Anaemia remains one of the most common and intractable public health problems, particularly in developing countries. According to global estimates, as many as two billion people worldwide suffer from anaemia, with pregnant women, adolescent girls, and infants and young children facing the greatest burden of this disease( 1 , 2 ). The causes of anaemia are multifactorial, including nutritional deficiencies, infections and blood disorders( 1 , Reference Stoltzfus, Chwaya and Montresor 3 ).
A crude but often cited estimate suggests that as much as 50 % of anaemia can be attributed to Fe deficiency globally( 2 ). The global burden of Fe deficiency has been estimated from anaemia prevalence surveys, and though the methodology has its limitations, the estimates are large and point to a major public health problem. The WHO has estimated that 41 % of women and 27 % of preschool children suffer from anaemia due to Fe deficiency( 2 ), with considerable variability by geography and age group. The consequences of Fe-deficiency anaemia (IDA) have been well documented( 1 , Reference Lozoff 4 – Reference Beard 6 ). In children, IDA is associated with delayed or impaired cognitive and physical development. Among adults, IDA may result in reduced physical work capacity and productivity. Anaemic women and children are at a greater risk of dying during the perinatal period. The economic consequence of Fe deficiency (ID), resulting from motor and mental impairment in children and low work productivity in adults, estimated from ten developing countries, is 4 % of gross domestic product( Reference Horton and Ross 7 ).
During the first 6 months of life, full-term, normal-birth-weight babies are virtually self-sufficient with regard to their Fe needs. These babies are born with sufficient Fe stores to meet their physiological Fe needs( Reference Domellöf 8 ). Though Fe concentrations in breast milk are very low, Fe is in a highly bioavailable form. The combination of high Fe stores at birth and limited, but highly bioavailable, Fe in breast milk ensures that no additional Fe is required until approximately 6 months of age, after which requirements increase rapidly at a time when hepatic Fe stores accumulated during gestation are depleted. Fe requirements during the 6–11-month age period are higher than those at any other period of life, corresponding to rapid infant growth and brain development. Beginning at approximately 6 months of age, infants require additional sources of Fe to prevent Fe deficiency and its ensuing sequelae. Typically, this additional source of Fe is found in Fe-rich complementary foods, which may be meat products or Fe-fortified foods. In developing countries, the low availability and lack of access to Fe-fortified foods or costly animal-source foods and the poor Fe bioavailability in typical cereal-based complementary foods place large numbers of children at an increased risk of developing ID and anaemia during the critical complementary feeding phase.
While being born with a full endowment of Fe stores and ensuring adequate dietary Fe intake after 6 months of age are critical to prevent the development of anaemia among infants and young children, these alone are insufficient in the presence of infections. Infection control is an essential complementary strategy. Anaemia brought about by infections, referred to as anaemia of inflammation or anaemia of chronic disease, is mediated by pro-inflammatory cytokines that induce changes in Fe homeostasis, the proliferation of erythroid progenitor cells, the production of erythropoietin and the lifespan of erythrocytes, all of which contribute to the pathogenesis of anaemia( Reference Weiss and Goodnough 9 ). Additionally, during inflammation, pro-inflammatory cytokines stimulate the production of the hormone hepcidin, which in turn reduces Fe absorption, independent of Fe status, leading to tissue Fe deficiency( Reference Nemeth and Ganz 10 ).
In Bangladesh, estimates suggest that anaemia affects twenty-seven million children, adolescents and women and that the economic costs are up to 7·9 % of gross domestic product( 11 ). The objectives of the present study were to examine the contribution that ID, infections and feeding practices make to anaemia, among Bangladeshi infants during the critical early complementary feeding period between 6 and 11 months of age. A strength of the present analysis is the availability of multiple Fe status indicators and markers of subclinical infections that allows for a close examination of the proportion of anaemia that is due to ID, infections and poor feeding practices. This information can help guide the development of targeted interventions that can alleviate the burden that anaemia and ID impose on rural Bangladeshi infants.
Methods
Study design and population
The present study was conducted in twenty subdistricts (upazillas) in rural Bangladesh that are part of BRAC's Essential Health Care programme. Baseline results are presented from a survey of 1600 infants aged 6–11 months who were recruited from 200 villages into a cluster-randomised 2 × 2 factorial design study investigating the impact of the sale of micronutrient powders (Pushtikona) by frontline health workers to households (HH) with young children and/or targeted behaviour change communication interventions to improve infant and young child feeding (IYCF) practices. HH were randomly selected based on the presence of a child aged 6–11 months who resided in the HH.
Data collection was conducted between April and June 2010. Trained interviewers visited the HH and administered a questionnaire that included demographic, health, nutrition, food security and socio-economic information at the child, maternal and HH levels. Over 95 % of eligible HH were approached for the study and they consented, and 98·5 % of all HH provided a blood sample for a child of 6–11 months of age. The HH questionnaire for the survey was based on the UNICEF conceptual framework on the causes of undernutrition( 12 ).
The present study was conducted according to the guidelines laid down in the Declaration of Helsinki, and all procedures involving human subjects were approved by the Bangladesh Medical Research Council and the Institutional Review Board at the International Food Policy Research Institute. Verbal informed consent was obtained from all subjects. Verbal consent was witnessed and formally recorded.
Biomarker collection and processing
Trained phlebotomists collected one drop of blood through great toe pricks from all infants of 6–11 months of age for Hb measurements, which were carried out using a Hemocue haemoglobinometer (Hb 201+; HemoCue America). An additional 300 μl of blood were collected in lithium-heparin-coated microvettes (CB 300; Sarstedt) for additional biomarker assessments. Immediately after phlebotomy, these pre-labelled microvettes were placed in an ice chest/cold box with ice packs, maintained at 4°C for transportation to a central laboratory in Dhaka. Blood samples were transported from the field to Dhaka on a daily basis and were processed no later than 24 h after phlebotomy. Precautions were taken to maintain proper temperature and to avoid physical shocks in order to prevent haemolysis of blood cells during transportation. On arrival in Dhaka, the blood samples were centrifuged for plasma separation. For analysis, 100 μl of plasma were aliquoted. The remaining plasma was aliquoted separately and stored as back-up. Pre-printed labels having identical numbers were provided to the technicians at the central laboratory for use on the aliquots containing plasma before placing them in the freezer. The samples were temporarily stored in a freezer at − 20°C in the central laboratory until shipment on dry ice for further analyses. Retinol-binding protein, C-reactive protein (CRP), α-1-acid glycoprotein (AGP), plasma ferritin (PF) and soluble transferrin receptor (TfR) levels were measured at the ‘VitA-Fe Tech’ laboratories (Willstaett, Germany) by a combined sandwich ELISA( Reference Erhardt, Estes and Pfeiffer 13 ). The CV (% CV) for these tests ranged from a low value of 3·67 % for AGP to a high value of 5·58 % for CRP.
Anthropometry and infant and young child feeding practices
Anthropometric measures included weight and recumbent length measurements of infants and weight and height measurements of their mothers. The weights of children and mothers were measured using the Health-o-meter® electronic scales (Sunbeam Products, Inc.) and recorded to the nearest 100 g. Locally manufactured, collapsible length/height boards, which were precise to 1 mm, were used to measure the recumbent length of infants and the standing height of mothers. All anthropometrists underwent rigorous training on the standardisation process( Reference Cogill 14 ).
Child feeding practices were assessed using the WHO-recommended methodology based on the previous-day recall( 15 ). This method and the indicators that can then be created are widely accepted and used to capture optimal feeding practices in populations. Optimal IYCF practices captured by these indicators cover a range of practices and criteria to assess the adequacy of these practices. These include age-appropriate breast-feeding practices (timing, duration and exclusivity) and timely introduction and adequate frequency of consumption and type of complementary foods, including consumption of Fe-rich foods.
Data analysis
Recognising the effect that subclinical infections have on micronutrient status indicators, attempts were made to control for this by measuring two different markers of inflammation – AGP and CRP – that capture both acute (CRP) and chronic (AGP) inflammation( Reference Thurnham, Mburu and Mwaniki 16 ). Subclinical infections were defined using a combination of elevated values of either AGP or CRP inflammation markers categorised by an AGP value >1 g/l or a CRP value >5 mg/l.
The following three different iron status indicators were measured: Hb; PF; TfR. Anaemia was defined as a Hb value < 105 g/l. This cut-off is lower than the 110 g/l cut-off proposed by the WHO and Centers for Disease Control (CDC) for children aged 6 months to 5 years. However, the 105 g/l cut-off reflects the only reference value derived from Fe supplementation data that were collected from breast-fed infants( Reference Domellöf 8 ) and is frequently used by researchers. Severe anaemia was defined as a Hb cut-off < 70 g/l( 17 ). We used three different definitions of ID using established cut-offs for PF and TfR. First, ID using the PF cut-off alone, after PF concentrations were adjusted for the presence of inflammation according to the methodology proposed by Thurnham et al. ( Reference Thurnham, Mccabe and Haldar 18 ), was defined as a PF value < 12 μg/l. Second, ID using the TfR cut-off alone was defined as a TfR value >8·3 mg/l. Third, ID was also derived using either the low PF or elevated TfR values given above. IDA was defined using combinations of the ID definitions given above and the anaemia definition. In analyses that examined the determinants of Fe stores among infants, the ID definition using the PF cut-off was used. Vitamin A deficiency was defined as a retinol-binding protein concentration < 0·7 μmol/l.
Weight and length measurements were used to derive Z-scores by comparing each child's anthropometric measurements with the 2006 WHO child growth standards for his/her age and sex. The three indicators created were length-for-age Z-score, weight-for-age Z-score and weight-for-length Z-score. Stunting is defined as a length-for-age Z-score < − 2, underweight is defined as a weight-for-age Z-score < − 2 and wasting is defined as a weight-for-length Z-score < − 2 Z-scores( 19 ).
Statistical analysis was conducted using STATA 11 (Stata Corp). An initial exploratory analysis was performed to generate descriptive statistics of the study population. Statistical trends of micronutrient status indicators were examined by age trends using the nptrend command in STATA. Bivariate and multivariate logistic regression models were run using the logistic command to generate OR. These models adjusted for the clustering of data at the unit of randomisation level (the subdistrict) using the cluster option in STATA.
Logistic regression analysis was used to model known predictors of anaemia; the models also included known or hypothesised confounding factors. These include (1) age, sex, nutritional status (child underweight), subclinical infections, ID, previous-day consumption of Fe-rich foods (defined as flesh and organ meat, fish, fortified infant foods and formulas, and micronutrient powders containing Fe)( 15 ) and the IYCF indicator representing a minimum acceptable diet at the level of the child; (2) maternal short stature (height < 145 cm) and education (quartiles of years of schooling); (3) HH size and total HH expenditure. Total HH expenditure (including food and non-food items) during the previous 30 d was obtained from each HH, and quartiles were derived and used in all analyses as a proxy of socio-economic status. The logit model for ID included all variables in the anaemia model, as well as maternal perception of relative birth size, as a proxy for birth weight data, which were unavailable. These variables were retained in the model being tested, regardless of whether they were significantly associated with the outcome variable in bivariate analyses. Adjusted population attributable fractions of anaemia were calculated from the multivariate logistic regression models using the aflogit command in STATA to estimate the proportion of anaemia in this population that might be prevented by the elimination of individual risk factors. A P value < 0·05 was considered significant for all inferential statistics, except for interactions that were considered significant at a P value < 0·10.
Results
Descriptive statistics
Of the 1600 infants aged 6–11 months recruited into the present study, blood samples were available for 1579 infants, and complete biomarker data were available for 1497 infants. There were no differences in demographic characteristics for those infants who had complete biomarker data compared with the full sample. Given the known influence that subclinical infections have on micronutrient status indicators, study characteristics are presented for both the whole study population and the subsample in whom inflammation was absent (Table 1). With the exception of PF, CRP and AGP concentrations, there were no differences in demographic or biological characteristics between the full sample and subsample. The burden of subclinical infections was high in this sample population with over one-third of all infants being classified as having some subclinical infection based on either elevated AGP or CRP levels. Anaemia was highly prevalent, with over 68 % of the infants having Hb concentrations < 105 g/l. When the WHO-recommended cut-off < 110 g/l was applied, the prevalence of anaemia increased to 84 %. The prevalence of ID, using either the PF or the TfR cut-off, was 57 % in the full sample and 55 % in the subsample. However, the prevalence differed markedly when ID was defined using the PF or TfR cut-off alone. The prevalence of ID was substantially higher when the TfR cut-off was used than on using the PF cut-off. Similarly, the prevalence of IDA varied depending on what criteria were used; the prevalence of IDA using either the PF or TfR cut-off in combination with the Hb cut-off was 44 % in the full sample and 41 % in the subsample. The burden of undernutrition as reflected by anthropometric indices was high in this population, especially considering the young age of our sample (6–11 months). Both stunting and wasting were highly prevalent, with 26 % of the infants being stunted (mean length-for-age Z-score: − 1·1 (sd 1·4)) and 18 % of the infants being wasted (mean weight-for-length Z-score: − 0·9 (sd 1·3)).
Table 1 Sample characteristics (Mean values and standard deviations; geometric means, percentages and 95 % confidence intervals)

PF, plasma ferritin; TfR, soluble transferrin receptor; AGP, α-1-acid glycoprotein; CRP, C-reactive protein; RBP, retinol-binding protein; LAZ, length-for-age Z-score; WLZ, weight-for-length Z-score; WAZ, weight-for-age Z-score; ID, Fe deficiency; IDA, Fe-deficiency anaemia; VAD, vitamin A deficiency.
* AGP >1 g/l or CRP >5 mg/l.
† PF concentrations adjusted for inflammation.
‡ LAZ < − 2.
§ WLZ < − 2.
∥ WAZ < − 2.
In both the full sample and subsample, a consistent pattern of increasing prevalence of anaemia and ID with age was observed, up until 8 months (trend statistically significant for infants aged 6–8 months), after which prevalence remained high, but generally plateaued (trend not statistically significant for infants aged 9–11 months) (Fig. 1). Anaemia peaked among infants of 8 months of age at 75 %, while ID (using a combination of either inflammation-corrected PF or TfR) peaked among infants of 9 months of age at 63 %. The prevalence of subclinical infections across age was roughly consistent, ranging from 31 to 38 %.
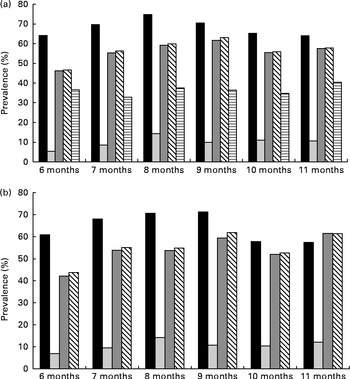
Fig. 1 Prevalence of anaemia, iron deficiency (ID) and subclinical infections in infants of 6–11 months of age, by age. (a) Full sample. There was a statistically significant (P< 0·001) trend for all indicators, except for inflammation, between 6 and 8 months of age. (b) Subsample (individuals without subclinical infections). There was a statistically significant (P< 0·001) trend for all indicators between 6 and 8 months of age. ■, Anaemia (Hb concentration < 105 g/l); , ID (adjusted plasma ferritin (PF) < 12 μg/l);
, ID (soluble transferrin receptor (TfR) >8·3 mg/l);
, ID (adjusted PF or TfR);
, any inflammation (α-1-acid glycoprotein >1 g/l or C-reactive protein >5 mg/l).
Infant and young child feeding practices
As expected, the consumption of all food groups increased with age (Fig. 2). By 11 months of age, however, only 23 % of all infants had consumed Fe-rich foods during the previous day. In the 8- and 9-month age groups, the age range at which anaemia and Fe deficiency peak, only 13 and 16 % of the infants, respectively, had consumed Fe-rich foods during the previous day. The most commonly consumed Fe-rich food was fish, which was consumed by up to 19 % of the infants by 11 months of age. Overall, feeding practices, in particular complementary feeding practices, of infants in this population were suboptimal, with less than 14 % of the infants having consumed Fe-rich foods on the previous day or having met the minimum recommended diversity in their diet and only 6 % having met the criteria for a minimum acceptable diet.
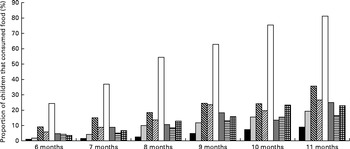
Fig. 2 Diversity of complementary foods fed to infants aged 6–11 months in the past 24 h, by age. There was a statistically significant (P< 0·001) trend for all foods between 6 and 11 months of age. ■, Flesh and organ meat; , fish;
, vitamin A-rich foods (pumpkin, orange yam, orange-red-flesh sweet potatoes, carrots, ripe papaya or ripe mango);
, green leafy vegetables; □, rice;
, pulses/lentils;
, eggs;
, iron-rich foods (flesh and organ meat, fish, iron-fortified infant foods and formulas, and micronutrient powders).
Risk factors for anaemia and iron deficiency: multivariate models
In multivariate logistic regression models, ID (using one of the three ID classifications) and subclinical infections were consistently statistically significant predictors of anaemia (Table 2). No statistically significant association between IYCF practices and Fe status indicators was found when modelled either as continuous or categorical variables. Among infants who were Fe deficient, the odds of being anaemic were 2·6–5·0 times higher (models 1, 2 or 3) than those in infants not deficient (P< 0·001) and 1·4–1·5 times higher (models 1, 2 or 3) among infants with subclinical infections (P< 0·01) than in those without subclinical infections. There were no differences by age group, with the exception of the 8-month-old infants who had a statistically significant higher risk of being anaemic (P< 0·05), compared with the reference group of infants of 6 months of age. Additional risk factors for anaemia were short maternal stature ( < 145 cm) and being male. HH socio-economic status, previous-day consumption of Fe-rich foods and the combined IYCF indicator of a minimum acceptable diet were not associated with anaemia. Population attributable fraction estimates show that 67 % (95 % CI 62, 71) of anaemia in this population might be prevented by the elimination of ID and 16 % (95 % CI 11, 19) by eliminating subclinical infections.
Table 2 Logistic regression models predicting anaemia in infants of 6–11·9 months of age (Odds ratios and 95 % confidence intervals)
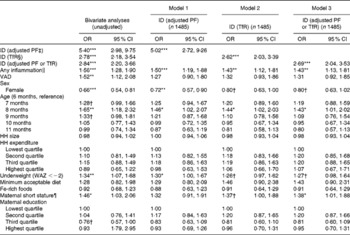
ID, Fe deficiency; PF, plasma ferritin; TfR, soluble transferrin receptor; VAD, vitamin A deficiency; HH, household; WAZ, weight-for-age Z-score.
* P< 0·05, ** P< 0·01, *** P< 0·001.
†P< 0·1.
‡ Adjusted PF concentration < 12 μg/l.
§ TfR concentration >8·3 mg/l.
∥ α-1-Acid glycoprotein >1 g/l or C-reactive protein >5 mg/l.
¶ Maternal height < 145 cm.
Similarly, subclinical infections, age and male sex were also significant risk factors for ID (Table 3). Additionally, babies whose mothers recalled them being born small relative to other babies in their community had greater odds of being Fe deficient, with a clear linear relationship. When the analysis was restricted to individuals without subclinical infections (data not shown) and a PF cut-off < 12 μg/l was used to indicate true depleted Fe stores, infants of 8–9 months of age were at a greatest risk of having depleted Fe stores (adjusted OR 2·00, 95 % CI 0·9, 4·4), and males were at a greater risk than females (adjusted OR 2·2, 95 % CI 1·5, 3·3). Indicators of complementary feeding practices were not statistically associated with ID.
Table 3 Logistic regression models predicting iron deficiency (ID) in infants of 6–11·9 months of age (Odds ratios and 95 % confidence intervals)
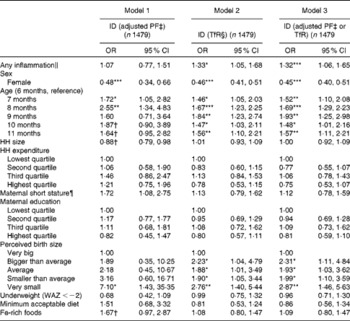
PF, plasma ferritin; TfR, soluble transferrin receptor; HH, household; WAZ, weight-for-age Z-score.
* P< 0·05, ** P< 0·01, *** P< 0·001.
†P< 0·1.
‡ Adjusted PF concentration < 12 μg/l.(18)
§ TfR concentration >8·3 mg/l.
∥ α-1-Acid glycoprotein >1 g/l or C-reactive protein >5 mg/l.
¶ Maternal height < 145 cm.
Though younger infants and those with subclinical infections were at a greatest risk of developing anaemia and ID, there were no differential associations between any other key risk factors such as consumption of Fe-rich foods or other IYCF indicators with subclinical infections or age; none of the tests for interactions between these variables and child age or subclinical infections was statistically significant.
Discussion
The present study reinforces the public health significance of anaemia, Fe deficiency and infections among infants of 6–11 months of age in rural Bangladesh. Anaemia affected 68 % of the infants, whereas more than half of the infants suffered from ID and one-third had subclinical infections. The present findings also indicate that up to 67 % of all cases of anaemia in this sample could be attributed to ID and 16 % to infections.
By any measure, the prevalence estimates of anaemia are alarming, and higher than expected, based on previous data. According to the Bangladesh national strategy for anaemia prevention and control( 11 ), approximately 65 % of children of 6–23 months of age are anaemic when using the WHO cut-off of Hb < 110 g/l, compared with 84 % of infants in our population using this standard. Two recent studies have reported prevalence estimates among infants of 6 months of age in rural Bangladesh of 46( Reference Eneroth, Persson and El Arifeen 20 ) and 55 %( Reference Shakur, Choudhury and Hyder 21 ) using the cut-off used in the present analysis. In the population of the present study, the prevalence of anaemia among infants of 6 months of age was 65 %. One plausible explanation for the higher prevalence of anaemia that we observed, compared with that reported in other studies carried out in Bangladesh, is the substantially higher prevalence of subclinical infections, which is more than twice as high as that observed in other reported studies.
The prevalence of ID varied significantly depending on the criteria used to define ID; using a combination of indicators that reflect either depleted Fe stores (PF) or decreased supply of Fe to tissues (TfR), it was observed that the prevalence of ID was 57 %. Reliable estimates of the prevalence of ID are scarce, given the wide set of indicators used to define ID and the influence that infections have on the more frequently used indicators. There is no global consensus as to which indicators are to be used to define ID particularly when infections are widespread. Rather, current recommendations urge the use of multiple Fe indicators as well as indicators of subclinical infections( 1 ) as has been done in the present study. However, these recommendations do not provide clarity on how exactly Fe indicators should be analysed in combination with the indicators of subclinical infections. The present study used a recently developed methodology( Reference Thurnham, Mccabe and Haldar 18 ) that adjusts PF concentrations for the presence of inflammation. Given the use of multiple Fe status indicators, it is important to understand what each of these indicators indicates. PF is a measure of the amount of Fe in body stores, and concentrations below the cut-offs used in our analysis represent depleted Fe stores. TfR has greater utility as a functional indicator of Fe deficiency; it reflects the demand for Fe and is a marker of the severity of Fe insufficiency only when Fe stores have been exhausted. TfR, unlike PF, is purported to be uninfluenced by inflammation. In the present study, these indicators were used separately and in combination in the multivariate analyses where ID was both an outcome of interest and a key determinant of anaemia.
In our sample, the risk of anaemia and ID increased significantly with age up until 8 months, after which the prevalence decreased slightly albeit remaining high. From the multivariate regression models, it was found that the risk of anaemia was greatest among 8-month-old infants, while the risk of ID was greatest among 8- or 9-month-old infants, depending on the definition of ID. This pattern of increased risk of anaemia up until approximately 8–9 months of age within a narrow 6-month age range (6–11 months of age) was unexpected. An earlier study carried out in Nepal has reported anaemia rates to be increasing progressively from 4 until 17 months of age, with 15–17-month-old children being almost four times more likely to be anaemic than infants aged 4–5 months( Reference Siegel, Stoltzfus and Khatry 22 ). The child feeding literature highlights the risk of anaemia and Fe deficiency associated with exclusive breast-feeding beyond 6 months of age( Reference Dewey 23 , Reference Meinzen-derr, Guerrero and Altaye 24 ). However, in our sample, exclusive breast-feeding rates, even among the youngest age group (6–8 months of age), were low (2·9 %) and exclusive breast-feeding practice was not a significant predictor of anaemia when statistically modelled.
We posit that suboptimal infant feeding practices, and in particular very low consumption of Fe-fortified foods or animal-source foods, combined with the poor Fe bioavailability of cereal-based complementary foods consumed during the 6–8-month age period corresponding to a period of increasing physiological Fe requirements are the main reasons for the increasing risk of anaemia found between 6 and 8 months of age. Overall, consumption of Fe-rich foods was very low in our sample; only 13·6 % had consumed such foods in the previous 24 h. Consumption of Fe-rich foods increased slightly with age, but in the 6–8-month-old group (corresponding to the increasing prevalence of anaemia), only 6·3 % had consumed flesh or organ meat on the previous day, 5·2 % had consumed fish and 7·7 % had consumed any Fe-rich foods.
Our analyses did not show a statistically significant association between consumption of Fe-rich foods and ID. This may have been due to the small percentage of infants who had consumed any Fe-rich foods, but also due to a hypothesised small quantity consumed. Additionally, our measure of consumption of Fe-rich foods captured intake for only 1 d (previous 24 h) of any such foods rather than the usual intake. Although the present study did not measure amounts consumed, a previous study has highlighted the fact that small amounts of complementary foods are indeed a major constraint in Bangladesh in this age group( Reference Kimmons, Dewey and Haque 25 ). The study showed that although meal frequency and energy density of complementary foods were consistent with recommendations, foods were generally of low micronutrient density and offered in small amounts, resulting in low micronutrient intakes among infants during the second half of infancy( Reference Kimmons, Dewey and Haque 25 ). Additionally, a recent analysis of 24 h dietary recalls among Bangladeshi children aged 6–23 months has also shown that only 10 % of children met their recommended daily Fe intake requirements( Reference Rasheed, Haider and Hassan 26 ). This is particularly striking, given that this sample included children in their second year of life who are more likely than infants of 6–11 months of age to consume Fe-rich foods. It is, therefore, likely that the lack of an observed association between consumption of Fe-rich foods and ID in our sample is due to a combination of a low proportion of children having consumed such foods and those who did with insufficient intakes. The contribution of Fe-rich foods to Fe intake is, therefore, likely to be insufficient to fulfil the dietary Fe requirements of rapidly growing infants.
In the present study, a significant sex difference was observed in the risk of anaemia and ID, with male children being at a greater risk. Several studies have corroborated these findings globally( Reference Domellof, Lonnerdal and Dewey 27 – Reference Yang, Lo and Adu-Afarwuah 29 ), but not in Bangladesh( Reference Eneroth, Persson and El Arifeen 20 , Reference Shakur, Choudhury and Hyder 21 ). Several biological mechanisms have been postulated including that boys have lower body Fe stores at birth or higher intestinal Fe losses than girls or that genetic or hormonal factors may play a role( Reference Domellof, Lonnerdal and Dewey 27 , Reference Wieringa, Berger and Dijkhuizen 30 ). The evidence concerning intra-HH food distribution and a possible bias towards favouring male children is mixed. Data suggest that, in South Asia, in particular, there is a pro-male bias in food choice as well as in the quantity of food offered, although a considerable variation within the region is found( Reference Haddad, Pena and Nishida 31 ). These differences are likely to arise due to inequalities in the intra-HH distribution of food as well as in differences in real v. perceived food requirements of different members in the HH based on their age and sex. That males were consistently at a greater risk of developing anaemia and ID than females in the present study is noteworthy because it goes against conventional wisdom of a possible pro-male bias in intra-HH food distribution in South Asia, but is in line with the proposed biological mechanisms.
Although birth weight data were unavailable, information on the mothers' perception of the size of their newborn babies was collected. This variable was found to be significantly associated with ID, with babies perceived to be smaller than normal at birth being at a greater risk of developing ID. This finding is plausible, given the well-documented fact that low-birth-weight babies are more vulnerable to early Fe depletion( Reference Stoltzfus 32 ).
Programmatic implications
The results of the present study suggest that a multipronged strategy for anaemia control, as proposed by the National Strategy for Anaemia Prevention and Control in Bangladesh( 11 ), is needed to address anaemia in rural Bangladesh. First, given the strong contribution of Fe deficiency to anaemia and the very low consumption of Fe-rich foods in the population studied, innovative ways to improve access to, and demand for, Fe supplements, Fe-rich foods or Fe-fortified foods or products for infants and young children are essential. This can be achieved either through routine Fe supplementation or by improving complementary diets. Though routine low-dose Fe supplementation among children aged 6 months and above is recommended by the WHO( Reference Stoltzfus and Dreyfuss 33 ), it is rarely implemented at scale. Therefore, delivery of Fe through Fe-fortified complementary foods or home fortificants (e.g. Fe-fortified micronutrient powders or lipid-based nutrient supplements) may be programmatically more feasible. The evidence base of the impact of micronutrient-fortified complementary foods and home fortificants among infants and young children is substantial. Data from trials of commercially processed Fe-fortified complementary foods( Reference Gera, Sachdev and Boy 34 , Reference Dewey and Adu-afarwuah 35 ) and home fortificants, including micronutrient powders and lipid-based nutrient supplements( Reference Dewey and Adu-afarwuah 35 , Reference De-Regil, Suchdev and Vist 36 ), have shown that these result in significant increases in Hb and ferritin concentrations as well as in a reduced risk of anaemia and ID. However, a few studies have considered alternative, logistically feasible and relatively inexpensive distribution systems and delivery platforms that reduce the economic burden on HH or health systems of already costly products while simultaneously reaching at-risk populations( Reference Olney, Rawat and Ruel 37 ).
A second and complementary strategy is to coordinate with infection prevention and control strategies, given the high burden of subclinical infections evident among young children and its contribution to the development of anaemia. Interventions to reduce the prevalence of anaemia have been justifiably focused on parasitic disease control strategies, particularly malaria and soil-transmitted helminth infections. These interventions should be continued to be implemented. However, a renewed focus on water, sanitation and hygiene interventions is warranted, given the emerging hypothesis that environmental enteropathy is a major cause of child undernutrition and anaemia( Reference Humphrey 38 , Reference Prendergast and Kelly 39 ). This condition, caused by faecal bacteria ingested in large quantities by young children, is characterised by small-intestine inflammation, impaired absorptive capacity and increased intestinal permeability. It is thought to be highly prevalent among infants and young children living in conditions of poor sanitation and hygiene, particularly in developing countries. Therefore, a central tenet of any anaemia prevention and control strategy where water, sanitation and hygiene conditions are suboptimal should also include the promotion of adequate sanitation facilities and hand-washing after faecal contact and before feeding to reduce or prevent the adverse effects of environmental enteropathy on child undernutrition. One of the several indicators used to assess environmental enteropathy includes systemic immune activation, partly measured by the elevated levels of markers of subclinical infection including AGP and CRP, which was prevalent among one in three infants in the present study.
In summary, the burden of anaemia and ID is very high in young rural Bangladeshi infants during the critical transition period of increased physiological Fe requirements corresponding to the early complementary feeding phase between 6 and 11 months of age. Neither dietary nor infection control interventions are sufficient on their own. The increasing prevalence of anaemia and ID during the first 3 months of the complementary feeding period highlights the need to support mothers to introduce Fe supplements or Fe-rich foods or products in their infants' diet as soon as they start giving them complementary foods. Concurrently, the high burden of subclinical infections and its contribution to anaemia and ID highlight the need to include water, sanitation and hygiene and parasitic disease control strategies to control anaemia and ID in this population. Coordination of these two broad strategies is essential if any reductions of anaemia and ID among infants and young children are to be achieved.
Acknowledgements
The present study was funded by the Global Alliance for Improved Nutrition and Alive & Thrive, through a grant from the Bill & Melinda Gates Foundation. The authors' contributions were as follows: P. M. was the principal investigator of the project and designed the study with R. R., K. K. S. and M. R.; R. R. wrote the manuscript and analysed and interpreted the data with A. K.; K. K. S. and F. R. coordinated and supervised the data collection. All authors reviewed multiple versions of the manuscript and provided comments. All authors approved the final manuscript. Conflict of interest statement: at the time of conducting the fieldwork, F. R. was affiliated with Global Alliance for Improved Nutrition, a not-for-profit organisation that supports and promotes infant and young child nutrition programmes. All the other authors have no conflicts of interest to declare.