Vegetarian dietary patterns are becoming increasingly popular for various ecological, ethical or health-related reasons. A high consumption of red and processed meat has been consistently demonstrated to elevate mortality risk from certain cancers( Reference Tantamango-Bartley, Jaceldo-Siegl and Fan 1 ) and CVD( Reference McEvoy, Temple and Woodside 2 , Reference Orlich, Singh and Sabaté 3 ). Yet, it is unclear whether the reduced mortality risk in lacto-ovo vegetarians, found in some studies, is related to the omission of meat and fish, or rather to more prevalent diet-unrelated health-conscious behaviour. A balanced lacto-ovo-vegetarian diet differs in nutrient intake from an omnivorous diet, for example by increased intake of fibre, Mg and antioxidants, but lower intake of n-3 (omega-3) fatty acids and vitamin B12 ( Reference Leitzmann 4 ), all of which is reasonably well documented in the scientific literature. However, the impact of reduced to near absent intake of carnitine, carnosine and creatine in a vegetarian diet is less well established and could be relevant in relation to muscle function, exercise capacity and sports performance.
Carnitine, carnosine and creatine are preferentially or solely found in tissues of animals, with highest concentrations in skeletal muscle tissue (hence the names, derived from latin carnis and greek kreas, flesh). They neither qualify as micronutrients, because they are ingested in relatively high amounts (range of 0·1–5 g/d), nor as macronutrients, because they are not primarily serving an energy provision role. Yet, although not essential, carnitine, carnosine and creatine are nitrogenous molecules that possess properties with relevance to muscle metabolism and function. Phosphorylated creatine plays a crucial bioenergetic role in tissues with high metabolic demand by rephosphorylating ADP to synthesise ATP( Reference Gualano, Rawson and Candow 5 ). Carnitine is known to play a key role within several cellular energy producing pathways such as the transport of long-chain fatty acids into the mitochondria( Reference Steiber, Kerner and Hoppel 6 – Reference Seiler, Koves and Gooding 8 ). It is of highest abundance in meat; however, it is also present in a number of non-meat nutrients (e.g. nuts, potatoes, milk). In contrast, the dipeptide carnosine (β-alanyl-l-histidine) is exclusively present in meat and fish. Carnosine has a wide spectrum of bioactive properties such as pH buffering, Ca2+ regulation, anti-glycation and antioxidant activity( Reference Blancquaert, Everaert and Derave 9 ). Numerous studies in the literature demonstrated the beneficial effects of creatine, carnitine (combined with carbohydrates) or β-alanine (precursor amino-acid of carnosine) supplementation on exercise performance in athletes( Reference Hobson, Saunders and Ball 10 – Reference Twycross-Lewis, Kilduff and Wang 12 ), and therapeutic potential towards certain disorders in which muscle function is a key component( Reference Gualano, Rawson and Candow 5 , Reference Boldyrev, Aldini and Derave 13 , Reference Pinto, Botelho and Pimentel 14 ).
Cross-sectional studies suggested that the homeostasis of carnitine, creatine and carnosine in humans is negatively affected by long-term vegetarianism. Somewhat lower plasma carnitine concentrations in vegetarians than in omnivores are reported( Reference Novakova, Kummer and Bouitbir 15 – Reference Stephens, Marimuthu and Cheng 18 ), but no consistency exists regarding muscle carnitine content. Stephens et al.( Reference Stephens, Marimuthu and Cheng 18 ) reported 17 % lower muscle total carnitine concentrations in vegetarian women, whereas no differences were found in same measure in the study of Novakova et al.( Reference Novakova, Kummer and Bouitbir 15 ). Until now, no longitudinal intervention study in human volunteers has investigated the effect on carnitine homeostasis of transiently switching omnivores onto a vegetarian diet. It is known, however, that carnitine has a slow turnover rate (300–500 µmol/d)( Reference Steiber, Kerner and Hoppel 6 ) and, as it is still somewhat present in a vegetarian diet, it can be suggested that carnitine homeostasis would be maintained during the 1st months of vegetarianism.
Chronic and complete restriction of dietary creatine and carnosine has been demonstrated to result in lower plasma creatine( Reference Delanghe, De Slypere and De Buyzere 16 ) and decreased intramuscular creatine( Reference Burke, Chilibeck and Parise 19 ) and carnosine( Reference Everaert, Mooyaart and Baguet 20 , Reference Harris, Jones and Hill 21 ) concentrations as compared with omnivorous subjects in cross-sectional studies. Thus, these reports suggest that the endogenous synthesis capacity may not be sufficient to maintain muscle creatine and carnosine concentrations, and therefore muscle availability, the principal site of storage, may depend on habitual dietary intake. Creatine is known to have a far higher turnover rate (approximately 2 g/d)( Reference Wyss and Kaddurah-Daouk 22 ) than carnitine( Reference Steiber, Kerner and Hoppel 6 ) and carnosine( Reference Baguet, Reyngoudt and Pottier 23 ). Consequently, a 3-week vegetarian intervention in omnivorous subjects resulted in a 10 % decline in muscle creatine content( Reference Lukaszuk, Robertson and Arch 24 ) and longer-term interventions might thus even deplete creatine to a greater extent. On the contrary, a 5-week vegetarian intervention study indicated no significant reduction in muscle carnosine content( Reference Baguet, Everaert and De Naeyer 25 ). However, in contrast to carnitine, carnosine is completely absent in vegetarian diets, suggesting that carnosine homeostasis might be affected by longer-term interventions.
This study aimed therefore to investigate the effect of a 6-month vegetarian diet on body creatine, carnitine and carnosine stores in omnivorous women. We hypothesised that homeostasis of creatine and carnosine would be disrupted when their dietary intake was missing. For carnitine, however, we hypothesised that homeostasis can be maintained given its slow turnover rate and its presence in some non-meat nutrients. A second aim was to investigate whether supplementation of creatine and β-alanine (the rate-limiting precursor of carnosine synthesis), concurrently with a lacto-ovo-vegetarian diet, was able to correct for potentially emerging deficiencies. Third, we aimed to investigate whether disturbed homeostasis of creatine or carnosine affects outcomes of an incremental cycling test. This type of exercise was chosen because previous studies demonstrated beneficial effects of both creatine or β-alanine supplementation on time to exhaustion (TTE) during an incremental cycling test( Reference Nelson, Day and Glickman-Weiss 26 , Reference Stout, Cramer and Zoeller 27 ).
Methods
Subjects
Forty healthy female omnivores volunteered to participate in this 6-month intervention study. Only women were included in order to reduce the variability in baseline values of muscle carnosine, which is known to be dependent on sex( Reference Baguet, Everaert and Achten 28 ). Exclusion criteria were smoking, chronic use of medication, athletes participating in competitions, vegetarianism or eating meat or fish <5 times a week. One woman dropped out within 2 months for personal reasons. None of the subjects was taking supplements containing creatine, carnosine, β-alanine or carnitine in the 3 months before the start of the study. This study was conducted according to the guidelines laid down in the Declaration of Helsinki and all procedures involving human subjects were approved by the local ethics committee (Ghent University Hospital, Belgium; 2012/666). Written informed consent was obtained from all subjects. Registry name of this study is ‘Creatine, Carnitine and Carnosine in Vegetarians’ and identification no. is NCT03194334 (https://register.clinicaltrials.gov/prs/app/action/SelectProtocol?sid=S00077UP&selectaction=Edit&uid=U0002EMW&ts=2&cx=3y2e4y).
Study design
The study was scheduled over a period of 6 months and measurements were performed 1 week before the intervention (0 months; 0M), after 3 months (3M) and within the last week (6M). In all, ten women continued their omnivorous diet throughout the entire study (controls) and the other twenty-nine subjects switched to a lacto-ovo-vegetarian diet for 6 months. The vegetarian group was split in two groups, matched for age, weight, height and baseline carnosine concentrations in soleus and gastrocnemius medialis muscles on group level. A total of fourteen of them were supplemented with β-alanine and creatine (Veg+Suppl) and the other fifteen women received a placebo (Veg+Pla) (Fig. 1). A co-worker, not involved in the study design and analysis, performed the randomisation and prepared the containers with supplements. With regard to supplementation, the study was a double-blind placebo-controlled study. The lacto-ovo-vegetarian diet consisted of vegetables, fruits, seeds, grains, meat substitutes, eggs and dairy products and the exclusion of meat, poultry and fish. Subjects were asked to complete a 3-d food diary at the start and after 3 months and received nutritional advice by a dietitian during the study to prevent deficiencies in macronutrients and micronutrients. Furthermore, vegetarian recipes were provided by email to support the subjects in their vegetarian diet.

Fig. 1 Participant flow chart.
Supplements
The supplementation protocol included simultaneously daily oral administration of creatine monohydrate (Creapure®; AlzChem AG) and slow-release β-alanine (Carnosyn®; Natural Alternatives International) or a placebo (maltodextrin; Natural Spices). The supplements are considered safe and efficacious( Reference Kreider, Kalman and Antonio 29 , Reference Décombaz, Beaumont and Vuichoud 30 ). The Veg+Suppl group ingested 1 g of creatine monohydrate (two capsules of 500 mg) and 0·8 g of β-alanine (one Carnosyn® tablet) each day for the first 3 months. Intermediate analysis of muscle carnosine content at 3 months revealed that 0·8 g of β-alanine was too high to maintain carnosine at baseline concentration, and therefore the β-alanine dose was reduced to 0·4 g/d during the last 3 months of the study, with creatine dosing remaining at 1 g/d. The Veg+Pla group was supplemented with an identical number of capsules and tablets of maltodextrin. Subjects were instructed to take the supplements together with a meal, as this was shown to improve muscle metabolite loading( Reference Green, Hultman and Macdonald 31 , Reference Stegen, Blancquaert and Everaert 32 ). All subjects were asked not to take any other supplements than those provided by the current study. Compliance was checked by asking the subjects to return the containers and counting the pills that were left. The Veg+Pla group and the Veg+Suppl group ingested 94·4 (sd 5·4) % and 96·4 (sd 4·8) % of creatine/placebo pills, respectively. Compliance ranged between 81·7 and 100 %. For the β-alanine/placebo tablets, the Veg+Pla group and the Veg+Suppl group ingested 96·6 % (sd 3·7) % and 98·1 (sd 2·1) %, respectively, and compliance ranged between 88·3 and 100 %. Because all subjects ingested more than 80 % of the supplements, no subjects were excluded based on compliance. The control group, in which the participants remained on an omnivorous diet, did not receive any supplements.
Incremental cycling test
At 0M, 3M and 6M, subjects performed an incremental cycling test. On the first visit, subjects were screened to be medically fit before starting the incremental cycling protocol to exhaustion. The test was performed on an electrically braked cycling ergometer (Excalibur; Lode). VO2 was measured continuously via a computerised breath-by-breath system (Jaeger Oxycon Pro). Following a 3-min warm-up at 40 W, the workload was increased by 40 W every 3 min until the point the subjects failed to continue to pedal at 60 rpm. Capillary blood samples were taken before and immediately after the incremental cycling test, and pH and lactate were measured (blood gas analysis: GEM, Premier TM 3000; Instrumentation Laboratory).
Sample collection
Blood samples were collected from a forearm vein after an 8-h overnight fast into heparin plasma tubes and serum gel tubes after 0M, 3M and 6M. Heparin tubes were immediately centrifuged (5 min, 16 000 g ) after collection to separate plasma. Serum tubes were left at rest at room temperature to obtain complete coagulation, before performing the same centrifugation process as for plasma. All samples were frozen at −20°C until subsequently analysed. True-cut muscle biopsies were taken at baseline and after 3M from the vastus lateralis of the left leg at rest. Following local anaesthesia of the skin and subcutaneous tissues with 5 ml lidocaine, two muscle samples were taken with a 14-gauge true-cut biopsy needle (Bard Magnum Biopsy gun; Bard, Inc.). The muscle samples were then immediately frozen in liquid N2 and stored at −80°C until subsequent analysis. A far too low number of subjects consented to have muscle biopsies taken at 6M. Therefore, muscle analyses were restricted to the 0M and 3M time points (Fig. 1). Urine samples (24h collection) were collected at baseline and after 3M and 6M into a plastic container and a 5-ml aliquot was stored at −20°C.
Quantification of 25-hydroxyvitamin D
25-Hydroxyvitamin D was measured in serum samples of all participants using the Elecsys Vitamin D total assay (Cobas Instrument). In brief, the assay kit uses a vitamin-D-binding protein (VDBP) as capture protein to bind vitamin D3 and D2. First, the sample (15 µl) is incubated with pretreatment reagent 1 and 2 for 9 min. Thereby, the natural VDBP in the sample is denatured to release the bound vitamin D (25-OH). Second, the sample is further incubated with a recombinant ruthenium-labelled VDBP to form a complex of vitamin D (25-OH) and the ruthenylated-VDBP. Third, with the addition of a biotinylated vitamin D (25-OH), a complex consisting of the ruthenium-labelled VDBP and the biotinylated vitamin D (25-OH) is formed. The entire complex becomes bound to the solid phase (by the interaction of biotin and streptavidin-coated microparticles, which are magnetically captured on the surface of the elecrode). Unbound substances are removed with ProCell/ProCell M. Applying voltage to the electrode induces chemiluminescent emission, which is measured by a photomultiplier. Results are determined via an instrument-specific calibration curve, which is generated by two-point calibration and a calibration master curve provided via the reagent barcode.
Quantification of plasma and urinary compounds
Plasma creatine, creatinine, guanidinoacetate, carnitine and acetylcarnitine were measured by liquid chromatography-double MS (LC-MSMS) with butanol-HCl derivatisation. Creatine, creatinine and guanidinoacetate were quantified as previously described( Reference Cognat, Cheillan and Piraud 33 ). For plasma carnitine and acetylcarnitine, 400 µl of methanol were added to 100 µl of each plasma sample. Vials were mixed for 2 min, incubated for 1 h at 8° and centrifuged for 15 min at 16 000 g . The supernatant (50 µl) was evaporated to dryness under N2 at room temperature. The remaining residues were subsequently derivatised with 50 µl of butanol-HCl. Samples were dried again under N2 at 45°C, and 100 µl of mobile phase (80 % methanol) was added. We injected 5 µl in the LC-MSMS system. Urinary creatinine was measured using the Cobas Instrument (Roche). Plasma β-alanine and urinary biomarkers for meat intake (pi-methylhistidine, tau-methylhistidine and anserine) were measured by HPLC. Plasma and urine of all participants at three time points were deproteinised using 35 % sulfosalicylic acid and centrifuged (16 000 g for 5 min). Two-point 6 µl of deproteinised supernatant was mixed with 77·4 µl of AccQ Fluor Borate buffer and 20 µl of reconstituted Fluor Reagent from the AccQTag chemistry kit (Waters). The same method was applied to the combined standard solutions of β-alanine (Sigma) for plasma, and pi-methylhistidine (Sigma), tau-methylhistidine (Sigma) and anserine (Sigma) for urine. The derivatised samples were applied to a Waters HPLC system with the following parameters: AccQTag column (3·9×150 mm, 5 µm) for β-alanine quantification and Xbridge BEH column (4·6×150 mm, 2·5 µm) for pi-methylhistidine, tau-methylhistidine and anserine quantification, both with fluorescence detector (excitation/emission wavelength: 250/395 nm). The column was equilibrated with buffer A (10 % eluent A (Waters) – 90 % H2O), buffer B (100 % acetonitrile) and buffer C (100 % H2O) at a flow rate of 1 ml/min at room temperature.
Quantification of muscle carnitine and creatine
Wet muscle samples were frozen in liquid N2 and subsequently freeze-dried before being powdered and checked for all visible blood and connective tissue, which were removed under low-grade microscopy. Then muscle metabolites were extracted by adding cold 0·5 m perchloric acid (PCA, containing 1 mm EDTA) to the muscle powder while on ice in a ratio of 1 ml of PCA:every 12·5 mg of muscle powder. Then, samples were gently vortexed for 10 min before being centrifuged (10 000 rpm for 3 min at 4°C). Supernatants were then carefully removed to new test tubes and neutralised with 2·2 m KHCO3. The perchlorate precipitates were removed by centrifugation (10 000 rpm for 3 min at 4°C), and the supernatants (metabolite extracts) were removed to new test tubes and stored at −80°C for subsequent analysis. Muscle PCA extracts were used to determine muscle total creatine content and free and acetylcarnitine content using spectrophotometric and radioactive methods described by Harris et al.( Reference Harris, Hultman and Nordesjö 34 ) and Cederblad et al.( Reference Cederblad, Carlin and Constantin-Teodosiu 35 ), respectively. Muscle total creatine and carnitine contents were expressed as the sum of creatine and phosphocreatine, and the sum of free and acetylated carnitine, respectively.
Proton magnetic resonance spectroscopy
The carnosine levels in soleus and gastrocnemius muscles of all subjects at 0M, 3M and 6M was measured by proton magnetic resonance spectroscopy (1H-MRS), as previously described( Reference Baguet, Bourgois and Vanhee 36 ). The subjects were lying in supine position and the lower leg was fixed in a holder with the angle of the ankle at 20° plantar flexion. All the MRS measurements were performed with a 3 Tesla whole-body MRI scanner (Siemens Trio) equipped with a spherical knee-coil. Single-voxel point-resolved spectroscopy(PRESS) sequence with the following parameters was used: repetition time (TR)=2·000 ms, echo time (TE)=30 ms, number of excitations=128, 1·024 data points, spectral bandwidth of 1·200 Hz and a total acquisition time of 4·24 min. The average voxel size was 40×10×28 mm for soleus and 40×11×29 mm for gastrocnemius and the line width of the water signal was on average 25·2 Hz (soleus) and 28·3 Hz (gastrocnemius), following shimming procedures. The absolute carnosine content (in mm) was calculated as described before by Baguet et al.( Reference Baguet, Bourgois and Vanhee 36 ).
Statistics
A one-way ANOVA was performed to compare the difference in baseline values (age, height, weight, BMI, muscle carnosine concentration and VO2max). A 3×3 repeated-measures ANOVA with baseline values of each parameter as a covariate (ANCOVA) was used to evaluate plasma and urinary metabolite concentrations, muscle carnosine, TTE and VO2max with ‘group’ (Veg+Suppl; Veg+Pla; control) as between-subject factor and ‘time’ (0M; 3M; 6M) as within-subject factor (SPSS statistical software; SPSS Inc.). For the analysis of capillary lactate and pH, measurements before and after the incremental cycling test were included as another within-subject factor (start; end). In case of significance, analyses were repeated for each group separately and pairwise comparisons were used to compare the different time points. A 3×2 repeated-measures ANCOVA (with baseline values of each parameter as a covariate) was used to analyse muscle biopsy metabolite content and dietary macronutrient intake with ‘group’ (Veg+Suppl; Veg+Pla; control) as between-subject factor and ‘time’ (0M; 3M) as within-subject factor. In case of significance, pairwise comparisons were performed to compare 0 to 3 months for each group separately. Correlations between serum 25-hydroxyvitamin D and plasma and muscle carnitine concentrations were obtained by means of Pearson’s correlations. Values are presented as means and standard deviations and statistical significance threshold was set at P≤0·05.
The sample size was calculated in accordance with the primary outcome of the trial: a total sample size of twenty-four subjects is necessary to detect an effect size of 0·25 of a vegetarian diet on muscle creatine, as we know that muscle creatine declines following a 22-d vegetarian diet( Reference Lukaszuk, Robertson and Arch 24 ). The sample size of fifteen subjects in each vegetarian group was thus chosen to warrant for drop-out as the study is a long-term intervention. As the study was less onerous for the control group and investigated variables were hypothesised to be constant following an omnivore diet, only ten subjects were included in this group.
Results
Participants
At the start of the study, the age, weight, height and BMI of the sample as a whole was 25·6 (sd 7·3) years, 62·7 (sd 7·9) kg, 168 (sd 6·3) cm and 22·3 (sd 2·6) kg/m2, respectively. After the participants were assigned to their group, no significant differences emerged for age, weight, height and BMI (Table 1). Furthermore, there was no significant difference in baseline muscle carnosine levels for both soleus and gastrocnemius (Table 1). The groups did not differ in physical fitness determined by VO2max values (control: 40·4 (sd 6·4) ml/min per kg, Veg+Pla: 39·4 (sd 6·4) ml/min per kg, Veg+Suppl: 36·6 (sd 6·3) ml/min per kg; P=0·504).
Table 1 Baseline values of the three experimental groups (Mean values and standard deviations)

Veg+Pla, vegetarian+placebo; Veg+Suppl, vegetarian+supplemental creatine and β-alanine.
Compliance control via urinary biomarkers for meat intake
Both dietary meat intake urinary markers pi-methylhistidine and anserine were significantly lower or revealed a trend to significance at 6M than at 0M in the Veg+Pla and Veg+Suppl groups (pi-methylhistidine: P=0·006 and P=0·004, anserine: P=0·075 and P=0·048, respectively), but not in the control group (pi-methylhistidine: P=0·411; anserine: P=0·526) (Table 2). Although urinary tau-methylhistidine excretion at 6M was significantly lower than at 0M in all experimental groups, the magnitude of this decline was more pronounced in the Veg+Pla and Veg+Suppl groups than in the control group.
Table 2 Urinary concentrations of the meat-intake biomarkers pi-methylhistidine, tau-methylhistidine and anserine for the three experimental groups at baseline (0 months; 0M), 3 months (3M) and 6 months (6M) analysed by a repeated-measures ANOVA (Mean values and standard deviations)

Veg+Pla, vegetarian+placebo; Veg+Suppl, vegetarian+supplemental creatine and β-alanine.
* P<0·05 compared with 0M; ** P<0·001 compared with 0M. † 0·05<P<0·10 compared with 0M.
Creatine moieties
To evaluate the effect of a long-term vegetarian diet in previous omnivores on creatine moieties, plasma creatine (Fig. 2(a)), creatinine (Fig. 2(b)) and guanidinoacetate (Fig. 2(c)), urinary creatinine (Fig. 2(d)) and muscle total creatine (Fig. 3) were measured. For plasma creatine, a significant interaction effect (P<0·001) was found (Fig. 2(a)), demonstrating a decrease of 46 % from baseline in the Veg+Pla group (P=0·008) and an increase of 195 % in the Veg+Suppl group (P=0·008) after 6 months. Plasma creatinine (P=0·102), guanidinoacetate (P=0·414) and urinary creatinine (P=0·344) were not influenced by any intervention. As can be seen in Fig. 3, a significant interaction effect (P=0·017) was found for muscle total creatine. Adhering to a creatine-poor vegetarian diet for 3 months caused a significant decline (14·6 %) in muscle total creatine (153·5 (sd 24·0) to 128·8 (sd 11·6) mmol/kg dry weight (dw); P=0·009), whereas this was slightly increased in the Veg+Suppl (9·7 %) and control (6·8 %) groups (P=0·387 and P=0·068, respectively).
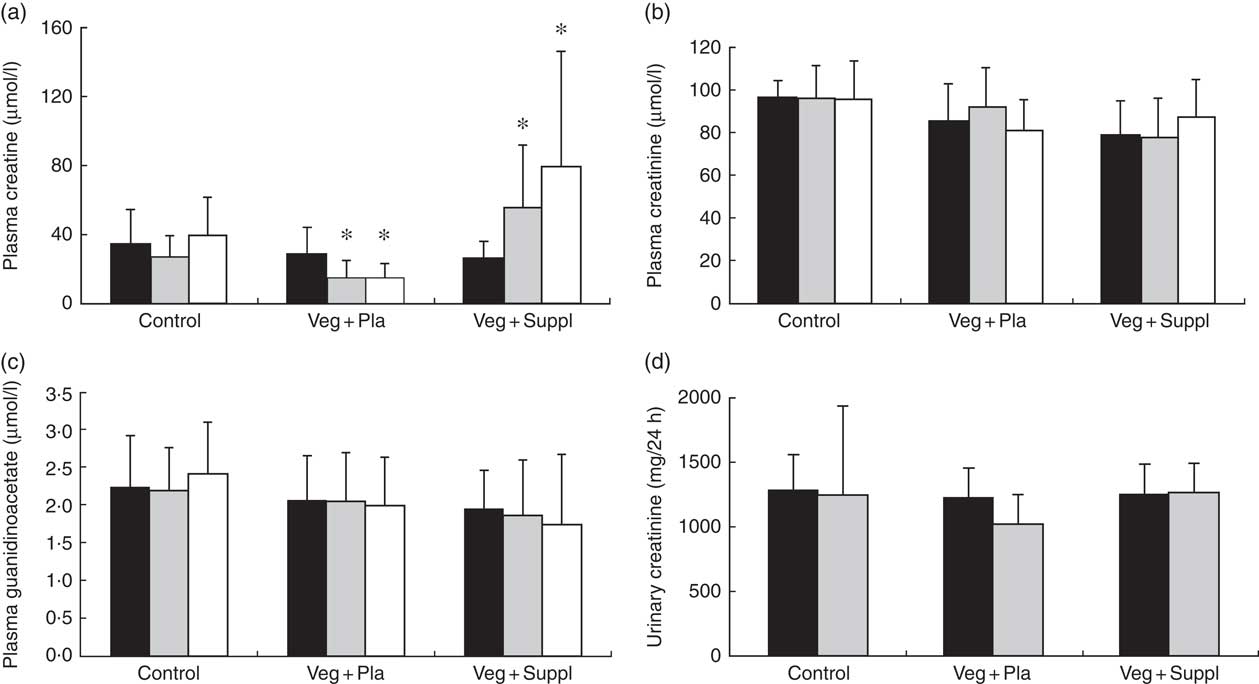
Fig. 2 Effect of an omnivorous diet (control), a vegetarian diet (Veg+Pla) and a vegetarian diet combined with creatine and β-alanine supplements (Veg+Suppl) on (a) plasma creatine, (b) plasma creatinine, (c) plasma guanidinoacetate and (d) urinary creatinine concentrations, analysed by a repeated-measures ANCOVA. Values are means and standard deviations represented by vertical bars. , Baseline (0 months);
, 3 months;
, 6 months. * P<0·05 compared with baseline.

Fig. 3 Effect of an omnivorous diet (control), a vegetarian diet (Veg+Pla) and a vegetarian diet combined with creatine and β-alanine supplements (Veg+Suppl) on muscle total creatine content analysed by a repeated-measures ANCOVA. Values are means and standard deviations represented by vertical bars. , Baseline (0 months; 0M);
, 3 months; dw, dry weight. * P<0·05 compared with 0M.
Carnitine moieties
Plasma and muscle free carnitine, acetylcarnitine and total carnitine (free+acetylcarnitine forms) concentrations were measured to investigate the effect of induced vegetarianism on the carnitine moieties in previous omnivore subjects. No significant interaction effect was found for any of these parameters. However, as shown in Fig. 4(a)–(c) and 5(a)–(c), significant main effect of time for plasma carnitine (P=0·003), acetylcarnitine (P=0·002) and total carnitine (P=0·001), and muscle carnitine (P=0·049) and total carnitine (P=0·001) were found. As most of the carnitine parameters seem to decline independently from intervention, but rather as a result of a seasonal pattern, especially towards the 3M time point that coincided with the end of the winter, we decided, therefore, to evaluate whether carnitine seasonal pattern could be related to the vitamin D status.

Fig. 4 Effect of an omnivorous diet (control), a vegetarian diet (Veg+Pla) and a vegetarian diet combined with creatine and β-alanine supplements (Veg+Suppl) on (a) plasma carnitine, (b) plasma acetylcarnitine and (c) plasma total carnitine concentrations, analysed by a repeated-measures ANCOVA. For all these parameters, a significant main effect of time was found (P=0·003; P=0·002; P=0·001, respectively). Values are means and standard deviations represented by vertical bars. , Baseline;
, 3 months;
, 6 months.

Fig. 5 Effect of an omnivorous diet (control), a vegetarian diet (Veg+Pla) and a vegetarian diet combined with creatine and β-alanine supplements (Veg+Suppl) on (a) muscle carnitine, (b) muscle acetylcarnitine (c) and muscle total carnitine content, analysed by a repeated-measures ANCOVA. For muscle carnitine and muscle total carnitine, a significant main effect of time was found (P=0·049 and P=0·001, respectively). Values are means and standard deviations represented by vertical bars. , Baseline;
, 3 months; dw, dry weight.
Serum 25-hydroxyvitamin D
Similar to most of the carnitine forms, a significant main effect of time (P<0·001) for serum 25-hydroxyvitamin D concentration was found, exemplified by a significant decrease from 0 to 3M and a return to baseline at 6M (Fig. 6(a)). To check whether the 25-hydroxyvitamin D levels were the underlying mechanism for the observed pattern of carnitine levels in plasma and muscle, correlations between these parameters were explored. Surprisingly, a significant negative rather than positive correlation between serum 25-hydroxyvitamin D levels and plasma total carnitine was observed at all time points (r −0·34, P<0·001) (Fig. 6(b)). No significant correlation between 25-hydroxyvitamin D levels and total muscle carnitine was observed (r −0·104, P=0·422). After clustering the subjects in vitamin-D-deficient (<25 ng/ml at 0M or 3M) and non-deficient (>25 ng/ml) groups, significant or trend to significant higher total plasma carnitine levels in the deficient than in the non-deficient group were found at each time point (0M, P=0·013; 3M, P=0·064; 6M, P=0·002). 25-Hydroxyvitamin D-deficient subjects also displayed a significant lower decrease in vitamin D levels from 0M to 3M compared with non-deficient subjects (−4·53 v. 8·50 ng/ml, P=0·014).
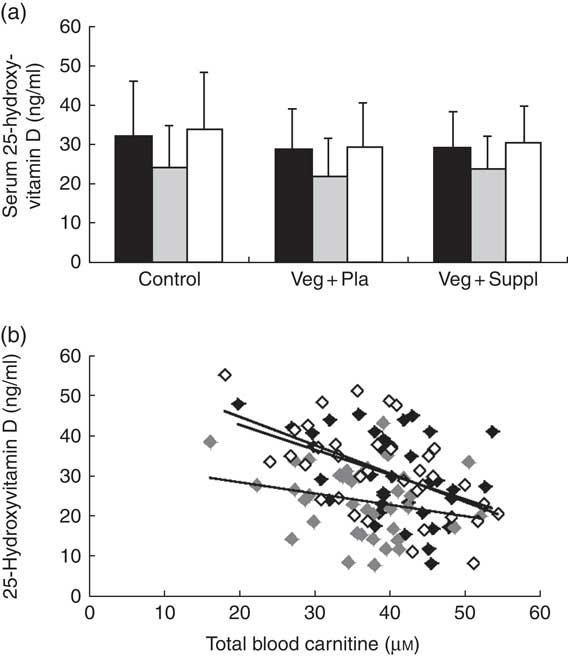
Fig. 6 Effect of an omnivorous diet (control), a vegetarian diet (Veg+Pla) and a vegetarian diet combined with creatine and β-alanine supplements (Veg+Suppl) on (a) serum 25-hydroxyvitamin D concentrations analysed by a repeated-measures ANOVA and (b) correlations between 25-hydroxyvitamin D and total plasma carnitine content at baseline (0 months; 0M) (r −0·432, P=0·008), 3 months (3M) (r −0·24, P=0·153) and 6 months (6M) (r −0·561, P≤0·001), analysed by Pearson’s correlation. Values are means and standard deviations represented by vertical bars. a: , 0M;
, 3M;
, 6M; b:
, 0M;
, 3M;
, 6M.
Carnosine availability
Fasting plasma β-alanine concentrations remained stable throughout the 6-month intervention period in the Veg+Pla and control group, but not in the Veg+Suppl group (Fig. 7(a)). The latter group showed a significant increase in plasma β-alanine concentrations after 3 months of vegetarian diet combined with 0·8 g of β-alanine/d (+27·2 %; P=0·027), with no further increase in the following 3 months when the daily dose of β-alanine was lowered to 0·4 g/d (P=0·740 v. 3 months). A significant (P=0·001) interaction effect (time×group) was found for soleus muscle carnosine content (Fig. 7(b)). In accordance with plasma β-alanine, soleus carnosine did not change over time in the Veg+Pla group (P=0·619), nor in the control group (P=0·790). When a 6-month vegetarian diet was combined with β-alanine supplementation (Veg+Suppl group), soleus carnosine content increased by 26 % (P<0·001). This increase was already established after 3 months (+28 %; P<0·001), with no further increase in the subsequent 3 months. For gastrocnemius muscle, similar results were found (Fig. 7(c)): a significant increase in the Veg+Suppl group of 28 and 41 % after 3 (P=0·009) and 6 (P=0·001) months, respectively, whereas carnosine remained stable in the Veg+Pla (P=0·275) and control group (P=0·293).
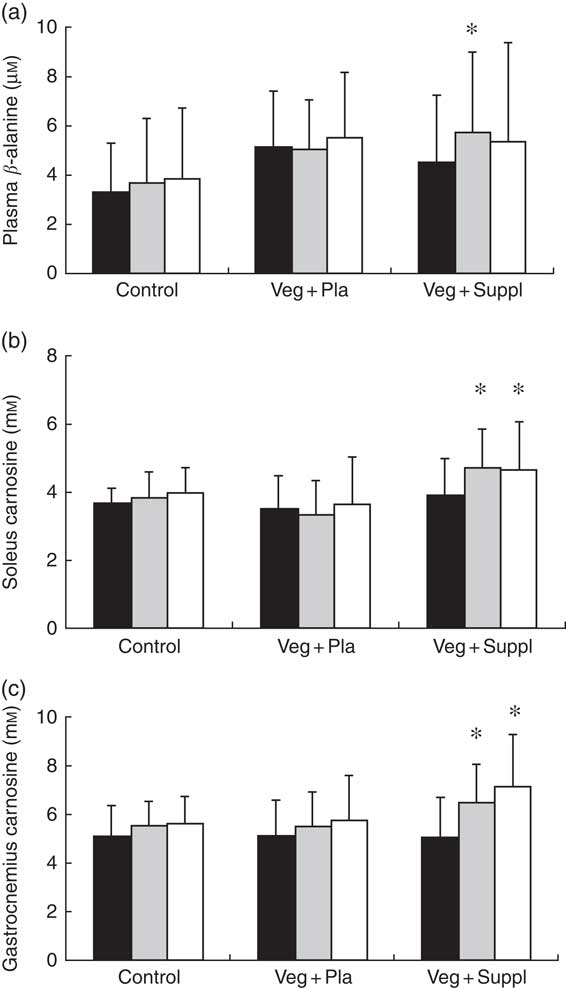
Fig. 7 Effect of an omnivorous diet (control), a vegetarian diet (Veg+Pla) and a vegetarian diet combined with creatine and β-alanine supplements (Veg+Suppl) on (a) plasma β-alanine, (b) soleus carnosine and (c) gastrocnemius carnosine concentrations, analysed by a repeated-measures ANCOVA. Values are means and standard deviations represented by vertical bars. , Baseline (0 months; 0M);
, 3 months;
, 6 months. * P<0·05 compared with 0M.
Incremental cycling test
VO2max and TTE did not differ between the experimental groups at baseline, nor did it change during the 6-month intervention period (Table 3). Blood lactate increased to a range of 11–14 mmol/l by the end of cycling. Blood pH at rest was approximately 7·41–7·42 and was not affected by the subsequent intervention (vegetarian/supplementation). The incremental cycling test elicited a marked acidosis (pH 7·23–7·29) in all intervention groups (Table 4). These parameters (VO2max, TTE, lactate and pH) showed neither a significant intervention nor time or interaction effect.
Table 3 VO2max and time to exhaustion (TTE) in an incremental cycling exercise test for the three experimental groups at baseline (0 months; 0M), 3 months (3M) and 6 months (6M) analysed by a repeated-measures ANOVA (Mean values and standard deviations)

Veg+Pla, vegetarian+placebo; Veg+Suppl, vegetarian+supplemental creatine and β-alanine.
Table 4 Capillary lactate and pH at baseline (0 months; 0M), 3 months (3M) and 6 months (6M) at rest and at the end of the incremental cycling test (Mean values and standard deviations)

Veg+Pla, vegetarian+placebo; Veg+Suppl, vegetarian+supplemental creatine and β-alanine.
Dietary registration
There was no significant difference between the vegetarian and control subjects concerning the daily intake of energy content, proteins, carbohydrates and fat through the regular diet both at the start (0M) and after 3 months (3M) (P>0·05 for all variables) (Table 5). Although the vegetarian group seemed to have slightly higher total energy consumption, there was no group difference in energy consumption from each macronutrient (protein: 14·7 v. 14·5 %; carbohydrates: 46·4 v. 44·6 %; fat: 35·6 v. 35·7 % for control and vegetarian subjects, respectively).
Table 5 Daily intake of energy content, proteins, carbohydrates and fat through the regular diet both at the start (0 months; 0M) and after 3 months (3M) (Mean values and standard deviations)

Veg, vegetarian subjects.
Discussion
The present study demonstrates that whole-body creatine homeostasis was disturbed by a 3-month vegetarian diet in omnivorous women, whereas carnosine and carnitine homeostasis was not affected. Further quantification of muscle carnosine content demonstrated that muscle carnosine homeostasis is also not affected after 6 months of vegetarianism. Unfortunately, no muscle creatine and carnitine data were available at this time point; however, plasma measurements suggest that carnitine homeostasis was also maintained at 6 months, whereas creatine homeostasis continued not to be. Total creatine is, alongside glycogen, the most abundant metabolite in human skeletal muscle with concentrations of about 120–150 mmol/kg dw. As previously mentioned, it is known to have a high turnover rate in comparison with carnitine and carnosine, with 1·7 % of the total body creatine pool turned over per day. Consequently, in the women participating in this study, with a body pool of approximately 110 g of total creatine, approximately 1·6 g/d has to be replaced by dietary creatine intake and de novo biosynthesis( Reference Wyss and Kaddurah-Daouk 22 ). It has been demonstrated that in a diet containing meat and fish the average creatine intake is approximately 1·0 g/d( Reference Balsom, Söderlund and Ekblom 37 ) and 1–2 g/d of creatine is endogenously synthesised from arginine, glycine and methionine in liver, kidney and pancreas( Reference Wyss and Kaddurah-Daouk 22 ). Thus, in the case of a creatine-poor diet, approximately twice as much creatine needs to be endogenously synthesised to maintain the body creatine pool, which the current data demonstrate is not possible when omnivores switch to a vegetarian diet. When muscle creatine stores decline in response to a vegetarian diet, one would also expect urinary creatinine clearance to decline by a similar magnitude, as there is no enzymatic control for the hydrolysis of creatine and total muscle mass is assumed to remain stable. Muscle total creatine content declined by 14·6 % (interaction effect P=0·017) in the Veg+Pla group at 3 months. Although the relative change in urinary creatinine was of the same magnitude (−14·2 %), this did not reach significance (interaction effect P=0·344), which is most likely related to the higher inter-individual variability of the latter variable.
Carnitine and carnosine have a lower muscular concentration (20–30 mmol/kg dw) and a much slower turnover rate than creatine. Healthy subjects excrete carnitine at a rate of approximately 5 µmol/kg/d, which, for the women participating in this study, amounts to ~315 µmol/d (corresponding to approximately 0·05 g/d and 0·02 % of total body carnitine pool)( Reference Steiber, Kerner and Hoppel 6 ). For muscle carnosine, washout time after a period of carnosine loading by β-alanine supplementation was shown to be a slow process that takes 6–20 weeks( Reference Baguet, Reyngoudt and Pottier 23 ), demonstrating the slow degradation rate of carnosine. Baguet et al.( Reference Baguet, Reyngoudt and Pottier 23 ) calculated that, in absolute terms, the elimination of carnosine is about 0·5 g/d, which corresponds to approximately 0·15 % of total body carnosine pool and is thus 10-fold slower than that for creatine (1·7 %).
To our knowledge, this is the first long-term intervention study demonstrating that body creatine stores decline after initiation of a vegetarian diet. Our results are in accordance with cross-sectional data on plasma and urinary creatine and creatinine values in vegetarian subjects( Reference Delanghe, De Slypere and De Buyzere 16 ). Muscle total creatine decreased within 3 months by 14 % in our subjects, which is in accordance with the cross-sectional decrease (−10 %) in muscle total creatine found in the study of Burke et al.( Reference Burke, Chilibeck and Parise 19 ) and the longitudinal decrease (−10 %) after a 3-week intervention period in omnivorous subjects( Reference Lukaszuk, Robertson and Arch 24 ). However, this decrease did not affect VO2max nor TTE of the incremental cycling test. Although the exercise modes were much different in nature, this is in accordance with previous studies that did not find a difference between vegetarian and non-vegetarian subjects for exercise performance measures, such as 3 20-s bouts of maximal cycling( Reference Shomrat, Weinstein and Katz 38 ) and 1-repetition maximum bench press and leg press( Reference Burke, Chilibeck and Parise 19 ). However, reduced muscle total creatine levels might affect other short-term high-intensity performances such as sprinting, in which phosphocreatine plays a key role.
Interestingly, low-dose creatine supplementation combined with a vegetarian diet was able to maintain muscle total creatine content at baseline levels after 3 months and even triple plasma creatine concentrations at 6 months, thereby making creatine a valid supplement for vegetarian athletes participating in high-intensity exercises. A daily dose of 1 g of creatine monohydrate seems to be a sufficient dose to prevent deficiencies, which is considerably lower than the 3–20 g/d required to elevate muscle creatine stores above the baseline set point( Reference Hultman, Söderlund and Timmons 39 ). As the daily ingestion of 0·88 g creatine (1 g creatine monohydrate) is adequate to avoid a decline in body creatine stores on the background of a creatine-free vegetarian diet, the remainder of the daily creatine requirement is probably met by endogenous synthesis in kidney and liver.
Carnitine homeostasis of either plasma or muscle was not influenced by initiation of a vegetarian diet. Dietary carnitine intake is mainly attributable to the intake of meat and fish (animal source), although it is not completely absent in dairy products and some vegetables, fruit and cereals( Reference Krajčovičová-Kudláčková, Šimončič and Béderová 40 ). Thus, carnitine is also present in moderate amounts in a vegetarian diet and has a slow turnover rate relative to that of creatine, which is in line with the absence of an effect of 6 months vegetarianism on plasma carnitine concentrations, as we hypothesised. As all experimental groups display the same pattern in the current study, namely lower carnitine and acetylcarnitine concentrations at 3M, which seemed to be restored at 6M, this could have reflected seasonal variations rather than effects of the intervention. The study was initiated in mid-December and the intermediate measurements were performed in March; thus, the first half of the intervention period was during winter, whereas the second part, with the final measurements in the second half of June, was during spring. Therefore, we hypothesised that vitamin D status may be an underlying mechanism for the observed seasonal fluctuations in carnitine homeostasis. This hypothesis was based on the findings of Dursun( Reference Dursun 41 ) who found decreased plasma carnitine levels and increased carnitinuria in severe vitamin D deficiency patients (rickets), and rodent studies( Reference Park, Kim and Kang 42 ) have documented that low vitamin D status impairs lipid oxidation capacity, including carnitine palmitoyltransferase-1 expression. Our results indicate that, similar to carnitine, seasonal fluctuations are found in serum vitamin D concentration on group level. Yet, when looking at individual level, a significant negative correlation between serum vitamin D and plasma total carnitine content was found, which would indicate that vitamin D cannot explain the seasonal fluctuations in carnitine homeostasis in the present study. The discrepancy with previous literature( Reference Dursun 41 , Reference Park, Kim and Kang 42 ) probably relates to the severity of vitamin D deficiency in the latter studies, as compared with the physiological range in the present study.
Published cross-sectional data showed lower plasma carnitine concentrations in vegetarians than in omnivores( Reference Novakova, Kummer and Bouitbir 15 – Reference Stephens, Marimuthu and Cheng 18 ), which is in contrast to our present data. However, this is probably explained by difference in the duration of vegetarianism (1·5–20 years v. 6 months the present study). It is hypothesised that renal conservation mechanisms and thus higher carnitine reabsorption may occur in vegetarians to compensate for the lower carnitine concentrations obtained from the diet, thereby keeping plasma carnitine concentrations within a relatively normal range( Reference Lombard, Olson and Nelson 17 , Reference Rebouche, Lombard and Chenard 43 ). Furthermore, some inconsistency exists also regarding muscle carnitine content, as Novakova et al.( Reference Novakova, Kummer and Bouitbir 15 ) did not find a lower muscle content in vegetarians compared with omnivores, whereas Stephens et al.( Reference Stephens, Marimuthu and Cheng 18 ) reported a 17 % difference. However, it should be noted that the study of Novakova et al.( Reference Novakova, Kummer and Bouitbir 15 ) included only male vegetarians who consumed a vegetarian diet for at least 1·5 years, whereas the study of Stephens et al.( Reference Stephens, Marimuthu and Cheng 18 ) included both male and female subjects who are vegetarians for 11 years on average. It can be concluded that seasonal variation may have a greater impact on body carnitine homeostasis than a change to vegetarian dietary habits in the short-term; however, long-lasting vegetarianism (>1·5 years) is likely to have a bigger impact.
Given that β-alanine is the rate-limiting precursor for carnosine synthesis in human muscle cells and vegetarian diets are free of β-alanine and carnosine (including its methylated variants anserine and balenine), it is somewhat surprising that muscle carnosine content was unaltered after a 6-month vegetarian diet in previously omnivorous subjects. This suggests that maintenance of carnosine homeostasis does not depend on the nutritional supply of β-alanine. Thus, endogenous β-alanine synthesis can probably entirely compensate for the absence of dietary β-alanine and thereby maintain body homeostasis, at least during the 1st months of vegetarianism. Current findings on muscle carnosine content are somewhat in contrast with cross-sectional data on long-term vegetarians (>7years) reported in the study of Everaert et al.( Reference Everaert, Mooyaart and Baguet 20 ) and preliminary report of Harris et al.( Reference Harris, Jones and Hill 21 ). However, it should be noted that in the study of Everaert et al.( Reference Everaert, Mooyaart and Baguet 20 ), although the lower muscle carnosine content in vegetarians (n 12) v. omnivores (n 38) reached significance in the gastrocnemius, this was not the case for soleus and tibialis anterior muscles. Furthermore, the report of Harris et al.( Reference Harris, Jones and Hill 21 ) included only six vegetarians and was not sex- and age-matched (which is important for carnosine( Reference Baguet, Everaert and Achten 28 )).
Interestingly, low dose of β-alanine supplementation (0·8 g/d) for 3 months in vegetarians significantly increased plasma β-alanine and muscle carnosine content by 20–30 %. Knowing that the average daily intake of β-alanine from an omnivore Western diet has been calculated to amount to approximately 330 mg/d( Reference Everaert, Mooyaart and Baguet 20 ), the daily intake of an additional approximately 500 mg/d, which corresponds to a total ingested dose of approximately 46 g in 3 months, is responsible for this increase. Because of the increase in carnosine above baseline concentration with this initial dose (0·8 g/d), β-alanine dose was reduced to 0·4 g/d during the last 3 months of the study. This dose did not further enhance plasma β-alanine and muscle carnosine concentrations, neither did it restore these concentrations to baseline, suggesting that this dose was still higher than the normal dietary β-alanine intake of these subjects. However, in contrast to previous research( Reference Stout, Cramer and Zoeller 27 ), no effect of β-alanine supplementation was found on TTE of the incremental cycling test. This is most likely owing to the different dosing strategy of β-alanine (up to 6·4 g/d( Reference Stout, Cramer and Zoeller 27 ) v. 0·8 and 0·4 g/d in current study) and consequently different effects on muscle carnosine.
Some urinary biomarkers of meat intake are known, such as pi-methylhistidine, tau-methylhistidine and anserine( Reference Cross, Major and Sinha 44 – Reference Altorf-van der Kuil, Brink and Boetje 46 ). A clear reduction in urinary excretion of these compounds, for pi-methylhistidine to an order of magnitude lower than the original value, was observed in the vegetarian subjects, proving that they complied with the vegetarian intervention. A limitation of the current study is that data of the muscle biopsies at 6M are missing, making it impossible to draw conclusions on the effect of a 6-month vegetarian diet on intramuscular creatine and carnitine concentrations.
It can be concluded that changing to a vegetarian diet reduces the body creatine pool in omnivorous women after 3 months, which can be avoided by dietary creatine supplementation. This points to creatine being a suitable supplement for vegetarian athletes, especially those participating in resistance training and short-term, high-intensity exercise performance. Carnitine homeostasis was not disrupted by switching to a 3-month vegetarian diet, and carnosine homeostasis was unaffected by a 6-month vegetarian diet. This suggests that for carnitine and carnosine, which have a comparatively lower turnover rate than creatine, endogenous synthesis can maintain homeostasis for at least 3 to 6 months, respectively.
Acknowledgements
This study was financially supported by grants from the Research Foundation – Flanders (FWO G.0243.11 and G.0352.13N).
The authors’ responsibilities were as follows: L. B., A. B., T. B., I. E., S. D. H. and W. D. designed the research and formulated the research question; L. B., A. B., T. B., I. E., A. V., M. P., C. V., S. D. H. and W. D. conducted and supervised the study intervention; L. B., A. B., T. B., A. V., I. E., J. D., D. C.-T., P. G. and W. D. performed data analysis and statistical analysis; L. B., A. B., P. G. and W. D. wrote the paper; and W. D. had primary responsibility for final content. He is the guarantor of this work, and, as such, had full access to all the data in the study and takes responsibility for the integrity of the data and the accuracy of the data analysis. All authors provided intellectual input for the manuscript and read and approved the final manuscript.
Creatine supplements were kindly provided by Dr Ulrike Braun from AlzChem (Trostberg, Germany) and β-alanine supplements were provided by Dr Roger Harris and Dr Zimin Liu from Natural Alternatives International (NAI, San Marcos, USA). The authors declare that there are no conflicts of interest.