Osteoporosis, with its clinical sequelae of fracture, is a well-recognised health problem among women, but also represents a significant health burden among men. The remaining-lifetime risk for fracture for a man aged 50 years is approximately 22 %( Reference Kanis, Johnell and Oden 1 ), and one-third of hip fractures worldwide occur in men( Reference Seeman 2 ). Men tend to lose bone slowly but continuously from the time peak bone mass is achieved in early adulthood( Reference Looker 3 – Reference Slemenda, Christian and Reed 5 ), with rates of loss increasing with advancing age( Reference Jones, Nguyen and Sambrook 6 ). As a result of the rapid ageing of the population and increased longevity among men, the health burden of osteoporotic fractures among men is increasing( Reference Kanis, Johnell and Oden 1 , Reference Cummings and Melton 7 ). There is thus presently a need for evidence-based recommendations that reduce bone loss and fracture risk in men.
In clinical practice guidelines, increasing Ca intake to the recommended levels is one of the most widely recommended strategies for osteoporosis prevention( Reference Wang, Bolland and Grey 8 ). However, most recommendations regarding the level of Ca intake required for bone health have been developed based on the findings of early Ca-balance studies( 9 ), a method with several deficiencies( Reference Kanis and Passmore 10 , Reference Reid, Bristow and Bolland 11 ). Importantly, Ca balance has never been shown to be associated with fracture risk. The advent of bone densitometry has since allowed the accurate assessment of bone mineral density (BMD), which does predict fracture risk( Reference Stone, Seeley and Lui 12 ). Sequential measurements of bone density over time allow the direct measure of bone balance, obviating the need for the surrogate measure of Ca balance.
The small effects of Ca supplements on bone in clinical trials have also been considered evidence that higher dietary Ca intakes are necessary for bone health( 9 ). There are issues with using such trials to estimate the relationship between long-term dietary intake and bone. For example, Ca supplementation results in only a one-off gain in BMD of approximately 1 %, with no further effect on bone loss beyond 1 year of treatment( Reference Tai, Leung and Grey 13 ). Furthermore, most trials have examined doses of 1000 mg/d of supplemental Ca in addition to mean dietary Ca intakes of 700–800 mg/d, and therefore cannot provide information about the effects of variation in Ca intake within the normal dietary range.
For these reasons, prospective cohort studies with sequential measurements of bone density provide the best tool for estimating the relationship between long-term dietary Ca intake and bone health. Although many studies have examined the relationship between dietary Ca intake and bone loss in women, only a few have done so in men( Reference Hannan, Felson and Dawson-Hughes 14 – Reference Zhou, Langsetmo and Berger 17 ). We previously reported the results of a randomised controlled trial in men( Reference Reid, Ames and Mason 18 ), in which treatment with 1200 mg/d of supplemental Ca for 2 years increased BMD by 1–1·5 % relative to the placebo, and 600 mg/d had no effect. However, for the reasons described above, the effects of short-term, high-dose Ca interventions on BMD are unlikely to represent those of long-term dietary intake. We have therefore used data from this trial of men to examine the relationship between dietary Ca intake and BMD at baseline, and in the placebo group, dietary Ca intake and change in BMD over time. Higher Ca intakes are thought to improve BMD by decreasing the parathyroid hormone (PTH) level and thereby bone turnover, hence we also examined the influence of Ca intake on these parameters.
Methods
Participants
The present cohort (n 323) is from a randomised, controlled trial in healthy men, designed to assess the effects of Ca supplementation on bone density over a period of 2 years. This study has previously been described in detail( Reference Reid, Ames and Mason 18 ). We recruited participants through newspaper advertisement. Participants were aged over 40 years and not receiving therapy for osteoporosis or taking Ca supplements. At recruitment, they were free of major illness (including untreated hypo- or hyperthyroidism, liver disease, malignancy or metabolic bone disease), had serum creatinine <0·2 mmol/l and serum 25-hydroxyvitamin D >25 nmol/l. Lumbar spine and total hip BMD z-scores were >−2 (USA (Combined National Health and Nutrition Examination Survey (NHANES)/Lunar), Femur Reference Population (v112) and AP Spine Reference Population (v112)). None of the participants had been regular users of testosterone, anabolic steroids, glucocorticoids or bisphosphonates in the previous 1 year. None of the participants were taking vitamin D supplements. The study started in 2004 and was completed in 2007. A total of 107 men were randomised to the placebo arm of this trial, of whom 104 completed the study. Three participants from this group received a bone-active medication (Ca, prednisone or bisphosphonates) at some time during the study, and thus were excluded from the longitudinal analyses presented here. Two participants did not complete the baseline dietary Ca assessment and were also excluded, resulting in n 99 for the longitudinal analyses.
Measurements
BMD was measured at baseline and at 24-months at the lumbar spine (L1-4), at both total hips and at the total body using a Prodigy dual-energy X-ray absorptiometer (GE Lunar). The CV for measurement of total hip and lumbar spine BMD in our laboratory are 1·1 and 1·4 %, respectively. The serum 25-hydroxyvitamin D (25(OH)D) level was measured by RIA (DiaSorin) in the first 212 men, and by a chemiluminescent assay (Nichols) in the last 111 men. The interassay CV for the DiaSorin assay was 8·8 % and for the Nichols assay was 7·5 % in our laboratory. Our laboratory meets the performance targets for the Vitamin D External Quality Assessment Scheme( Reference Carter, Carter and Gunter 19 ) for both assays. Results obtained using the Nichols assay were converted to DiaSorin results as we have described previously( Reference Bolland, Grey and Ames 20 ). Total alkaline phosphatase (ALP) activity was assessed using the Roche assay on the Roche Modular autoanalyser (Roche Diagnostics), which is an International Federation of Clinical Chemistry standardised method. The CV of the assay was 2 %. Serum C-telopeptide (CTX) and serum procollagen type-1 N-terminal propeptide (PINP) were measured using an electrochemiluminescence method on the Roche Elecsys 2010 platform (Roche Diagnostics). The CV were 5·1 and 1·9 %, respectively. Intact PTH was measured using an electrochemiluminescence immunoassay on the Roche E170 Modular analyser (Roche Diagnostics). The interassay CV for PTH was 6·1 %. These assays were performed in 150 participants (fifty from the placebo group and fifty from each of the two active treatment groups).
Dietary Ca intake was assessed at baseline and at 2 years using an abbreviated FFQ previously validated for use with Caucasian women( Reference Angus, Sambrook and Pocock 21 ). We have previously shown Ca intake assessed with this instrument to be inversely related to circulating PTH levels in normal postmenopausal women( Reference Bolland, Grey and Ames 22 ). Dietary vitamin D intake was determined using a 24-h food diary analysed with FoodWorks software. Body weight was measured using electronic scales, and height was measured using a Harpenden stadiometer (Holtain). Fracture history, smoking status and physical activity level were assessed by a questionnaire, with the metabolic equivalent of task calculated as described previously( Reference Wilson, Paffenbarger and Morris 23 ). This study was conducted according to the guidelines laid down in the Declaration of Helsinki and all procedures involving human subjects were approved by the local ethics committee. Written informed consent was obtained from all participants. The study was registered with the Australian Clinical Trials Registry, ACTRN 012605000274673.
Statistics
Analyses were completed using SAS (SAS version 9.4; SAS Institute Inc.). In a cross-sectional analysis involving all available baseline data, univariate correlation (Pearson’s normally distributed variables) and ordinary least squares linear regression as well as multiple linear regression were performed with BMD, PTH and bone turnover markers as the dependent variables, and dietary Ca, age, weight, height, serum 25(OH)D, physical activity and smoking status as the independent variables. Variables were chosen for the multivariable analysis on the basis that they were considered likely to be related to the dependent variables and to avoid over-fitting; reduced models were then run including any variable in the fully saturated model with P<0·15. Collinearity diagnostics and goodness-of-fit statistics were examined for model stability. Differences in the means of each dependent variable between tertiles of baseline dietary Ca were tested using ANOVA (general linear modelling) with and without potentially confounding covariates (age, weight, height, serum 25(OH)D, physical activity and smoking status).
A similar analysis was carried out on the change from baseline to 2 years (longitudinal subset, n 99) for each of the dependent variables in the subset of participants randomised to placebo, and who did not commence bone-active medications during follow-up.
All tests were two-tailed. P<0·05 was considered statistically significant and no adjustment for multiplicity was made.
Justification of sample size
This study efficiently uses all data from a randomised placebo controlled trial of Ca supplementation and BMD( Reference Reid, Ames and Mason 18 ). In testing for associations between variables cross-sectionally at baseline in the entire study cohort (n 323), there is adequate power (80 %) at the 5 % significance level to detect correlations of at least 0·16. In the longitudinal analysis (n 99), correlations of at least 0·28 could be detected. These correlations represent small to medium effect sizes and are likely to be of scientific interest. Estimation of minimum detectable effect size for a correlation was made using PASS 2002.
Results
The baseline characteristics of the men are presented in Table 1. The mean Ca intake was 870 (range 110–2630) mg/d. The mean age of men was 57 (range 39–88) years. The cohort was unremarkable with respect to clinical and biochemical variables.
Table 1 Baseline characteristics of participants (Mean values and standard deviations; numbers and percentages)

MET, metabolic equivalent of task; PINP, procollagen type-1 N-terminal propeptide; CTX, C-telopeptide; NHANES, National Health and Nutrition Examination Survey.
* Only men in the placebo group of the trial were included in the longitudinal analysis.
† Bone density t-score refers to the number of sd above or below the young adult mean (USA (Combined NHANES/Lunar) and Femur Reference Population (v112))
‡ Measured in a subset of 150 participants (fifty from the placebo group of the trial and fifty from each of the two Ca-supplemented groups).
Cross-sectional analysis
First, we examined correlations between dietary Ca intake, BMD, PTH and the markers of bone turnover at baseline (n 323 for BMD and n 150 for PTH and the markers of bone turnover). Baseline dietary Ca intake was not correlated with baseline BMD at the total body, the total hip or at the lumbar spine (Fig. 1). To determine whether associations with dietary Ca intake were present once potential confounders had been adjusted for, we performed multiple regression analyses with BMD at each site as the dependent variable and dietary Ca, age, weight, height, serum 25(OH)D, physical activity and smoking status as the independent variables. Dietary Ca did not reach the required level of significance (P>0·15) for inclusion in the models for BMD at any site. To test for non-linear associations, we examined the mean BMD by tertile of dietary Ca intake (<660, 660–920 and >920 mg/d). No differences in BMD were found across the tertiles at the total body (P=0·39), total hip (P=0·57) or at the spine (P=0·35); this did not change after adjusting for weight, height, age, serum 25(OH)D, physical activity and smoking.

Fig. 1 Scatter plots of (a) lumbar spine bone mineral density (BMD) (b) total hip BMD and (c) total body BMD v. dietary calcium intake at baseline in older men. Values are line of best fit and 95 % CI (n 323).
PTH and the markers of bone turnover ALP, CTX and PINP were measured in a subset of 150 participants at baseline (fifty from the placebo group of the original trial and fifty from each of the two Ca-supplemented groups). Dietary Ca intake was inversely correlated with PTH at baseline (Fig. 2; r −0·19, P=0·02). In multiple regression analyses, dietary Ca remained a significant predictor of PTH (P=0·03) in a model that also included age, height, weight and serum 25(OH)D (Table 2). Dietary Ca intake was not correlated with ALP (r −0·09, P=0·26), CTX (r −0·10, P=0·25) or PINP (r 0·003, P=0·96) and was not a significant predictor of these markers in multiple regression analyses. When we compared these parameters across tertiles of dietary Ca intake, no significant differences were found for PTH (P=0·20), ALP (P=0·48), CTX (P=0·58) or PINP (P=0·33), and this did not change after adjusting for covariables.

Fig. 2 Scatter plot of parathyroid hormone (PTH) v. dietary calcium intake at baseline in older men. Line of best fit and 95 % CI are shown (n 150).
Table 2 Predictors of parathyroid hormone (PTH) in menFootnote *
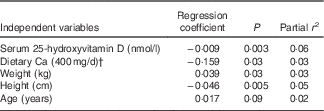
* Model with PTH as the dependent variable, P for model <0·0001, r 2 for model 0·18 (n 150).
† Regression coefficient shows the change in PTH per 400 mg/d increase in dietary Ca intake.
Longitudinal analysis
Over the 2-year study period (placebo group of the original trial only, n 99), total body BMD did not significantly change from baseline (−0·002 g/cm2, P=0·31) but total hip BMD decreased by −0·004 g/cm2 (P=0·02). Lumbar spine BMD increased over 2 years by 0·009 g/cm2 (P=0·01). Dietary Ca intake was not correlated with the change in BMD at any site (Fig. 3). When we performed multiple regression analyses with change in BMD at each site as the dependent variable and dietary Ca, age, weight, height, serum 25(OH)D, physical activity and smoking status as the independent variables, dietary Ca intake did not reach the required level of significance (P<0·15) for inclusion in the models for change in BMD at any site.

Fig. 3 Scatter plots of (a) change in lumbar spine bone mineral density (BMD) (b) change in total hip BMD and (c) change in total body BMD over 2 years v. dietary calcium intake at baseline in healthy older men. Line of best fit and 95 % CI are shown (n 99).
Dietary Ca intake was assessed again at the end of the 2-year study period (placebo group only, n 99) and had increased by an average of 126 mg/d (P=0·003). We repeated the analyses described above using the average of baseline and 2-year dietary Ca intake as the independent variable, with no material change in the findings. We also repeated the analyses using only 2-year dietary Ca intake as the independent variable, and again the findings were similar.
Discussion
We utilised data from a cohort of healthy men enrolled in a randomised controlled trial to examine the relationship between dietary Ca and bone health. Dietary Ca intake was not associated with BMD at baseline (n 323), nor with change in BMD over 2 years (n 99). Ca intake was inversely associated with PTH, but was not related to bone turnover assessed through the markers ALP, CTX and PINP (n 150). A body of research suggests dietary Ca intake does not influence bone loss in older women( Reference Reid, Bristow and Bolland 11 ). The present study extends these findings to men, and suggests that efforts to increase Ca intake are unlikely to have an impact on the prevalence of and morbidity from male osteoporosis.
In men, there is evidence of a slow but continuous loss of bone from the time of early adulthood. Rates of bone loss of −0·1 to −0·5 %/year have been reported in men during middle to older age( Reference Slemenda, Christian and Reed 5 , Reference Bakhireva, Barrett-Connor and Kritz-Silverstein 24 ), with the rate of loss tending to increase among elderly men( Reference Jones, Nguyen and Sambrook 6 , Reference Bakhireva, Barrett-Connor and Kritz-Silverstein 24 ). These rates are broadly consistent with those observed in our study at the total hip (−0·2 %/year) and at the total body (−0·1 %/year). The increase we observed in lumbar spine BMD has been reported before( Reference Looker 3 ). This is likely an artifact of the projected area of the spine declining more rapidly than the mineral content, probably secondary to the decreasing height of intervertebral discs. The average age of men in the present cohort was 57 years, with the range of ages extending from 39 to 88 years. As fracture risk in men increases markedly at about 70 years( Reference Jones, Nguyen and Sambrook 25 ), the cohort of men studied represents one of key interest when considering interventions or lifestyle recommendations aimed at reducing future fracture risk. However, it is possible that because of the relatively low rate of bone loss among this age group, our study may have been underpowered to detect a very small effect of Ca. Whether such a small relationship between Ca intake and bone loss would translate into a clinically important relationship between Ca intake and fracture risk is questionable.
Few previous studies have assessed the relationship between Ca intake and bone density in men. Three studies in older men have also reported no relationship between Ca intake and bone loss( Reference Hannan, Felson and Dawson-Hughes 14 , Reference Dennison, Eastell and Fall 16 , Reference Zhou, Langsetmo and Berger 17 ), although one reported a trend for lower bone loss at the femoral neck with higher Ca intakes( Reference Burger, de Laet and van Daele 15 ). We also found no relationship between Ca intake and bone density in the cross-sectional analysis at baseline. Other cross-sectional studies of men investigating Ca intake and current bone mass have reported no relationship between Ca intake and BMD( Reference Lau, Leung and Kwok 26 , Reference Bischoff-Ferrari, Kiel and Dawson-Hughes 27 ), an association only at very low intakes (<400 mg/d)( Reference Kim, Chon and Noe 28 ) or only very small differences in BMD (<2 %) across the normal dietary range of Ca( Reference Cauley, Fullman and Stone 29 ). An absent or a weak relationship between dietary Ca intake and bone density is supported by a recent meta-analysis of prospective cohort studies, which found no relationship between dietary Ca intake and fracture risk in men or women( Reference Bolland, Leung and Tai 30 ). This is consistent with the finding from the development of fracture risk calculators that Ca intake does not have utility in fracture prediction( Reference Kanis, Oden and Johansson 31 , Reference Nguyen, Frost and Center 32 ).
In the present analysis, dietary Ca intake was inversely related to PTH at baseline (r −0·19, n 323). We have previously reported an inverse correlation with PTH in a group of postmenopausal women using the same Ca questionnaire (r −0·26)( Reference Bolland, Grey and Ames 22 ). Three additional studies in older adults have found inverse correlations between dietary Ca intake and PTH (r −0·08 to −0·27)( Reference Kinyamu, Gallagher and Rafferty 33 – Reference Joo, Dawson-Hughes and Kim 35 ), and a study including a younger population identified an inverse association only among those who were markedly vitamin D deficient( Reference Steingrimsdottir, Gunnarsson and Indridason 36 ). This relationship presumably reflects an appropriate homoeostatic response of the parathyroids to Ca intake. An explanation for the absence of a relationship between dietary Ca and bone loss in observational studies has been that dietary Ca intake was not accurately measured. However, the biologically plausible relationship with PTH identified in the present study and others, indicates the tools used to assess dietary Ca intake are able to detect clinically meaningful differences in Ca intake.
As a low Ca intake is thought to promote bone loss by increasing the rate of bone turnover, we also examined associations between Ca intake and the markers of bone turnover ALP, CTX and PINP at baseline (n 150). No relationship between Ca intake and bone turnover was found. Ca intake was similarly not related to bone turnover in a cross-sectional analysis of men aged 20–87 years( Reference Chaitou, Boutroy and Vilayphiou 37 ). The absence of a relationship between Ca intake and the bone turnover rate is consistent with the notion that Ca intake does not influence bone loss in men.
The inverse correlation between Ca intake and PTH, but lack of a relationship with bone loss and bone turnover, reflects the efficiency of the mechanisms involved in Ca homoeostasis. At low Ca intakes, higher PTH levels increase fractional Ca absorption in the gut (through hydroxylation of vitamin D) and its reabsorption in the renal tubule, whereas at higher Ca intakes both effects are reversed. The ability of humans to maintain Ca homoeostasis across a wide range of intakes, as suggested in the present study, is reflected in an analysis of Ca-balance data( Reference Hunt and Johnson 38 ). In this analysis, neutral Ca balance was achieved at an intake of 741 mg/d, regardless of age or sex, but, importantly, Ca homoeostasis was found to be tightly controlled across the typical dietary range (415–1740 mg/d).
The absence of a relationship between dietary Ca intake and bone loss in observational studies appears to be contradicted by the small beneficial effects observed in clinical trials of Ca interventions. In these trials, Ca supplementation is consistently shown to reduce PTH and bone turnover( Reference Bristow, Gamble and Stewart 39 ) and slow bone loss, resulting in a small gain in BMD (approximately 1 %)( Reference Tai, Leung and Grey 13 ). However, these gains occur during the first year of treatment, with no cumulative benefit to bone density with a longer duration of supplementation( Reference Tai, Leung and Grey 13 ). Thus, the early effects of a large increase in Ca intake on bone loss are probably due to the reduction in bone turnover and the associated filling-in of the remodelling space, and do not persist once a steady-state in bone remodelling is restored. Collectively, evidence from intervention and observational studies suggests long-term Ca intake doesn’t influence the rate of bone loss, but large increases in Ca intake induce a transient change.
The present analysis was based on a cohort of predominantly white, vitamin D-sufficient men, and the null relationship we observed between Ca intake and BMD may not apply to other ethnic groups or to vitamin D-deficient populations. The observational nature of the study means there is the risk of residual confounding. We did not have data on energy intake, which correlates with body size and Ca intake, and therefore we were unable to adjust for this variable. However, if confounding from energy intake was present, we would have expected to see less bone loss at higher Ca intakes as opposed to the null relationship observed. Although our sample size was relatively small, as little research on bone metabolism has been performed in men, the present analysis provides a valuable contribution to this area. In addition to its focus on men, a strength of this study was the inverse relationship we observed between Ca intake and PTH. Although the FFQ we used to assess dietary Ca intake had not been previously validated in men, the inverse correlation between Ca intake and PTH provided an internal validation of this instrument. Furthermore, the relationship between Ca intake and BMD was substantiated through examination of the relationship between Ca intake and bone turnover.
The present demonstration of an absence of an effect of dietary Ca intake on current bone mass or on bone loss in normal men, together with the absence of an effect of Ca intake on bone turnover, contributes to the body of evidence suggesting that Ca intake, within the range studied here, is not a critical factor in the maintenance of bone health in older adults. Many of the messages being promulgated at the present time are based on the findings of Ca-balance studies and the short-term effects of high-dose Ca interventions, which do not reflect those of long-term dietary intake. Messages to increase dietary Ca could be directing at-risk individuals away from considering interventions and strategies proven to influence long-term fracture risk.
Acknowledgements
This study was supported by the Health Research Council of New Zealand. S. M. B. is supported by an Auckland Medical Research Foundation Edith C. Coan Research Fellowship and a Kelliher Charitable Trust Emerging Research Start-Up Award. The funders had no role in the design, analysis or writing of this article.
S. M. B. and I. R. R. formulated the research question and designed the study, A. M. H. carried out the study, G. D. G. analysed the data and S. M. B. and I. R. R. wrote the manuscript, which was critically revised by all authors.
None of the authors has any conflicts of interest to declare.