as the variety of their nutritional sources and food matrices, largely influences the effects of polyphenols in humans. In addition, the inter-individual variability in bioavailability (which is governed by absorption, distribution, metabolism and excretion), as well as the inter-individual variability in bioactivity of (the bioavailable) dietary polyphenols, undoubtedly play a pivotal role(Reference Manach, Milenkovic and Van de Wiele114). Besides, the inconsistency of results from human studies might be influenced, at least in part, by the heterogeneity of study populations, study designs and intervention periods.
Inter-individual variability in response to consumption of polyphenols
Ideally, dietary recommendations for intake of polyphenols (and plant food bioactives in general) should be determined by their (a) bioavailability and (b) bioactivity, taking into account the inter-individual variability. In the first place, the inter-individual variability of absorption, distribution, metabolism and excretion affects the bioavailability of polyphenols. Further, the bioactivity of the bioavailable polyphenols (and/or their metabolites) is most likely additionally affected by the inter-individual variability of their cellular molecular metabolism in the target tissues, as well as the inter-individual variability of the cellular processes they modulate. All these factors together affect the inter-individual variability in response to consumption of polyphenols. Furthermore, age, sex, health status, genetics and epigenetic factors have been suggested as the main determinants of the inter-individual variability of response(Reference Milenkovic, Morand and Cassidy115). However, the specific contribution of each of these factors is not completely understood. Indeed, we are at the very early stage of the research in this field. The information we currently have about the inter-individual variability was mainly generated during the post hoc analyses, not from the studies that had initially been designed to study this subject. This issue should be well addressed in future.
To obtain more information about the inter-individual variability in cardiometabolic response of consumption of nutritional polyphenols, four meta-analyses were conducted recently, using the available data from the literature. The meta-analysis of the cardiometabolic health effects of flavonols confirmed that they improve plasma lipid status and decrease systolic and diastolic blood pressure and plasma glucose. Deeper study of the available data, aiming to address the inter-individual variability, demonstrated that these effects are more pronounced in participants with diagnosed disease or dyslipidaemia(Reference Menezes, Rodriguez-Mateos and Kaltsatou116). The meta-analysis on flavanols also confirmed that they beneficially modulate several cardiometabolic risk factors, such as plasma lipids, BMI and waist circumference. However, higher efficacy was demonstrated in the participants with BMI ≥ 25 kg/m2 and in non-medicated individuals, revealing the inter-individual variability(Reference Gonzalez-Sarrias, Combet and Pinto117). Similarly, the beneficial effects of ellagitannins and anthocyanins on selected cardiometabolic risk factors were found to be more prominent in overweight and obese subjects(Reference Garcia-Conesa, Chambers and Combet118), and the effects of hydroxycinnamic acids may be greater in individuals at higher cardiometabolic risk(Reference Martini, Chiavaroli and Gonzalez-Sarrias119).
These meta-analyses are of high importance because they introduce and emphasise the significance of the inter-individual variability in response to consumption of dietary polyphenols to the scientific community. However, their main limitations were the following: (a) unavailability of individual data, pre- and post-intervention and (b) low number of studies. Importantly, even though facing these limitations, the authors were able to demonstrate significant inter-individual variability of several crucial cardiometabolic risk factors, which opens perspectives for further research in the field. However, in order to be able to conduct more robust meta-analyses in future, availability of individual data should be encouraged, of course in compliance with ethical standards. Understanding the factors that determine the inter-individual variability in response to consumption of polyphenols will lead us towards tailoring of personalised dietary recommendations, at both population group and individual level.
Conclusion and perspective
In this review, we aim to address the key aspects of the impact of polyphenols on human cardiometabolic health, historically and in perspective, as summarised in Fig. 1. Historically, there was a tendency to attribute the positive effects of polyphenols on human cardiometabolic health exclusively to their free radical scavenging capacity. Nowadays, we understand that they express their antioxidant activity mainly through modulation of transcription of genes involved in antioxidant defence and detoxification. Apart from their antioxidant activity, polyphenols also contribute to the cardiometabolic health by modulating a plethora of cellular processes that are not directly associated with the antioxidant enzymes, which are subject to extensive research. In the cells, dietary polyphenols and/or their metabolites are present at low concentrations. Administered at very high concentrations, polyphenols may cause toxic effects in humans. Informed about the beneficial effects of polyphenols on human cardiometabolic health, the temptation among the general population for taking supplements of pure compounds or plant extracts, which are readily available as over-the-counter pharmaceutical forms, is realistic. However, the vigilance for their potential toxicity, if taken at high doses, should remain high.
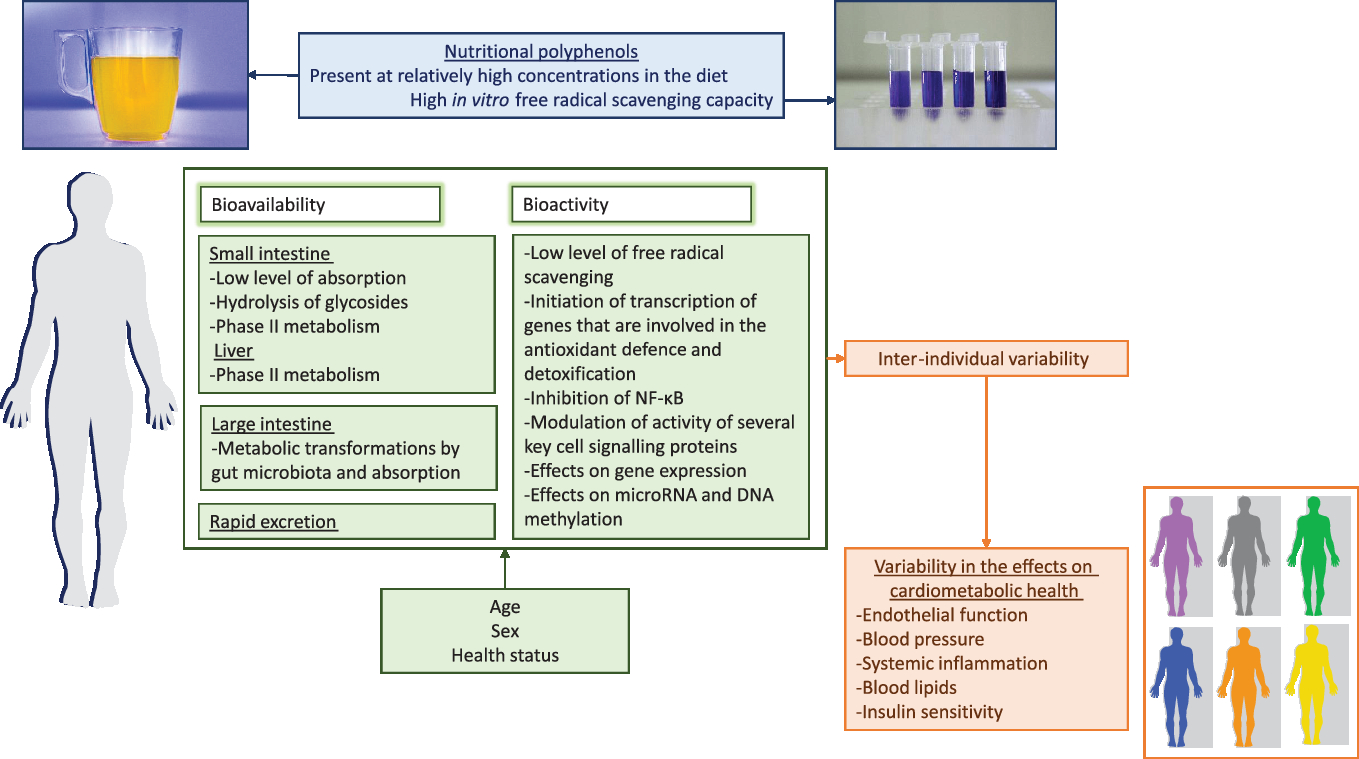
Fig. 1. Polyphenols in the human diet – in vitro and in vivo.
Growing scientific evidence demonstrates the beneficial effects of dietary polyphenols on human cardiometabolic health. Numerous RCT report improvement of the key biomarkers of cardiometabolic health such as blood pressure, flow-mediated dilatation, blood lipids, blood glucose and insulin sensitivity. The inconsistency of the results of some studies triggered interest towards post hoc analysis of individual data, which led to identification of the inter-individual variability in the response. Resolving the puzzle of the inter-individual variability will lead us towards personalised dietary recommendations, with ultimate goal to decrease the risk for cardiometabolic diseases and preserve the health for better ageing. For this purpose, future studies shall be specifically designed to address the various aspects of the inter-individual variability in response to consumption of polyphenols and plant food bioactives in general.
Acknowledgements
The authors are members of COST Action FA1403 – POSITIVe ‘Interindividual variation in response to consumption of plant food bioactives and determinants involved’ supported by COST (European Cooperation in Science and Technology, http://www.cost.eu/).
The authors thank Kosta Zamanovski and Ljubica Janeska for original photographs and figure elements.
This research received no specific grant from any funding agency, commercial or not-for-profit sectors.
T. R., V. M. and D. M. conceived the structure, wrote the manuscript and approved the final version.
There are no conflicts of interest.