The behavioural impact of consuming the n-3 PUFA DHA and EPA in the diet has received a great deal of attention in recent years. The effect of n-3 PUFA deficiency in animals is well documented; an inadequate supply of dietary n-3 PUFA can result in a 50–80 % decline in brain DHA and is associated with impaired learning and memory (reviewed in Moriguchi & Salem(Reference Moriguchi and Salem1)). Such data are not readily translated into humans where equivalently low levels of n-3 PUFA are rare. However, there is evidence to suggest that lower n-3 PUFA status (compared with controls and measured by plasma/serum or erythrocyte concentrations) is a risk factor for a plethora of adverse health outcomes including CVD(Reference Mori and Woodman2), inflammatory disease(Reference De Caterina and Basta3) and many neurodevelopmental and psychiatric conditions such as attention deficit hyperactivity disorder, dyslexia, depression, schizophrenia and dementia (reviewed in Bourre(Reference Bourre4)).
There is also some evidence to suggest that n-3 PUFA status may be linked to cognitive function in healthy individuals. It is well known that n-3 PUFA are essential for optimal fetal development(Reference Innis5), and higher oily fish consumption in pregnancy is associated with better developmental outcomes in children at 4 years of age(Reference Hibbeln, Davis and Steer6, Reference Helland, Smith and Saarem7). In addition, a prospective epidemiological study from Sweden revealed that incorporating fish meals in the diet on a regular basis is associated with better cognitive performance in adolescents (18 years) regardless of socio-economic background(Reference Aberg, Aberg and Brisman8). Further, epidemiological studies(Reference Heude, Ducimetiere and Berr9–Reference Morris, Evans and Tangney11) in older adults have suggested that increased intake of n-3 PUFA is associated with a reduced risk of cognitive decline. The consumption of n-3 PUFA is generally low in nations following a typical ‘Western’ diet, and in the UK specifically, consumption of oily fish, the predominant dietary source of n-3 PUFA, is just a third of recommended levels(12). Therefore, the investigation of the effects of increased intake of n-3 PUFA on cognitive function is a logical avenue of research to pursue. A number of intervention studies in both children(Reference Kennedy, Jackson and Elliott13–Reference Ryan and Nelson17) and older adults(Reference Dangour, Allen and Elbourne18, Reference Yurko-Mauro, McCarthy and Rom19) have addressed this question with mixed results, but there are few available data describing the effects of n-3 PUFA on cognitive function in healthy younger adults ( < 50 years of age). Hamazaki et al. (Reference Hamazaki, Sawazaki and Itomura20) reported that 1·5–1·8 g/d DHA (from fish oil (FO)) for 3 months in forty-one healthy students prevented increases in aggression towards others during times of mental stress (university examinations), but did not engender performance differences in comparison with the control group on the Stroop or the dementia-detecting test adapted for this age group. In two other studies, the effects of 4 weeks' dietary supplementation with 4 g FO on cognitive performance and mood have been examined. Fontani et al. (Reference Fontani, Corradeschi and Felici21) reported that participants administered FO achieved faster reaction times on a Go/No-Go task and a Sustained Attention task, and reported improved ratings of anger, anxiety, fatigue, depression, confusion and vigour on the self-report Profile of Mood States questionnaire compared with their pre-treatment scores. However, in this study, no comparison was made between the treatment and placebo groups. On the other hand, Antypa et al. (Reference Antypa, Van der Does and Smelt22) found no effect of treatment on any of the cognitive measures that they employed including attention, memory, response inhibition and emotion recognition; the only reported effect on mood was a small effect on self-rated mental fatigue (Profile of Mood States).
From these studies, it is clear that any effects of n-3 PUFA supplementation on behavioural outcomes in healthy young adults remain to be adequately addressed. Animal studies have suggested that the intervention period of both of the latter studies would not be sufficient for adequate incorporation of the fatty acids to occur in certain tissues, including the brain(Reference Connor and Neuringer23); therefore, the reported results may not reflect the effects that might occur following a longer intervention period. Further, a recurrent theme in the n-3 PUFA supplementation literature, both in healthy and patient populations, is the use of a wide range of treatment formulations and doses. Both dietary DHA and EPA are known to influence mechanisms that support cognitive function, but given the evidence provided by the studies listed above, it is not possible to identify the properties of an n-3 PUFA supplement, for instance in terms of comparative levels of DHA or EPA, that might have the greatest effect on cognitive performance. Lastly, the previous intervention studies have either only utilised a small number of tasks(Reference Hamazaki, Sawazaki and Itomura20), focused on one cognitive domain(Reference Fontani, Corradeschi and Felici21), or used tasks specifically involving emotional information processing(Reference Antypa, Van der Does and Smelt22). In order to address these issues, the present study assessed the effects of two different formulations of FO in parallel, one with a higher ratio of EPA:DHA and the other with a higher ratio of DHA:EPA, at doses consistent with the current recommended daily intake of oily fish, across a range of cognitive domains. The aim of the present investigation was therefore to specifically evaluate the effects of 12 weeks' supplementation of DHA-rich FO and EPA-rich FO dietary supplements on cognitive function in healthy young adults maintaining a regular diet containing oily fish not more than once a week. Self-report mood assessments formed the secondary part of the investigation.
Materials and methods
Participants
Volunteers were invited to participate if they declared that they were in good health and that they were a non-smoker, free from prescription, herbal, illicit or recreational drugs (females taking the contraceptive pill were included), n-3 supplements, that they were a native English speaker and eating no more than one portion of oily fish a week on average (less than or equal to four portions per month). All volunteers were either students attending Northumbria University or were university graduates living in Newcastle upon Tyne and the surrounding area. As no previous research has provided adequate statistical information required for an accurate power calculation, the treatment groups of fifty participants were arrived at by considering the sample sizes employed in intervention studies in adults with psychiatric disorders, the literature regarding the cognitive effects of natural products in similar cohorts and the resources available.
Of the 159 participants that were enrolled in the study, nine participants did not attend the baseline session and eight participants were excluded on the basis that their declared average oily fish intake exceeded four portions per month. Before the last day of the study (week 12), two participants withdrew from the study. In both cases, the reasons were unrelated to the intervention (glandular fever, moving home). In addition, two participants had below 50 % compliance as they had only taken 1 × 500 mg capsule/d and were excluded from the analysis at the blind data review point. The remaining per-protocol sample consisted of 140 males and females; their demographic details are presented in Table 1.
Table 1 Participants' demographic information and baseline characteristics*
(Mean values with their standard errors and number of subjects)

FO, fish oil.
* P values are given for separate one-way ANOVA that were conducted on these baseline data.
The study was conducted according to the guidelines laid down in the Declaration of Helsinki and all procedures involving human participants were approved by the Northumbria University School of Life Sciences Ethics Committee. Written informed consent was obtained from all participants. This trial was registered at clinicaltrials.gov: NCT01075919.
Treatments
Before the start of the study, a restricted randomisation list matching treatments to participant code numbers was computer generated. Participants were therefore randomly allocated to one of three treatment groups: DHA-rich FO, EPA-rich FO and placebo. The 1 g daily dose was provided by 2 × 500 mg capsules. The active capsules contained 497·5 mg of deodorised FO plus 2·5 mg mixed tocopherols. The total daily dose of n-3 PUFA for DHA-rich FO was 450 mg DHA+90 mg EPA (5:1 DHA:EPA) and for EPA-rich FO, this amount was 300 mg EPA+200 mg DHA (3:2 EPA:DHA) (It is noted that the ratio of EPA:DHA in EPA-‘rich’ FO is closer to 1 compared with DHA-rich FO; however, in this case, EPA-rich denotes containing more EPA than DHA). The total daily dose for the placebo treatment was 1 g olive oil. The ratios of DHA and EPA contained in the active treatments are reflective of the extremes of the range that is found naturally in fish; DHA-rich FO resembles that of yellowfin tuna and herring, and some species of salmon have a similar ratio to that present in EPA-rich FO. Treatment oils were purchased from EPAX AS (Aalesund, Norway) and encapsulated by Cardinal Health UK. All capsules used a brown bovine gelatine casing and all treatments were packaged, labelled and randomised on site by a disinterested third party.
Assessment of cognitive performance and mood
Performance was assessed using the Computerised Mental Performance Assessment System, a software platform for the presentation of classic and bespoke computerised cognitive tasks. The cognitive tasks were presented in the same order as outlined below (cognitive domain in brackets). All presentations were made via a laptop computer with the exception of the Word Recall and Verbal Fluency tasks, for which participants used pen and paper to make their responses. All reaction times were measured in milliseconds; accuracy refers to the percentage of correct responses made.
Word Presentation
A unique set of fifteen words is presented. Words were selected at random from a large bank of words derived from the Medical Research Council Psycholinguistic Database(Reference Fearnley24) matched for word length, frequency, familiarity and concreteness. Stimulus duration was 1 s, as was the inter-stimulus duration.
Immediate Word Recall (episodic memory)
The participant was allowed 60 s to write down as many of the words as possible. The task was scored for the number of correct responses and number of errors.
Picture Presentation
A total of fifteen black-and-white photographic images of objects and outdoor and indoor scenes were presented sequentially on the screen for the participant to remember at the rate of 1 every 3 s, with a stimulus duration of 1 s. The same set of fifteen pictures was presented to each participant in a random order. Participants were informed that there would be a recognition task later in the test session and therefore instructed to remember the details in the pictures as they were presented.
Face Presentation
A set of twelve passport-style photographic images of people were presented sequentially in a random order to participants. A first and last name was assigned to each photograph and presented on the screen underneath the person's face. Stimulus duration was 1 s, with a 3 s inter-stimulus duration. Participants were informed that there would be a recognition task later in the test session and therefore instructed to remember the faces and names that were presented.
Simple Reaction Time (psychomotor speed/attention)
The participant was instructed to press the ‘space bar’ on the laptop keyboard as quickly as possible every time an upward pointing arrow appeared on the screen. A total of fifty stimuli were presented with an inter-stimulus duration that varied randomly between 1 and 3·5 s. Mean reaction time was recorded.
Choice Reaction Time (attention/response inhibition)
An arrow appeared on the screen pointing to the left or to the right. Participants responded with a left or right key press corresponding to the direction of the arrow. There was a randomly varying inter-stimulus interval of between 1 and 3 s for a total of fifty stimuli. Accuracy and mean reaction time were recorded.
Four-Choice Reaction Time (attention)
A visual representation of the four direction arrow keys of a standard keyboard was presented on the screen. The arrows ‘lit up’ at random on the screen until the corresponding key press was made. In all, each arrow was the target stimulus twelve times, forming a total of forty-eight stimuli for this task. Accuracy and mean reaction time were recorded.
Stroop Task (attention/response inhibition)
A computerised version of the Stroop Task(Reference Stroop25) was created. Words describing colours (GREEN, BLUE, RED, YELLOW) were randomly presented in incongruently coloured text (e.g. GREEN was presented in blue text, etc.). For each of the fifty stimulus presentations, participants were instructed to use the computer mouse and cursor to click the colour box located on the right side of the screen that matched the colour of the text the word was presented in. Accuracy and mean reaction time were recorded.
Verbal Fluency (executive function/semantic memory)
Participants were presented with a letter on the screen and were given 60 s to write down as many words as they could beginning with that letter. The standard letters for this task – F, A, S – were presented at baseline and C, M, T were presented at week 12, which were matched for beginning-of-word frequency(Reference Lewand26). An overall ‘Verbal Fluency’ score was calculated by summing the number of permitted words given in each of the three trials.
Numeric Working Memory (working memory)
In this task, five random digits from 1 to 9 were presented sequentially for the participant to hold in memory. This was followed by a series of thirty probe digits (fifteen targets and fifteen distractors) for each of which the participant indicated whether or not it had been in the original series by a simple key press. The task consisted of three separate trials. Accuracy and mean reaction time were recorded.
Alphabetic Working Memory (working memory)
This task was identical to Numeric Working Memory, the only difference being that the stimuli were letters.
Corsi Blocks Task (spatial working memory)
In this task, nine identical blue squares appeared on the screen in non-overlapping random positions. A set number of blocks changed colour from blue to red in a randomly generated sequence. The cursor was locked in position until the entire sequence had been presented, at which point the participants were instructed to repeat the sequence by clicking on the blocks using the mouse and cursor. The task was repeated five times at each level of difficulty. The sequence span increased from four upwards, until the participant could no longer correctly recall the sequence, resulting in a span measure of non-verbal working memory, calculated by averaging the level of the last five correctly completed trials.
Three-Back Task (working memory)
A continuous string of letters (upper and lower case; inter-stimulus interval of 2·5 s) was presented; forty-five letters in total with fifteen target pairs. For each stimulus, participants were instructed to indicate whether this was the same letter that appeared three letters before. Accuracy and mean reaction time were recorded.
Telephone Number Working Memory Task (working memory)
A nine-digit ‘telephone number’ was presented on the screen for 5 s which participants were instructed to hold in their memory. After a delay of 10 s, participants had to input the number using the numerical numbers at the top of the computer keyboard. Participants scored correctly only if the entire nine-digit number was entered exactly as the number that was presented. There were eight trials in total. Accuracy and mean total time taken to complete the trials were recorded.
Delayed Word Recall (episodic memory)
The participant was again given 60 s to write down as many of the words presented previously as possible. The total number of correct responses was recorded.
Delayed Word Recognition (episodic memory)
The original fifteen words plus fifteen distractor words were presented one at a time in a random order. For each word, the participant indicated whether or not it was included in the original list of words by pressing appropriate ‘yes’ and ‘no’ keys as quickly as possible. Stimuli remained on the screen until an appropriate response had been made. Accuracy and mean reaction time were recorded.
Delayed Picture Recognition (episodic memory)
The original fifteen pictures plus fifteen distractor pictures were presented one at a time in a randomised order. For each picture, participants indicated whether or not it was recognised as being from the original series by pressing appropriate ‘yes’ and ‘no’ keys as quickly as possible. Stimuli remained on the screen until an appropriate response had been made. Accuracy and mean reaction time were recorded.
Names-to-Faces Recall (episodic memory)
The twelve original photographs presented at the outset were again presented on the screen, one at a time. Underneath each picture, there was a list of four different first names and four different last names. For each photograph, participants were instructed to choose the first and last name that was originally presented with the photograph. The number of correct responses for the first and last names was recorded and collapsed to give an overall score for this task.
Cognitive demand battery
The second half of the testing session comprised three repetitions of a 10-min computerised ‘cognitive demand battery (CDB)’. The battery has four components: serial 3 subtractions (2 min), serial 7 subtractions (2 min), rapid visual information processing (RVIP, 5 min) and a ‘mental fatigue’ visual analogue scale. For the serial subtraction tasks, participants subtracted either 3 or 7 consecutively from an original randomly generated number between 800 and 999 for the duration of the task. The RVIP task required that participants respond with a key press every time they detected three consecutive odd or even numbers in a sequence of rapidly (100/min) presented single digits (1–9). Previously, the battery has been effectively used to investigate the effects of various nutritional interventions on cognitive and mental fatigue during periods of sustained cognitive processing (see Reay et al. (Reference Reay, Kennedy and Scholey27) for a full description). For this study, data from the three repetitions of the battery were averaged for each outcome from the battery's four-component tasks.
Assessment of mood
For the assessment of mood, two subjective ratings were taken. A computerised version of the sixteen Bond–Lader visual analogue scales was delivered before the CDB. The results were combined to form three mood factors: ‘alert’, ‘calm’ and ‘content’(Reference Bond and Lader28). Participants also completed the Depression, Anxiety and Stress Scales (DASS). This forty-two-item self-report questionnaire requires participants to rate how much each statement of negative emotional state applied to them during the past week. This measure is designed to assess both current state and change in state over time (see Lovibond & Lovibond(Reference Lovibond and Lovibond29) for a full description).
Procedure
Participants attended the laboratory on three occasions (training day, baseline and week 12). Testing took place in a suite of testing facilities, with participants visually isolated from each other. The training day comprised the obtaining of informed consent, training on the cognitive tasks and mood questionnaires, collection of demographic data and collection of a blood sample. No more than 8 d following the training day, participants then attended the laboratory at 08.30 hours in an overnight fasted state on the first day (baseline) and at the end of the 12-week treatment regimen (week 12: average time on treatment 86·71 (sd 4·97) d). On arrival at the baseline session, participants were randomised to treatment group and completed the full battery of Computerised Mental Performance Assessment System tasks, Bond–Lader visual analogue scales, three repetitions of the CDB and filled in the DASS. Participants' blood pressure and heart rate were subsequently recorded and at the end of the session, each participant received their allocated capsules and were instructed to commence the treatment regimen that day. Participants from whom a blood sample could not be obtained on the training day were invited to attempt to give another sample at the end of this session. The session at week 12 followed exactly the same protocol as at the baseline session, with the exception that all unused capsules were collected before testing, and at the very end of the session, participants were asked to indicate which treatment they thought they had been taking. The assessment schedule is presented in Fig. 1.
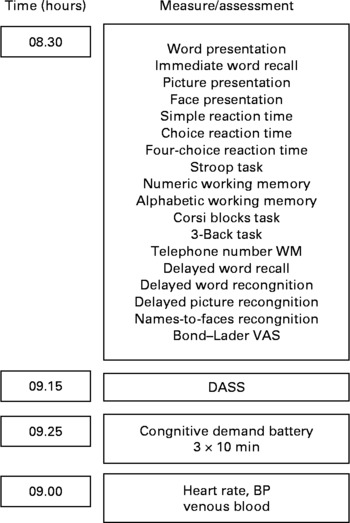
Fig. 1 Baseline and week 12 assessment schedule. The pre-treatment venous blood sample was collected on either the training day or at baseline. Times are approximate. VAS, visual analogue scale; DASS, Depression, Anxiety and Stress Scale; BP, blood pressure; WM, Working Memory.
Blood samples
Participants were required to give two blood samples, the first on either the training day (n 108) or at the end of the baseline session (n 3) before supplementation and again at week 12 (n 99) after completing all the behavioural tasks. Blood samples were collected by venepuncture into a serum gel monovette (4·9 ml). The samples were immediately centrifuged at 3000 g for 10 min at 20°C using an Allegra X-22 centrifuge (Beckman Coulter, High Wycombe, Bucks, UK). The serum was decanted into a 1·5 ml microtube and immediately frozen at − 80°C before analysis.
Methylation and extraction of the serum fatty acids was carried out using a modified version of the method described by Masood et al. (Reference Masood, Stark and Salem30). The procedure consisted of adding the following components, in order, to a screw-top glass tube: 0·1 ml methyltricosanoate (internal standard; Fluka, Sigma-Aldrich, Gillingham, Dorset, UK), 0·1 ml of blood serum sample and 1·8 ml of an acetyl chloride–methanol mix (1:25, prepared immediately before the start of the analysis; the methanol contained 50 mg butylated hydroxytoluene/l to prevent fatty acid oxidation). The mixture was swirled to mix and then heated to 100°C for 60 min. After cooling, 2 ml of n-hexane were added to the tube, vigorously mixed for 30 s and the n-hexane layer, containing the derivatised fatty acid methyl esters (FAME), was then transferred to a 2 ml micro-reaction vessel (Supelco, Sigma-Aldrich, Gillingham, Dorset, UK) using a Pasteur pipette. A further 1 ml of n-hexane was added to the tube and the process was repeated. The n-hexane in the vials was then evaporated to dryness under a N2 flow at 40°C, and the residue was resuspended in 0·1 ml n-hexane.
Analysis was carried out using a Thermo Scientific Focus gas chromatograph fitted with a 30 m DB5 column (0·25 mm in internal diameter) and a dual-stage quadrupole mass spectral detector operating in the single-ion monitoring mode to maximise sensitivity (GC conditions were as follows: carrier gas, He; flow rate, 1·5 ml/min; initial temperature, 140°C, hold for 5 min, ramp to 280°C at 4°C/min, hold for 2 min). All components had eluted by 35 min. The split ratio was 7:1 and the injection port temperature 270°C. Individual FAME concentrations were calculated from the ratio of peak areas for the component and the internal standard, corrected for the previously determined relative response factor of the component and expressed as a weight percentage (i.e. mg per 100 mg) of the total FAME concentration (excluding the internal standard).
Serum fatty acid data are presented in Table 2.
Table 2 Physiological measures by treatment group at baseline and week 12
(Mean values with their standard errors and number of subjects)

LA, linoleic acid; FO, fish oil; AA, arachidonic acid; ALA, α-linolenic acid; SBP, systolic blood pressure; DBP, diastolic blood pressure.
Mean values were significantly different from those of baseline concentrations: *P < 0·05, **P < 0·01, ***P < 0·005 (paired-samples t test).
Statistical methods
Data were analysed by one-way between-subject (treatment group) ANCOVA, with data from the relevant outcome measure at baseline as the covariate. A priori planned comparisons were made between placebo and both the active treatments (DHA-rich FO and EPA-rich FO) utilising t tests with the mean squares error from the ANCOVA as an error term and adjusted means(Reference Keppel31). To ensure the overall protection level against type I errors, comparisons were strictly planned before the commencement of the study and only those comparisons associated with a significant main effect on the omnibus ANCOVA were calculated. No correction was applied for family-wise error rate as each separate analysis addressed a specific experimental question made before testing. All testing was two-tailed and all data were analysed using SPSS 16.0 (SPSS, Inc., Chicago, IL, USA).
Baseline characteristics
Results from separate between-group (treatment condition) ANOVA using data collected on either the training day or baseline session revealed no differences between participants in all three treatment groups on age, BMI, oily fish consumption (see Table 1), and serum concentrations of DHA and EPA (see Table 2). Additionally, no between-group differences were detected on having been breast-fed, white fish consumption, and any of the family background questionnaire items, with the exception of the mother's level of education. For this item, the ANOVA revealed a significant main effect of treatment group (F(2,137) = 3·91, P = 0·022). Consequently, all of the analyses were duplicated with the mother's level of education as a second covariate. However, the results from both analyses were identical with regard to the main effects, so, for brevity, the analysis including the mother's level of education is not reported.
Missing data
Data capture errors led to a reduced number of datasets for the Corsi Blocks Task and two participants' data were lost from the entire CDB due to a fire drill during their baseline testing session. During the data cleaning process (conducted before treatment unblinding), a small number of instances were identified and removed wherein the participant had failed to follow the task instructions correctly (e.g. by responding to a task with the incorrect button). The subsequent reduced datasets for Stroop, serial 7 subtractions and RVIP tasks are indicated in Tables 3 and 4. For the subsequent ANCOVA that were carried out on the outcomes from these tasks, missing data were omitted using list-wise deletion.
Table 3 Cognitive task performance and mood outcomes by treatment group at baseline and week 12
(Mean values with their standard errors and number of subjects)

FO, fish oil; RT, reaction time; DASS, Depression, Anxiety and Stress Scale.
* Mean value was significantly different from that of the placebo treatment (P < 0·05).
† From the primary ANCOVA.
Table 4 Outcome measures on the cognitive demand battery by treatment group at baseline and week 12
(Mean values with their standard errors and number of subjects)

FO, fish oil; RVIP, rapid visual information processing; RT, reaction time.
** Mean value was significantly different from that of the placebo treatment (P < 0·01).
† From the primary ANCOVA.
Results
Physiological data and compliance
Results for compliance as measured by capsule count were similar in all three treatment conditions (91 % DHA-rich FO, 90 % EPA-rich FO and 92 % placebo). In addition, the results of the paired-sample t tests shown in Table 2 reveal that serum concentrations of DHA and EPA increased significantly in both treatment groups but not in the placebo group, indicating good adherence to the treatment regimen.
In a separate set of analyses, data from the serum samples collected at week 12 were analysed by one-way ANCOVA, with relevant serum data collected at baseline as the covariate to investigate the presence of between-group differences on serum PUFA following the intervention. A significant main effect of treatment group was revealed for serum concentrations of both DHA and EPA (F(2,85) = 18·10, P < 0·001; F(2,84) = 3·24, P = 0·044, respectively). Post hoc calculations (Bonferroni) revealed that participants in the DHA-rich FO group had higher serum DHA than those in the placebo (P < 0·001) and EPA-rich FO groups (P = 0·022). The participants in the EPA-rich FO group also had higher serum DHA than those in the placebo group (P = 0·004). Interestingly, with regard to serum EPA concentrations, post hoc calculations did not reveal any significant differences between either treatment group and placebo.
The same analysis was applied to the arachidonic acid (AA):EPA ratio data and a significant main effect of group was also detected (F(2,84) = 33·61, P < 0·001). The ratio of AA:EPA was higher in the placebo group than in both the DHA-rich FO (P < 0·001) and EPA-rich FO (P < 0·001) groups. There was no significant difference between the active treatment groups on the ratio of AA:EPA.
At the end of the week 12 session, participants were asked to say if they thought they had been taking FO or placebo capsules; participants were just as likely to give the correct answer in the EPA-rich FO treatment group (49 %) as those taking placebo (66 %) (χ2 (1) = 0·08, P = 0·109), whereas those in the DHA-rich FO group were less likely (37 %) to give the correct answer (χ2 (1) = 0·003, P = 0·006).
With regard to the other physiological measures that were taken, there was no evidence of an effect of treatment on either blood pressure or heart rate. These data are also presented in Table 2.
Cognitive performance battery (Computerised Mental Performance Assessment System)
There was evidence of a significant main effect of treatment on two of the outcome measures: reaction time on the Stroop Task (F(2,133) = 3·27, P = 0·041) and the number of correctly matched items on the Names-to-Faces Task (F(2,136) = 3·73, P = 0·026). Planned comparisons revealed that participants in the DHA-rich FO group were faster on the Stroop Task compared with placebo (t(133) = 2·50, P = 0·014, r 0·26) but participants in both the DHA-rich and EPA-rich FO groups matched fewer items on the Names-to-Faces Task (t(136) = 2·00, P = 0·047, r 0·21; t(136) = 2·52, P = 0·013, r 0·26, respectively). Performance data collected at baseline and week 12 for every outcome can be found in Table 3.
Cognitive demand battery
Performance data collected at baseline and week 12 on the CDB can be found in Table 4.
There was no evidence of a main effect of treatment on any of the cognitive performance measures from the CBD. However, a main effect of treatment was detected for self-reported mental fatigue during the CDB (F(2,134) = 4·36, P = 0·015). Planned comparisons revealed that mental fatigue scores for participants in the EPA-rich FO group were significantly lower compared with placebo (t(134) = − 2·67, P = 0·009, r 0·27).
Mood
There was no effect of treatment on either the Bond–Lader visual analogue scales or the DASS. The scores from both these measures can be found at the bottom of Table 3.
Discussion
In the present study, the effects of 12 weeks' supplementation with DHA-rich and EPA-rich FO in a sample of healthy adults who were recruited simply on the basis of not consuming a high amount of oily fish (less than or equal to one portion of oily fish a week on average) were explored. Given the number of different tasks, along with the small number of significant outcomes that were detected and the bidirectional nature of the results, these findings do not provide any solid evidence of cognitive or mood (Bond–Lader, DASS) enhancement following supplementation with FO at a dose that is roughly equivalent to the current UK recommendation(32).
Based on these findings, one possible interpretation could be that even though three-quarters of the present sample reported consuming less oily fish than the current recommendation, and despite the fact that serum concentrations of DHA and EPA were similarly low(Reference Antypa, Van der Does and Smelt22) or even slightly lower than those that have been observed in normal healthy participants in previous studies(Reference Rogers, Appleton and Kessler33, Reference Morse34), endogenous n-3 PUFA levels may not have been below the level that would predispose the participants to performance deficits, and render supplementation effective or necessary. In particular, one study in animals does support the idea of a threshold of n-3 PUFA intake (and subsequent cellular incorporation), below which performance is adversely affected. Jensen et al. (Reference Jensen, Skarsfeldt and Hoy35) compared the effects of four different diets on brain phospholipids and performance on the Morris Water Maze task in rats following four generations of breeding, adhering to the dietary interventions. These authors have reported that when brain levels of n-3 PUFA (DHA+docosapentaenoic acid) fell to 22 % (placebo), performance on the Morris Water Maze task was worse, with significantly longer escape latencies compared with rats fed vegetable and marine oils, in which levels of n-3 PUFA were 24 and 27 %, respectively. Although a similar threshold has yet to be established in humans, one study conducted in undernourished children reported beneficial effects of a FO intervention(Reference Dalton, Wolmarans and Witthuhn16), compared with those in well-nourished children, which have reported no effect of treatment on cognitive or other performance outcomes(Reference Kennedy, Jackson and Elliott13, Reference Kirby, Woodward and Jackson14, Reference Ryan and Nelson17), despite evidence of improved fatty acid profiles following the intervention(Reference Kirby, Woodward and Jackson14, Reference Ryan and Nelson17).
Another possibility is that, apart from having a lower intake of n-3 PUFA, another important factor when considering the effects of n-3 PUFA supplementation in otherwise healthy samples is the presence of performance decrements at the outset. For example, two recent publications described the effects of n-3 PUFA supplementation in healthy older adults who were not suffering from cognitive impairment or dementia. A key difference between these studies was that in one study, participants were selected on the basis of self-reported memory complaint and identified as having age-related cognitive decline(Reference Yurko-Mauro, McCarthy and Rom19), as opposed to the other study, in which participants were not suffering from any decline(Reference Dangour, Allen and Elbourne18). Although the intervention period was longer for the latter study (24 months), no effects of the treatment were observed, compared with improvements on a measure of learning and episodic memory (Paired-Associate Learning task) in the sample suffering cognitive decline after 6 months' supplementation in the study reported by Yurko-Mauro et al. (Reference Yurko-Mauro, McCarthy and Rom19). Similarly, an interesting secondary analysis of the data presented by Dalton et al. (Reference Dalton, Wolmarans and Witthuhn16) revealed that the effects of a DHA-rich fish flour spread given to schoolchildren for 6 months were more pronounced in children whose baseline performance scores were lower(Reference Smuts, Dalton and Wolmarans36), again suggesting that any effects of n-3 PUFA supplementation in healthy unimpaired samples might only be observed when performance is below average to begin with. This may certainly explain the recent string of null results from this and other studies that have investigated the effects of added n-3 PUFA in healthy participants, even when these samples are not consuming the recommended levels of oily fish(Reference Kennedy, Jackson and Elliott13–Reference Osendarp, Baghurst and Bryan15, Reference Ryan and Nelson17, Reference Dangour, Allen and Elbourne18).
A final possibility is that the dose of n-3 PUFA provided by the active treatments (DHA-rich FO: 450 mg DHA+90 mg EPA; EPA-rich FO: 300 mg EPA+200 mg DHA) was too low for an effect to be observed, or alternatively that the intervention period was too short. However, reports from similar intervention trials in healthy adults that have administered higher doses of n-3 PUFA over a longer period than the present study have also yielded null results(Reference Dangour, Allen and Elbourne18, Reference Antypa, Van der Does and Smelt22, Reference Rogers, Appleton and Kessler33). Therefore, overall, it must be concluded at present that the available data do not provide any evidence that supplementation can enhance cognitive function in healthy populations. This is not to say that n-3 PUFA have no effect on overall brain function despite the absence of performance benefits. Data from our own laboratory(Reference Jackson, Reay and Scholey37) and McNamara et al. (Reference McNamara, Able and Jandacek38) reveal that changes in the functional activation of areas of the brain following supplementation over short periods (8–12 weeks) are not necessarily accompanied by simultaneous changes on performance measures. It is possible that such functional changes, i.e. increased activation and cerebral blood flow, could help explain the epidemiological data that show a positive association between fish consumption and cognitive function(Reference Hibbeln, Davis and Steer6–Reference Morris, Evans and Tangney11). Naturally, these hypotheses require further investigation and comprise a number of potential future lines of investigation.
In the present study, one significant finding that may merit further discussion is the beneficial effect of EPA-rich FO on self-reported mental fatigue during 30 min of cognitively demanding tasks. Previous reports have found reduced ratings of mental fatigue on the Profile of Mood States following FO supplementation for 4 weeks in healthy adults(Reference Fontani, Corradeschi and Felici21, Reference Antypa, Van der Does and Smelt22). Further, it is known that increased intake of EPA modulates the production of pro-inflammatory AA-derived eicosanoids(Reference Ferrucci, Cherubini and Bandinelli39) and, at least at the epidemiological level, increased levels of pro-inflammatory cytokines are positively associated with tiredness(Reference Whalley, Jacobs and Hyland40), suggesting that a reduced AA:EPA ratio may be particularly relevant in times of high cognitive demand. However, it is notable that reduced mental fatigue was not observed in the DHA-rich FO treatment group where the serum AA:EPA ratio was also significantly lower than that of the placebo group. Future research could investigate the relationship between self-reported mental fatigue and these biomarkers, or evaluate the efficacy of EPA-rich FO for alleviation of symptoms of mental and physical fatigue, in populations who subjectively report fatigue, such as individuals in full-time work, primary caretakers of children and carers, for example.
With regard to the physiological effects of the treatments, both DHA-rich and EPA-rich FO were effective at significantly increasing serum DHA (450 and 200 mg, respectively) over placebo. In contrast, there was no significant difference in serum EPA between placebo and either treatment group at week 12, which may be rectified in future studies by providing a higher dose of EPA. The observed changes in fatty acid concentrations in the placebo group may be a consequence of measuring serum fatty acids, reflective only of dietary intake in the prior 4 weeks, as opposed to whole blood analysis which provides information on intake in the previous 3–4 months. With regard to the other physiological measures, there was no evidence of an effect of either of the active treatments on heart rate or blood pressure. This latter finding is in agreement with a meta-analysis reported by Morris & Sacks(Reference Morris, Sacks and Rosner41) who found no effect of n-3 PUFA supplements on blood pressure in healthy adults.
A strength of the present study was the full assessment of cognitive function that was employed. The combination of numerous outcome measures analysed using a powerful (i.e. least conservative) statistical approach that resulted in the detection of only a limited number of significant results suggests that a type II error has not been committed. It must also be noted that if corrections for multiple testing had been applied, these results would disappear. Therefore, as no coherent pattern of either positive or negative effects of either active treatment was detected, it can be confidently concluded that the behavioural effects of FO supplements on healthy young adults are minimal. These results are comparable with those reported in normally developing children(Reference Kennedy, Jackson and Elliott13–Reference Ryan and Nelson17) and older adults free from cognitive impairment(Reference Dangour, Allen and Elbourne18). Equivalent findings have also been reported in animal studies which show that rats raised on a balanced and n-3-adequate diet but who are not given any additional n-3 PUFA perform no worse on behavioural tests than animals given the additional n-3 PUFA(Reference de Wilde, Farkas and Gerrits42). It may be that very long periods of supplementation (2+ years) are necessary to show any effects of treatment, which are unfortunately much less feasible to investigate in human studies.
Conclusions
The present study describes a novel investigation where the effects of DHA-rich and EPA-rich FO on cognitive function and mood were investigated in parallel in comparison with placebo. The primary aim of the present study was to assess the effects of n-3 PUFA supplementation in a population where few data are available regarding cognitive performance and mood. A 1 g dose of FO was chosen so that the results could be readily translated into meaningful dietary advice. As such, the data presented here do not favour the use of FO supplements for cognitive or mood enhancement. The results from the present and similar studies in healthy individuals suggest that simply giving more n-3 PUFA does not necessarily result in improved performance, and that the relationship between dietary intake of n-3 PUFA and their effect on the brain, and consequently cognitive function, is far more complex than originally thought. While the results here could not be taken as an encouragement for further investigations of the direct effect of n-3 PUFA supplementation on cognitive function, the use of neuroimaging techniques, even in the absence of behavioural modification, may well be useful in delineating the effects that n-3 PUFA are exerting on overall brain function and physiology. Further, it is probable that ongoing research into the interaction between n-3 PUFA intake and genes such as those for lipid desaturase (SCD-1, FADS1 and FADS2; stearoylCoA desaturase, and fatty acid desaturase 1 and 2, respectively) or the APOE-e4 allele, for example, will be able to address some of the issues relating to individual differences in the propensity to develop diseases associated with low n-3 PUFA intake. It is evident that dietary n-3 PUFA are important, yet understanding exactly how and why will be the objective of many future investigations.
Acknowledgements
This study was completed as part of the PhD of P. A. J., which was funded by Ginsana SA, Switzerland. Ginsana SA did not have any other input into the study design or analysis of the data. P. A. J., D. O. K., J. L. R. and A. B. S. all contributed to the design and analysis of the study. P. A. J. collected the cognitive and mood data. M. E. D. analysed the blood samples for fatty acids. All authors contributed to the writing of the manuscript. The authors declare that they have no conflicts of interest. The authors would like to thank Anthea Milne, Ruth Steinberg, Joanne Forster and Jade Elliott for their technical assistance in collecting the venous blood samples.