Niacin (vitamin B3) is an essential water-soluble vitamin, comprising two main forms as nicotinic acid and nicotinamide. Niacin is the precursor to NAD+ and NADP+, which are important coenzymes in catabolic and anabolic redox reactions, respectively. Besides being precursor to coenzymes, nicotinic acid is also known for its anti-lipolytic action via the hydroxycarboxylic acid-2 receptor (HCA2)(Reference Carlson1). On one hand, the National Research Council suggested that niacin supplementation may not be necessary for lactating dairy cows in terms of improving production performance(Reference Grossblatt2). On the other hand, a number of studies found that pharmacological doses of niacin did have some beneficial effects for cow health, especially under challenging conditions. For instance, supplementing pharmacological doses of niacin was reported to decrease the ketosis prevalence in early lactation(Reference Havlin, Robinson and Garrett3) and reduce the heat stress(Reference Wrinkle, Robinson and Garrett4) in dairy cows. These benefits are likely related to pharmacological effects of niacin on lipid metabolism or vasodilation rather than alleviating niacin deficiency, because the ruminal synthesis of niacin could cover the requirement(Reference Grossblatt2). However, the reported effects of niacin supplementation are not always consistent across studies. The synthesis and absorption of niacin, and effects of non-rumen-protected niacin (non-RPN) supplementation on milk yield and composition, and plasma metabolite concentrations in dairy cows were thoroughly reviewed by Niehoff et al. (Reference Niehoff, Hüther and Lebzien5) In the past decade, the RPN was developed, which could be more efficient than free niacin. Moreover, HCA2 was found to be widely expressed in different tissues in dairy cows(Reference Xiao, Rungruang and Hall6), which provides new insight into niacin’s mechanisms of action. Therefore, this aim of the current review is to present the updated information on ruminal niacin synthesis, the role of HCA2, effects of niacin supplementation on ruminal metabolism and nutrient digestibility, metabolic status, milk yield and composition, insulin resistance during early lactation and heat stress, with emphasis on RPN and studies published in the last decade. An attempt was made to point out the problems in relation to niacin nutrition in dairy cows for future research.
Niacin sources and bioavailability
Niacin is naturally present in animal by-products and plant-source feedstuffs. The niacin content of forage is highly variable, and the niacin content of grains seems relatively low (Table 1). The daily intake of niacin in dairy cows is largely dependent on feed intake and diet composition, varying from 325 to 4434 mg/d as reported by various researchers in different studies(Reference Seck, Linton and Allen7–Reference Castagnino, Seck and Beaudet11). In addition to the niacin supplied from feed, ruminants can synthesise niacin from tryptophan and quinolinic acid. This synthesis, however, is relatively inefficient, and tryptophan is preferentially utilised for protein synthesis(Reference Frye, Williams and Graham13).
Table 1. Niacin contents in several feedstuffs

Ruminal synthesis of niacin by micro-organisms is considered as the main source of niacin for ruminants. Nevertheless, the actual ruminal synthesis of niacin is difficult to measure. The apparent ruminal synthesis (ARS) of niacin, calculated as the niacin content in duodenal flow minus daily niacin intake, is often used to estimate the microbial niacin synthesis. It was estimated that ARS of niacin in a 650-kg cow producing 35 kg/d of 4 % fat-corrected milk was 1804 mg/d(Reference Grossblatt2). Niehoff et al. (Reference Niehoff, Hüther and Lebzien5) reviewed six studies published between 1985 and 2006 and suggested that ARS of niacin is affected by forage to concentrate ratio or non-fibre carbohydrate content. In the last decade, several studies also investigated the influence of different types of feed on the ARS of niacin (Table 2). Niehoff et al. (Reference Niehoff, Hüther and Lebzien14) observed that ARS of niacin was less in cows fed the low concentrate diet compared with the high or medium concentrate diet, which is consistent with the findings of Seck et al. (Reference Seck, Linton and Allen7) In addition, the diet with high niacin content(Reference Beaudet, Gervais and Graulet9,Reference Castagnino, Kammes and Allen12) would likely result in negative ARS of niacin. Especially, 98·5 % of supplementary nicotinamide(Reference Santschi, Berthiaume and Matte15) and 88–94 % of supplementary nicotinic acid(Reference Niehoff, Hüther and Lebzien14) disappeared in the rumen, resulting in a greater negative ARS of niacin. The exact mechanism of this phenomenon is not clear. It is suggested that a niacin homeostatic system may exist in the rumen: synthesis will occur when the niacin content is below the optimal level, and niacin is degraded by bacteria when the niacin content is above the optimal level(Reference Niehoff, Hüther and Lebzien5). In order to increase the bioavailability of niacin in the small intestine, RPN was developed. It was stated that the ruminal stability of RPN is approximately 90 % and could deliver daily about 40 % bioavailable niacin(Reference Morey, Mamedova and Anderson16,Reference Zeitz, Weber and Most17) . Thus, it can be expected that feeding RPN is more effective compared with the traditional free niacin.
Table 2. Apparent ruminal synthesis (ARS) of niacin in dairy cows

NS, niacin supplementation; DMI, DM intake; NI, niacin intake; DNF, duodenal niacin flow.
* Significant difference (P ≤ 0·05) between groups within a study. Niehoff et al. (Reference Niehoff, Hüther and Lebzien14) did not illustrate the significance.
† Organic matter intake was determined in the study of Niehoff et al. (Reference Niehoff, Hüther and Lebzien14).
Unlike the traditional free niacin, the reported effects of RPN supplementation on plasma niacin concentration were relatively consistent. All four studies that determined plasma niacin concentration detected a significant increase in niacin content in plasma after an RPN supplementation(Reference Morey, Mamedova and Anderson16–Reference Rungruang, Collier and Rhoads19). This could be related to the increased bioavailability of RPN. Rungruang et al. (Reference Rungruang, Collier and Rhoads19) reported that RPN supplementation (0, 4, 8 or 12 g/d) increased plasma and milk niacin concentrations in a linear manner. However, the niacin concentration in that study was determined by microbiological assay, which is not possible to distinguish between nicotinic acid and nicotinamide. Morey et al. (Reference Morey, Mamedova and Anderson16) found the plasma concentration of nicotinic acid was not influenced by RPN supplementation and were about 100 times lower than plasma nicotinamide concentration. In the study by Zeitz et al. (Reference Zeitz, Weber and Most17), plasma nicotinic acid concentration was below the detection limit (0·025 μg/l). Those results are in agreement with an earlier study by Kollenkirchen et al. (Reference Kollenkirchen, Kuhnigk and Breves20) who reported that only nicotinamide was present in the blood of sheep after nicotinic acid or nicotinamide supplementation. It is generally accepted that nicotinic acid is readily converted into NAD+ in the intestine and liver and then is cleaved to produce nicotinamide into blood for extrahepatic tissues(Reference Lenglet, Liabeuf and Guffroy21). It implies that nicotinamide might be the main transport form in blood, which could partly explain the undetectable or extremely low concentration of nicotinic acid in blood. Additionally, the kinetics of niacin supplementation in dairy cows is not completely clear. In humans, the maximal plasma concentration of nicotinic acid is reached after 30–60 min when administered orally(Reference Bodor and Offermanns22), and the plasma half-life of nicotinic acid is about 20–45 min, whereas the half-life of nicotinamide is about 4 h(Reference Rennick, Kalakeche and Seel23). In dairy cows, the half-life of nicotinamide could probably be longer than 4 h, because Zimbelman et al. (Reference Zimbelman, Baumgard and Collier18) found the serum niacin concentration returned to presupplementation values by 3 d after a dose of 12 g/d RPN supplementation. In above-mentioned studies, the difference in half-life time could be another reason for the different content in blood of two niacin vitamers as the blood samples were collected before feeding. However, Campbell et al. (Reference Campbell, Murphy and Christensen24) reported that concentrations of plasma nicotinic acid and plasma nicotinamide did not differ over time in 11 h after 12 g/d of free nicotinic acid or nicotinamide supplementation. Thus, further research are required to clarify the kinetics of niacin, especially RPN, in ruminants.
Effects of free niacin supplementation on ruminal metabolism and nutrient digestibility
Effects of free niacin supplementation on ruminal metabolism and nutrient digestibility have been inconsistent (Table 3). Although free niacin supplementation has been reported to increase microbial protein synthesis in two in vitro studies(Reference Shields, Schaefer and Perry32,Reference Horner, Windle and Coppock25) , microbial protein production increased in only two of seven in vivo studies. However, five in vivo studies reported that protozoa number (mainly Entodinium) in rumen fluid was increased by niacin supplementation. Ruminal protozoa are unable to synthesise niacin and the niacin from diet and ruminal bacteria might not be adequate to cover their requirement(Reference Doreau and Ottou28,Reference Kumar and Dass29) . Thus, the free niacin supplementation may improve the growth for ruminal protozoa in the rumen and increase the protozoal predation of bacteria(Reference Firkins, Yu and Morrison33). Aschemann et al. (Reference Aschemann, Lebzien and Hüther30) proposed that the free niacin supplementation might change the composition of microbial protein by increasing the protozoal protein and decreasing the bacterial protein, which could be the reason for the unchanged microbial protein content reaching the duodenum. In addition, those authors suggested the reduced faecal N excretion in niacin supplemented animals could also be related to the increased protozoal protein because the digestibility of protozoal protein is greater than bacterial protein(Reference Aschemann, Lebzien and Hüther34).
Table 3. Effects of nicotinic acid (NA) or nicotinamide (NAM) supplementation on ruminal metabolism and nutrient digestibility
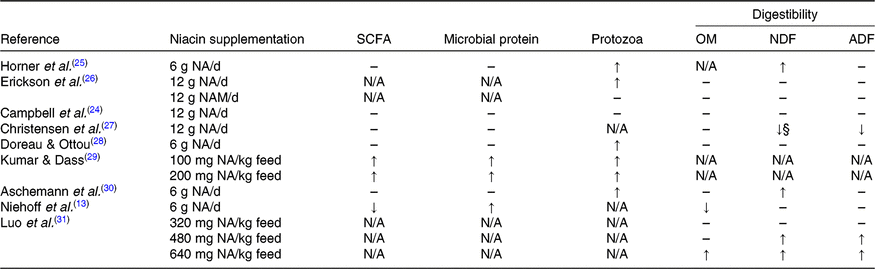
OM, organic matter; NDF, neutral-detergent fibre; ADF, acid-detergent fibre; N/A, not available.
↑, Significantly increased (P ≤ 0·05); ↓, significantly decreased (P ≤ 0·05); ↓§, tended to decrease (0·1 ≥ P > 0·05); – , no significant effect of niacin supplementation (P > 0·1).
The ruminal protozoa digest 25–30 % of the total fibre(Reference Lee, Ha and Cheng35). Accordingly, the increased protozoa number during free niacin supplementation could be expected to improve the fibre digestibility. Indeed, the neutral-detergent fibre digestibility increased in two studies(Reference Aschemann, Lebzien and Hüther34,Reference Luo, Gao and Lu31) , but not in other studies(Reference Niehoff, Hüther and Lebzien14,Reference Campbell, Murphy and Christensen24,Reference Doreau and Ottou28,Reference Erickson, Trusk and Murphy26) . This discrepancy could be related to dietary composition. Aschemann et al. (Reference Aschemann, Lebzien and Hüther34) suggested that niacin may be less beneficial to fibre degradation when the N supply from the diet is optimal. In addition, the protozoa number could possibly influence the ruminal fermentation pattern, since the protozoa number was reported to be negatively related to acetic acid concentration in the rumen(Reference Dönmez, Karslı and Çınar36). In line with this, free niacin supplementation led to a decrease in acetic acid proportion(Reference Aschemann, Lebzien and Hüther30) and tended to decrease the acetic acid content(Reference Christensen, Overton and Clark27). Nevertheless, propionate, butyrate and total SCFA were mostly unaffected by free niacin supplementation in in vivo studies. In summary, free niacin supplementation increases the protozoa number in the rumen, but the changed protozoa population may not be sufficient to significantly affect the fermentation pattern and nutrient digestibility in ruminants.
Niacin supplementation during transition periods
Metabolic status
During early lactation, dairy cows typically experience a negative energy balance because the feed intake is not adequate to meet the nutrient demand for the rapidly increasing milk yield(Reference van Knegsel, van der Drift and Čermáková37). Body fat is mobilised to compensate for the energy deficit that results in a substantial increase in plasma NEFA, β-hydroxybutyrate (BHBA) and liver TAG in dairy cows(Reference Chen, Gross and van Dorland38). The negative energy balance-induced metabolic changes have been associated with increased incidence of metabolic disorders and infectious diseases, increased culling rate and reduced fertility(Reference Grummer39 ,Reference Butler40 ,Reference Seifi, LeBlanc and Leslie41 ). Nicotinic acid has been long known as a lipid-lowering compound due to its anti-lipolytic action(Reference Romani, Hofer and Katsyuba42). Thus, nicotinic acid is expected to suppress lipolysis in early lactation to improve the health of dairy cows, but the reported effects are inconsistent.
Circulating NEFA is an important indicator of adipose mobilisation and metabolic health in dairy cows(Reference Ospina, Nydam and Stokol43). In some studies(Reference Yuan, Shaver and Bertics44–Reference Wei, Cai and He46), supplementation of nicotinic acid, RPN or nicotinamide during transition periods was reported to decrease the plasma NEFA concentration, but in some other studies(Reference Zeitz, Weber and Most17,Reference Tienken, Kersten and Frahm47,Reference Kenéz, Tienken and Locher48) supplementation had no influence (Table 4). Additionally, Morey et al. (Reference Morey, Mamedova and Anderson16) reported that 12 g/d RPN supplementation in early lactation decreased peak plasma NEFA concentration but did not affect the average plasma NEFA concentration. The niacin supplementation did not affect feed intake or milk yield in early lactation in most studies, thus the discrepancy in plasma NEFA concentration among studies could not be attributed to the differences in energy input or milk energy output. It is likely that niacin supplementation level may play a role in the anti-lipolytic effect in dairy cows. In early lactation, the relatively low dosages of free nicotinic acid or nicotinamide supplementation (6–24 g/d) in studies summarised by Niehoff et al. (Reference Niehoff, Hüther and Lebzien5) and two more recent studies(Reference Tienken, Kersten and Frahm47,Reference Kenéz, Tienken and Locher48) demonstrated only minor effects, whereas 12 g/d of RPN(Reference Morey, Mamedova and Anderson16,Reference Yuan, Shaver and Bertics44) , 45 g/d of nicotinamide(Reference Wei, Cai and He46) or 120 g/d of nicotinic acid(Reference Hristovska, Cincović and Stojanović45) supplementation demonstrated a greater influence on plasma NEFA concentration. The extensive degradation of free niacin in the rumen may partly explain the lack of effects when a low level of nicotinic acid was supplemented.
Table 4. Effects of niacin supplementation during transition periods on DM intake (DMI), milk yield, milk composition and plasma metabolites

BHBA, β-hydroxybutyrate; RPN, rumen-protected nicotinic acid; N/A, not available; NA, nicotinic acid; NAM, nicotinamide.
↑, Significantly increased (P ≤ 0·05); ↓, significantly decreased (P ≤ 0·05); ↑§, tended to increase (0·1 ≥ P > 0·05); –, no significant effect of niacin supplementation (P > 0·1).
* Treatment × parity × time interactions were detected for plasma NEFA and BHBA concentrations.
Pires et al. (Reference Pires, Pescara and Grummer49) reported that abomasal infusion of nicotinic acid delivered as a single bolus or hourly could significantly decrease the plasma NEFA concentration during feed restriction. Later, Pescara et al. (Reference Pescara, Pires and Grummer50) and Pires et al. (Reference Pires, Stumpf and Soutullo51) confirmed that finding and found continuous abomasal infusion of 3 mg nicotinic acid/h per kg of body weight was sufficient to depress plasma NEFA concentration during feed restriction. However, a dramatic rebound of plasma NEFA concentration was observed 2–4 h after termination of infusion, and the duration of the rebound lasted 4–9 h(Reference Pires, Pescara and Grummer49,Reference Pescara, Pires and Grummer50) . These observations indicate that a certain level of rumen bypassing niacin supplementation could suppress lipolysis, but the rebound of plasma NEFA concentration may interfere with the evaluation of niacin’s anti-lipolytic effect in dairy cows. Therefore, the blood sampling time may be another reason for the differences in plasma NEFA concentrations among studies, because the plasma NEFA concentration could vary greatly depending on the time interval between feeding and blood sampling.
Plasma NEFA can be partially oxidised to form BHBA or esterified to be stored in the liver as TAG(Reference Grummer39). As expected, Hristovska et al. (Reference Hristovska, Cincović and Stojanović45) and Wei et al. (Reference Wei, Cai and He46) found the plasma BHBA concentration decreased after niacin supplementation. Similar to plasma NEFA concentration, abomasal infusion of niacin decreased plasma BHBA concentration, and the rebound of plasma BHBA concentration after termination of infusion was found in feed-restricted dairy cows(Reference Pescara, Pires and Grummer50,Reference Pires, Stumpf and Soutullo51) . However, plasma BHBA and liver TAG concentrations were not affected, while plasma NEFA concentration decreased after RPN supplementation in the study by Yuan et al. (Reference Yuan, Shaver and Bertics44) It was suggested that plasma NEFA concentration may not be the only factor that determines the plasma BHBA(Reference Chen, Gross and van Dorland38). In addition, Zeitz et al. (Reference Zeitz, Weber and Most17) found plasma BHBA concentration, but not plasma NEFA concentration, decreased after supplementation with 55 g/d RPN. The authors declared that effects of nicotinic acid on ketogenesis might occur only at very high levels. In contrast, 3·5 g/d of RPN supplementation in early lactation tended to decrease ketosis prevalence from 36 to 20 %, but 14 g/d RPN supplementation had no effect on ketosis prevalence in a study involving 906 dairy cows(Reference Havlin, Robinson and Garrett3). These findings indicate that it is possible to decrease the ketosis prevalence in dairy herd by RPN supplementation, though the level of supplementation still needs to be determined.
In human studies, it was shown that niacin may decrease the rate of liver TAG synthesis by inhibition of the diglyceride acyltransferase-2 which is independent of niacin’s anti-lipolytic action(Reference Ganji, Tavintharan and Zhu52,Reference Lauring, Taggart and Tata53) . However, this was not found in dairy cows. Several studies reported that RPN supplementation had no effects on liver TAG concentration. It is not clear whether this is also related to the plasma NEFA rebound(Reference Morey, Mamedova and Anderson16,Reference Zeitz, Weber and Most17,Reference Yuan, Shaver and Bertics44) .
Insulin resistance
Insulin resistance is defined as a state of either decreased sensitivity or responsiveness to insulin in the insulin-sensitive body tissues(Reference Kahn54). Insulin resistance together with the lowered plasma insulin concentration in early lactation facilitates lipolysis in adipose tissue and spare glucose for milk synthesis(Reference Butler40). It is believed that high plasma NEFA concentration is the major cause of insulin resistance in dairy cows(Reference Pires, Souza and Grummer55), thus niacin supplementation could possibly decrease the insulin resistance in dairy cows in early lactation. Hristovska et al. (Reference Hristovska, Cincović and Belić56) concluded that niacin supplementation had a dual influence on insulin resistance during early lactation based on the revised quantitative insulin sensitivity check index (RQUICKI), which is a surrogate index for insulin sensitivity. Another study also concluded that RPN supplementation could improve the peripheral tissue sensitivity based on RQUICKI(Reference Youssef, El-Ashker and Younis57). However, RQUICKI was derived from human-based studies and may not be suitable to assess the sensitivity of the peripheral tissues to insulin in early lactation in dairy cows(Reference Alves-Nores, Castillo and Hernandez58). Several studies demonstrated no correlation between RQUICKI and the results obtained by the gold standard tests (the hyperinsulinaemic–euglycaemic clamp test) or intravenous glucose tolerance test in dairy cows(Reference De Koster, Hostens and Hermans59,Reference Mann, Yepes and Duplessis60) . Therefore, the reported effects of niacin on insulin sensitivity based on RQUICKI might not accurately reflect the actual insulin sensitivity states and could lead to confusing conclusions.
Pires et al. (Reference Pires, Pescara and Grummer49) evaluated the effects of hourly abomasal infusion of niacin on insulin resistance using intravenous glucose tolerance test in a short-term study (11 h), and these authors found niacin supplementation enhanced insulin response in feed-restricted dairy cows probably by lowering plasma NEFA concentration. Nonetheless, the same research group found that a long-term (74 h) abomasal infusion of niacin decreased plasma NEFA concentration but caused an insulin resistance state in feed-restricted dairy cows(Reference Pires, Stumpf and Soutullo51). Apparently, the niacin anti-lipolytic effect did not result in an enhanced insulin response in the long-term niacin abomasal infusion, but the underlying mechanisms are still unknown.
Milk yield and composition
Milk yield and milk protein percentage were not affected by either RPN or free niacin supplement in early lactation in several studies(Reference Morey, Mamedova and Anderson16,Reference Zeitz, Weber and Most17,Reference Yuan, Shaver and Bertics44,Reference Wei, Cai and He46–Reference Kenéz, Tienken and Locher48) , which is in line with most of the earlier studies investigating the effects of free niacin supplementation on milk yield and milk protein percentage(Reference Niehoff, Hüther and Lebzien5). Supplementation of RPN in early lactation decreased milk fat percentage in two studies(Reference Zeitz, Weber and Most17,Reference Yuan, Shaver and Bertics44) . It was assumed that niacin supplementation might affect milk composition by affecting ruminal microbial growth or adipose tissue lipolysis(Reference Schwab, Schwab and Shaver8). As discussed above, the niacin supplement has minor effects on microbial protein synthesis or ruminal nutrient digestibility and the RPN is unlikely to affect ruminal metabolism due to its high ruminal stability. Hence, the decreased milk fat percentage after the RPN supplementation might mainly contribute to the decreased plasma NEFA concentration because plasma NEFA could be used for milk fat synthesis by mammary glands(Reference Yuan, Shaver and Bertics44). However, decreased(Reference Zeitz, Weber and Most17) or tendency of increased(Reference Tienken, Kersten and Frahm47) milk fat percentage without affecting plasma NEFA concentration was found after niacin supplementation in early lactation. It is possibly that niacin may directly affect lipid metabolism in the mammary gland independent of the anti-lipolytic function. Further studies are needed to confirm this hypothesis.
Supplementation of niacin prepartum
Two studies evaluated the effects of supplementation of 48 g/d free nicotinic acid(Reference Aragona, Chapman and Pereira61) or 12 g/d RPN(Reference Youssef, El-Ashker and Younis57) on colostrum quality only in prepartum or on metabolic status in early lactation. Aragona et al. (Reference Aragona, Chapman and Pereira61) found nicotinic acid supplementation prepartum significantly increased IgG concentration but did not affect calf immunity or calf body weight. The authors suggested that the increased IgG may be related to the increased IgG transferred to colostrum due to increased blood flow or the increased microbial protein synthesis after niacin supplementation. Surprisingly, plasma NEFA concentration increased in the first week after calving in dairy cows supplemented with nicotinic acid, which the author attributed to the rebound of NEFA concentration(Reference Aragona, Chapman and Pereira61). In the study of Youssef et al. (Reference Youssef, El-Ashker and Younis57), no significant effects of RPN supplementation prepartum were observed on plasma NEFA, BHBA, glucose or insulin concentrations in early lactation. Those findings indicate that niacin supplementation may improve the colostrum quality, but niacin supplementation only in prepartum had limited or even negative effects on metabolic status in early lactation.
Niacin supplementation during heat stress
Skin flushing, resulting from a cutaneous vasodilation, is considered as the most common side effect of nicotinic acid in humans(Reference Gille, Bodor and Ahmed62). The vasodilatory reaction of nicotinic acid could be used to improve heat dissipation through enhanced evaporative heat loss and then to alleviate heat stress in dairy cows. In addition, Rungruang et al. (Reference Rungruang, Collier and Rhoads19) found the niacin concentration in whole blood was significantly decreased in heat-stressed dairy cows, which implies that the niacin metabolism might increase and dietary supplementation of niacin might be necessary in heat stress conditions. Indeed, RPN or free nicotinic acid supplementation were reported to reduce the vaginal temperature(Reference Zimbelman, Baumgard and Collier18,Reference Pineda, Drackley and Garrett63,Reference Zimbelman, Collier and Bilby64) or skin temperatures(Reference Di Costanzo, Spain and Spiers65) during moderate to severe heat stress conditions with a temperature humidity index (THI) > 72. However, Lohölter et al. (Reference Lohölter, Meyer and Rauls66) reported that 24 g/d free nicotinic acid supplementation did not affect skin or rectal temperatures during a mild heat stress (THI > 68). The relatively lower THI in that study could be the cause of the lack of niacin supplementation on body temperature. Di Costanzo et al. (Reference Di Costanzo, Spain and Spiers65) found free nicotinic acid supplementation decreased skin temperatures during severe heat stress (mean maximum THI = 85·1) but not in no heat stress condition (mean maximum THI = 75). Also, a dual role of niacin in regulating heat shock protein expression was reported in a recent in vitro study, in which niacin decreased the mRNA expression of heat shock protein under thermoneutral conditions, but increased the mRNA expression of heat shock protein in heat stress condition in mammary and uterine cells(Reference Xiao, Rungruang and Hall6). Rungruang et al. (Reference Rungruang, Collier and Rhoads19) carried out a trial in environmentally controlled rooms during winter and found that RPN supplement did not affect the sweating rate and core body temperature during moderate to severe thermal stress (THI = 72–80). They proposed seasonal depression in sweat gland function could be the reason for that result.
Heat stress caused feed intake depression is usually related to the reduced milk yield. Supplementation of RPN during a more severe heat stress condition increased(Reference Zimbelman, Collier and Bilby64) or tended to increase(Reference Pineda, Drackley and Garrett63) the milk yield, despite no effect observed on feed intake. Similar findings were observed for free nicotinic acid supplementation(Reference Lohölter, Meyer and Rauls66). However, niacin supplementation did not affect milk yield in several other studies(Reference Wrinkle, Robinson and Garrett4,Reference Zimbelman, Baumgard and Collier18,Reference Di Costanzo, Spain and Spiers65) . It seems that increased milk after niacin supplementation is not necessarily related to the alleviation of heat stress in dairy cows but the reasons remain unclear. Unlike in the early lactation, RPN supplementation in heat stress were reported to increase milk fat percentage(Reference Zimbelman, Baumgard and Collier18,Reference Pineda, Drackley and Garrett63) . Although the milk fat percentage decreased after niacin supplementation, the milk fat yield increased due to the increased milk yield in the study by Lohölter et al. (Reference Lohölter, Meyer and Rauls66) Wrinkle et al. (Reference Wrinkle, Robinson and Garrett4) found milk fat percentage increased in mid-lactation but decreased in early lactation after RPN supplementation in heat-stressed dairy cows. It seems likely that different mechanisms other than anti-lipolytic effect are involved in the regulation of lipid synthesis in mammary glands of heat-stressed compared with the early lactation dairy cows. In addition, Di Costanzo et al. (Reference Di Costanzo, Spain and Spiers65) and Rungruang et al. (Reference Rungruang, Collier and Rhoads19) reported no effects of niacin supplementation on milk yield or milk composition in heat stress. The discrepancy among studies could be related to the different degrees of heat stress, lactation stage, dosage of niacin supplementation or the form of niacin.
In short, niacin supplementation may alleviate heat stress in dairy cows possibly through vasodilation during moderate to severe heat stress. Niacin supplementation in heat stress seems to exert different effects on milk synthesis compared with early lactation, but the mechanism is unknown. In addition, Rungruang et al. (Reference Rungruang, Collier and Rhoads19) detected a linear relationship between RPN supplementation and water intake. It would be interesting to investigate whether the same relationship could also be detected in early lactation and to study whether the increased water intake affects ruminal metabolism, animal health and performance in dairy cows.
Role of the hydroxycarboxylic acid-2 receptor
HCA2, formerly also known as GPR109a, is a Gi protein-coupled receptor. In 2003, HCA2 was identified as the receptor of nicotinic acid and mediates the anti-lipolytic effect of nicotinic acid through reducing cyclic AMP (cAMP) accumulation and inhabiting hormone-sensitive lipase (HSL) in adipose tissue, independently by three research groups(Reference Soga, Kamohara and Takasaki67–Reference Wise, Foord and Fraser69). The BHBA is reported to be the endogenous ligand of HCA2 and butyrate also possesses the property to activate HCA2(Reference Graff, Fang and Wanders70), but another form of niacin, nicotinamide, is inactive on HCA2(Reference Wise, Foord and Fraser69). In ruminants, Titgemeyer et al. (Reference Titgemeyer, Mamedova and Spivey71) reported that HCA2 mRNA expression was more abundant in the liver than in adipose tissues and muscle, and HCA2 mRNA was also expressed in the brain. Later HCA2 mRNA expression was found even higher in skin and the udder than that in the liver, ovary and uterus(Reference Xiao, Rungruang and Hall6). The widely expressed HCA2 mRNA in various tissues imply that HCA2 may be involved in various physiological processes in dairy cows.
To date, studies regarding HCA2 in dairy cows mainly focused on its role in anti-lipolytic effects of nicotinic acid. The HCA2 mRNA abundance in adipose tissue decreased during early lactation in dairy cows, suggesting a feedback mechanism to reduce the anti-lipolytic effects of BHBA in early lactation(Reference Lemor, Hosseini and Sauerwein72). Kenéz et al. (Reference Kenéz, Locher and Rehage73) found nicotinic acid, but not nicotinamide, was able to suppress isoproterenol-induced lipolysis by reducing the extent of HSL phosphorylation in the isolated bovine adipose tissues. Those authors suggested that stimulating HCA2 by nicotinic acid triggers the anti-lipolytic pathway. However, supplementing niacin in feed did not influence the HCA2 mRNA abundance in the liver(Reference Morey, Mamedova and Anderson16,Reference Zeitz, Weber and Most17) or the extent of HSL phosphorylation in early lactation(Reference Kenéz, Tienken and Locher48). The short half-life of nicotinic acid or the ruminal degradation probably explains the lack of effect of niacin on HCA2 mRNA abundance or HSL phosphorylation. In addition, in an in vitro study, nicotinic acid treatment increased adiponectin concentration via HCA2 in differentiated bovine adipocytes, indicating an improvement in insulin sensitivity(Reference Kopp, Hosseini and Singh74). Besides mediating anti-lipolysis in adipose tissue, the expression of HCA2 in brain, mammary glands, and liver indicates that niacin might play a role in monitoring plasma BHBA concentrations in the central nervous system(Reference Titgemeyer, Mamedova and Spivey71) or allow the niacin to affect milk synthesis or lipid metabolism directly.
Recent studies demonstrated that nicotinic acid has a profound anti-inflammatory effect by an HCA2-dependent mechanism in mouse alveolar macrophages(Reference Zhou, Li and Yao75), colonic macrophages, dendritic cells(Reference Singh, Gurav and Sivaprakasam76), human retinal pigmented epithelial cells, kidney cells(Reference Gautam, Banskota and Shah77), etc. This raises the question of whether nicotinic acid exerts a similar anti-inflammatory effect in ruminants. Indeed, nicotinic acid was found to inhibit Staphylococcus aureus-induced NF-κB activation in bovine mammary gland cells, indicating the reduced inflammatory response(Reference Wei, Fu and Zhou78), which could be possibly related to HCA2. By contrast, 24 g/d free nicotinic acid supplementation during the transition period did not show an effect on NF-κB mRNA abundance in blood leucocytes(Reference Bühler, Frahm and Tienken79). This could be due to the extensive degradation of free nicotinic acid in the rumen. However, Ringseis et al. (Reference Ringseis, Zeitz and Weber80) evaluated the effects of high-dosage RPN supplementation (approximately 55 g/d) on liver metabolism by analysing the liver transcriptome. These authors found that RPN supplementation may amplify the systemic inflammation-like condition in early lactation, and they suggested the markedly high-dosage RPN might be related to the indications of inflammation-like condition. To support this hypothesis, Gautam et al. (Reference Gautam, Banskota and Shah77) found low concentration of niacin down-regulated the pro-inflammatory cytokines, but the high concentration of niacin induced cell apoptosis.
To sum up, nicotinic acid could suppress lipolysis and inflammation in in vitro studies but no effects were found in vivo (Fig. 1). The dosage of niacin supplementation and ruminal degradation of free nicotinic acid may partly explain the discrepancy between in vitro and in vivo findings.

Fig. 1. The effects of niacin on ruminal metabolism, inflammation of mammary epithelial cells and lipolysis in adipocytes: (1) Niacin increases the number of protozoa (mainly Entodinium) in the rumen. (2) Niacin suppresses isoproterenol-induced lipolysis in bovine adipocytes by reducing the intracellular level of cyclic AMP (cAMP) and inhabiting hormone-sensitive lipase (HSL) via the hydroxycarboxylic acid-2 receptor (HCA2). (3) Niacin increases adiponectin concentration in bovine adipocytes via HCA2. (4) Niacin could reduce inflammation in bovine mammary epithelial cells, which was triggered by Staphylococcus aureus by suppressing the toll-like receptor (TLR)–NF-κB signalling pathway possibly via HCA2.
Conclusions and future research
Because of the high ruminal stability, RPN supplementation increased plasma niacin concentration in most studies. However, RPN supplementation did not always show beneficial effects on cow performance or health during the transition period or in heat-stressed conditions. The following issues should be addressed in the future research for better understanding of niacin’s mechanisms of action and better application of niacin in practice:
1. Results from in vitro studies or from abomasal infusion studies clearly showed that nicotinic acid could depress lipolysis in dairy cows. Despite the high ruminal stability, RPN supplementation still led to inconclusive results in in vivo studies. We postulated that the short half-life of nicotinic acid, which only transiently depresses lipolysis, may be one of the reasons. Thus, further studies are required to determine the kinetics of RPN in dairy cows.
2. The rebound of plasma NEFA concentration could be another reason for the inconsistent results regarding the anti-lipolysis effects of RPN supplementation. Studies concerning the mechanism of plasma NEFA rebound are desirable. In addition, further studies should determine whether the plasma NEFA rebound aggravates the metabolic stress during early lactation.
3. Previous studies demonstrated high dosages of RPN supplementation may have adverse effects on cow performance and health. More studies are needed to elucidate the optimal dosage of niacin supplementation.
4. HCA2 mRNA expression is relatively high in the mammary gland and liver. Studies should be conducted to test the hypothesis that niacin may directly influence lipid metabolism or milk synthesis through HCA2.
5. A number of studies reported the anti-inflammatory effects of nicotinic acid through an HCA2-dependent mechanism. Studies should be carried out to investigate whether nicotinic acid depresses inflammation in bovine mammary glands via stimulating HCA2.
Acknowledgements
We thank our colleagues for the stimulating discussions.
The present study was financially supported by Fundamental Research Funds for the Central Universities (grant no. SWU118126).
J. C. conceived this project and wrote the manuscript. G. D. critically revised the manuscript. Z. Y. studied all of the publications cited in this paper. All authors read and approved the manuscript.
The authors declare that there are no conflicts of interest.