Recently, Lee and colleagues(Reference Lee, Sowa and Hinoi1, Reference Ferron, Hinoi and Karsenty2) proposed the hypothesis that the skeleton may act as an endocrine organ to regulate energy metabolism. A key concept of this hypothesis is that this regulation of energy metabolism is mediated by the bone-specific protein osteocalcin (OC), which was invoked as a new hormone facilitating β-cell proliferation, insulin secretion and peripheral sensitivity to insulin. Evidence was presented that the increased insulin sensitivity was due to the stimulatory effect of OC on the expression of the adiponectin gene in adipocytes. OC is a major bone protein synthesised by osteoblasts and contains three residues of the unusual amino acid γ-carboxy glutamic acid (Gla) which are formed during a post-translational vitamin K-dependent modification step(Reference Berkner and Runge3). Vitamin K is a group name for a number of closely related compounds of which phylloquinone (K1) and the K2 vitamins (menaquinones (MK)) are the most important. MK are classified according to the length of their aliphatic side-chain (MK-n), where n represents the number of isoprenyl residues in that chain. Where phylloquinone is synthesised by green plants, the MK are produced by bacteria and dietary MK are mainly found in fermented food products. The synthetic form of one of the MK, MK-4, is used in Japan as an ethical drug in the treatment of osteoporosis(Reference Iwamoto, Takeda and Sato4). MK-7, derived from the Japanese food natto (fermented soyabeans), was shown to have a longer half-life time (3 d) than phylloquinone and MK-4 (1–2 h)(Reference Schurgers, Teunissen and Hamulyak5).
The vitamin K content in most human diets is insufficient to support the full γ-carboxylation of OC(Reference Booth, Martini and Peterson6). Hence, both carboxylated (cOC) and uncarboxylated (ucOC) species are found in the circulation(Reference Vermeer, Shearer and Zittermann7). Many nutritional studies have established that circulating ucOC is a useful biochemical marker of osteoblastic vitamin K status which quickly responds to changes in vitamin K intake(Reference Binkley, Krueger and Kawahara8, Reference Iwamoto, Sato and Takeda9). To date, seventeen Gla-containing proteins have been described and in those whose function is known, the Gla-residues are essential for their activity and functionality.
The most surprising aspect of the report of Lee et al. (Reference Lee, Sowa and Hinoi1) is their hypothesis that the putative hormonal functions are mediated by the ucOC rather than the cOC. This was concluded by Ferron et al. (Reference Ferron, Hinoi and Karsenty2) after mice treated with ucOC in combination with a high-fat diet gained less weight than untreated mice on a high-fat diet. A logical consequence of this hypothesis is that low vitamin K intake might promote fat metabolism and weight loss, whereas high vitamin K intake might lead to an increase of body mass and accumulation of adipose tissue. Whereas the data supporting the hormonal activity for ucOC were mainly obtained in cell cultures and transgenic animal model systems, it is unclear whether the results can be extrapolated to humans. On the contrary, a limited number of studies suggest that an increased vitamin K intake has a potentially beneficial role in glucose homeostasis in rats and in young men(Reference Sakamoto, Kimura and Hiraike10–Reference Sakamoto, Nishiike and Iguchi12). More recently, several studies were performed among healthy older men and women demonstrating that high intakes of phylloquinone or a long-term treatment with phylloquinone had no adverse effects on glucose metabolism(Reference Yoshida, Booth and Meigs13–Reference Kumar, Binkley and Vella15). It was also demonstrated that increased adiposity is associated with poor vitamin K status in the elderly(Reference Shea, Booth and Gundberg16).
The purpose of the present investigation was to test the hypothesis that increased carboxylation of OC by vitamin K supplementation is accompanied by an increased weight in humans. First, we tested the potential association between OC carboxylation, vitamin K status, circulating adiponectin concentrations and body composition in a cohort of healthy postmenopausal women. Additionally, we investigated whether circulating adiponectin, body weight and BMI are affected by vitamin K supplements using archived samples and data from two previously completed intervention studies at our institute.
Subjects and methods
Study design and subjects
Study I
Baseline measurements of body composition, serum OC and adiponectin were made in a cohort of 244 healthy postmenopausal women, who were pre-screened for their eligibility to participate in a 3-year MK-7 intervention study, which started in April 2008 and is still ongoing. Participants were recruited from the general population through advertisements in local newspapers. All subjects were in good health, at least 2 years postmenopausal, between 55 and 65 years of age at intake, and of the Caucasian race. Trial registration code: clinicaltrials.gov NCT00642551.
Study II
Measurements of circulating OC and adiponectin were made in a MK-7 dose–response study. This double-blind, randomised, placebo-controlled study was performed between May and September 2007 to establish the minimal effective dose of MK-7 for the aforementioned clinical trial (study I). Overall, twenty healthy men and twenty-two healthy premenopausal women (age range: 25–45 years) were randomised into seven groups of six subjects each. Each group received a daily supplement containing either 0, 10, 20, 45, 90, 180 or 360 μg MK-7 for 12 weeks. Blood sampling occurred at subsequent time points within the 12-week period (day 0, 1, 3, 7, 14, 28, 42, 56, 70 and 84). For the present paper, the data on circulating OC and adiponectin at start and after 12 weeks of supplementation were used. BMI was only determined at baseline, because of the short intervention period of 12 weeks. Trial registration code: clinicaltrials.gov NCT00483431.
Study III
A detailed description of this study design has been published elsewhere(Reference Knapen, Schurgers and Vermeer17). In brief, 325 healthy postmenopausal women (age range: 55–75 years) were recruited between October 1999 and August 2000 for a double-blind, randomised, placebo-controlled trial to investigate the influence of MK-4 (45 mg/d) on bone loss and bone geometry. BMI and circulating markers were measured at baseline and after 3 years of treatment in the 257 women who completed the study (MK-4 group: n 133; placebo group: n 124). Circulating adiponectin was assessed in samples from those women of whom blood samples were still available: eighty-nine women in the MK-4 group and seventy-five in the placebo group. The existing data of these 164 women were used and included body weight, BMI, circulating ucOC, cOC and the ratio between ucOC and cOC (UCR) at baseline and after 3 years of treatment.
The design of the three studies as well as the treatment regimens and measurements performed are summarised in Table 1. Exclusion criteria in all three studies were: BMI >30 kg/m2, a medical history or use of drugs known to interfere with vitamin K-, Ca- and/or glucose-metabolism. The present studies were conducted according to the guidelines laid down in the Declaration of Helsinki, and all procedures involving human subjects were approved by the Ethics Committee of Maastricht University Medical Centre, The Netherlands. A written informed consent was obtained from all participants before entering the trials.
Table 1 Study designs, subject characteristics, treatments and measurements performed
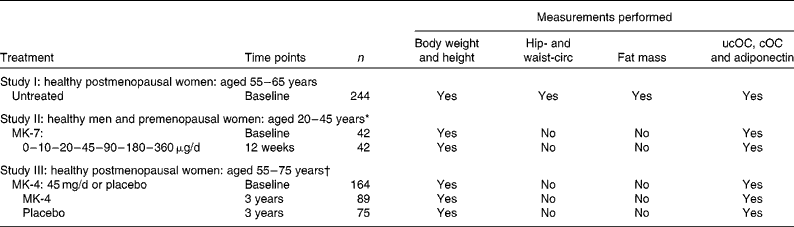
circ, Circumference; ucOC, uncarboxylated osteocalcin; cOC, carboxylated osteocalcin; MK-7, menaquinone-7; MK-4, menaquinone-4.
* Overall, twenty men and twenty-two premenopausal women were randomised to seven dosage groups of six persons each.
† Postmenopausal women (n 325) entered the study and were randomised to MK-4 (n 161) and placebo (n 164)(Reference Knapen, Schurgers and Vermeer17). Analysis of circulating adiponectin was performed in 164 blood samples, which were still available (MK-4: n 89; placebo: n 75).
Biochemical measurements
Blood was taken by venepuncture after an overnight fast in studies I and II. In study III, the women were allowed to eat a small, low-fat breakfast in the morning of sampling. All blood collections occurred between 08.00 and 11.00 hours. Serum was prepared by centrifugation at 3000 g for 10 min, at room temperature and stored at − 80°C until analysis. Commercially available ELISA test kits were used to determine serum ucOC and cOC (Takara Shuzo Company Limited). The inter- and intra-assay CV for ucOC were 7·7 and 4·8 % and for cOC 10·6 and 2·3 %, respectively. UCR, a sensitive marker for bone vitamin K status, was calculated as the ratio between circulating ucOC and cOC. Elevated values of UCR indicate a low vitamin K status. Total serum adiponectin was measured by an ELISA obtained from BioSource Europe SA for which the inter- and intra-assay CV were 8·2 and 4·4 %, respectively.
Body composition variables
Body height was measured to the nearest 0·5 cm using a wall-mounted stadiometer. Body weight was measured to the nearest 0·1 kg, with participants wearing light clothing and no shoes. BMI was calculated as body weight (kg) divided by the square of height (m2). Waist:hip ratio was calculated as the waist circumference (cm) divided by the hip circumference (cm), with a precision of 0·5 cm. Whole-body fat mass, fat mass of the trunk (FMT) and whole-body lean mass, all expressed in kg, were measured by dual X-ray absorptiometry (Discovery A; Hologic), using the whole-body absorptiometry software package (APEX version 2.3.1; Hologic).
Statistical analyses
Normality was tested with the one-sample Kolmogorov–Smirnov test. Adiponectin and UCR values from studies I and III were log-transformed.
Analyses at baseline
Baseline characteristics between treatment groups in study II were tested with a one-way ANOVA, using Tamhane's T2 post hoc test for multiple comparisons. Independent t tests were performed to compare the differences between men and women in study II as well as the differences between the placebo and MK-4 groups in study III. Pearson correlations were used to test for significant associations between variables.
Analyses after vitamin K treatment
In study II, multivariate linear regression was used to determine the effect of MK-7 treatment on circulating adiponectin, ucOC, cOC and UCR. Dummy variables were created for the different treatment groups. Analyses were adjusted for age, sex, BMI and baseline values of each dependent variable.
In study III, the effect of MK-4 treatment on adiponectin, ucOC, cOC, UCR, body weight and BMI within and between the placebo and MK-4 groups was investigated by using the paired t test and independent t test, respectively. Multivariate linear regression analysis was performed to investigate the effect of MK-4 treatment on the changes in body weight (model 1) and BMI (model 2) adjusted for age and current smoking. Model 1 was also adjusted for baseline body weight and height and model 2 for baseline BMI values. Multivariate linear regression analysis was also performed to investigate whether the changes in body weight and BMI are related to the changes in adiponectin, ucOC, cOC or UCR in both treatment groups adjusted for age, current smoking, baseline values of body weight and height or BMI, and baseline values of the independent variable (adiponectin, ucOC, cOC or UCR).
All analyses were performed using SPSS for Windows (version 17.0; SPSS Inc.) and were considered to be statistically significant at P < 0·05 (two-sided).
Results
Baseline characteristics of study populations I, II and III
Baseline characteristics are summarised in Table 2. In study population II, serum adiponectin concentrations were significantly higher in women than in men, whereas cOC concentrations were significantly higher in men than in women. As expected, height and weight were significantly higher in men. BMI values were comparable between both sexes.
Table 2 Baseline characteristics of study populations I, II and III
(Mean values and standard deviations or percentages)
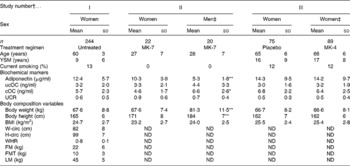
MK-7, menaquinone-7; MK-4, menaquinone-4; YSM, years since menopause; ucOC, uncarboxylated osteocalcin; cOC, carboxylated osteocalcin; UCR, ucOC:cOC ratio; W-circ, waist-circumference; ND, not determined; H-circ, hip-circumference; WHR, waist:hip ratio; FM, total fat mass; FMT, fat mass of the trunk; LM, total lean mass.
Mean values were significantly different: * P < 0·05, ** P < 0·005.
† Study I: a cross-sectional analysis in postmenopausal women; study II: a 12-week intervention trial in premenopausal women and men treated with various amounts of MK-7 and study III: a 3-year intervention trial among postmenopausal women treated with either placebo or 45 mg MK-4(Reference Knapen, Schurgers and Vermeer17).
‡ Independent t tests between men and women in study II and between the placebo and MK-4 group in study III.
Associations of adiponectin, uncarboxylated osteocalcin, carboxylated osteocalcin and uncarboxylated osteocalcin:carboxylated osteocalcin ratio with body composition variables (study population I)
Serum adiponectin was inversely associated with body weight, BMI and fat mass. However, these associations were weak. Stronger inverse associations were found between adiponectin and indices for fat distribution of the trunk (waist circumference, waist:hip ratio, FMT). Similar but still stronger inverse associations were found between the body composition variables and cOC. No associations were found between ucOC and any of the body composition variables. As might be expected from the inverse correlation of body composition variables with cOC, the marker of bone vitamin K status UCR was positively associated with the same body composition variables. The associations of UCR with body weight and BMI were less pronounced. Adiponectin was also inversely associated with lean mass, whereas ucOC, cOC and UCR were not (Table 3).
Table 3 Pearson correlations (r) between circulating adiponectin, uncarboxylated osteocalcin (ucOC), carboxylated osteocalcin (cOC), ucOC:cOC ratio (UCR) and body composition variables at baseline in healthy postmenopausal women aged 55–65 years (study I)
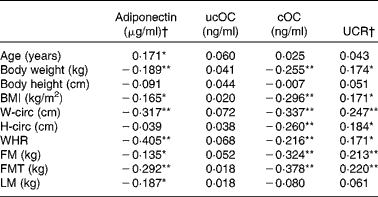
W-circ, waist circumference; H-circ, hip circumference; WHR, waist:hip ratio; FM, total fat mass; FMT, fat mass of the trunk; LM, total lean mass.
Correlation values were significantly differnt: * P < 0·05, ** P < 0·005.
† Variables were log-transformed if not normally distributed (adiponectin and UCR).
Associations of adiponectin with uncarboxylated osteocalcin, carboxylated osteocalcin and uncarboxylated osteocalcin:carboxylated osteocalcin ratio (study populations I, II and III)
No associations were found between adiponectin and ucOC, cOC or UCR in studies I, II and III. However, in study population II (the only study that included both men and women), a positive association was found between adiponectin and cOC in women (r 0·508; P = 0·022), whereas no such association was found in men (r 0·070; P = 0·776).
Effect of vitamin K supplementation on circulating adiponectin, uncarboxylated osteocalcin, carboxylated osteocalcin and uncarboxylated osteocalcin: carboxylated osteocalcin ratio (study populations II and III)
In study population II, baseline values of adiponectin, ucOC, cOC and UCR of the seven treatment groups did not differ from each other (Fig. 1). After 12 weeks of MK-7 supplementation, circulating adiponectin had not changed in any of the treatment groups (Fig. 1(a)). Daily intakes of 90 μg MK-7 or higher resulted in a significant decrease in ucOC (Fig. 1(b)). The increase in cOC (Fig. 1(c)) and the decrease in UCR (Fig. 1(d)) were significant in the treatment groups taking 180 and 360 μg MK-7/d.
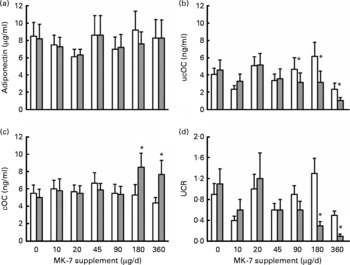
Fig. 1 (a) Circulating adiponectin, (b) uncarboxylated osteocalcin (ucOC), (c) carboxylated osteocalcin (cOC) and (d) ucOC:cOC ratio (UCR) before (□) and after 12 weeks () of treatment with seven different daily doses of menaquinone-7 (MK-7) in healthy men and premenopausal women aged 25–45 years. Values are means (n 6 for each dosage group), with standard errors represented by vertical bars. No significant differences (P>0·05) were found between the treatment groups at baseline (one-way ANOVA post hoc Tamhane's T2). * Multivariate linear regression analysis to determine the effect of extra MK-7 intake on circulating adiponectin, ucOC, cOC and UCR, respectively (P < 0·05). Dummy variables were created for the different MK-7 regimens. Each analysis was adjusted for age, sex, BMI and baseline values of the dependent variable.
In study population III, 3-year MK-4 supplementation had also no effect on circulating adiponectin. The mean decreases in both the placebo- and MK-4 groups were comparable and between-group analysis showed that these changes were not significantly different. Consistent with the data reported previously(Reference Knapen, Schurgers and Vermeer17), the changes in serum ucOC and cOC in the placebo group were significantly different from those in the MK-4 group. Obviously, this was also the case for the changes in UCR in both treatment arms (Table 4).
Table 4 Changes in adiponectin, uncarboxylated osteocalcin (ucOC), carboxylated osteocalcin (cOC), ucOC:cOC ratio (UCR), body weight and BMI after 3 years of treatment with either placebo or 45 mg menaquinone-4 (MK-4)/d in healthy postmenopausal women aged 55–75 years (study III)
(Mean values and standard deviations)
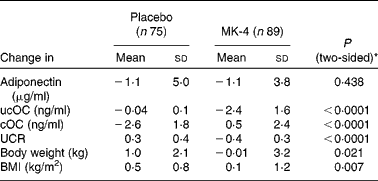
* Independent t test between changes in the placebo and MK-4 groups. Variables were log-transformed if not normally distributed (adiponectin and UCR).
Effect of vitamin K supplementation on body weight and BMI (study population III)
As reported previously(Reference Knapen, Schurgers and Vermeer17), among the 325 participants included in this study, seven had complaints about weight gain (2 %): four women in the placebo group and three women in the MK-4 group. Two women in the placebo group withdrew from the study for this reason; the other five women completed the study regularly.
In the cohort of 164 women (study III), 3-year supplementation with placebo or high-dose MK-4 resulted in a significant increase of body weight and BMI in the placebo group (from 66·7 (sd 8·2) to 67·6 (sd 8·7) kg (P < 0·0001) and from 25·5 (sd 2·4) to 26·0 (sd 2·5) kg/m2 (P < 0·0001), respectively). In the MK-4 group, body weight and BMI were not altered after 3 years of treatment (from 66·6 (sd 8·1) to 66·6 (sd 8·9) kg; P = 0·974 and from 25·4 (sd 2·8) to 25·5 (sd 3·0) kg/m2; P = 0·463). Between-group analysis revealed that the changes in body weight and BMI in the two treatment groups differed significantly (Table 4). The difference between the MK-4 and placebo groups remained significant after multivariate linear regression analysis: P = 0·038 (body weight) and P = 0·013 (BMI) (Table 5).
Table 5 Associations between 3 years of menaquinone-4 (MK-4) treatment and changes in body weight and BMI in healthy postmenopausal women aged 55–75 years (study III)
(Unstandardised β-coefficients with their standard errors and partial correlation coefficients (r part))
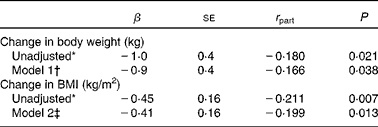
* Unadjusted model: change in body weight or in BMI as dependent variable and MK-4 treatment as the independent variable.
† Model 1: adjusted for age, current smoking, baseline values of body weight, height, (log)adiponectin, uncarboxylated osteocalcin and carboxylated osteocalcin.
‡ Model 2: as in Model 1, but instead of baseline body weight and height, baseline BMI.
Since these data are only based on a part of the initial study cohort, we have also analysed the total group of 257 women who had completed the trial, and similar changes in weight and BMI were observed. In the placebo group, the body weight change was +0·8 (sd 2·7) kg after 3 years and in the MK-4 group, the change was − 0·1 (sd 3·5) kg (between-group analysis: P = 0·025). BMI increased in the placebo group with 0·5 (sd 1·1) kg/m2 and in the MK-4 group with 0·1 (sd 1·3) kg/m2 (between-group analysis: P = 0·011).
In the placebo as well as in the MK-4 group, the changes in body weight were significantly and inversely associated with the changes in adiponectin (unadjusted: partial correlation coefficients (r part) − 0·292, P = 0·011 and r part − 0·288, P = 0·006, respectively). Comparable associations were found for the changes in BMI and adiponectin (unadjusted: placebo: r part − 0·262, P = 0·023 and MK-4: r part − 0·274, P = 0·009). After controlling for potential confounders (age, baseline values of adiponectin and body weight or BMI, current smoking), the significance of these associations was lost (body weight: placebo r part − 0·200, P = 0·097 and MK-4 r part − 0·197, P = 0·073; BMI: placebo r part − 0·186, P = 0·121 and MK-4 r part − 0·187, P = 0·086). However, no such associations were found for ucOC, cOC or UCR in both treatment arms.
Discussion
In the present paper, we demonstrate that in postmenopausal women (study I) there were no associations between ucOC and any of the body composition variables, body weight, BMI, waist-circumference, waist:hip ratio, fat mass and FMT, whereas circulating adiponectin and cOC were inversely and independently associated with these variables. As a result, high values of UCR (suggestive of poor vitamin K status) were associated with high body weight, BMI and fat mass. We also demonstrate that in all three studies adiponectin was not associated with the degree of OC carboxylation as decided from circulating ucOC, cOC and UCR.
Supplementation with vitamin K (MK-7 or MK-4) did not affect circulating adiponectin concentrations (studies II and III), nor did it lead to an increase in body weight or BMI (study III). On the contrary, we observed that body weight and BMI remained unchanged in the high-dose MK-4 group, and increased significantly in the placebo group after adjustment for potential confounders, such as age and current smoking. Our finding that a high dose of 45 mg/d of MK-4 prevented the increase of BMI as observed in the placebo group is consistent with data from Yoshida et al. (Reference Yoshida, Jacques and Meigs14) who found that 3 years of phylloquinone supplementation had a protective effect on insulin resistance in men; our data are also consistent with an experimental animal study showing a significantly decreased fat mass at high vitamin K intake(Reference Sogabe, Maruyama and Baba18).
If, as suggested by the hypothesis made by Lee et al. (Reference Lee, Sowa and Hinoi1), ucOC is a hormonal regulator of energy metabolism, there would need to be mechanisms controlling its generation and release into the circulation. They postulate that this regulation is carried out by the protein osteotesticular-protein tyrosine phosphatase. However, besides the data presented in this paper, there is a wealth of data in humans that the degree of γ-carboxylation of OC is not under tight regulation and is readily and rapidly influenced by dietary variations in vitamin K intakes(Reference Schurgers, Teunissen and Hamulyak5, Reference Binkley, Krueger and Kawahara8, Reference Iwamoto, Sato and Takeda9, Reference Sokoll, Booth and Davidson19, Reference Bugel, Sorensen and Hels20), or by pharmaceutical interactions (e.g. oral vitamin K antagonists)(Reference Knapen, Hellemons-Boode and Langenberg-Ledeboer21–Reference Yasui, Uemura and Umino23). In particular, even relatively modest increases in dietary intakes of vitamin K can dramatically reduce ucOC(Reference Bolton-Smith, McMurdo and Paterson24) and pharmacological doses of vitamin K decrease this uncarboxylated fraction even further(Reference Knapen, Schurgers and Vermeer17, Reference Binkley, Krueger and Engelke25). The fact that the proportions of ucOC to cOC can be readily overridden by the diet or vitamin K antagonists suggests that the γ-carboxylation status of OC is largely uncontrolled rather than being tightly regulated as expected for a hormonal function.
Extrapolating the results obtained in cell culture and GM mouse models by Lee and others(Reference Ferron, Hinoi and Karsenty2, Reference Ferron, Wei and Yoshizawa26–Reference Rached, Kode and Silva28) to humans implies that shifting the balance between ucOC and cOC in favour of ucOC (i.e. vitamin K-insufficiency) would result in accelerated fat metabolism and loss of adipose tissue, whereas shifting the balance towards cOC (i.e. high vitamin K intake and vitamin K sufficiency) would be associated with high body fat, high BMI and obesity. Our results in healthy human volunteers provide convincing evidence that such an extrapolation is not valid presumably because of the increased level of complexity of cross-talk between bone and adipose tissue in humans.
The strong inverse association we found between adiponectin and fat mass, especially with abdominal fat mass (waist circumference, FMT and FMT%) has been reported by others(Reference Ziemke and Mantzoros29). Here, we demonstrate that cOC and not ucOC was inversely associated with the fat mass indices, suggesting that the vitamin K status was lower (e.g. high UCR) in subjects with higher weight, waist circumference and fat mass. Subjects with a high degree of OC carboxylation were leaner and had less body fat than those with lower OC carboxylation. This is consistent with data from Hwang et al. (Reference Hwang, Jeong and Ahn30), who demonstrated that body weight and BMI in healthy middle-aged men were significantly lower in the highest tertile of cOC, whereas body weight and BMI were similar among the tertiles of ucOC. Recently, Shea et al. (Reference Shea, Booth and Gundberg16) showed that high concentrations of vitamin K are present in human adipose tissue, and that women with the highest percentage of body fat had the lowest plasma vitamin K levels and a poorer vitamin K status.
In the literature(Reference Fernandez-Real, Izquierdo and Ortega31–Reference Kanazawa, Yamaguchi and Yamauchi35), data on an association between circulating adiponectin and OC species are conflicting. In the large majority of the participants of our studies, no such association was found. Only in premenopausal women serum adiponectin was associated with cOC; this was a small group, however, and should be confirmed in more elaborate studies.
Gla-residues are essential for the binding of Gla-proteins to extracellular surfaces (phospholipids or hydroxyapatite). Since such binding does not seem to be required for the postulated hormone function of OC, it seems at least plausible that domains outside the Gla-domain are important for its regulatory function in fat metabolism. This would imply that both cOC and ucOC species possess the putative hormone function. It should be kept in mind, however, that in the presence of Ca ions cOC adopts a tertiary structure that is completely different from that of ucOC, which may have implications for the molecular structure outside the Gla-domain and for the relative hormonal activity of ucOC and cOC. Our data suggest that cOC is the most active form in this respect. Another possible explanation for the effect of vitamin K on body weight maintenance is that it acts directly on cellular functions, which are independent of γ-carboxylation. Takeuchi et al. (Reference Takeuchi, Suzawa and Fukumoto36) presented evidence that MK-4, but not phylloquinone, inhibited adipogenesis and stimulated osteoblastic differentiation in vitro. This is in line with a body of evidence that MK-4 has direct effects on a variety of cellular processes and pathways by activating the steroid and xenobiotic receptor on the nuclear membrane(Reference Shearer and Newman37).
Limitations of our findings are that in the MK-4 trial (study III) blood sampling was performed in the non-fasting state, so that apart from adiponectin no other biomarkers for energy metabolism (glucose, insulin, homeostasis model assessment of insulin resistance index) could be reliably measured. Moreover, the analyses were performed retrospectively on archived samples from previous studies in which the hypothesis investigating the association between vitamin K status and fat mass/body weight/BMI was formulated post hoc, and not as the primary hypothesis. Another limitation is that our findings are only based on healthy, non-obese, non-diabetic subjects. Whether these results can be extrapolated to obese and/or diabetic subjects remains to be seen.
In conclusion, consistent data were obtained in three independent studies among healthy, non-obese volunteers, suggesting that a high vitamin K intake or status does not lead to increased body weight, BMI or fat mass in humans. The fact that high vitamin K intake was associated with improved weight maintenance in postmenopausal women (study III) needs to be confirmed in specifically designed vitamin K supplementation trials.
Acknowledgements
The authors declare that they have no conflicts of interest. The financial support by NattoPharma (Oslo, Norway) and Eisai (Tokyo, Japan) is gratefully acknowledged. These companies took no part in any aspect of the studies (such as the design, recruitment and interpretation of the results). The authors' contributions are as follows: L. J. S., M. J. S., P. N. and C. V. contributed to the overall scientific plan; C. V. and M. H. J. K. designed the studies; M. H. J. K. collected the data and contributed to the laboratory analyses; M. H. J. K. and E. T. completed the statistical analyses and interpretation; M. H. J. K. wrote the draft manuscript; L. J. S., M. J. S., P. N., E. T. and C. V. critically reviewed the final manuscript.