Amoebiasis, caused by the protozoan Entamoeba histolytica, is the third leading cause of death by parasitic diseases, surpassed only by malaria and schistosomiasis(Reference Pritt and Clark1). Worldwide, approximately 50 million people are infected with E. histolytica, resulting in nearly 100 000 deaths annually. E. histolytica is transmitted by the faecal–oral route through contaminated food or water and is often endemic in regions with poor hygiene. Infection is initiated through ingestion of the semi-dormant cyst form of the parasite, which passes through the stomach and small intestine to reach the colon, where it differentiates into motile trophozoites that can colonise and invade the host's mucosa and cause dysentery. In rare cases, trophozoites metastasise from the colon and cause extra-intestinal abscesses mainly in the liver, and also in the lung or the brain(Reference Pritt and Clark1). Colonisation and invasion require the attachment of trophozoites to the host's mucosa. Trophozoites that cannot attach are carried downstream and are excreted with the faeces without causing disease.
Breast-fed infants are at lower risk of acquiring E. histolytica infections than formula-fed infants(Reference Islam, Stoll and Ljungstrom2). This could be explained by a reduced exposure to contaminated water that might be used to prepare formula and/or by amoebicidal components in human milk. The latter has been supported by studies showing that human milk kills trophozoites(Reference Islam, Stoll and Ljungstrom2, Reference Gillin, Reiner and Wang3). While most studies focused on the amoebicidal activity of milk proteins such as soluble IgA, lactoferrin and lysozyme(Reference Leon-Sicairos, Lopez-Soto and Reyes-Lopez4), 1 litre of human milk also contains more than 10–15 g of unbound complex oligosaccharides (reviewed in Newburg et al. (Reference Newburg, Ruiz-Palacios and Morrow5), Kunz et al. (Reference Kunz, Rudloff and Baier6) and Bode(Reference Bode7, Reference Bode8)), which exceed the amount of total milk proteins. In addition to their prebiotic effects, human milk oligosaccharides (HMO) have been reported to protect the breast-fed infant from infection with viral and bacterial pathogens such as Campylobacter jejuni, pathogenic Escherichia coli strains, Vibrio cholerae, as well as certain Shigella and Salmonella strains (reviewed in Newburg et al. (Reference Newburg, Ruiz-Palacios and Morrow5)). To establish infection, these pathogens bind with their surface lectins to glycans on the luminal side of the host's intestinal epithelial cells. HMO serve as decoy glycan receptors, block the pathogens from binding to the host's epithelium and prevent infection(Reference Newburg, Ruiz-Palacios and Morrow5, Reference Morrow, Ruiz-Palacios and Jiang9). E. histolytica also employs a lectin that is involved in parasite attachment and host cell toxicity(Reference Saffer and Petri10, Reference Saffer and Petri11). Whether HMO affect E. histolytica attachment and cytotoxicity, has not been described.
The E. histolytica lectin recognises galactose (Gal), N-acetylgalactosamine (GalNAc) and lactose(Reference Cano-Mancera and Lopez-Revilla12), and HMO contain some of these sugars. HMO consist of five monosaccharide building blocks: glucose (Glc), N-acetylglucosamine (GlcNAc), Gal, fucose (Fuc) and sialic acid. Lactose forms the reducing end and can be elongated by one or more lacto-N-biose or lactosamine disaccharides (Galβ1–3/4GlcNAc) to yield complex oligosaccharides. Lactose or the (poly-)lacto-N-biose/lactosamine backbone is often fucosylated and/or sialylated to create the variety of more than 150 different HMO identified to date (reviewed in Newburg et al. (Reference Newburg, Ruiz-Palacios and Morrow5), Kunz et al. (Reference Kunz, Rudloff and Baier6) and Bode(Reference Bode7, Reference Bode8)).
Bovine milk-based infant formulas contain only trace amounts of HMO. In an attempt to mimic some of the health benefits of HMO, formula manufacturers have begun to fortify their products with non-digestible galacto-oligosaccharides (GOS). GOS are synthesised enzymatically and are built out of 1–7 Gal residues attached to a Glc molecule at the reducing end. GOS have been reported to be prebiotic and to promote an intestinal microbiota composition which resembles that of breast-fed infants(Reference Moro, Minoli and Mosca13, Reference Boehm, Fanaro and Jelinek14). Recently, GOS have been shown to competitively inhibit V. cholerae toxin binding to its monosialotetrahexosylganglioside (GM1) receptor on the host cell surface(Reference Sinclair, de Slegte and Gibson15).
Although in vitro studies have shown that Gal, GalNAc and lactose inhibit trophozoite attachment and host cell cytotoxicity(Reference Cano-Mancera and Lopez-Revilla12, Reference Ravdin and Guerrant16), these mono- and disaccharides are mostly digested and absorbed in the small intestine and are usually not found in the colon, the site of E. histolytica colonisation. In contrast, HMO and GOS resist digestion and reach the colon(Reference Gnoth, Kunz and Kinne-Saffran17–Reference Albrecht, Schols and van Zoeren20). Based on their structural composition and their occurrence in the colon, we hypothesise that HMO and/or GOS interact with E. histolytica attachment and cytotoxicity. The present study aimed to examine the effects of isolated HMO and GOS on E. histolytica trophozoite viability, attachment and cytotoxicity in in vitro co-cultures with human enterocytes.
Materials and methods
Human milk oligosaccharide standards
For the present study, 2′-fucosyllactose (2′FL), 3-fucosyllactose, 3′-sialyllactose, 6′-sialyllactose, lacto-N-tetraose (LNT) and lacto-N-fucopentaose 1 (LNFP1) were purchased from V-Laboratories. LNFPx, the mixture of LNFP1, lacto-N-fucopentaose 2 (LNFP2) and lacto-N-fucopentaose 3 (LNFP3), was generously provided by Dr James Paulson (The Scripps Research Institute, La Jolla, CA, USA). GOS syrup (Vivinal, DM 75 %) was generously provided by Friesland Campina Domo. Apart from 59 % GOS, the product also contains 21 % lactose, 19 % Glc and 1 % Gal.
Isolation of pooled human milk oligosaccharides from human milk
Human milk was obtained from eight healthy volunteers recruited at the UCSD Medical Center (San Diego, CA, USA) and approved by the University's Institute Review Board. Oligosaccharides were isolated from pooled human milk as previously described(Reference Kunz, Rudloff and Hintelmann21). Briefly, after centrifugation, the lipid layer was removed and proteins were precipitated from the aqueous phase by the addition of ice-cold ethanol and subsequent centrifugation. Ethanol was removed from the HMO-containing supernatant by roto-evaporation. Lactose and salts were removed by size-exclusion chromatography.
Cell culture
Trophozoites of the E. histolytica strain HM-1:IMSS (isolated from a human subject in Mexico, obtained from the Instituto Mexicano del Seguro Social, Mexico City) were grown axenically in 9 ml glass screw cap tubes in 8 ml TYI-S-33 medium at 37°C(Reference Diamond, Harlow and Cunnick22). Log-phase trophozoites were harvested by chilling at 4°C, concentrated by centrifugation (300 g, 5 min) and resuspended in the appropriate medium at the specified concentration (see next).
The human colon adenocarcinoma HT-29 cell line was cultured in Dulbecco's modified Eagle's medium supplemented with 10 % heat-inactivated fetal calf serum, 60·6 μg/ml penicillin and 100 μg/ml streptomycin in a 5 % CO2 incubator at 37°C. Following this, 7·5 × 104 cells were seeded in wells of a ninety-six-well plate and used after reaching confluency (5 d).
Attachment assay
E. histolytica trophozoites were resuspended at a concentration of 1 × 105 cells/ml TYI-S-33 and allowed to attach to the glass of a 2 ml vial for 1 h at 37°C. Subsequently, the medium was replaced and attached trophozoites were incubated with different concentrations of HMO or GOS in TYI-S-33 for specified times at 37°C. At the end of the incubation, tubes were inverted three times and detached trophozoites were collected and kept on ice. The remaining attached trophozoites were detached in ice-cold PBS for 5–10 min. Detached and ‘attached’ trophozoites were separately concentrated by centrifugation (300 g, 5 min, 4°C) and counted in a Bürker chamber. The sum of attached and detached trophozoites was considered 100 %. To assess reattachment, detached trophozoites were washed and resuspended in fresh TYI-S-33 and allowed to reattach for 2 h at 37°C.
Cytotoxicity assay
E. histolytica trophozoites were resuspended at a concentration of 5 × 105 cells/ml in 100 μl/well HT-29 growth medium in the presence or absence of HMO or GOS, seeded onto HT-29 cell monolayers and incubated at 37°C for the time indicated. Control cell layers were incubated alone or in the presence of HMO or GOS. Co-cultures were stopped after 2 h, and the majority of trophozoites were removed by four washes with ice-cold PBS. Co-cultures were fixed with 4 % paraformaldehyde for 10 min at 37°C. Destruction of HT-29 cell layers was measured as described previously(Reference Mirelman, Bracha and Sargeaunt23). Briefly, fixed cell layers were stained with 0·1 % methylene blue for 10 min and washed three times with PBS. The cell-incorporated methylene blue was extracted with 0·1 m-HCl and measured in a spectrophotometer at 660 nm. The extent of HT-29 cell layer destruction is inversely related to the optical density at 660 nm. Because of the day-to-day variability in trophozoite cytotoxicity, we expressed the cytoprotective effect of HMO as ‘% cell rescue’ where untreated co-cultures are considered 0 %.
Entamoeba viability assay
Trophozoites were allowed to attach to the wall of 2 ml glass vials for 1 h at 37°C. Subsequently, the media was replaced and attached trophozoites were incubated with or without 20 mg/ml HMO or 8 mg/ml GOS for 2 h. Cell viability was determined by Trypan blue exclusion.
Separation and identification of human milk oligosaccharides and galacto-oligosaccharides by HPLC with fluorescence detection and MS analysis
HMO and GOS were fluorescently labelled with 2-aminobenzamide and separated by HPLC on an amide-80 column (4·6 mm inner diameter × 25 cm, 5 μm; Tosoh Bioscience) with a 50 mm-ammonium formate–acetonitrile buffer system (L Bode et al., unpublished results). Separation was performed at 25°C and monitored by a fluorescence detector at 360 nm excitation and 425 nm emission.
Peak-fraction samples were lyophilised and taken up in 50 % methanol–water and analysed by MS on a Thermo LCQ Duo Ion trap mass spectrometer equipped with a Nano-ESI-source. MS was carried out in positive-ion mode. Sodiated ions were detected with m/z values increased by 120 Da corresponding to the 2-aminobenzamide label. All mass spectra were acquired over approximately 30 s in a mass/charge range of 300–2000 Da.
Galacto-oligosaccharide fractionation by fast protein liquid chromatography
GOS were subjected to automated size-exclusion fast protein liquid chromatography (FPLC) (BioLogic Duo Flow; Bio-Rad) on a P-2 biogel column (Bio-Rad). Fractions were collected in 1 ml aliquots and their glycan content monitored by spotting 1 μl aliquots onto a silica plate and staining with 5 % H2SO4 in methanol. The forty-two glycan-positive fractions were pooled in threes and the fourteen resulting fractions were adjusted to 8 mg/ml total GOS. Oligosaccharide composition in each fraction was determined by HPLC with fluorescence detection (HPLC-FL).
Enzymatic digest
Following these, 2′FL (1·65 mg, 3·38 μmol) was incubated with or without 10 μl α1,2-fucosidase (New England BioLabs) in 100 μl reaction buffer for 84 h at 37°C. Reactions were stopped by enzyme inactivation at 65°C for 20 min. Complete digest of 2′FL was confirmed by HPLC-FL analysis.
Statistical analysis
Results are expressed as mean values and standard deviations of three independent sets of experiments. Each condition was performed in duplicate for the detachment assays and in three to five replicates for the cytotoxicity assays. Data sets were analysed using one-way ANOVA followed by Dunnett's multiple comparison test. HMO-mediated detachment of E. histolytica trophozoites over time and differences in the cytotoxicity assays in the presence of GOS or the corresponding lactose concentrations were analysed using two-way ANOVA followed by Bonferroni multiple comparison test. To test for differences between individual HMO in the cytotoxicity assay, one-way ANOVA was followed by Bonferroni post-test. The level of significance was set at P < 0·05 (Graph Pad Prism 5.0; GraphPad Software) and indicated with one asterisk; two asterisks indicate high significance (P < 0·01) and three asterisks indicate extreme significance (P < 0·001).
Results
Human milk oligosaccharides detach Entamoeba histolytica trophozoites
Since lactose has been reported to affect E. histolytica attachment and cytotoxicity(Reference Cano-Mancera and Lopez-Revilla12), we first analysed the lactose content of the isolated, pooled HMO by HPLC-FL and found it to comprise less than 2 % of all glycans in our preparation (Fig. 1). We then incubated attached E. histolytica trophozoites with the isolated, pooled HMO at the specified concentrations and times and found that HMO detached E. histolytica trophozoites in a dose-dependent manner (Fig. 2(a)). At 10 mg/ml, the average HMO concentration in mature human milk, HMO detached more than 80 % of the trophozoites within the first 30 min of exposure. Light microscopic observations showed that detached trophozoites rounded up and floated in clusters (Fig. 2(b)). Since trophozoites have been reported to be susceptible to osmolality changes(Reference Gillin and Diamond24, Reference Gillin and Diamond25), we verified that HMO did not alter the osmolality of the media (data not shown). In fact, Trypan blue staining revealed that HMO did not kill the trophozoites. After incubation with HMO (10 mg/ml) for 2 h, 98·1 (sd 2·0) % of the trophozoites remained viable, which was similar to the untreated controls (93·8 (sd 2·6) %). The majority of trophozoites re-attached after the removal of HMO, which confirmed that HMO do not kill the trophozoites (data not shown). We determined the amount and composition of HMO in the culture media after 6 h of incubation with trophozoites and found no significant HMO loss or degradation. This indicates that trophozoites do not metabolise HMO (data not shown).
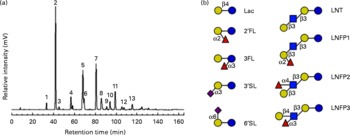
Fig. 1 HPLC with fluorescence detection (HPLC-FL) spectrum of isolated, pooled human milk oligosaccharides (HMO). (a) Representative HPLC-FL chromatogram of pooled HMO. The numbered peaks represent the following oligosaccharides: 1: lactose (Lac); 2: 2′-fucosyllactose (2′FL); 3: 3-fucosyllactose (3FL); 4: 3′-sialyllactose (3′SL); 5: lacto-N-tetraose (LNT); 6: lacto-N-neo-tetraose; 7: lacto-N-fucopentaose 1 (LNFP1); 8: lacto-N-fucopentaose 2 (LNFP2); 9: sialyl-lacto-N-tetraose b; 10: sialyl-lacto-N-tetraose c; 11: lacto-N-difuco-hexaose; 12: disialyl-lacto-N-tetraose; 13: fucosyl-lacto-N-hexaose. (b) Structural comparison of individual HMO. Symbols represent the following monosaccharides: blue circle: glucose; yellow circle: galactose; blue square: N-acetyl-glucosamine; red triangle: fucose; purple diamond: N-acetyl-neuraminic acid. 6′SL, 6′-sialyllactose; LNFP3, lacto-N-fucopentaose 3. (A colour version of this figure can be found online at http://www.journals.cambridge.org/bjn)

Fig. 2 Human milk oligosaccharides (HMO) detach Entamoeba histolytica trophozoites. (a) Attached E. histolytica trophozoites were incubated with isolated, pooled HMO at specified concentrations for 30 min. Detached and attached cells were counted and the percentage of detached cells was calculated and normalised to HMO-untreated controls. Data points represent the means and standard deviations of three independent experiments performed in duplicate. *** Mean values were significantly different (P < 0·001). (b) Representative light microscopic images of control (left) and HMO-treated (right) E. histolytica trophozoites (Eh).
Human milk oligosaccharides reduce Entamoeba histolytica-induced destruction of intestinal epithelial cells
E. histolytica trophozoites kill and phagocytose human intestinal epithelial cells in vivo and in co-culture(Reference Burchard, Prange and Mirelman26). We asked whether HMO can prevent the destruction of intestinal epithelial cells. E. histolytica trophozoites destroyed HT-29 monolayers within 2 h (Fig. 3(a)). Adding HMO at the beginning of the co-cultures rescued most of the HT-29 monolayers from E. histolytica-induced cytotoxicity (Fig. 3(a)). The effects were dose-dependent within a concentration range typical of human milk (Fig. 3(b)). To compare the effects of HMO with the previously described effects of other glycans, we estimated the average molecular weight of HMO as 1000 g/mol. Thus, a 10 mg/ml HMO concentration would equal 10 mm. Based on this assumption, we found that HMO were as effective in preventing E. histolytica-induced cytotoxicity as lactose and much more effective than Gal (Fig. 3(c)). Glc and Fuc alone did not prevent cytotoxicity, which confirms previous reports(Reference Cano-Mancera and Lopez-Revilla12).

Fig. 3 Human milk oligosaccharides (HMO) prevent Entamoeba histolytica-induced destruction of intestinal epithelial cell layers. (a) Representative images of methylene blue stained HT-29 cell layers alone (top) or co-cultured with E. histolytica trophozoites (Eh) in the absence (middle) or presence of HMO (bottom). (b) HT-29 cell layers were co-cultured for 2 h with E. histolytica trophozoites in the presence or absence of HMO and cell layer destruction determined by methylene blue staining. Data points are the means and standard deviations of four independent experiments performed in duplicate and represent the percentage cell rescue. (c) Bars represent the percentage cell rescue of HT-29 cell layers co-cultured with E. histolytica trophozoites in the presence of 10 mm-galactose (Gal), glucose (Glc), lactose (Lac) or fucose (Fuc). The cytoprotective effect of lactose is comparable to that of isolated, pooled HMO in (b). Mean values were significantly different: *P < 0·05, ***P < 0·001. (A colour version of this figure can be found online at http://www.journals.cambridge.org/bjn)
To assess whether HMO can rescue intestinal epithelial cells that had already been in contact with E. histolytica trophozoites, we initiated co-cultures of HT-29 cells and E. histolytica trophozoites in the absence of HMO and then added HMO to the co-cultures after varying periods of time. We found that 31·0 (sd 4·7) % of the intestinal epithelial cells could still be rescued when HMO were added to the co-cultures as late as 90 min after co-culture initiation (Fig. 4(a)). These results suggest that HMO can prevent both initiation and progression of E. histolytica-induced host cell cytotoxicity.
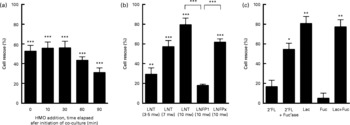
Fig. 4 Human milk oligosaccharides (HMO) rescue existing co-cultures and the effects are structure-dependent. (a) HMO rescue HT-29 intestinal epithelial cell layers when added to existing co-cultures with Entamoeba histolytica trophozoites. HT-29 cell layers were co-cultured with E. histolytica trophozoites in the absence of HMO for the time indicated. At that time, HMO were added to the co-cultures up to a total co-culture time of 2 h. HT-29 cells that were co-cultured with E. histolytica trophozoites in the absence of HMO for the entire 2 h were considered to have 0 % cell rescue. (b) Lacto-N-tetraose (LNT) but not lacto-N-fucopentaose 1 (LNFP1) rescue E. histolytica-induced HT-29 cell destruction. HT-29 cells and E. histolytica trophozoites were co-cultured in the presence of individual HMO. While physiological and superphysiological concentrations of LNT rescued HT-29 cells in a dose-dependent manner, LNFP1 had no effect even at 10 mm. However, LNFPx, a mixture of LNFP1, lacto-N-fucopentaose 2 and lacto-N-fucopentaose 3, rescued HT-29 cells. For structural differences, see Fig. 1(b). (c) Removal of terminal fucose restores cytoprotective effect. While 2′-fuosyllactose (2′FL) did not rescue HT-29 cells from E. histolytica-induced cytotoxicity, removal of the terminal fucose by incubation with fucosidase (Fuc'ase) restored the cytoprotective effect, which was almost as pronounced as that of lactose (Lac) alone. Fucose (Fuc) alone had no effect, but it also did not reduce the effect of lactose. Bars represent the means and standard deviations of four independent experiments. Mean values were significantly different: *P < 0·05, ** P < 0·01, *** P < 0·001.
The protective effects of human milk oligosaccharides are structure-specific
HMO are complex mixtures of structurally diverse oligosaccharides (reviewed in Newburg et al. (Reference Newburg, Ruiz-Palacios and Morrow5), Kunz et al. (Reference Kunz, Rudloff and Baier6) and Bode(Reference Bode8)). To assess which of the individual HMO are effective in the cytotoxicity assay, we tested six commercially available HMO in physiologically relevant concentrations. While lactose is a potent inhibitor of E. histolytica-induced cytotoxicity, it is most remarkable that the fucosylated and sialylated forms of lactose failed to prevent intestinal epithelial cell destruction (data not shown). Of the individual HMO tested, only LNT, a neutral unsubstituted tetrasaccharide (see Fig. 1(b)), significantly protected the intestinal epithelial cells (Fig. 4(b)). In contrast, LNFP1, a fucosylated form of LNT had no effect on rescuing the intestinal epithelial cells. Since the only structural difference between LNT and LNFP 1 is a Fuc residue α1–2-linked to the terminal Gal (see Fig. 1(b)), our findings suggest that the terminal Gal on HMO is required for their cytoprotective effect. To confirm this hypothesis, we tested equimolar concentrations of LNT and LNFP1 in the cytotoxicity assay. LNT had a dose-dependent effect reaching 79·0 (sd 8·3) % cell rescue at 10 mm, whereas the effect of an equimolar concentration of LNFP1 was significantly lower (16·9 (sd 1·7) %) (Fig. 4(b)). Interestingly, a 10 mm mixture of the isomers LNFP1, LNFP2 and LNFP3 consisting of 62 % LNFP1 had a significantly higher effect (61·7 (sd 5·5) %) than 10 mm-LNFP1 alone. This suggests that LNFP2 and/or LNFP3 strongly inhibit E. histolytica-induced cytotoxicity. Both LNFP2 and LNFP3 are substituted by Fuc at the subterminal GlcNAc, leaving the terminal Gal accessible (see Fig. 1(b)). This supports our hypothesis that the terminal Gal is required for the cytoprotective effect of HMO. To further verify this hypothesis, we used 2′FL (see Fig. 1(b)), which also carries an α1–2-linked Fuc residue at the terminal Gal, and removed the Fuc with a specific α1–2-fucosidase as confirmed by HPLC-FL and MS (data not shown). While the intact 2′FL had no cytoprotective effect, enzymatic removal of Fuc from the terminal Gal resulted in a significant cytoprotective effect similar to that of equimolar concentrations of either lactose alone or a mixture of lactose and Fuc (Fig. 4(c)). These results strongly suggest that the blockage of the terminal Gal, not the presence of Fuc per se, renders 2′FL ineffective.
Galacto-oligosaccharides prevent Entamoeba histolytica-induced cytotoxicity independent of its lactose content
GOS, which are widely added to infant formula as a prebiotic, consist of varying numbers of Gal residues attached to a single Glc at the reducing end. Since our data indicate that terminal Gal is essential for the cytoprotective effect of HMO, we tested whether GOS are also effective against E. histolytica-induced cytotoxicity. We found that GOS indeed protect HT-29 cell monolayers from destruction by trophozoites in a dose-dependent manner (Fig. 5(a)). At only 5 mg/ml, GOS fully protected HT-29 cells from E. histolytica-induced cytotoxicity.
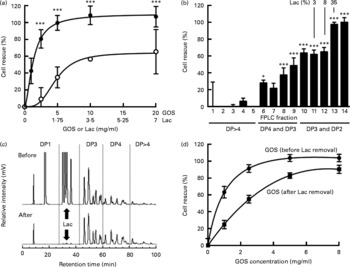
Fig. 5 Galacto-oligosaccharides (GOS) prevent Entamoeba histolytica-induced destruction of intestinal epithelial cells and the effects are lactose-independent. (a) Bars show the % cell rescue of HT-29 cell layers co-cultured with E. histolytica trophozoites and treated with GOS (●) or the concentration of lactose (Lac) (○) that is present in GOS (35 %). Data points represent the means and standard deviations of four independent experiments. *** Mean values were significantly different between the effects of the GOS preparation and the effects of lactose alone (P < 0·001). (b) Cytoprotective effects of GOS size-exclusion chromatography fractions. Fast protein liquid chromatography (FPLC) size-exclusion chromatography was used to remove lactose from the GOS preparation. Early fractions contain oligosaccharides with higher molecular weights (more monosaccharide residues, higher degree of polymerisation (DP)). Later fractions contain oligosaccharides with lower molecular weight, including lactose (% lactose is given above the bars). Bars represent the means and standard deviations of four independent experiments. (c) HPLC with fluorescence detection chromatograms of the GOS preparation before (top) and after FPLC size-exclusion chromatography (bottom). (d) Cytoprotective effects of lactose-containing GOS before FPLC size-exclusion chromatography (●) and lactose-depleted GOS (■). Data points represent the means and standard deviations of four replicates.
GOS are synthesised enzymatically by the addition of Gal to lactose. Hence, the resulting mixture of oligosaccharides also contains unreacted lactose and monosaccharides. To rule out that the cytoprotective effect is due to the unreacted lactose only, we tested lactose at the respective concentrations present in the GOS preparation and found that the effects of lactose alone were significantly lower than the effects of the lactose-containing GOS preparation (Fig. 5(a)). These results suggest that the GOS preparation contains oligosaccharides other than lactose that prevent cytotoxicity. To confirm these results, we separated the oligosaccharides in the GOS preparation by size-exclusion chromatography and determined the lactose content and cytoprotective effect of the resulting fourteen fractions. Consistent with their high lactose content, fractions 13 and 14 have a high cytoprotective effect on co-cultures (Fig. 5(b)). HPLC-FL analysis of the different FPLC fractions followed by MS analysis of the HPLC peaks showed that FPLC fractions 10–12 contain almost exclusively trisaccharides (degree of polymerisation (DP)3) and hardly any lactose. These fractions had significant cytoprotective effects. Fractions 6–9 contain predominantly tetrasaccharides (DP4) and mediate lower but still significant cell rescue. Early eluted fractions containing larger oligosaccharides (DP4–DP8) had no effect on cytotoxicity, which might be due to the much lower abundance of oligosaccharides DP>4 in the GOS preparation (Fig. 5(c)). We prepared lactose ‘free’ GOS ( < 0·5 % lactose) by pooling all GOS fractions with a lactose content ≤ 3 % and compared the cytoprotective effect of the pooled fractions to equal concentrations of the lactose-containing GOS preparation (Fig. 5(d)). In accordance with the additional effect of GOS compared to lactose alone (Fig. 5(a)), we found a dose-dependent cell rescue effect in lactose-free GOS, indicating a lactose-independent effect of GOS. Remarkably, at 8 mg/ml, the concentration used to supplement infant formula, lactose-free GOS almost completely rescued E. histolytica-induced destruction of intestinal epithelial HT-29 cells. Similar to HMO, GOS are not toxic to trophozoites (96·6 (sd 1·3) % viable compared to 93·8 (sd 2·6) % in the control), detach trophozoites from glass (81·3 (sd 7·3) % detachment within 30 min compared to control) and provide substantial protection to cell layers in established co-cultures with E. histolytica trophozoites (Fig. 5(d)). These results confirm that the protective effect of GOS is lactose-independent and comparable to the effects of pooled HMO.
Discussion
We have shown that physiological concentrations of HMO and formula-based concentrations of GOS inhibit E. histolytica attachment and cytotoxicity to enteric cell layers in vitro in a dose-dependent manner. Interestingly, HMO and GOS also rescued cell layers from E. histolytica-induced cytotoxicity when added after the initiation of the co-cultures, indicating that HMO can halt ongoing cytotoxicity.
HMO did not affect attachment and viability of the mucosal protozoan pathogen Giardia lamblia (T Lauwaet, unpublished results), suggesting that HMO interfere with E. histolytica-specific attachment mechanisms. We speculate that HMO bind to the Gal/GalNAc lectin on the surface of E. histolytica trophozoites. The Gal/GalNAc lectin mediates trophozoite attachment and invasion and consists of three subunits: heavy, intermediate and light. The extracellular part of the heavy subunit contains a carbohydrate recognition domain and can be inhibited by lactose, Gal and N-acetyl-galactosamine but not by Glc and Fuc(Reference Cano-Mancera and Lopez-Revilla12). We found that the cytoprotective effects of HMO and GOS are not due to contaminating lactose as our isolated, pooled HMO preparation was virtually lactose free (Fig. 1(a)). Similarly, the GOS preparation still prevented E. histolytica attachment and cytotoxicity after lactose removal by size-exclusion chromatography. These findings are important since most of the lactose is cleaved and absorbed in the small intestine, while HMO and GOS are only minimally digested and reach the colon(Reference Gnoth, Kunz and Kinne-Saffran17, Reference Engfer, Stahl and Finke18), the site of E. histolytica colonisation.
Both HMO and GOS are heterogeneous mixtures of structurally diverse oligosaccharides with potentially very different effects. Here, we have shown that the cytoprotective effects of HMO are indeed structure-specific and that a free terminal Gal is necessary to prevent E. histolytica attachment and cytotoxicity. HMO are not cytoprotective if the terminal Gal is covered with an α1–2-linked Fuc as it is the case for 2′FL and LNFP1. Considering the inter-individual and genetic differences in HMO composition found in the milk of different women(Reference Thurl, Munzert and Henker27, Reference Coppa, Gabrielli and Zampini28), these results may have important physiological relevance. Evidently, 2′FL and LNFP1 are among the most abundant oligosaccharides in the milk of so-called secretor women that express a specific α1–2-fucosyltransferase. In contrast, 2′FL and LNFP1 are completely absent in the milk of non-secretor women who lack α1–2-fucosyltransferase. Based on our in vitro results, one could hypothesise that the milk of non-secretor women confers better protection against E. histolytica infections than the milk of secretor women, where the majority of HMO is covered with α1–2-linked Fuc. The opposite has been shown for C. jejuni infections. C. jejuni binds to α1–2-fucosylated oligosaccharides(Reference Ruiz-Palacios, Cervantes and Ramos29), and the milk of secretor women provides infants with a better protection against C. jejuni infections(Reference Morrow, Ruiz-Palacios and Altaye30). These results clearly demonstrate that some of the beneficial effects of HMO are highly structure-specific and that the amount and composition of oligosaccharides in mother's milk confer different degrees of protection against specific pathogens.
Despite vast improvements in glycan synthesis over the last few years, the still relatively high costs of chemical or enzymatic oligosaccharide synthesis are likely to limit the use of individual HMO as templates for anti-amoebic therapies, especially in developing countries, where amoebiasis is most prevalent. GOS, however, can be produced in large quantities and at low costs and have been used in infant formula for almost a decade now. However, the high lactose concentration in crude GOS preparations may be difficult to tolerate for amoebiasis patients who often present with secondary lactose intolerance(Reference Rana, Bhasin and Vinayak31). Instead, the use of refined, lactose-depleted GOS preparations might be an affordable anti-amoebic therapy.
Our results suggest that HMO contribute to the anti-amoebic effects of human milk, which may help explain why breast-fed infants are at lower risk to acquire E. histolytica infections(Reference Islam, Stoll and Ljungstrom2). Future in vivo studies are needed to confirm that regular intake of HMO and GOS can prevent and/or treat E. histolytica infections. HMO and GOS are heat tolerant, stable, safe and in the case of GOS, inexpensive. Since most areas with a high rate of E. histolytica infections also have an extreme climate and very limited resources, heat tolerance, stability and low costs are valuable characteristics that make these oligosaccharides ideal candidates as alternative preventive and therapeutic anti-amoebic agents.
Acknowledgements
We are grateful to Ken Hirata for providing us with E. histolytica culture media, to Kirin Demuynck for help with the initial attachment experiments, and Caroline Nissan and Lauren Hsiao for their technical assistance with HMO purification and analysis. We thank Dr James Paulson at The Scripps Research Institute, La Jolla, CA, for kindly providing us with LNFPx and Friesland Campina Domo for their generous gift of Vivinal GOS. This project has been funded in part by National Institutes of Health (NIH) grants K99/R00 DK078668 (L. B.), R03 HD059717S1 (L. B.) and R01 AI42488 (F. D. G.). The contributions of the authors to this study were as follows: L. B. and T. L. designed the research; E. J.-K., T. L. and L. A. B. conducted the research; L. B., E. J.-K. and T. L. analysed the data; E. J.-K., T. L., S. L. R., F. D. G. and L. B. wrote the paper. L. B. had primary responsibility for the final content. All authors read and approved the final manuscript. E. J.-K., T. L., L. A. B., S. L. R., F. D. G. and L. B. have no conflicts of interest to declare.