Biotin, a water-soluble vitamin, is a member of the vitamin B-complex family. Biotin functions as a cofactor for four carboxylases, namely, propionyl CoA carboxylase, pyruvate carboxylase, β-methylcrotonyl CoA carboxylase and acetyl CoA carboxylase. These carboxylases are involved in intermediary metabolic processes such as gluconeogenesis, energy production, and the synthesis and metabolism of fatty acids and amino acids; they catalyse carbon dioxide transfer. Individuals lacking biotinidase activity, which is essential for biotin recycling, exhibit conditions such as dermatitis, alopecia and neurological abnormalities that can be prevented by biotin administration.
Recent studies have revealed the pharmacological effects of biotin on glucose homeostasis. In rats, biotin deficiency has been associated with hyperglycaemia and impaired glucose tolerance(Reference Dakshinamurti, Modi and Mistry1, Reference Deodhar and Mistry2). Additionally, it has been demonstrated that biotin administration improves glucose and insulin tolerance in genetically diabetic mice(Reference Reddi, DeAngelis, Frank, Lasker and Baker3) and rats(Reference Zhang, Osada, Maebashi, Ito, Komai and Furukawa4). Administration of a pharmacological dose of biotin increases insulin secretion from perifused rat pancreas(Reference Sone, Ito, Sugiyama, Ohneda, Maebashi and Furukawa5) and a culture of isolated islets(Reference Romero-Navarro, Cabrera-Valladares, German, Matschinsky, Velazquez, Wang and Fernandez-Mejia6, Reference Sone, Ito, Shimizu, Sasaki, Komai and Furukawa7). In addition, biotin influences the expression of several genes, including those encoding glucokinase(Reference Romero-Navarro, Cabrera-Valladares, German, Matschinsky, Velazquez, Wang and Fernandez-Mejia6, Reference Chauhan and Dakshinamurti8) and phosphoenolpyruvate carboxykinase(Reference Dakshinamurti and Li9), that play a critical role in glucose homeostasis(Reference Chauhan and Dakshinamurti8–Reference De La Vega and Stockert12). Serum biotin concentration was reported to be significantly lower in type 2 diabetic patients than in control subjects(Reference Maebashi, Makino, Furukawa, Ohinata, Kimura and Sato13). Besides us, another group has shown that administration of a higher dose of biotin reduces the fasting glucose and TAG levels in type 1 and type 2 diabetic patients(Reference Maebashi, Makino, Furukawa, Ohinata, Kimura and Sato13, Reference Coggeshall, Heggers, Robson and Baker14); however, one study reported that biotin did not reduce the fasting glucose levels in type 2 diabetic patients(Reference Baez-Saldana, Zendejas-Ruiz, Revilla-Monsalve, Islas-Andrade, Cardenas, Rojas-Ochoa, Vilches and Fernandez-Mejia15).
Insulin resistance is associated with numerous metabolic diseases, including hypertension. A genetically hypertensive rat strain, namely, the spontaneously hypertensive rat (SHR) strain, has been widely used for investigating hypertension and insulin resistance. In the present study, we investigated the effects of biotin administration on insulin resistance and hypertension in an SHR-derived strain, i.e. the stroke-prone SHR (SHRSP) strain. The SHRSP is a model for human essential hypertension, which accompanies stroke, abnormal blood glucose levels(Reference Yamori, Ohtaka, Ueshima, Nara, Horie, Shimamoto and Komachi16), and insulin resistance in adipocytes(Reference Collison, Glazier, Graham, Morton, Dominiczak, Aitman, Connell, Gould and Dominiczak17) and skeletal muscles(Reference James, Cairns, Salt, Murphy, Dominiczak, Connell and Gould18). We used the following two controls: Wistar/Slc (Wistar) and Wistar–Kyoto (WKY) rat strains. The WKY strain is a normotensive reference strain from which the SHR were originally derived. Using the aforementioned strains, we attempted to evaluate the effect of the administration of a pharmacological dose of biotin on blood pressure and the incidence of stroke. Here, we describe a novel beneficial effect of biotin supplementation on hypertension.
Materials and methods
Materials
N G-nitro-l-arginine methyl ester (l-NAME) and 1H-[1,2,4] oxadiazole [4,3-α]quinoxalin-1-one (ODQ) were purchased from Dojindo Laboratory Co., Ltd (Kumamoto, Japan) and Cayman Chemical Company (Ann Arbor, MI, USA), respectively. All other chemicals were purchased from Wako Pure Chemical Industries, Ltd (Osaka, Japan).
Experimental animals
Male SHRSP–Izumo and WKY–Izumo rats were obtained from the inbred colonies maintained in Tohoku University. Male Wistar rats were obtained from Funabashi Farms Co., Ltd (Chiba, Japan). All the rats were housed individually in standard cages under the following controlled conditions: temperature, 23°C; humidity, 50 ± 5 %; and a 12 h light–dark cycle. They were fed a commercial pelleted diet (F-2; Funabashi Farms Co., Ltd) containing 150 μg biotin/kg and had free access to food and drinking water. All procedures involving animals were performed and the animals were cared for in conformity with the institutional guidelines of Tohoku University.
Measurement of systolic blood pressure
Systolic blood pressure (SBP) was measured in the conscious state by the tail-cuff method(Reference Kato, Iwase, Kanazawa, Nishizawa, Zhao, Takagi, Nagata, Noda, Koike and Yokota19) using a blood pressure monitor for mice and rats (MK2000 instrument; Muromachi Kikai Co., Ltd, Tokyo, Japan) as described previously(Reference Shirakawa, Koseki, Ohinata, Hashizume and Komai20). The average of three consistent readings (less than 10 mmHg tolerance) was obtained and considered as the individual SBP.
Long-term biotin administration in the Wistar–Kyoto and stroke-prone spontaneously hypertensive rat strains
Based on their SBP, we divided twelve rats (4 weeks old) of each strain equally into the following two groups: a control group and a high-dose biotin group. Distilled water (DW) was provided to the former and DW containing biotin (3·3 mg/l) was provided to the latter for 8 weeks. The amount of water consumed by each rat was measured once every 2 d. During the experimental period, the SBP, body weight, and oral glucose tolerance of each rat were measured once per week. At the end of the experimental period, all the rats were fasted for 16 h, and blood was collected from the abdominal aorta under diethyl ether anaesthesia. The plasma was separated immediately by centrifugation and stored at − 20°C until analysis. The liver, kidney, adrenal gland, spleen, heart, and lung were excised and weighed. The liver, kidney, heart, and skeletal muscle were frozen at − 80°C for further analysis.
Administration of a single dose of biotin in stroke-prone spontaneously hypertensive rats
Biotin (0, 0·05, 0·5, and 5 mg) was dissolved in 1 ml saline (pH 7·3) and sterilised by filtering through a 0·2 μm cellulose acetate filter (Advantec Toyo Ltd, Tokyo, Japan). This solution was administered intraperitoneally into 8-week-old SHRSP, and SBP was measured before administration and at 2, 4, 6, 8 and 10 h after administration. For the purpose of oral administration, 0·5 mg biotin was dissolved in 0·5 ml DW, and this solution or vehicle was administered to the SHRSP (each group, n 18) by gastric intubation. SBP was measured before administration and at 0·5, 1, 2, 4, 6, 8 and 10 h after administration.
Stroke incidence in the stroke-prone spontaneously hypertensive rat strain
Based on their SBP, another eighteen SHRSP (10 weeks old) were divided into the following four groups: (i) DW group (n 5), wherein the rats were provided DW for drinking water; (ii) DW + biotin group (n 4), wherein the rats were provided DW containing biotin (3·3 mg/l); (iii) saline group (n 5); (iv) saline + biotin group (n 4), wherein the rats were provided saline containing biotin (3·3 mg/l). The onset of stroke and survival of each SHRSP was monitored. The onset of stroke was assessed by the appearance of neurological symptoms and behavioural changes such as hyper- or hypokinesia, paralysis, loss of body weight, decreased food consumption and increased water intake(Reference Tabuchi, Umegaki, Ito, Suzuki, Ikeda and Tomita21).
Treatment of the stroke-prone spontaneously hypertensive rat strain with blocking reagents
ODQ was dissolved in dimethyl sulfoxide and diluted with saline. We injected 1 ml ODQ solution (0·8 mg ODQ/ml saline containing 15 % dimethyl sulfoxide) or vehicle subcutaneously into 16-week-old SHRSP. After 15 min, 0·5 mg biotin dissolved in 1 ml saline was injected subcutaneously. SBP was measured before and 2 h after biotin administration. For l-NAME administration, 7·5 mg l-NAME was dissolved in 0·5 ml saline and injected intraperitoneally into 10-week-old SHRSP. After 15 min, 0·5 mg biotin dissolved in 1 ml saline was injected intraperitoneally. SBP was measured before and 2 h after biotin administration.
Oral glucose tolerance test
We performed the oral glucose tolerance test once per week during the experimental period. All the rats were deprived of food and water for 16 h. Subsequently, glucose (1·8 g/kg body weight) was administered to each rat by gastric intubation. Blood was collected from the tail vein without anaesthesia before and at 30, 60 and 120 min after glucose administration. The plasma glucose and insulin concentrations were determined using the Glucose CII-test Wako kit (Wako Pure Chemicals Industries Ltd, Osaka, Japan) and ShionoRIA insulin kit (Shionogi & Co., Ltd), respectively. The incremental area under the curves for glucose and insulin concentrations were calculated according to the method described in the report of a joint FAO/WHO expert consultation(22).
Measurement of biotin concentration
Biotin concentration was determined by using the Lactobacillus plantarum bioassay as described previously(Reference Furukawa, Kinoshita, Satoh, Kikuchi, Ohkoshi, Maebashi, Makino, Sato, Ito and Kimura23). Briefly, the tissues were homogenised with five volumes of 40 mm-phosphate buffer (pH 7·2) using a Biotron homogenizer (Biotrona, Küssnacht, Switzerland). The plasma and tissue homogenates were hydrolysed with an equivalent volume of 2·8 m-H2SO4 at 120°C for 2 h and subsequently neutralised with 5 m-NaOH. Each sample was added to the assay medium for d-biotin (Nissui Co., Ltd, Japan) and sterilised at 121°C for 10 min. Next, a sample of the bacterial solution was added to the assay medium and incubated at 37°C for 24 h. The absorbance was measured at 660 nm and compared against a biotin standard curve.
Histological analysis
The lower half of the dissected heart was immediately fixed in 10 % phosphate-buffered formaldehyde (pH 7·2) for 48 h. The fixed samples were washed, hydrated and embedded in paraffin according to the standard procedure. The 4 μm thick sections were cut transversely and stained with haematoxylin and eosin. The ratio of the wall thickness to the lumen diameter of the coronary artery, which is representative of the arteriosclerotic index, was determined using an eyepiece micrometer at 600 × magnification.
Statistical analysis
Statistical significance was evaluated by using ANOVA along with Scheffé's test. A P value of less than 0·05 was considered as statistically significant. Statistical analysis was performed using the StatView 5 software (SAS Institute, Cary, NC, USA).
Results
Long-term administration of biotin in the Wistar–Kyoto and stroke-prone spontaneously hypertensive rat strains
First, we investigated the effects of long-term biotin administration in the SHRSP strain. We divided twelve rats (4 weeks old) of each strain into a control group (normal biotin group) and a high-dose biotin group and provided these groups with DW or DW containing biotin (3·3 mg/l), respectively, for 8 weeks. The growth curve of the rats in each group is shown in Fig. 1 (A). There was no difference with regard to body-weight gain in any of the strains between the two groups. The body weights of the WKY rats were significantly higher than those of the Wistar and SHRSP strains (P < 0·05). Fig. 1 (B) shows the total water intake of the rats in each group during the experimental period. In both the control and biotin groups, the water intakes were significantly higher in the WKY strain than in the Wistar strain (P < 0·05); additionally, the water intakes were significantly higher in the SHRSP strain than in the WKY strain. There was no significant difference with regard to the water intakes between the control and biotin groups in any of the strains.
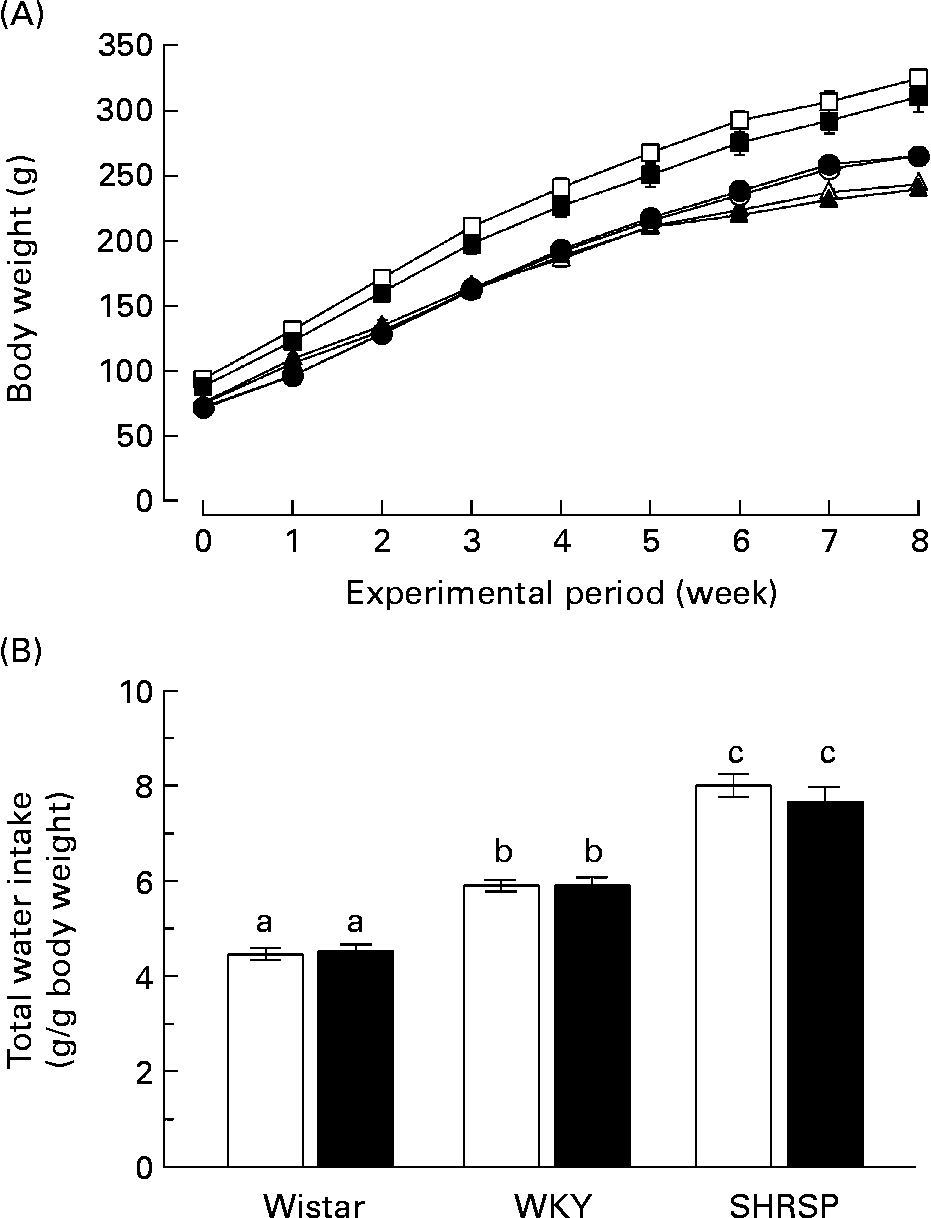
Fig. 1 (A) Growth curves of rats in each group during the experimental period. Twelve rats (4 weeks old) of each strain (Wistar/Slc (Wistar), Wistar–Kyoto (WKY) and stroke-prone spontaneously hypertensive rats (SHRSP)) were divided into two groups and provided with distilled water or distilled water containing biotin (3·3 mg/l) for 8 weeks. (○), Wistar controls; (●), Wistar high-dose biotin; (□), WKY controls; (■), WKY high-dose biotin; (Δ), SHRSP controls; (▲), SHRSP high-dose biotin. Values are means for six rats per group, with standard errors represented by vertical bars. (B) Total water intake. The amount of water consumed within 1 week was divided by the body weight of the rats during that week. The values obtained during the experimental period were summated. Values are means for six rats per group, with standard errors represented by vertical bars. Intake of 1 g water is equivalent to the ingestion of 3·3 μg biotin. (□), Control group; (■), high-dose biotin group. a,b,c Mean values with unlike letters are significantly different (P < 0·05).
All the rats were killed at the end of the 8-week experimental period. The biotin concentrations in the plasma and organs are shown in Table 1. With regard to both the WKY and SHRSP strains, the plasma concentrations of biotin were significantly higher in the biotin group than in the control group. Additionally, the biotin concentration in the skeletal muscle of the SHRSP control group was significantly lower than that observed in the other strains. Specifically with regard to the SHRSP strain, the biotin concentration in the skeletal muscle was significantly increased subsequent to biotin administration. With regard to the biotin concentration in the liver, no significant differences were observed between the groups of any strain. The pooled concentrations of hepatic biotin were high in all the rats, thereby indicating sufficient biotin intake.
Table 1 Biotin concentrations in plasma (ng/ml) and organs (ng/g wet tissue)
(Mean values with their standard errors for six rats per group)

Wistar, Wistar/Slc; ND, not determined; WKY, Wistar–Kyoto; SHRSP, stroke-prone spontaneously hypertensive rat.
a,b,c Mean values within a column with unlike superscript letters are significantly different (P < 0·05).
Biotin showed little effect on glucose homeostasis in the stroke-prone spontaneously hypertensive rat strain
The SHRSP strain is an experimental model for human essential hypertension and insulin resistance. We examined the effect of biotin administration on glucose tolerance in the SHRSP strain. The response of blood glucose and insulin to the oral glucose tolerance test was calculated as the incremental area under the curve. Fig. 2 shows the glucose tolerance of the rats in each group. Biotin had little effect on the incremental area under the curves of blood glucose (Fig. 2 (A)) and insulin (Fig. 2 (B)) in the SHRSP strain, at least under these experimental conditions. Further, biotin had no effect on glucose tolerance in the non-diabetic Wistar rats.

Fig. 2 The effect of biotin administration on glucose tolerance in the Wistar/Slc (Wistar) and stroke-prone spontaneously hypertensive rat (SHRSP) strains. The response of blood glucose (A) and insulin (B) to the oral glucose tolerance test is presented as the incremental area under the curve (IAUC). (○), Wistar controls; (●), Wistar high-dose biotin; (□), SHRSP controls; (■), SHRSP high-dose biotin. Values are means for six rats per group, with standard errors represented by vertical bars.
Biotin decreased systolic blood pressure in the stroke-prone spontaneously hypertensive rat strain
We also evaluated the effect of biotin administration on hypertension in the SHRSP strain. The WKY strain that comprises normotensive rats with impaired glucose homeostasis and insulin resistance was used as the control. During the experimental period, SBP was recorded once per week in each rat. As shown in Fig. 3 (A), biotin administration significantly decreased the SBP of the SHRSP; however, it did not affect the SBP of the WKY strain. In the SHRSP stain, the antihypertensive effect of biotin was evident at 2 weeks from the commencement of the experimental period. At this time, the SBP of all the rats in the SHRSP control group exceeded 140 mmHg.
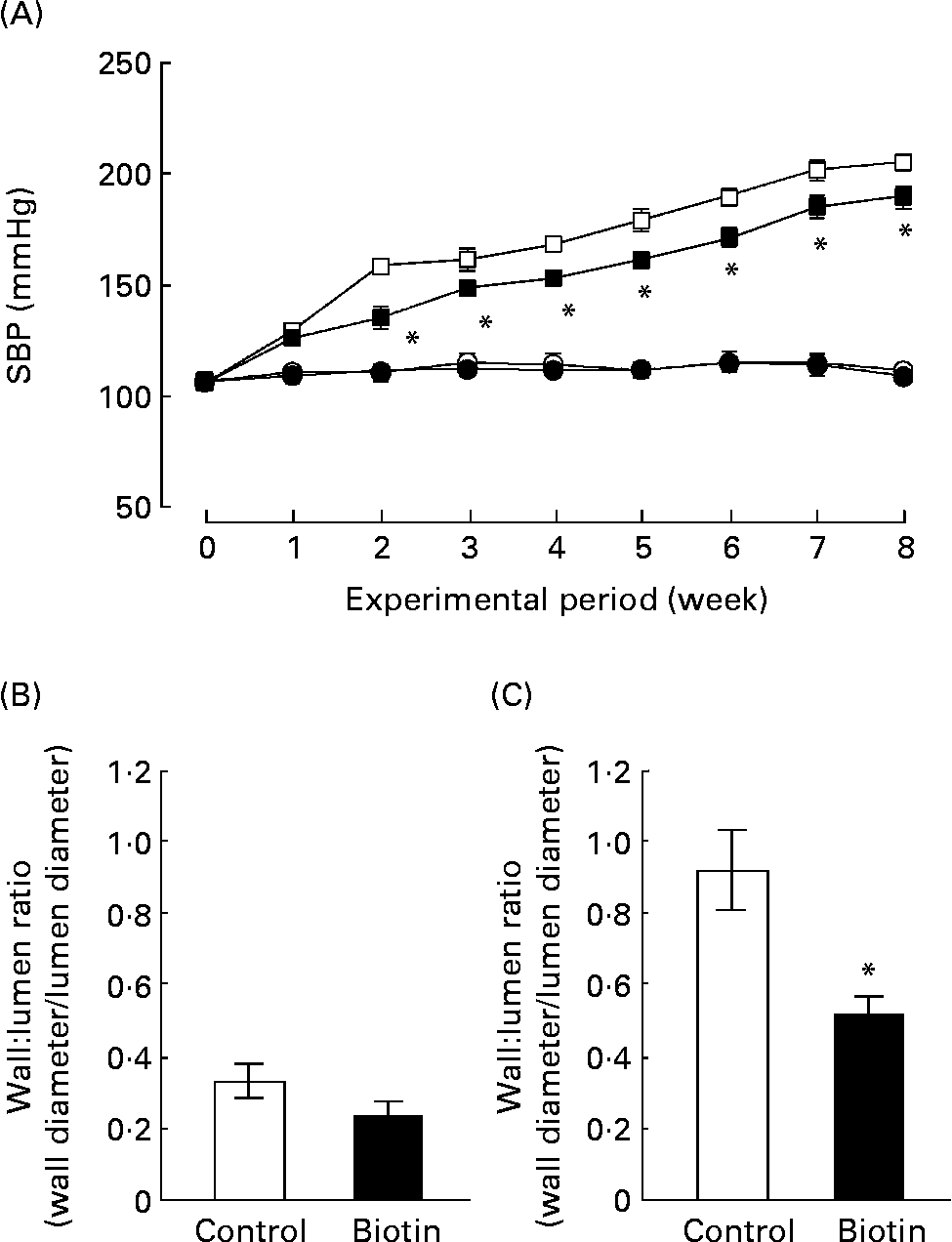
Fig. 3 The effect of biotin administration on the systolic blood pressure (SBP) (A) and wall:lumen ratio of the coronary artery in the Wistar–Kyoto (WKY) (B) and stroke-prone spontaneously hypertensive rat (SHRSP) (C) strains. (A) SBP was measured by the tail-cuff method with the MK2000 instrument. (○), WKY controls; (●), WKY high-dose biotin; (□), SHRSP controls; (■), SHRSP high-dose biotin. Values are means for six rats per group, with standard errors represented by vertical bars. * Mean value is significantly different from the corresponding value of the control group (P < 0·05). The ratio between the wall thickness and lumen diameter of the coronary artery was determined from 4 μm heart sections in WKY rats (B) and SHRSP (C). (□), Control group; (■), high-dose biotin group. Values are means for six rats per group, with standard errors represented by vertical bars. * Mean value is significantly different from that of the control group (P < 0·05).
At the end of the experimental period, the ratio of the wall thickness to the lumen diameter (i.e. wall:lumen ratio) of the coronary artery was measured as an index of coronary arterial thickening. As shown in Fig. 3 (B) and (C), this ratio was three times higher in the SHRSP strain than in the WKY strain. In the SHRSP strain, biotin administration decreased the wall:lumen ratio of the coronary artery to approximately half of that observed in the control group. These results indicate that biotin administration resulted in the reduction of coronary medial thickening in the SHRSP strain.
Table 2 shows the relative weights of the organs of the rats in each group. The relative weights of the liver, kidney, spleen, and heart of the control group of the SHRSP strain were significantly greater than those of the corresponding group of the WKY strain (P < 0·05). No significant difference was observed with regard to the relative weights of these organs between the control and biotin groups in any strain.
Table 2 Relative weights of the organs*
(Mean values with their standard errors for six rats per group)

WKY, Wistar–Kyoto; SHRSP, stroke-prone spontaneously hypertensive rat.
a,b Mean values within a column with unlike superscript letters are significantly different (P < 0·05).
* The weights are adjusted with respect to body weight and are expressed as g/100 g body weight.
Biotin delayed the onset of stroke in the stroke-prone spontaneously hypertensive rat strain
The SHRSP strain is salt sensitive, and SHRSP demonstrate a high incidence of cerebrovascular stroke. Further experiments were undertaken to investigate the effect of biotin on the incidence of stroke. We fed 10-week-old SHRSP for 8 weeks with saline or saline containing biotin (3·3 mg/l) instead of DW. At the end of the experimental period, all the rats that consumed saline without biotin (i.e. the saline group; n 5) exhibited stroke. Mortality in the saline group was estimated as 40 %. In contrast, the rats that consumed saline containing biotin (i.e. the saline + biotin group; n 4) showed no signs of stroke and remained alive throughout the experimental period. The incidence of stroke in the rats provided exclusively DW (i.e. the DW group; n 5) and DW with biotin (i.e. the DW + biotin group; n 4) were 20 and 0 %, respectively. The mean daily fluid intake of the rats in each group was as follows: DW group, 35·7 (sem 1·8) g/d; DW + biotin group, 36·1 (sem 1·4) g/d; saline group, 66·1 (sem 2·5) g/d; saline + biotin group, 50·5 (sem 7·2) g/d. There were no significant differences with regard to the fluid intake between the DW and DW + biotin groups and between the saline and saline + biotin groups. These results indicate that biotin has the potential to offer protection against stroke in the SHRSP strain; however, this requires further investigation using a larger sample size.
Administration of a single dose of biotin immediately decreased systolic blood pressure in the stroke-prone spontaneously hypertensive rat strain
Subsequently, we addressed whether administration of a single dose of biotin could reduce hypertension in the SHRSP strain. Therefore, SHRSP with hypertension were intraperitoneally administered saline or saline containing 0·05, 0·5 or 5 mg biotin, and the time course of alterations in SBP was measured. Surprisingly, the single dose of biotin immediately decreased SBP in the SHRSP strain (Fig. 4). The rats administered 0·5 and 5 mg biotin showed significantly lower SBP than the control rats (i.e. only the vehicle was administered) after 6–10 h of biotin administration. We confirmed the hypotensive action of biotin by oral administration of a single dose of biotin (data not shown). These results indicate that biotin has a direct hypotensive effect in the SHRSP strain.
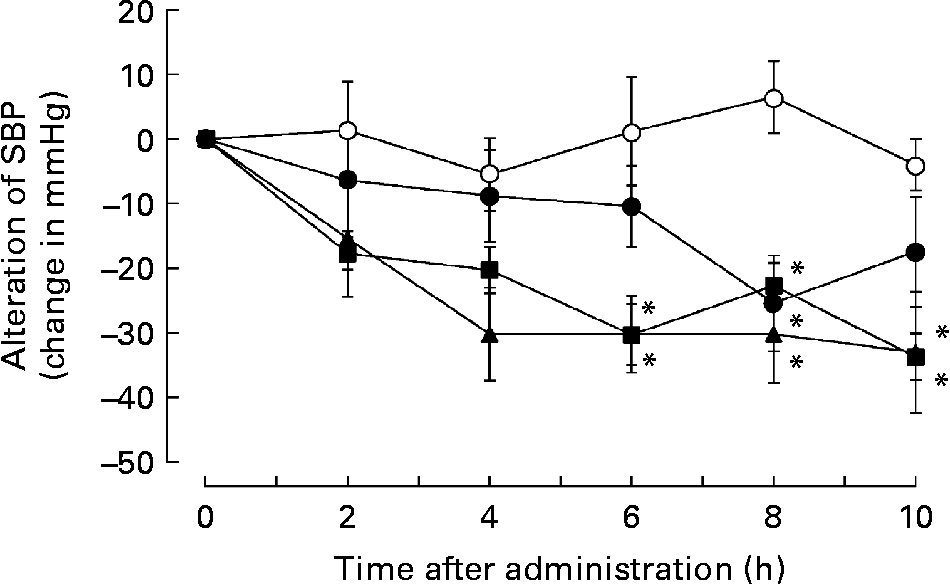
Fig. 4 The effect of single-dose biotin administration on systolic blood pressure (SBP) in the stroke-prone spontaneously hypertensive rat (SHRSP) strain. Eight-week-old SHRSP were administered saline containing 0 (○; n 6), 0·05 (●; n 5), 0·5 (■; n 7) and 5 (▲; n 5) mg biotin intraperitoneally, and the time course of alterations in the SBP was measured. Values are means, with standard errors represented by vertical bars. * Mean value is significantly different from that of the group that received 0 mg biotin (vehicle) (P < 0·05).
The guanylate cyclase inhibitor 1H-[1,2,4]oxadiazole [4,3-α]quinoxalin-1-one prevented the action of biotin on systolic blood pressure
It has been reported that biotin increases soluble guanylate cyclase (sGC) activity and cGMP level(Reference Singh and Dakshinamurti24–Reference Spence and Koudelka26). sGC (EC 4·6·1·2) belongs to an enzyme family that catalyses the conversion of GTP to cGMP. The cascade involving sGC- and cGMP-dependent kinases plays an important role in the relaxation of smooth muscles and the consequent lowering of blood pressure.
Accordingly, we investigated whether biotin affected the cGMP synthesis pathway in the SHRSP strain. In order to block the cGMP cascade, we administered ODQ, a selective sGC inhibitor, along with biotin to the SHRSP. In this experiment, the hypertensive SHRSP were pretreated with 0·8 mg ODQ or vehicle by a subcutaneous injection 15 min before the administration of a single dose of biotin. As shown in Fig. 5 (A), subcutaneous injection of 0·5 mg biotin into the SHRSP markedly decreased their SBP, whereas this hypotensive action was completely abolished by the ODQ pretreatment.
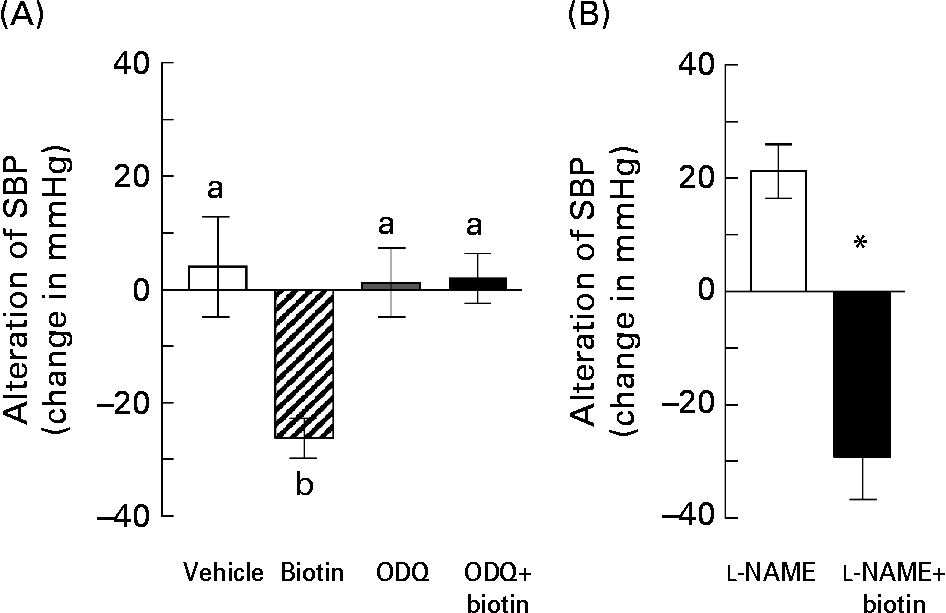
Fig. 5 The effects of treatment with blocking reagents on the hypotensive action of biotin in the stroke-prone spontaneously hypertensive rat (SHRSP) strain. (A) Biotin (0·5 mg) was administered subcutaneously into 16-week-old SHRSP 15 min after administering 0·8 mg 1H-[1,2,4]oxadiazole [4,3-α]quinoxalin-1-one (ODQ). Systolic blood pressure (SBP) was measured before and 2 h after biotin administration. (□), Vehicle only (n 3); (▨), biotin only (n 4); (), ODQ only (n 5); (■), ODQ and biotin (n 4). Values are means, with standard errors represented by vertical bars. a,b Mean values with unlike letters are significantly different (P < 0·05). (B) Biotin (0·5 mg) was administered intraperitoneally into 10-week-old SHRSP 15 min after administering 7·5 mg N G-nitro-l-arginine methyl ester (l-NAME). SBP was measured before and 2 h after biotin administration. (□), l-NAME only (n 6); (■), l-NAME and biotin (n 5). Values are means, with standard errors represented by vertical bars. *Mean value is significantly different from that of the l-NAME group (P < 0·05).
Further, we investigated the involvement of NO in the hypotensive effect of biotin in the SHRSP strain. NO is known to mediate vasodilation by functioning as an endothelium-derived relaxing factor via sGC activation. l-NAME is a universal inhibitor of NO synthase, which is a rate-limiting enzyme for NO synthesis. In this experiment, 7·5 mg l-NAME was administered intraperitoneally in 10-week-old SHRSP 15 min before the administration of a single dose of biotin. Unlike ODQ, l-NAME had no inhibitory effects on the hypotensive action of biotin (Fig. 5 (B)). On the other hand, l-NAME treatment without biotin administration significantly increased the SBP in the SHRSP strain, thereby indicating that the l-NAME dose is sufficient for blocking NO synthesis. Taken together, these results suggest that the hypotensive action of biotin in the SHRSP strain could have resulted from the elevation of the cGMP level via NOS-independent sGC activation.
Discussion
According to the results, biotin exerted a notable antihypertensive effect in the SHRSP strain. A pharmacological dose of biotin administration caused a prompt decrease in the SBP values in the SHRSP strain. Further, it decreased the hypertension-induced coronary arterial thickening and delayed the onset of stroke in this strain. This is the first report regarding the potentially therapeutic effects of biotin in lowering blood pressure and offering protection against stroke.
It is well known that NO release and the cGMP cascade play crucial roles in the regulation of blood pressure. Several studies have suggested that the impaired NO release from the endothelial cells(Reference Malinski, Kapturczak, Dayharsh and Bohr27) and kidneys(Reference Hirata, Hayakawa and Kakoki28) is related to the hypertensive state of SHRSP. Biotin is known to function as a direct sGC activator(Reference Vesely25) and it rapidly increases the cGMP level in a dose-dependent manner(Reference Spence and Koudelka26). In the present study, we found that pretreatment with sGC inhibitor ODQ eliminated the hypotensive effect of biotin in the SHRSP (Fig. 5 (A)), whereas pretreatment with NO synthase inhibitor l-NAME had no effect on the biotin activity (Fig. 5 (B)). Hence, we propose that the mechanism underlying the hypotensive action of biotin in the SHRSP strain is based on an NO-independent direct activation of sGC. Since the hypotensive effect of biotin was not observed in the normotensive WKY strain, we believe that biotin administration may have improved the depressed endothelium-dependent vasodilation in the SHRSP strain(Reference Tesfamariam and Halpern29, Reference Mayhan, Faraci and Heistad30); however, elucidating the actual mechanism responsible for this warrants further investigation.
Further, biotin delayed the onset of stroke in the SHRSP strain, although this finding is based on a relatively small sample size. Hypertension is one of the major risk factors for cardiovascular, coronary artery and renal vascular diseases. The incidence of stroke mortality in the SHRSP strain is salt sensitive and closely correlated with their hypertensive states. Under the salt-loaded condition, SHRSP showed signs of stroke during the 8-week experimental period, and biotin administration effectively suppressed the incidence of stroke under this condition. The SHRSP develop ischaemic stroke due to hypertension and endothelial dysfunction. Coronary arterial thickening was observed in the SHRSP strain, in that the wall:lumen ratio was approximately three times than that observed in the WKY strain (Fig. 3 (B) and (C)). Biotin administration significantly decreased this ratio in the SHRSP strain (Fig. 3 (B)). Thus, biotin might prevent cardiovascular damage in the SHRSP strain by lowering the blood pressure and offering protection against medial hyperplasia.
Organic nitrates have been widely used for the treatment of angina pectoris, IHD, heart failure and hypertension. The nitrates provide NO during catabolism and induce vasodilation by activating the cGMP cascade. On the other hand, the acute hypotensive effect of nitrates and the attenuated sensitivity of vascular smooth muscle to the nitrates, termed nitrate tolerance, have limited this efficacy. In contrast, biotin did not show tolerance to the hypotensive effect in the SHRSP strain, and its effect on SBP was moderate (Fig. 3 (A)). Biotin is a safe water-soluble vitamin with no serious adverse side effects even when administered in high doses, and it would be a good candidate for the adjunctive treatment of hypertension in combination with other antihypertensive drugs.
Numerous studies have shown that biotin affects glucose homeostasis in both animals and human subjects. In the SHRSP strain, we observed that biotin exerted a stronger effect on SBP than on glucose tolerance. We did not determine whether the effect of biotin on SBP is dependent on the same mechanism that is responsible for its regulatory effect on glucose homeostasis; however, biotin did not bring about SBP modulation in the WKY strain, which has impaired glucose homeostasis and insulin resistance. These findings suggest that biotin directly decreased the blood pressure of the SHRSP and that this effect was not due to the result of the improved glucose homeostasis. This also implies that biotin may have the potential to improve the pathophysiology of hypertension in non-diabetic animals.
In conclusion, the present study shows that biotin has a beneficial effect on hypertension and the incidence of stroke in the SHRSP strain. Since biotin deficiency is a rare nutritional disorder, the importance of biotin has not been adequately addressed within the vitamin B-complex family. It has been suggested that biotin improves glucose metabolism and insulin sensitivity. Chromium picolinate with biotin (DIACHROME) is used as a cost-effective and safe adjunctive treatment for the management of type 2 diabetes(Reference Fuhr, He, Goldfarb and Nash31). In addition, biotin has several biological functions, including its role as a cofactor for carboxylases. It has been demonstrated that biotin regulates the expression of several genes via sGC activation(Reference Chauhan and Dakshinamurti8, Reference Solorzano-Vargas, Pacheco-Alvarez and Leon-Del-Rio10–Reference De La Vega and Stockert12). Mock et al. reported that marginal asymptomatic biotin deficiency is commonly observed during normal human pregnancy(Reference Mock and Stadler32, Reference Mock, Stadler, Stratton and Mock33) and is associated with potential teratogenesis(Reference Mock, Quirk and Mock34). We believe that our findings add to this important albeit limited information regarding the functions of this vitamin.
Acknowledgements
We gratefully acknowledge the technical assistance provided by Nur Hayati, Ardiansyah, Mutsumi Ose, Takuya Hashimoto, Yumi Sugita, Motoko Murakami and Mario Iwamoto. The present study was supported by a Grant-in-Aid for Scientific Research from the Japan Society for the Promotion of Science. M. W.-K. and S. K. both contributed equally to the present article.