Chemotherapy is the most common and widely used cancer treatment therapy. It consists of a drug mix that targets the neoplastic cells, promoting their elimination(Reference Wilkes1). Unfortunately, chemotherapeutic agents are not specific for cancer cells, they also affect the host’s cells, such as the immune and gastrointestinal epithelium cells, with a high proliferation rate(Reference Sak2). Chemotherapy can trigger some side effects, including nausea, vomiting, inappetence, diarrhoea and, the most common, mucositis(Reference Longley, Harkin and Johnston3,Reference Yeung, Chan and Jiang4) . The frequency of mucositis is approximately 40 % in patients under antineoplastic therapy and almost 100 % in patients with head and neck cancer undergoing chemotherapy(Reference Yeung, Chan and Jiang4,Reference Peterson, Bensadoun and Roila5) .
Mucositis is characterised by intense inflammation associated with pain, dysphagia, weight loss, diarrhoea and bleeding. It also affects epithelial barrier integrity, which allows bacterial colonisation, and invasion and triggering of inflammatory process(Reference Maria, Eliopoulos and Muanza6,Reference Ribeiro, Wanderley and Wong7) . Despite the high frequency of mucositis, there is no effective therapy for this pathological condition. However, current evidence has suggested gut microbiota modulation as a potential treatment(Reference Touchefeu, Montassier and Nieman8).
Fibre is a component derived from fruits, nuts and vegetables and serves as a substrate to the host’s commensal microbiota influencing its composition(Reference Dhingra, Michael and Rajput9). Physiological host benefits of diets enriched with fibre include not only the decrease of intestinal transit time and increase of faecal bulk excretion but also the reduction of obesity, risk of type 2 diabetes, arthritis and colon cancer, making it a widely recommended health diet(Reference Anderson, Baird and Davis10). In previous studies, our research group evaluated the beneficial effect of a soluble fibre diet, pectin, on colitis and gout arthritis, using an experimental murine model as the approach(Reference Macia, Tan and Vieira11,Reference Vieira, Galvao and Macia12) . Indeed, dietary pectin modulates bacterial communities in the gut in a beneficial way(Reference Larsen, Bussolo de Souza and Krych13).
The soluble fibre diet has been useful in treating inflammatory diseases, and the beneficial effects were related to the production of SCFA, microbial metabolites, which has the colon as the major production site requiring the presence of colonic bacteria(Reference Tan, McKenzie and Potamitis14). To date, many reported fibre effects have been associated with microbiota modulation and its ability to produce SCFA, like propionate, acetate and butyrate(Reference Thorburn, Macia and Mackay15). Acetate is the most produced SCFA in the colon. It is released systemically to the peripheral venous system, explaining its higher concentration in the blood(Reference Tan, McKenzie and Potamitis14), and is preferentially produced by pectin fermentation(Reference Gill, van Zelm and Muir16). Although butyrate has been shown to reduce inflammation in an experimental model of mucositis induced by 5-FU(Reference Ferreira, Leonel and Melo17), the role of acetate in mucositis still needs to be addressed. Among the benefits of high-fibre (HF) diet, its role in gut microbiota modulation and SCFA production has been shown to be important to the host by promoting gut homoeostasis(Reference Macia, Tan and Vieira11).
Currently, the crucial role of gut microbiota on mucositis development has been reported(Reference Roy and Trinchieri18,Reference Alexander, Wilson and Teare19) . It has been shown that germ-free mice are more resistant to 5-FU-induced mucositis and irinotecan, which reinforces the hypothesis that the microbiota is essential for the development of the disease(Reference Pedroso, Vieira and Bastos20,Reference Brandi, Dabard and Raibaud21) . Among the mice models used to evaluate the pathobiology of mucositis, the C57BL/6 strain is an attractive model due to its immunogenicity and because it is often used as a background strain of spontaneous or induced mutant mice(Reference Song and Hwang22,Reference Fontaine and Davis23) . Considering that gut microbiota modification plays a role in mucositis development, fibre dietary components may interfere with it. Furthermore, since the efficiency of current therapies in alleviating mucositis has been questioned, we sought to investigate here the effects of pectin-fibre diet and the microbial metabolite acetate on mucositis. We also addressed the effects of complete lack of dietary fibre on host microbiota composition, and inflammation induced by the chemotherapy drug irinotecan, in a murine model of mucositis.
Experimental methods
Animals
All experiments described in the present paper were conducted on female C57BL/6 mice (6–8 weeks old) obtained from the Animal Facility of the Federal University of Minas Gerais. Animals were kept in polycarbonate mini-isolators under controlled conditions: temperature around 22 (sd 1) °C, humidity of 60 ± 10 % and photoperiods of 12 h light/dark. Animals had free access to food and water. The experiments were in compliance with the National Council for Animal Experiments Control (CONCEA, Brazil) and were approved by the local Ethics Committee for Animal Use (CEUA-UFMG, protocol no. 186/2012). In order to avoid/decrease stress and aggression, nesting material and shelters were provided in each experimental group cage. Experiments were performed at the Microbiology Department animal facility (Institute of Biological Science, Federal University of Minas Gerais).
Diet and acetate treatment
Mice were fed either AIN-93M(Reference Reeves, Nielsen and Fahey24) or one of the two AIN-93M modified diets: HF diet, containing 15 % pectin, or low-fibre (LF) diet, containing low/zero fibre content. Composition of each fibre can be seen in Table 1. Mice were treated with sodium acetate (Sigma-Aldrich), 5 d prior to and during the mucositis induction. Acetate was added to the sterile drinking water of mice ad libitum at 150 mm(Reference Vieira, Galvao and Macia12). The water solution was changed every day to avoid contamination and significant changes.
Table 1. Diets used in this study

Experimental design
Mice were randomly separated into eight groups (six animals per group) and were fed control, HF or LF diet: control (CT), mucositis (MUC), control + high fibre (HF CT), mucositis + high fibre (HF MUC), control + low fibre (LF CT), mucositis + low fibre (LF MUC), control + acetate (CT + AC) and mucositis + acetate (MUC + AC). Mucositis induction started on day 10, and daily doses of irinotecan (75 mg/kg) were administered for three consecutive days, between 09.00 and10.00 hours, via intraperitoneal (i.p.), to the MUC groups. Control groups received PBS i.p. at the same time. Mice were euthanised on day 14 after being anaesthetised with a ketamine (80 mg/kg) and xylazine (15 mg/kg) mixture. Blood, intestines, faeces and femurs were collected for analysis. Body weight was measured daily after mucositis induction. Animals were visually assessed daily for evaluation of normal appearance and behavioural aspects.
Clinical score
Clinical scoring was performed using a range from 0 (no clinical symptoms) to 5 (maximal symptoms). Symptoms were evaluated using diarrhoea and faecal blood. Diarrhoea was assessed as previously described(Reference Lima-Junior, Freitas and Wong25), using the following grade score: 0 – normal stool, 1 – slightly wet and soft stool, 2 – wet and unformed stool and 3 – watery stool. Faecal blood was evaluated as previously described(Reference Arifa, Paula and Madeira26) using Hemoccult cards (INLAB-Diagnostica), and the grade score was based on the following criteria: 0 – no blood, 1 – trace of blood and 2 – presence of blood. The sum of the criteria was used to obtain the clinical score.
Intestinal myeloperoxidase and eosinophil peroxidase activity assays
Small intestine samples were collected to evaluate the white blood cell influx to intestinal tissue, especially neutrophils and eosinophils(Reference Vieira, Fagundes and Alessandri27,Reference Strath, Warren and Sanderson28) . Briefly, 100 mg of tissue was homogenised in 1·9 ml PBS (pH 7·4) using a tissue homogeniser. The homogenate was centrifuged (3000 g for 10 min), then the pellets were subjected to hypotonic lysis (1·5 ml of 0·2 % NaCl) and osmolarity was restored with 1·5 ml of a 1·6 % NaCl solution containing 5 % glucose. The samples were centrifuged again (3000 g for 10 min) and the pellets were resuspended in PBS containing 0·5 % hexadecyltrimethylammonium bromide. The tissue suspension was homogenised again, and the homogenates were freeze-thawed three times in liquid N2 and centrifuged for 15 min at 3000 g . The resulting supernatant was used to measure eosinophil peroxidase (EPO) and myeloperoxidase (MPO) activities.
For EPO assessment, 75 μl of supernatant was added to 75 μl of 1·5 mm o-phenylenediamine (Sigma-Aldrich), diluted in 0·075 mm Tris–HCl and 6·6 mm H2O2 and incubated at 37 °C for 30 min. For MPO quantification, 25 μl of supernatant was added to 25 μl of 1·6 mm 3,3,5,5′-tetramethylbenzidine (Sigma-Aldrich) in dimethyl sulfoxide (Sigma-Aldrich). After the addition of 100 μl 0·5 mm H2O2, the solution was incubated at 37 °C for 5 min. Both reactions were stopped by adding 50 μl of 1 m H2SO4. Absorbance was measured at 492 nm (EPO) and 450 nm (MPO) using a microplate spectrophotometer (Epoch; BioTek Instruments, Inc.) and the results were expressed as absorbance units.
Intestinal permeability
The chemotherapy toxic effect may weaken the intestinal epithelial barrier, allowing the lumen content to cross the paracellular space into the blood. Intestinal permeability was assessed either by blood radioactivity of diethylenetriamine pentaacetic acid labelled with 18·5 MBq of technecium-99 m (99mTc) or by serum fluorescence of dextran labelled with fluorescein isothiocyanate (FITC) conjugated. The diethylenetriamine pentaacetic acid is a probe that, under normal conditions of intestinal mucosa, is not absorbed in major quantities. Mice received 0·1 ml of 99mTc-diethylenetriamine pentaacetic acid containing 18·5 MBq 99mTc by gavage at the end of the experiment. After 4 h, the animals were euthanised and blood was collected, weighted and placed in appropriate tubes for radioactivity determination using a Wallac Wizard 1470-020 automated gamma counter (Perkin Elmer). The results were expressed as the percentage of injected dose per g (%ID/g) of blood.
The intestinal permeability assay by FITC-dextran was performed as described previously(Reference Canesso, Lacerda and Ferreira29). Briefly, mice had food and water withheld overnight. The animals then received oral administration of FITC-dextran (50 mg per 100 g of body weight in a 20 mg/ml concentration, MW 4000; Sigma-Aldrich). After 4 h, the serum was collected by cardiac puncture and fluorescence intensity was measured for each sample (excitation 492 nm; emission 525 nm). Whole blood FITC-dextran concentration was determined based on a standard curve generated by serial dilutions of FITC-dextran. Data are presented as fluorescence intensity of FITC-dextran in blood.
Myelosuppression
To assess myelosuppression, an incision was made in the region above the acetabulum, allowing removal of the femur and tibia. The muscle was removed in order to expose the medullary canal, then the epiphyseal ends were cut. The marrow was washed with a 26-gauge syringe using PBS 1X. Next, the cell suspension was homogenised and centrifuged at 1200 rpm for 10 min. The pellet obtained was resuspended in 2 ml of 1X erythrocyte lysis solution and incubated at 37°C for 5 min. Afterward, 4 ml of 1X PBS was added and the solution obtained was centrifuged at 1200 rpm for 5 min. The supernatant was discarded and the pellet resuspended in 1 ml of PBS 1X. For total cell count, 5 μl of the cell suspension was diluted in 95 μl of Turk’s solution. Finally, 10 μl of the sample was used for counting in a Neubauer chamber. The number of cells is in the range of 107 cells/ml.
Measurement of IL1β expression by real-time PCR
To evaluate IL1β cytokine expression, ileum samples were collected on the last day of the experimental protocol and placed in RNase-free microtubes containing 150 µl of RNAlater solution (Thermo Fisher). Then, the samples were stored at –20 °C until the time of analysis. The tissues were submitted to three stages: (1) RNA extraction; (2) synthesis of the complementary DNA; (3) amplification of complementary DNA by real-time PCR.
Total RNA was extracted from the ileum using the TRIzol reagent (Ludwig Biotec). The quality and quantity were evaluated, respectively, using 1·5 % agarose gel and the NanoDrop® 2000 spectrophotometer (Thermo Scientific). Next, the RNA samples were purified with DNAse I (Invitrogen) to remove any genomic DNA that could be present in the sample and interfere with the analysis. Subsequently, the DNAse I was inactivated, and complementary DNA was generated from 2 µg of RNA following the instructions of the Applied Biosystems™ High-Capacity complementary DNA Reverse Transcription kit (Thermo Fisher).
Quantitative PCR was performed using the Applied Biosystems 7900HT Fast Real Time PCR System, with the PowerUp™ SYBR® Green Master Mix (Thermo), under the following conditions: 50 °C for 2 min, 95 °C for 10 min and 40 cycles at 95 °C for 15 s and 60 °C for 1 min. The primers used in quantitative PCR amplification are listed in Table 2. Target gene expression was analysed using the 2−ΔΔCt method, with β-actin as an endogenous reference.
Table 2. Nucleotide sequence for primers forward and reverse of genes Muc1, Muc2, IL1β, TNF and β-actin

Intestinal histology
On the last day of the experiment, the mice were euthanised and small intestines were removed for length measurement; histological analysis was performed in the ileum. The intestines were placed on filter paper, measured and longitudinally opened. The lumen content was carefully washed, and rolls were made from the distal to the proximal ends. The rolls were fixed in buffered 4 % formaldehyde and processed for paraffin embedding. Histological sections of 4 µm were mounted on glass slides and stained with haematoxylin and eosin(Reference Sheikh and Solito30) for further evaluation of the following parameters: alterations in mucosal architecture and polymorphonuclear cells infiltrate. These criteria ranged from 0 to 3 points according to the area affected by cell infiltration (none, slight, moderate, severe), and the sum was used to obtain a histological score. The slides were coded and analysed by a single pathologist who was unaware of the experimental conditions of each sample.
Culture-dependent method for microbiota evaluation
Evaluation of microbiota composition was conducted using the culture-dependent method. Serial dilutions of equal amounts of intestinal contents collected from different parts of intestine (small, cecum and large) (1:100 w/v) at the end of the experiment were plated onto MacConkey (Difco), Sabouraud (Difco), MRS (Merck), Blood and Supplemented blood (0·1 % hemin, 0·1 % menadione and 5 % yeast extract) agar. The base for blood agar was Tryptic Soy agar (Difco), and for supplemented blood agar was Brucella agar (Difco). Samples (100 µl) were plated onto each culture medium and incubated at 37ºC either for 2 d in aerobic atmosphere (Blood, Sabouraud, MRS and MacConkey agar) or for 7 d in anaerobic atmosphere (MRS and Supplemented Blood agar). After incubation, the colonies were counted and data were expressed as the log of colony-forming units per g of faeces.
SCFA concentration
SCFA concentration was measured in stool and serum. Stool samples were extracted and resuspended in a 1:6 proportion (w/v), while serum samples were diluted in a 1:3 proportion (v/v) in H2SO4 0·01 N and homogenised in vortex. Then, the samples were centrifuged at 20 000 g for 30 min and the supernatant was filtered through a cellulose acetate membrane with 0·2 µm pore. A HPLC system (Shimadzu Corp.) was used to dose the samples of faeces and serum. For both stool and serum, 5 μl of the samples was injected into the equipment column. Seven-point external calibration curves were adopted to quantify acetate, butyrate and propionate in faecal and serum samples, using analytical grade SCFA (Sigma-Aldrich) as standards. SCFA concentrations were expressed in µmol/mg of wet stool and in µmol/ml of serum.
Statistical analysis
Data are presented as mean values with their standard errors. The Kolmogorov–Smirnov test was used to check for normal distribution of the data. One-way ANOVA followed by Newman–Keuls’ post hoc analysis was used to compare normalised data among all groups. Two-way ANOVA followed by the Bonferroni test was used to compare the variation of body weight among the experimental groups. The GraphPad Prism 5.0 software was used to analyse all data, and P < 0·05 was considered as statistically significant.
Results
High-fibre diet attenuated intestinal shortening and improved clinical parameters
To evaluate the effects of HF diet in mucositis, we first analysed the body weight. As expected, the mice submitted to mucositis (MUC) lost 10 % of body weight when compared with the control group. However, the HF diet (MUC + HF) and water with acetate (MUC + AC) added were not able to reverse the weight lost due to mucositis (Fig. 1(a)). The clinical scores were evaluated to detect the presence of blood in the stool and diarrhoea. Mice submitted to mucositis showed increased clinical scores, as is frequently seen in mucositis. Acetate-treated mice (MUC + AC) showed no significant decrease in clinical scores, while mice receiving the HF diet (MUC + HF) showed significant improvement in this matter when compared with the MUC group (Fig. 1(b)). To evaluate the effect of the HF diet and acetate treatment on mouse anatomy during mucositis, we measured the lengths of the animals’ intestines. Decrease in intestinal length was observed in mice submitted to mucositis (MUC) when compared with the control group, as expected. The HF diet prevented intestine shortening, and intestine length was significantly increased in the MUC + HF group when compared with the MUC group (Fig. 1(c)). Acetate treatment did not prevent intestine shortening. Also, water consumption was not affected by acetate added to the drinking water (online Supplementary Fig. 1).

Fig. 1. High-fibre (HF) diet improved clinical parameters on mucositis (MUC)-induced mice. Mice were either treated with acetate (AC – 150 mm) in drinking water or fed with control (CT) or HF diets (n 6 per group). Mucositis was induced with three doses of irinotecan (75 mg/kg per d – groups: MUC, MUC + AC and MUC + HF) and all animals were euthanised on day 14. Body weight (a) was accessed daily after mucositis induction and clinical score (b) and intestine length (c) were evaluated on the last day of the experiment. * Statistically significant differences compared with the MUC group (P < 0·05). Bars show mean values with their standard errors for six mice/group. Statistically significant differences compared with the control group: † P < 0·05, †† P < 0·01. Statistical analysis for body weight was done using two-way ANOVA, followed by Bonferroni’s post-test. Clinical score and intestine length analysis were performed using one-way ANOVA, followed by Newman–Keuls’ post-test. , CT;
, CT + HF;
, CT + AC;
, MUC;
, MUC + HF;
, MUC + AC.
High-fibre diet ameliorates inflammation and intestinal permeability
Histological analysis was performed to evaluate the capacity of a HF diet or acetate to protect crypt and villi from chemotherapy side effects. The destruction of the epithelial architecture in the intestine with the absence of crypt and villi (Fig. 2(a)) was observed in the chemotherapy-induced mucositis group (MUC), evidenced by an increase in the histopathological parameters (Fig. 2(b)). This effect was reversed in the MUC + HF group; short villi and crypt were seen, resembling the control group (Fig. 2(a)). Acetate treatment did not limit the tissue damage.

Fig. 2. Treatment with a high-fibre (HF) diet or acetate (AC) ameliorates inflammation and intestinal permeability and preserves tissue architecture. Mucositis (MUC) was induced using one daily dose of irinotecan (75 mg/kg per d) for 3 d (groups: MUC, MUC + AC and MUC + HF). Mice were either treated with acetate (AC − 150 mm) in drinking water or fed with control (CT) or HF diets (n 6 per group). Small intestine histology (a) and histopathological score (b). Mice received oral administration of fluorescein isothiocyanate conjugated dextran (FITC-dextran) by gavage to determine intestinal permeability using serum fluorescence (c). Evaluation of inflammatory infiltrate by measurement of myeloperoxidase (d) and eosinophil peroxidase (e) activity. mRNA levels of muc1 (f) and muc2 (g) in the ileal tissue determined by quantitative PCR. Bars show mean values with their standard errors for six mice/group. Statistically significant differences compared with the MUC group: * P < 0·05, ** P < 0·01. †† Statistically significant difference compared with the control group (P < 0·01). Statistical analysis was performed using one-way ANOVA, followed by Newman–Keuls’ post-test.
Intestinal permeability was measured, and only the MUC + HF group was able to maintain epithelial barrier integrity and decrease intestinal permeability at almost the same levels as the control group (Fig. 2(c)). The MPO and EPO levels were measured to evaluate the degree of inflammation in the gastrointestinal tract. Groups fed the HF (MUC + HF) and acetate (MUC + AC) diets showed a decrease in the neutrophil (MPO) and eosinophil (EPO) influx when compared with the MUC group (Fig. 2(d) and (e)). This suggests that acetate supplementation and a HF diet ameliorate the inflammation in mucositis.
We evaluated two distinct structural and functional members of the MUC gene family, MUC2 (secreted gel-forming mucin) and MUC1 (transmembrane mucin), in order to determine the expression of mucins in the intestine of mice submitted to mucositis. There was no difference in MUC1 expression among all groups (Fig. 2(f)). MUC2 expression was increased in the control mice, which received the HF diet. The expression in any mucositis group was very low (Fig. 2(g)).
While the high-fibre diet improves microbiota composition and SCFA production, the low-fibre diet reduces production of SCFA and potentialises microbial dysbiosis
To evaluate the changes in microbiota population in faeces, the mice were submitted to mucositis and treated with a HF diet and water with acetate. Here, we found that the control group has a higher prevalence of anaerobic cultivate bacteria while, in mice submitted to mucositis, the anaerobic bacteria disappear and the aerobic bacteria predominate (Fig. 3(a) and (b)). Fibre supplementation maintained the increased levels of anaerobic bacteria in the CT + HF group (Fig. 3(c)) and prevented the prevalence of aerobic bacteria and the disappearance of anaerobic bacteria in the MUC + HF group (Fig. 3(d)). Oral supplementation of acetate had a negative impact on microbiota composition in both the CT + AC and MUC + AC groups (Fig. 3(e) and (f)), showing high levels of aerobic bacteria and low levels of anaerobic bacteria. Additionally, treatment with the HF diet also increased the endogenous levels of acetate in faeces, suggesting that HF treatment was able to modulate not only the gut microbiota composition but also its metabolic ability to produce SCFA (Fig. 3(g)). On the other hand, acetate concentration in the faeces of mice treated orally with acetate was not significantly different compared with the CT and MUC groups (online Supplementary Fig. 2). This suggests that oral supplementation of acetate did not modulate the gut microbiota beneficially and, consequently, did not change the endogenous production of acetate during mucositis.
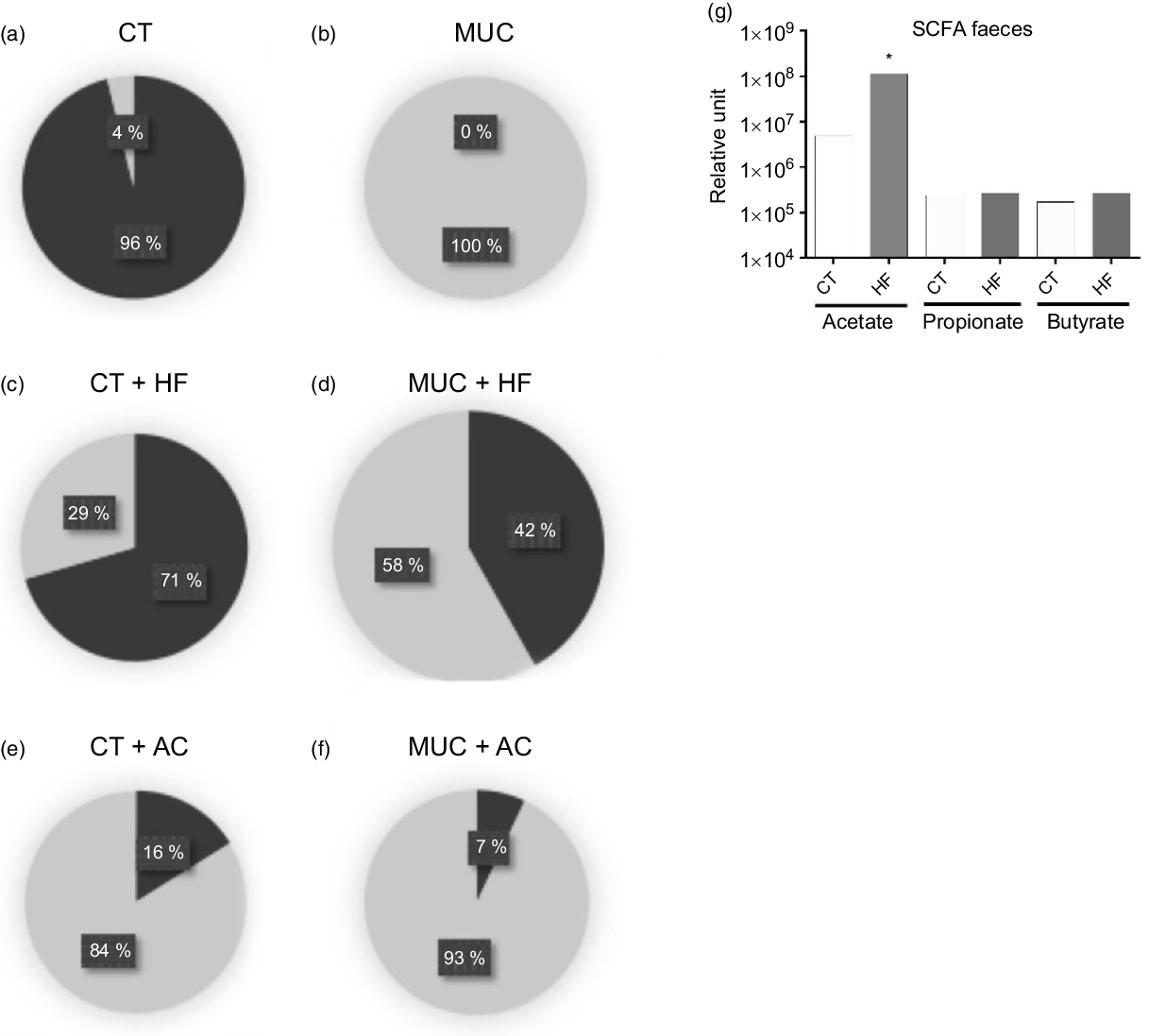
Fig. 3. High-fibre (HF) diet improves microbiota composition and increases production of acetate (AC). Mucositis (MUC) induction was achieved using one daily dose of irinotecan (75 mg/kg per d) for 3 d (groups: MUC, MUC + AC and MUC + HF). Mice were fed with either the control (CT) or the HF diet, or treated with acetate(Reference Touchefeu, Montassier and Nieman8) in drinking water (n 6 per group). Microbiota composition regarding aerobic or anaerobic metabolism in control (a), mucositis (b), HF diet control (c), HF diet mucositis (d), acetate control (e) and acetate mucositis (f) groups. Production of SCFA in faeces from the control and HF groups (g). Bars show means for six mice/group. * P < 0·05 using one-way ANOVA, followed by Newman–Keuls’ post-test. (a–f), , Blood agar anaerobic (colony-forming units total);
, blood agar aerobic (colony-forming units total).
Next, we evaluated the effect of the LF diet on mucositis-induced mice. Mice were fed with an LF or HF diet and submitted to mucositis to evaluate myelosuppression and gut microbiota composition. As expected, we observed that mucositis-induced mice presented a reduced number of total cells in the bone marrow compared with the control group (CT) (Fig. 4(a)). The HF diet (HF MUC) partially reversed myelosuppression induced by irinotecan, whereas the LF diet (LF MUC) had no effect on the number of cells from bone marrow (Fig. 4(a)). As shown in Fig. 3(g), the HF diet increases endogenous acetate production in faeces and is also able to increase acetate in the serum when compared with the CT group (Fig. 4(b)). However, the LF diet decreases the endogenous production of SCFA compared with the control group (Fig. 4(b)). Analysing microbiota composition, we observed that the HF diet reversed dysbiosis by increasing the levels of Lactobacillus and Bifidobacterium, while the LF diet increased dysbiosis by increasing the percentage of Enterobacteriaceae (Fig. 4(c–h) and online Supplementary Fig. 3).

Fig. 4. High-fibre (HF) diet prevents myelosuppression and increases SCFA concentration in serum while low-fibre (LF) diet increases microbial dysbiosis and reduces production of SCFA. Mice received three doses of irinotecan (75 mg/kg per d) to induce mucositis (MUC) (groups: MUC, MUC + acetate (AC) and MUC + HF). Mice were fed with control (CT), HF or LF diets (n 6 per group). Fibre intake increased total cell count in bone marrow and prevented myelosuppression (a). The LF diet decreased SCFA concentration in serum, while the HF diet increased it, mainly acetate (b). Evaluation of microbiota composition in the control (CT) (c), MUC (d), CT + HF (e), MUC + HF (f), CT + LF (g) and MUC + LF (h) groups. Bars show mean values with their standard errors for six mice/group. ** P < 0·01. Statistical analysis was performed using one-way ANOVA, followed by Newman–Keuls’ post-test. (c–h) , MRS anaerobic (Lactobacillus, Bifidobacterium);
, Sabouraud + chloramphenicol (fungi);
, MRS aerobic (Lactobacillus);
, MacConkey (Enterobacteriaceae).
Low-fibre diet exacerbates intestinal permeability and maintains inflammation
During irinotecan-induced mucositis, the LF diet exacerbates intestinal permeability when compared with the MUC group (Fig. 5(a)). As observed in the histological score, the LF diet worsened the tissue damage induced by irinotecan (Fig. 5(b) and (c)). The damage in the intestinal epithelial barrier was followed by increased expression of IL1β in the small intestine tissue of the MUC + LF group, while the HF diet reduced IL1β levels in the MUC + HF group when compared with the MUC group (Fig. 5(e)). This suggests that LF intake maintains the inflammation in the gut during mucositis. No changes were observed in the expression of MUC2 and TNF in the small intestine during mucositis, compared with control group (Fig. 5(c–f)).

Fig. 5. Low-fibre (LF) intake exacerbates intestinal permeability and high-fibre (HF) diet reduces IL1β expression. Mice were challenged with three doses of irinotecan (75 mg/kg per d) for mucositis (MUC) induction. Mice were fed with control (CT), HF or LF diets (n 6 per group). Intestinal permeability was assessed by measuring the radioactivity in the blood after oral administration of 99mTc-diethylenetriamine pentaacetic acid. The fibre gap increased the deleterious effect of irinotecan and exacerbated the intestine (a). Representative images of H&E-stained ileum sections (b) demonstrate that the LF diet did not improve tissue damage, as shown in the histopathological score (c). Quantitative reverse-transcription PCR analysis of TNF (c), muc2 (d) and IL1β (e). Bars show mean values with their standard errors for six mice/group. * Statistically significant differences compared with the MUC group (P < 0·05). Statistically significant differences compared with the control group: † P < 0·05, †† P < 0·01. Statistical analysis was performed using one-way ANOVA, followed by Newman–Keuls’ post-test.
Discussion
Mucositis is a frequent complication in cancer patients due to chemotherapy and radiotherapy which, like most other toxicities, is under-reported by clinicians(Reference Cinausero, Aprile and Ermacora31). It is the major side effect of antineoplastic treatment, leading to therapy interruption, limitations of cancer control and alteration of the chemotherapeutic dose(Reference Odenwald and Turner32). The present study emphasises two important aspects: 1 – the importance of dietary fibre for gut homoeostasis whereby fibre impacts on the intestinal epithelial barrier and the shaping of gut microbiota, and 2 – the absence of a fibre component on diet potentiates the deleterious side effects of anti-cancer therapy.
Mucositis is often associated with weight loss and diarrhoea, as previously reported (Reference Marinho, Custodio and Ferreira33,Reference Calixto-Lima, Martins de Andrade and Gomes34) . Although no effect on body weight was observed, fibre consumption, but not the acetate treatment, reduced the clinical score and improved diarrhoea and the presence of blood in the faeces. In addition, modulation of microbiota might have a beneficial effect in mucositis, since treatment with probiotics attenuates diarrhoea induced by 5-FU(Reference Yeung, Chan and Jiang4).
As previously reported, treatment with irinotecan induces intestinal shortening(Reference Arifa, Paula and Madeira26) associated with diarrhoea and altered gastrointestinal absorption(Reference Nightingale, Woodward and Small35). Here, the HF diet reversed the intestinal shortening, accompanied by an ameliorated histological score observed by lesser destruction of the epithelial architecture and reduced leucocyte infiltration.
Immune cell infiltration during mucositis is associated with the production of pro-inflammatory substances that increase tissue damage in the gastrointestinal mucosa(Reference Pedroso, Vieira and Bastos20). Our study demonstrated that a HF diet and acetate administration in mice reduced the influx of neutrophils and eosinophils, shown by the measurement of MPO and EPO. This effect might be due to the activation of G protein-coupled receptors expressed on the surface of leucocytes by SCFA, leading to pro-resolving effects during inflammation. Activation of GPR43, a type of G protein-coupled receptor, led to the resolution of inflammation in a gout model by inducing the apoptosis of neutrophils when mice were treated with either the HF diet or acetate(Reference Vieira, Galvao and Macia12). In addition, the HF diet and acetate administration reduced eosinophil recruitment into the lung mucosa, suggesting that both approaches protect the mucosa against excessive inflammation(Reference Thorburn, McKenzie and Shen36).
The biosynthesis of mucins is also affected during mucositis(Reference Thorpe37) and this might be a result of a decreased number of goblet cells(Reference Stringer, Gibson and Logan38). It has been shown that probiotics are able to restore mucin production and increase the number of goblet cells(Reference Yeung, Chan and Jiang4). In our study, however, neither the HF diet nor acetate was able to restore MUC1 and MUC2 expression. Another component important for maintaining gut homoeostasis is the epithelial barrier(Reference Okumura and Takeda39). It is well known that intestinal permeability is increased during mucositis and is associated with the disruption of the mucosal barrier, bacteremia and malnutrition(Reference van Vliet, Harmsen and de Bont40,Reference Sonis41) . In our study, the HF diet exerted a protective effect on the epithelial barrier and decreased the intestinal permeability, likely due to microbiota modulation and SCFA production. SCFA may strengthen the epithelial barrier by inducing mucin production, increasing the expression of tight junction proteins and inflammasome activation in intestinal epithelial cells in a G protein-coupled receptor-dependent manner, or by acting as histone deacetylase inhibitors(Reference Macia, Tan and Vieira11,Reference Bilotta and Cong42) . Since there were no significant differences in the expression of Muc1 and Muc2 in the present study, we hypothesise that the decreased intestinal permeability observed in the MUC + HF group might be due to microbiota modulation and SCFA production, which might have led to increased expression of tight junction proteins(Reference Peng, Li and Green43). The microbiota modulation observed in the HF diet led to increased levels of Lactobacillus and Bifidobacterium, two groups known to produce and favour the production of SCFA(Reference LeBlanc, Chain and Martin44,Reference Markowiak-Kopec and Slizewska45) . Our results also aligned with Llewellyn et al.(Reference Llewellyn, Britton and Contijoch46), who observed that the HF diet decreased intestinal permeability in colitis by modulating the microbiota in the intestine.
Interestingly, the absence of fibre in the diet aggravated the deleterious effect of chemotherapy on the epithelial barrier and increased intestinal permeability. One possible explanation for this outcome is the fact that diets poor in fibre are associated with a decrease in the thickness of the mucus layer, leading to the development of dysbiosis characterised by high levels of Enterobacteriaceae. In a fibre-deprived diet, microbiota bacteria start to use mucin glycoproteins as an alternative for the lack of nutrients attributed to the absence of fibre in the diet, reducing the thickness of the mucus layer(Reference Desai, Seekatz and Koropatkin47). Nutritional changes and mucin utilisation by gut microbiota in a diet poor in fibre confer growth advantages to Enterobacteriaceae, a group commonly associated with gut inflammation and epithelial barrier damage(Reference Zeng, Inohara and Nunez48,Reference Sicard, Le Bihan and Vogeleer49) .
In the present study, the HF diet decreased the expression of pro-inflammatory cytokine IL1β, possibly due to the inhibition of histone deacetylase by SCFA. SCFA can modulate gene expression and suppress NF-κB activity(Reference Vinolo, Rodrigues and Nachbar50), leading to reduced expression of pro-inflammatory substances, decreasing tissue damage and improving the epithelial barrier. On the other hand, the absence of fibre in the diet led to increased expression of IL1β, increasing the tissue damage and epithelial permeability. Studies have demonstrated that LF diets are associated with increased inflammatory response and increased intestinal epithelial tight junction permeability(Reference Vieira, Galvao and Macia12,Reference Al-Sadi and Ma51) .
The side effects of antineoplastic treatment, including irinotecan, are associated with the low specificity of chemotherapeutic drugs to cancer cells, which end up also affecting both healthy cells and bone marrow cells, leading to myelosuppression(Reference Arifa, Paula and Madeira26,Reference Rahnama, Madej-Czerwonka and Jastrzebska-Jamrogiewicz52) . In our study, we demonstrated that fibre intake was able to minimise the cytotoxic effect of irinotecan by smoothing out myelosuppression, which possibly is due to SCFA production from pectin fermentation in the gut. SCFA act through G protein-coupled receptor, activating signalling molecules that regulate the production and function of haematopoietic and non-haematopoietic cells(Reference Rooks and Garrett53). A study conducted by Trompette et al.(Reference Trompette, Gollwitzer and Pattaroni54) showed that fermentable fibre intake had a protective effect on lung inflammation by altering the bone marrow haematopoiesis and enhancing monocyte production. These results demonstrate not only the importance of fibre intake and its metabolites on bone marrow but also how they can contribute to the modulation of the systemic and extraintestinal immune response.
SCFA contributes to a range of physiological effects in the host organism. The different effects between the HF diet and oral administration of acetate may be related to gut microbiota modulation, since we did not find higher levels of acetate in mice treated with it. Pectin is a potential prebiotic and may favour the proliferation of SCFA-producing bacteria, which continuously maintain the high levels of acetate in gut. When acetate is administrated orally, this SFCA is rapidly metabolised and used as an energy source or as a substrate for biological processes unable to modulate the gut microbiota and maintain the increased levels of acetate in the faeces and serum. Therefore, the beneficial effect of the HF diet may be, in part, associated with the increased concentration of acetate, which is associated with the significant differences in the microbiota composition observed among the groups that received the HF diet and oral administration of acetate.
Diet is one of the major factors that affect microbiota composition and diversity, in which dietary fibre leads to different physiological effects in the host(Reference Makki, Deehan and Walter55). In our study, mucositis induction and the LF diet resulted in dysbiosis, showing high levels of Enterobacteriaceae, while fibre supplementation decreased the levels of this group and increased the levels of Lactobacillus and Bifidobacterium. Increase in the population of enterobacteria is often associated with the imbalance of the intestinal microbiota and the development of dysbiosis(Reference Zeng, Inohara and Nunez48). SCFA produced by resident colonic bacteria reduces colonic pH and inhibits Enterobacteriaceae, and favours Firmicutes growth, a group with SCFA-producing micro-organisms(Reference Simpson and Campbell56).
HF and LF diets were adapted from the AIN-93M diet, considering the energetic content for each diet, which was approximately the same for each group. The LF diet was high in maltodextrin. This component may play a role in chronic inflammatory disorders, although it is regarded as inert and safe by the US Food and Drug Administration(Reference Arnold and Chassaing57). Interactions between pectin and other dietary components may occur, but studies have demonstrated the beneficial effects of pectin itself(Reference Ishisono, Yabe and Kitaguchi58–Reference Ferrere, Wrzosek and Cailleux60). Although there are clinical trials involving pectin intake in human subjects(Reference Wilms, Jonkers and Savelkoul61–Reference Schwab, Louheranta and Torronen64), it is difficult to transpose the dose used in the present study to humans since the physiological effect of pectin is influenced by the individual’s microbiota composition, which varies from individual to individual.
Our results emphasise the importance of fibre intake during mucositis, and how it helps to modulate the immune system in the host and prevent the inflammatory process in the gut. However, it is important to highlight the limitations in the present study. The culture-dependent method used in the present study is known to underestimate the microbial composition in the sample. However, since we observed significant differences using this method, we speculated that these differences would also be seen if a culture-independent technique was used instead. Also, the low cost of this technique allowed us to replicate the experiment three times, making the results more reliable. As discussed before, the beneficial effect of pectin might be associated with interactions among other components in the diet. However, other studies have elucidated the health benefits of pectin alone in host physiology, which supports our hypothesis that pectin might play the key role in the positive results observed in the present study. Also, more studies are needed to transpose the dose of pectin used in the present study to humans, since the physiological effect of pectin depends mostly on the host microbiota, which varies from person to person. In conclusion, microbiota composition plays a fundamental role in host health and disease. Our results suggest the importance of fibre intake in the composition of the gut microbiota and the maintenance of homoeostasis as an important therapeutic strategy for mucositis and also for understanding the risks that a fibre-deficient diet can bring during chemotherapy.
Acknowledgements
We thank all members of our laboratories for their helpful contribution to the present study, and all authors for their support and expertise.
This work was supported by grants from the Brazilian National Council for Scientific and Technological Development (CNPq), Coordenação de Aperfeiçoamento de Pessoal de Nível Superior (CAPES), the Foundation for Research Support of the State of Minas Gerais (FAPEMIG – APQ-00593-14), (FAPEMIG – APQ-03328-18) and the programme ‘Para mulheres na ciência 2018’ supported by Loreal-UNESCO-ABC, Brasil.
A. T. V., B. G. and I. G. analysed data and wrote the paper. A. T. V., B. G., I. G., G. L., M. F. Q., R. O. S., V. C. M., V. M. R., L. M. T., L. C. L. J., V. M., L. C. A. and M. M. A. performed experiments and analysed data. G. L. performed histological analysis. L. C. A., M. M. A., V. A., V. N. C., F. S. M. and A. T. V. provided expertise and improvements in the issue, and helped with the paper discussion. A. T. V. and F. S. designed and supervised the research.
The authors declare that there are no conflicts of interest.
Supplementary material
To view supplementary material for this article, please visit https://doi.org/10.1017/S0007114520004924